ABSTRACT
Background
Halitosis refers to malodor emanating from the oral cavity. Several mouthrinses with halitosis-reduction exist on the market, but their effect on the oral microbiome is largely unknown. In this study, we used an efficient in vitro model system to investigate a test mouthrinse's impact on the oral microbiome.
Methods
Single halitosis-associated species and other common oral microorganism cultures were exposed to the test mouthrinse over time, and their viability was determined by culture-based selective plating. Next, the saliva-derived microbiome from healthy and halitosis-associated individuals was cultured in the presence of the test mouthrinse over time using the previously developed in vitro model system. The microbiome composition was assessed with 16S rRNA gene sequencing and downstream bioinformatics analyses.
Results
The test mouthrinse displayed antimicrobial activity against known anaerobic bacterial species producing halitosis-related compounds such as Fusobacterium nucleatum, F. periodonticum, and Prevotella intermedia but not against other common oral microorganisms. In the multispecies, saliva-derived cultures, mouthrinse exposure decreased the relative abundance of the Fusobacterium and Prevotella genera while not affecting overall diversity.
Conclusions
The test mouthrinse had promising anti-halitosis characteristics at the microbiome level, as demonstrated by the reduction in the relative abundance of halitosis-associated taxa while maintaining microbial diversity.
Introduction
Halitosis refers to malodor emanating from the oral cavity [Citation1]. Estimated to affect more than 25% of the population, severe halitosis can be debilitating, resulting in decreased social interactions and overall quality of life [Citation2–4]. Although extra-oral and systemic causes exist, 90% of people suffering from this affliction have halitosis originating in the oral cavity [Citation5]. In addition, oral pathologies such as periodontitis, xerostomia, or mucosal lesions have been associated with malodor [Citation6,Citation7].
The oral cavity consists of complex microbial ecosystems on the teeth, tongue, and saliva. Hundreds of species comprise the oral microbiome, with the vast scope of its functions and contributions to disease recently becoming better understood [Citation8]. Multifactorial in nature, halitosis is caused by the complex microbe–substrate and microbe-microbe interactions and has directly and indirectly been associated with bacteria such as Fusobacterium periodonticum, Fusobacterium nucleatum, Tannerella forsythia, Prevotella intermedia, Porphyromonas gingivalis, and Veillonella atypica [Citation7,Citation9–15]. Additionally, the production of volatile organic compounds by microbial degradation of proteins, peptides, and amino acids has been implicated as a source of malodor, especially the production of volatile sulfur compounds (VSCs) [Citation16,Citation17]. Various bacterial species present in the oral cavity produce VSCs, and many are also associated with oral conditions such as periodontitis [Citation18,Citation19].
Current halitosis treatments generally focus on reducing the overall bacterial growth through antimicrobial components in mouthrinses. Listerine, a mixture of essential oils originally formulated as a surgical antiseptic, is a commonly used mouthrinse [Citation20]. Other antimicrobial mouthrinses such as chlorhexidine (CHX), cetylpyridinium chloride (CPC), and triclosan are used to control oral pathologies, including halitosis, and have been shown to have a marked effect on oral microbes [Citation21,Citation22]. Recently, Therabreath Fresh Breath Rinse (FBR) with an ‘OXYD-8’, a patented formulation of chlorine dioxide, has been marketed as an anti-halitosis mouthrinse, but its efficacy has not been investigated. We hypothesized that this oxidative component may have an impact on the anaerobic halitosis-associated bacteria. While microbial overgrowth contributes to halitosis, the complexity and highly individualized nature of the oral microbial community creates a challenge in identifying a common cause and effective treatment. To date, the effect of halitosis mouthrinse has mainly been performed on single species, but its impact at the microbial community level has not been investigated due to the lack of a suitable experimental model.
In the current study, we investigated the antimicrobial activity of a test mouthrinse (FBR), specifically marketed to improve halitosis against a panel of individual oral microorganisms. By utilizing our laboratory-developed in vitro model system, we demonstrated the effect of the test mouthrinse on saliva-derived oral microbial communities isolated from both healthy volunteers and self-reported halitosis sufferers.
Materials and methods
Ethics
The study was approved by the Institutional Review Board at the University of California, Los Angeles (IRB #13-001075 for pooled saliva samples of healthy subjects and 17–001008 for saliva samples of self-reported halitosis subjects). All participants gave informed consent before participating.
Saliva collection
The healthy saliva community (‘O-mix’) was obtained from pooled saliva samples collected from healthy volunteers. Additionally, saliva was collected from participants with self-reported ‘severe’ halitosis (reported as ‘severely affecting the quality of life’). Participants were recruited and consented via email. Participants were mailed a 50-ml conical tube pre-filled with 75% glycerol with instructions to provide approximately 5 ml of saliva into the tube, seal tightly, and return via express mail to the laboratory. Upon arrival, the saliva samples were aliquoted and stored at −80°C until needed for experiments.
Mouthrinse antimicrobial activity against single species
Monocultures of strains listed in were grown overnight under their respective growth requirements, and subsequently re-inoculated in fresh media, and grown to the exponential phase. At OD600 of 0.8–1.0, cultures were spun down, washed twice, and resuspended in phosphate-buffered saline (PBS), the test mouthrinse (FBR, TheraBreath Fresh Breath Rinse, Los Angeles, CA), or Listerine Cool Mint mouthwash (Johnson & Johnson, Skillman, NJ, USA). After 2, 5, 10-, 20-, 30-, and 60-minutes incubation, 1 mL aliquots were collected, spun down, and washed twice with PBS. Serial dilutions and plating on appropriate agar were performed to count colony-forming units (CFU/mL).
Table 1. Species and strains and their growth conditions
Multispecies salivary community antimicrobial assay
Pooled saliva from healthy volunteers (‘O-mix’) previously collected [Citation23] and saliva samples from three individuals with self-reported halitosis were thawed from −80°C and cultured overnight at 37°C under microaerophilic conditions (2.6% O2, 5% CO2) in SHI media [Citation24]. Cultures were then spun down, washed twice, and resuspended in either PBS, FBR, or Listerine. After 5 minutes and 30 minutes of exposure, aliquots were collected, spun down, washed twice with PBS, resuspended in PBS, and inoculated into 3 ml of fresh SHI media and grown overnight at 37°C under microaerophilic conditions (2.6% O2, 5% CO2), at which time cultures were spun down and immediately frozen until used for DNA extraction.
DNA extraction and 16S microbiome sequencing
DNA was extracted from pelleted cultures using the Epicenter MasterPureTM DNA purification kit (Lucigen, USA) following the manufacturer’s instructions. Briefly, prior to the kit purification, the pellets were subjected to mechanical digestion with glass beads followed by lysozyme treatment for 2 hours at 37°C [Citation23]. Then, DNA quality and quantity were measured by a UV spectrophotometer (NanoDrop 2000, ThermoFisher Scientific) at 260 nm and 280 nm. Purified DNA was stored at −20°C until use.
Sample preparation for sequencing was performed using a previously published [Citation25]. Preparation of sequencing libraries and sequencing were performed at the UCLA Microbiome Core facility. Briefly, 10–50 ng of DNA was used in a PCR reaction with barcoded V3-V4 primers and purified using AMPure beads (Beckman Coulter). One hundred ng of each library was then pooled, gel-purified, and quantified (Bioanalyzer, Agilent), and 12 PhiXpM of the mixture, spiked with 20% PhiX, was run on a MiSeq (Illumina, San Diego, CA).
Bioinformatics and statistical analyses
Sequencing reads were de-multiplexed and adaptor sequences removed. Quality filtering removed bad reads and chimeric sequences prior to analysis. Sequencing data were analyzed using QIIME (Quantitative Insights into Microbial Ecology) version 1.9.1 [Citation25]. Sequences were clustered into operational taxonomic units (OTUs) using UCLUST [Citation26], then aligned and taxonomy assigned with the Human Oral Microbiome Database [Citation27] as reference. Alpha diversity (richness) was estimated by calculating the Shannon Diversity Index GraphPad Prism (GraphPad Prism version 8.0.0, GraphPad Software, San Diego, CA), used for visualizations and descriptive statistics.
Results
The test mouthrinse (FBR) displays antimicrobial activity against halitosis-associated microbes
We first assayed the antimicrobial effect of the test mouthrinse (FBR) on monocultures of a panel of selected oral microbial species (). Species were selected based on their presumed associations to halitosis or periodontitis, and from other common oral bacteria. Cultures were exposed to FBR or saline solution, and aliquots were collected at each time point and then plated for the CFU quantification (, ). FBR exhibited an antimicrobial activity to a subset of the organisms known to be associated with halitosis (). After 2 minutes of FBR exposure, the F. nucleatum and F. periodonticum cultures showed a 95.6% and 82.0% decrease in viability compared to the saline control, respectively. FBR also decreased T. forsythia by 86.5% within 5 minutes and displayed a very rapid effect against P. intermedia which decreased the CFUs by 96.5% after 2 minutes of exposure. On the other hand, FBR showed a more gradual activity against V. atypica, reducing viability by approximately 40% after 10 minutes and reaching 100% killing after 20 minutes. Notably, there was a negligible effect against other common oral microorganisms such as Streptococcus mutans, P. gingivalis, or Candida albicans, and little-to-no effect on Streptococcus species such as Streptococcus sanguinis and Streptococcus gordonii (). As a positive control, cultures were also tested in Listerine brand mouthwash, a known effective antimicrobial mouthrinse [Citation20]. In all tested species, treatment with Listerine led to the rapid killing of 100% of the culture in as little as 5 minutes ().
Table 2. Activity of fresh breath rinse against common oral species in monoculture
Figure 1. The test mouthrinse antimicrobial activity against common oral species in monoculture. Single-species cultures of Fusobacterium periodonticum, F. nucleatum, Prevotella intermedia, Tannerella forsythia, Veillonella atypica, Porphyromonas gingivalis, Streptococcus gordonii, S. sanguinis, S. mutans, and Candida albicans were exposed to the test mouthrinse (FBR, red symbol and line) or phosphate-buffered saline solution (PBS, blue symbol and line) for the indicated amount of time, and viability was assessed by CFUs. The curves represent the means and standard deviation of duplicated samples from two independent experiments. Differences in significance between groups were analyzed using two-way anova; *p < 0.05, **p < 0.005, ***p < 0.0005
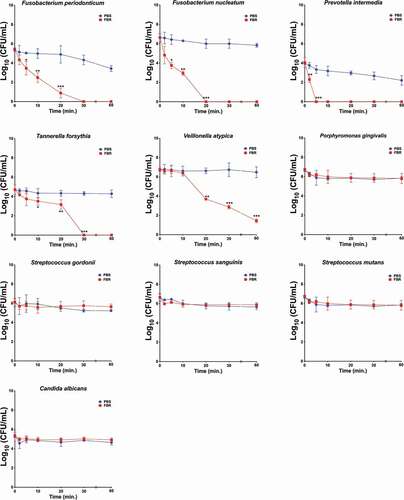
The test mouthrinse (FBR) effect on salivary microbial communities using the modified in vitro model system
To test the effects of the mouthrinse on a representative oral microbial community, pooled saliva from healthy volunteers (‘O-mix’) previously collected [Citation23] and saliva collected from three individuals with self-reported ‘severe’ halitosis (referred to as cultures H1, H2, and H3) were cultured separately in a modified in vitro model system using SHI media, a specialized medium developed by our laboratory that previously was shown to maintain 80% of the original species diversity [Citation24,Citation28].
Similar to the monoculture experiment, saliva-derived cultures were exposed to either saline, the test mouthrinse (FBR), or Listerine. Aliquots were collected after 5 minutes and 30 minutes of exposure. The community composition of the cultures was assessed at each time point using 16S sequencing. To limit DNA from dead cells interfering with the sequencing results, aliquots were re-cultured in fresh media before DNA was extracted for sequencing. We chose treatment times of 5 minutes and 30 minutes to reflect the practical use of the rinse, with 5 minutes representing the combined time of swishing and gargling suggested on the label and 30 minutes as the time suggested to refrain from eating or drinking after rinsing.
The relative abundance of identified taxa at the genus level is shown in . Visual comparison of the stacked bars revealed a shift in the composition of the FBR-treated samples compared to the saline-treated samples. This marked composition shift was more pronounced after 30 minutes of exposure. There was a striking difference between the saline-treated and FBR-treated samples with less identifiable bacterial taxa at 30-minute exposure time for the Listerine-treated samples. Differences in the relative abundance of the genus-level taxa of interest at each time point are shown in . The Fusobacterium and Stomatobaculum genera presented lower abundance in all FBR-treated cultures compared to the saline control. In all halitosis-associated salivary communities, Prevotella was less abundant in the FBR-treated cultures compared to the control. Despite taxa-level changes, alpha diversity (Shannon Index) of the communities was relatively unchanged in cultures exposed to FBR after 5 and 30 minutes compared to the control, while the Listerine-treated cultures had much lower richness compared to FBR-treated cultures after 30 minutes ().
Table 3. Decrease in genus relative abundance after 5 and 30 min exposure to FBR compared to saline control
Figure 2. Microbial composition of saliva-derived cultures. Saliva was first cultured in SHI media and subsequently exposed to the test mouthrinse (FBR), phosphate-buffered saline solution (PBS), or Listerine with aliquots collected 5 min and 30 min after treatment. Aliquots were pelleted and then re-cultured in SHI media overnight before extracting DNA and performing 16S sequencing. The relative abundance (percentage) of the genus-level taxonomic results are shown in the stacked bar graphs. (a) O-Mix; pooled saliva from healthy volunteers was used to generate a healthy community culture. (b-d) H1, H2, and H3 refer to saliva-generated cultures with saliva from individuals with self-reported halitosis
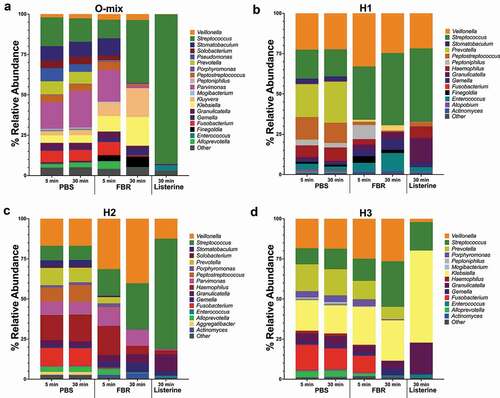
Figure 3. Alpha diversity analysis of saliva-derived microbial communities. The alpha diversity of saliva microbial communities upon treatment with the test mouthrinse (FBR), phosphate-buffered saline solution (PBS), or Listerine (5 min and 30 min after treatment) was analyzed using Shannon index, and the respective rarefaction curves are shown. (a) O-Mix; mixed saliva from healthy volunteers was used to generate a healthy community culture. (b-d) H1, H2, and H3 refer to saliva-generated cultures with saliva from individuals with self-reported halitosis
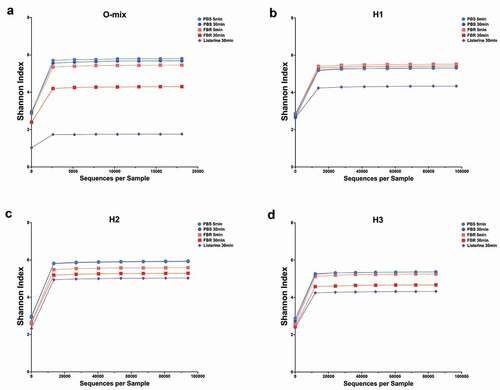
Discussion
The human oral cavity harbors one of the most diverse microbiomes in the human body, consisting of bacteria, archaea, protozoa, fungi, and viruses. This complex community plays a crucial role in oral health and disease and systemic health [Citation8,Citation29]. Microbial dysbiosis may underlie many oral health conditions, including halitosis.
Although numerous products and remedies are marketed to consumers claiming to reduce halitosis, most have not undergone rigorous testing [Citation30,Citation31]. In this study, we sought to develop a method to investigate the effects on the oral microbiome of a test mouthrinse (FBR) specifically marketed for halitosis relief. Using a modified in vitro model system developed previously in our laboratory [Citation24,Citation28], we performed exposure experiments to monitor how the mouthrinse altered the microbial community. We tested ex vivo a pooled ‘healthy’ saliva mix, along with saliva collected from self-reported halitosis sufferers. The results here provide preliminary evidence for more in-depth clinical studies and establish a template for efficient, economical testing for mouthrinses and other oral health care products.
Results from antimicrobial assays against a panel of select oral pathogens, commensals, and known potential contributors to malodor suggest that FBR may specifically target certain halitosis-associated organisms instead of a more generalized antimicrobial activity (). The rinse showed activity against halitosis- and periodontitis-associated organisms such as F. nucleatum [Citation9,Citation15], P. intermedia [Citation10,Citation14], and V. atypica [Citation13] but little-to-no activity against oral species typically regarded as commensals, such as S. sanguinis and S. gordonii () [Citation32,Citation33]. In comparison, the Listerine rinse immediately killed all species tested. We used Listerine as a comparison, as it is widely used and has known antimicrobial activity [Citation20].
As halitosis is a complex phenomenon resulting from the production of volatile compounds from various organisms in different niches of the oral cavity [Citation16,Citation34], considering the entire microbial community when testing therapeutics provides more biologically and practically relevant results than single-species assays. Our results with four different saliva-derived cultures (three individual samples from self-reported halitosis sufferers and one sample with pooled saliva from healthy volunteers) overall suggest that the test mouthrinse does not lead to widespread changes and indiscriminate antimicrobial activity. Instead, the diversity and overall structure of the microbial communities were maintained throughout the treatment duration. In comparison, treatment with Listerine resulted in decimating the community structure and the proliferation of a few taxonomic groups.
We observed important changes in abundance at the genus level (). The relative abundance of Fusobacterium decreased in each culture after 5 minutes of treatment; in one saliva-derived culture from an individual with halitosis, the abundance decreased by 94%. Furthermore, after 30 minutes of exposure, this genus was reduced in all tested saliva communities (). The Fusobacterium genus includes the species F. nucleatum, a known producer of volatile sulfur compounds in the oral cavity and previously shown to be increased in abundance in those exhibiting oral malodor [Citation16,Citation17]. Other taxonomic groups of interest for halitosis detected in the cultures include the genus Porphyromonas, in which we observed a decrease in abundance in three of the four cultures, and Prevotella, which decreased in the cultures derived from halitosis-associated saliva (). The genus Porphyromonas includes P. gingivalis, an important periodontal pathogen, and part of the so-called ‘red complex’ of oral pathogens, and a known producer of VSCs [Citation16,Citation35]. Taken together, these results suggest a possible benefit of this test mouthrinse through targeted reduction of halitosis-associated organisms. In the future, the potential clinically- relevant effect of the mouthrinse should be evaluated in a clinical study.
Previous clinical studies have demonstrated a reduction of the volatile sulfur compounds implicated in halitosis through the use of mouthrinses containing antimicrobial ingredients, such as cetylpyridinium chloride [Citation36] and chlorhexidine (CHX). However, there are a limited number of investigations into the overall effects of these products on the oral microbiome. Still, there is some evidence that CHX use leads to a drastic shift in the salivary microbiome [Citation21]. Thus, although a decrease in the total bacterial number may improve halitosis by reducing the overall levels of odorous compounds, it is unclear if this broad approach may lead to detrimental dysbiosis by promoting drastic shifts in the microbial community. Further clinical studies are needed to determine if a targeted approach can lead to more sustainable results.
The test mouthrinse active ingredient is their proprietary ‘OXYD-8’, a patented formulation of chlorine dioxide. The presumed mechanism of action is the creation of an oxygenated local environment that becomes inhospitable to anaerobes. Our observations support that hypothesis, as the rinse showed more potent antimicrobial activity against anaerobes, such as Fusobacterium species, while was less effective against organisms known to possess mechanisms to reduce reactive oxygen species (ROS), such as P. gingivalis [Citation37], S. mutans [Citation38], and C. albicans [Citation39].
This study aimed to develop an efficient assay and perform a preliminary investigation into the antimicrobial effects of an oral rinse marketed for bad breath. The modified in vitro model system is an efficient first step in generating evidence and establishing the microbiome-focused clinical relevance of a potential therapeutic. In addition to using a mixed saliva sample from healthy volunteers to obtain the oral community cultures, we also recruited individuals with self-reported halitosis as a means of providing additional relevance and generating hypotheses for future clinical testing.
This pilot study was limited by using saliva as the only oral sample type, the self-reported nature of the halitosis phenotype by the individuals in the study, and the inherent limitations of 16S sequencing analysis. The home-based collection method allowed participants to donate saliva samples at home conveniently but limited the collected saliva volume. Nevertheless, comparisons of the microbial composition of saliva with other oral sample types, such as tongue coating, suggest that saliva does indeed contain a sufficient representation of the oral microbiota [Citation40]. We relied on self-reported halitosis experience and cannot be certain of the severity or etiology of each individual’s malodor. Future clinical studies will incorporate halitosis severity and a validated measurement tool to corroborate the individual’s self-report information. Determining the clinical significance of these results will require further investigation in individuals with halitosis.
The results presented here provide evidence that the test mouthrinse has antimicrobial activity against certain halitosis-associated organisms and decreases the abundance of some producers of volatile sulfur compounds within a complex microbial community. Notably, the rinse maintained the microbial diversity of the community while still reducing the abundance of specific taxonomic groups.
Conclusion
In this study, an efficient in vitro assay reflecting the complexity of the oral microbial community was developed to investigate the antimicrobial effects of an oral rinse marketed for halitosis. The test mouthrinse selectively displayed antimicrobial activity against certain halitosis-associated bacteria while maintaining the overall oral microbial community diversity.
Acknowledgments
MD contributed to the design and execution of the experiments, analysis of data, and the drafting of the manuscript. MA contributed to the design and execution of experiments, analysis, and interpretation of data, and the drafting of the manuscript. XE and WS contributed to the conception and design of experiments and critically evaluated the manuscript. NCT contributed to the design of experiments, interpretation of data, and critically evaluated the manuscript. All authors gave their final approval and agreed to be accountable for all aspects of the work.
Disclosure statement
No potential conflict of interest was reported by the author(s).
Additional information
Funding
References
- Renvert S, Noack MJ, Lequart C, et al. The underestimated poblem of intra-oral halitosis in dental practice: an expert consensus review. Clin Cosmet Investig Dent. 2020;12:251–9.
- Bollen CM, Beikler T. Halitosis: the multidisciplinary approach. Int J Oral Sci. 2012;4(2):55–63.
- Kapoor U, Sharma G, Juneja M, et al. Halitosis: current concepts on etiology, diagnosis and management. Eur J Dent. 2016;10(2):292–300.
- Silva MF, Leite FRM, Ferreira LB, et al. Estimated prevalence of halitosis: a systematic review and meta-regression analysis. Clin Oral Investig. 2018;22(1):47–55.
- Campisi G, Musciotto A, Di Fede O, et al. Halitosis: could it be more than mere bad breath? Intern Emerg Med. 2011;6(4):315–319.
- Koshimune S, Awano S, Gohara K, et al. Low salivary flow and volatile sulfur compounds in mouth air. Oral Surg Oral Med O. 2003;96(1):38–41.
- Morita M, Wang HL. Association between oral malodor and adult periodontitis: a review. J Clin Periodontol. 2001;28(9):813–819.
- Dewhirst FE, Chen T, Izard J, et al. The human oral microbiome. J Bacteriol. 2010;192(19):5002–5017.
- Amou T, Hinode D, Yoshioka M, et al. Relationship between halitosis and periodontal disease - associated oral bacteria in tongue coatings. Int J Dent Hyg. 2014;12(2):145–151.
- Grover HS, Blaggana A, Jain Y, et al. Detection and measurement of oral malodor in chronic periodontitis patients and its correlation with levels of select oral anaerobes in subgingival plaque. Contemp Clin Dent. 2015;6(1):S181–7.
- Chukkapalli SS, Rivera-Kweh MF, Velsko IM, et al. Chronic oral infection with major periodontal bacteria Tannerella forsythia modulates systemic atherosclerosis risk factors and inflammatory markers. Patho Dis. 2015;73(3):3.
- Porter SR, Scully C. Oral malodour (halitosis). Bmj-Brit Med J. 2006;333(7569):632–635.
- Donaldson AC, McKenzie D, Riggio MP, et al. Microbiological culture analysis of the tongue anaerobic microflora in subjects with and without halitosis. Oral Dis. 2005;11(Suppl 1):61–63.
- Awano S, Gohara K, Kurihara E, et al. The relationship between the presence of periodontopathogenic bacteria in saliva and halitosis. Int Dent J. 2002;52(5):212–216.
- Apatzidou AD, Bakirtzoglou E, Vouros I, et al. Association between oral malodour and periodontal disease-related parameters in the general population. Acta Odontol Scand. 2013;71(1):189–195.
- Persson S, Edlund MB, Claesson R, et al. The formation of hydrogen-sulfide and methyl mercaptan by oral bacteria. Oral Microbiol Immun. 1990;5(4):195–201.
- Takeshita T, Suzuki N, Nakano Y, et al.Discrimination of the oral microbiota associated with high hydrogen sulfide and methyl mercaptan production. Sci Rep. 2012;2:215.
- Hampelska K, Jaworska MM, Babalska ZL, et al. The role of oral microbiota in intra-oral halitosis. J Clin Med. 2020;9(8):8.
- Foo LH, Balan P, Pang LM, et al. Role of the oral microbiome, metabolic pathways, and novel diagnostic tools in intra-oral halitosis: a comprehensive update. Crit Rev Microbiol. 2021;47(3):359–375.
- Fine DH. Listerine: past, present and future - A test of thyme. J Dent. 2010;38:S2–S5.
- Bescos R, Ashworth A, Cutler C, et al. Effects of Chlorhexidine mouthwash on the oral microbiome. Sci Rep. 2020;10(1):5254.
- Roldan S, Winkel EG, Herrera D, et al. The effects of a new mouthrinse containing chlorhexidine, cetylpyridinium chloride and zinc lactate on the microflora of oral halitosis patients: a dual-centre, double-blind placebo-controlled study. J Clin Periodontol. 2003;30(5):427–434.
- Agnello M, Cen L, Tran NC, et al. Arginine improves pH homeostasis via metabolism and microbiome modulation. J Dent Res. 2017;96(8):924–930.
- Tian Y, He X, Torralba M, et al. Using DGGE profiling to develop a novel culture medium suitable for oral microbial communities. Mol Oral Microbiol. 2010;25(5):357–367.
- Caporaso JG, Kuczynski J, Stombaugh J, et al. QIIME allows analysis of high-throughput community sequencing data. Nat Methods. 2010;7(5):335–336.
- Edgar RC. Search and clustering orders of magnitude faster than BLAST. Bioinformatics. 2010;26(19):2460–2461.
- Chen T, Yu W-H, Izard J, et al. The human oral microbiome database: a web accessible resource for investigating oral microbe taxonomic and genomic information. Database. 2010;baq013.
- Edlund A, Yang Y, Hall AP, et al. An in vitro biofilm model system maintaining a highly reproducible species and metabolic diversity approaching that of the human oral microbiome. Microbiome. 2013;1(1):25.
- Baker JL, Bor B, Agnello M, et al. Ecology of the oral microbiome: beyond bacteria. Trends Microbiol. 2017;25(5):362–374.
- ADA Council on Scientif Affairs. Oral malodor. J Am Dent Assoc. 2003;134(2):209–214.
- Slot DE, De Geest S, Van Der Weijden FA, et al. Treatment of oral malodour. Medium-term efficacy of mechanical and/or chemical agents: a systematic review. J Clin Periodontol. 2015;42(Suppl 16):S303–16.
- Abranches J, Zeng L, Kajfasz JK, et al. Biology of oral Streptococci. Microbiol Spectr. 2018;6(5):5.
- Zhu B, Macleod LC, Kitten T, et al. Streptococcus sanguinis biofilm formation & interaction with oral pathogens. Future Microbiol. 2018;13(8):915–932.
- Sterer N, Rosenberg M. Streptococcus salivarius promotes mucin putrefaction and malodor production by Porphyromonas gingivalis. J Dent Res. 2006;85(10):910–914.
- Mineoka T, Awano S, Rikimaru T, et al. Site-specific development of periodontal disease is associated with increased levels of Porphyromonas gingivalis, Treponema denticola, and Tannerella forsythia in subgingival plaque. J Periodontol. 2008;79(4):670–676.
- Feres M, Figueiredo LC, Faveri M, et al. The efficacy of two oral hygiene regimens in reducing oral malodour: a randomised clinical trial. Int Dent J. 2015;65(6):292–302.
- Henry LG, McKenzie RM, Robles A, et al. Oxidative stress resistance in Porphyromonas gingivalis. Future Microbiol. 2012;7(4):497–512.
- Yamamoto Y, Higuchi M, Poole LB, et al. Role of the dpr product in oxygen tolerance in Streptococcus mutans. J Bacteriol. 2000;182(13):3740–3747.
- Frohner IE, Bourgeois C, Yatsyk K, et al. Candida albicans cell surface superoxide dismutases degrade host-derived reactive oxygen species to escape innate immune surveillance. Mol Microbiol. 2009;71(1):240–252.
- Suzuki N, Yoneda M, Takeshita T, et al. Induction and inhibition of oral malodor. Mol Oral Microbiol. 2019;34(3):85–96.
- Loesche WJ. Role of Streptococcus mutans in human dental decay. Microbiolo Rev. 1986;50(4):353–380.
- Barbeau J, Séguin J, Goulet JP, et al. Reassessing the presence of Candida albicans in denture-related stomatitis. Oral Surg Oral Med OralPathol Oral Radiol Endod. 2003;95(1):51–59.