ABSTRACT
Background: Trauma-focused treatments for post-traumatic stress disorder (PTSD) are effective for many patients. However, relapse may occur when acquired extinction memories fail to generalize beyond treatment contexts. A subgroup of PTSD patients – potentially with substantial exposure to early-life adversity (ELA) – show dysregulation of the hypothalamic–pituitary–adrenal (HPA) axis, which results in lower cortisol levels. Glucocorticoids, including cortisol, appear to facilitate strength and generalization of emotional memories.
Objective: We describe the protocol of an integrated PTSD study. We investigate (A) associations between HPA-axis dysregulation, ELA, epigenetic markers, and PTSD treatment outcome (observational study); and (B) effects of exogenous glucocorticoids on strength and generalization of extinction memories and associated neural mechanisms [pharmacological intervention study with functional magnetic resonance imaging (fMRI)]. The objective is to provide proof of concept that PTSD patients with HPA-axis dysregulation often experienced ELA and may show improved strength and generalization of extinction learning after glucocorticoid administration.
Method: The observational study (n = 160 PTSD group, n = 30 control group) assesses ELA, follow-up PTSD symptoms, epigenetic markers, and HPA-axis characteristics (salivary cortisol levels during low-dose dexamethasone suppression test and socially evaluated cold-pressor test). The pharmacological intervention study (n = 80 PTSD group, with and without HPA-axis dysregulation) is a placebo-controlled fMRI study with a crossover design. To investigate strength and generalization of extinction memories, we use a differential fear acquisition, extinction, and extinction recall task with spatial contexts within a virtual environment. Prior to extinction learning, 20 mg hydrocortisone or placebo is administered. During next-day recall, strength of the extinction memory is determined by recovery of skin conductance and pupil dilation differential responding, whereas generalization is assessed by comparing responses between different spatial contexts.
Conclusion: The integrated study described in the current protocol paper could inform a personalized treatment approach in which these PTSD patients may receive glucocorticoids as a treatment enhancer in trauma-focused therapies.
Trial registration: The research project is registered in the European Union Drug Regulating Authorities Clinical Trials (EudraCT) database, https://eudract.ema.europa.eu/, EudraCT number 2020-000712-30.
HIGHLIGHTS
This protocol reports a proof-of-concept study for glucocorticoids as an enhancer for PTSD treatment.
The study examines whether glucocorticoids enhance the strength and generalization of extinction memory.
A further aim is to identify HPA-axis-related PTSD subgroups that may particularly benefit.
Antecedentes: Los tratamientos centrados en trauma para el trastorno de estrés postraumático (TEPT) son efectivos para muchos pacientes. Sin embargo, las recaídas pueden ocurrir cuando la extinción de los recuerdos adquiridos no logra generalizarse más allá de los contextos del tratamiento. Un subgrupo de pacientes con TEPT, potencialmente con una exposición importante a adversidades tempranas en la vida (ELA por sus siglas en inglés), muestran desregulación del eje hipotálamo-hipófisis-adrenal (HHA), lo cual resulta en niveles de cortisol más bajos. Los glucocorticoides, incluyendo el cortisol, parece facilitar la fuerza y generalización de los recuerdos emocionales.
Objetivo: Aquí describimos el protocolo de un estudio de TEPT integrado. Investigamos (A) asociaciones entre la desregulación del eje HHA, ELA, marcadores epigenéticos y resultados del tratamiento del TEPT (estudio observacional); y (B) los efectos de los glucocorticoides exógenos sobre la fuerza y generalización de la extinción de los recuerdos y los mecanismos neurales asociados (estudio de intervenciones farmacológicas con imágenes de resonancia magnética funcional [fMRI]). El objetivo es proporcionar prueba de concepto de que los pacientes con TEPT con desregulación del eje HHA con frecuencia han experimentado ELA y pueden mostrar una mayor fuerza y generalización del aprendizaje de extinción tras la administración de glucocorticoides.
Métodos: El estudio observacional (n=160 grupo TEPT, n=30 grupo control) evalúa ELA, seguimiento de los síntomas de TEPT, marcadores epigenéticos y características del eje HHA (niveles de cortisol en saliva durante el test de supresión de dexametasona en bajas dosis y la prueba de estimulación por frio evaluada socialmente). El estudio de intervención farmacológica (n=80 grupo TEPT, con y sin desregulación del eje HHA) es un estudio de fMRI controlado con placebo con diseño cruzado. Para investigar la fuerza y generalización de la extinción de los recuerdos, utilizamos una tarea de adquisición del miedo, extinción y recuerdo de extinción diferencial con contextos espaciales dentro de un entorno virtual. Previo al aprendizaje de extinción, se administra 20 mg. de hidrocortisona o placebo. Durante el recuerdo del siguiente día, la fuerza de la extinción del recuerdo está determinada por la recuperación de la conductancia táctil y la respuesta diferencial de la dilatación pupilar, mientras que la generalización se evalúa comparando respuestas entre diferentes contextos espaciales.
Conclusión: El estudio integrado descrito en el artículo del protocolo actual podría informar de una aproximación de tratamiento personalizado en el que los pacientes con TEPT podrían recibir glucocorticoides como potenciador del tratamiento en terapia centradas en trauma.
1. Introduction
Post-traumatic stress disorder (PTSD) is a common and debilitating psychiatric disorder that may develop after experiencing or witnessing one or more severely distressing and life-threatening events (American Psychiatric Association, Citation2013). The disorder is characterized by persistent and intrusive flashbacks, recurring nightmares or upsetting memories, avoidance, negative feelings, and changes in arousal and reactivity (e.g. difficulty concentrating, sleep problems, irritability, and/or hypervigilance) (American Psychiatric Association, Citation2013). First-line treatments for PTSD are trauma-focused psychotherapies, for example, prolonged exposure and eye movement desensitization reprocessing (Rousseau et al., Citation2019).
An important common factor of these treatments is that they are based on the extinction of conditioned fear by re-exposure to trauma-related memories. During exposure therapy (i.e. all forms of exposure-based trauma-focused treatment), patients are exposed to fear-provoking situations and activities in order to promote extinction of the fear response as well as learning of safety signals. This involves emotional learning, since new and adaptive safety memories are created that compete with maladaptive aversive memories (Ressler & Mayberg, Citation2007). If successful, recall of safety memories prevents the expression of traumatic memories. Exposure therapy is evidence based and generally effective for PTSD (Bradley et al., Citation2005; Cusack et al., Citation2016; Ehring et al., Citation2014; Hoffman et al., Citation2018), but not all patients achieve complete remission with these treatments. Failure to inhibit excessive fear and the inability to learn safety are hallmarks of PTSD and other fear-related disorders and contribute to non-response (Schottenbauer et al., Citation2014; Wessa & Flor, Citation2007; Wicking et al., Citation2016). In addition, return of fear is another well-recognized problem (Bandelow et al., Citation2007; Bouton et al., Citation2012). Exposure therapy is therefore insufficiently effective in the long term for around 30–50% of PTSD patients (Bradley et al., Citation2005; Cusack et al., Citation2016). This indicates the need to investigate innovative interventions that may enhance treatment success in non-responder and relapsing subgroups of PTSD patients, paving the way for a personalized treatment approach.
However, delineation of PTSD subgroups into meaningful subgroups that can provide information about treatment trajectories remains a challenge. Individuals with ‘complex PTSD’ can experience a more chronic course that often links back to substantial exposure to early-life adversity (ELA), for instance, chronic abuse or neglect (World Health Organization, Citation2018). In accordance with clinical observations, some previous studies suggests that individuals with ELA more often show less favourable PTSD treatment outcome (Bosch et al., Citation2020; Dorrepaal et al., Citation2014; Hoeboer et al., Citation2021). Other studies indicate that this subgroup generally responds well, albeit with lower effect size (Ehring et al., Citation2014; Wagenmans et al., Citation2018). Here, individual differences in treatment outcome may be more directly related to the adaptation of certain biological systems in response to chronic ELA. Previous research suggests that ELA may play an important role with regard to hypothalamic–pituitary–adrenal (HPA)-axis dysregulation in PTSD (Houtepen et al., Citation2016; Pitman et al., Citation2012; Zoladz & Diamond, Citation2013). Altered HPA-axis regulation could consequently affect the development of brain structure and functioning (Raymond et al., Citation2018). Neurobiological variation in fear-regulatory neural circuits that affect emotional learning may predict individual differences in exposure therapy treatment outcome. These factors may provide stress- and fear-related biological markers that could identify non-responder and relapsing individuals with PTSD and corresponding targets to develop more personalized PTSD treatments.
In PTSD, enhanced HPA-axis feedback regulation can lead to exaggerated suppression of cortisol, which results in lower circulating cortisol levels (Goenjian et al., Citation1996; Grossman et al., Citation2003; Pitman et al., Citation2012; Stein et al., Citation1997; Yehuda, Citation2009; Yehuda et al., Citation1993, Citation1995, Citation2002, Citation2004). Although most meta-analyses support this notion of HPA-axis dysregulation (Meewisse et al., Citation2007; Morris et al., Citation2012; Pan et al., Citation2018), another meta-analysis (Klaassens et al., Citation2012) and multiple studies that failed to replicate these findings suggest that low cortisol may only be observed under certain conditions, perhaps only within subgroups of individuals with PTSD (Meewisse et al., Citation2007; Pitman et al., Citation2012; Zoladz & Diamond, Citation2013). DNA methylation can suppress gene transcription and, as such, contribute to individual differences in glucocorticoid signalling (Turecki & Meaney, Citation2016; Zhang et al., Citation2013). Previous research indicated that decreased methylation of the glucocorticoid receptor (GR) gene NR3C1 is associated with ELA (Oberlander et al., Citation2008; Parent et al., Citation2018; Weaver et al., Citation2007). Moreover, allele-specific DNA demethylation of FKBP5 (a co-chaperone that regulates GR activity) mediates gene-by-ELA interactions and induces long-term HPA-axis dysregulation (Klengel et al., Citation2013; Koenig et al., Citation2018; Mehta et al., Citation2011). HPA-axis dysregulation and methylation status of NR3C1 and FKBP5 are also associated with increased lifetime risk for PTSD development if exposed to traumatic events (Labonté et al., Citation2014; van Zuiden et al., Citation2011; Vukojevic et al., Citation2014; Yehuda et al., Citation2015b). Beyond these observational findings, experimental research with rodents has provided causal support that ELA can lead to HPA-axis dysregulation. ELA was shown to cause lower glucocorticoid levels, which was accompanied by an upregulation of GRs within the hippocampus and medial prefrontal cortex and decreased later-life social behaviour, of which the latter was reinstated with glucocorticoid administration (Perry et al., Citation2019). Gene expression patterns thus tune the sensitivity of the stress response and negative feedback inhibition via epigenetic mechanisms.
Dysregulation of glucocorticoid signalling could play a key role in psychopathology and highlights the promise of pharmacological treatment with glucocorticoids, specifically also given the role of glucocorticoids in emotional memory: experimental studies in healthy participants have demonstrated that elevated cortisol levels caused by glucocorticoid treatment or stress induction before the extinction of fear memory strengthen the consolidation of safety memory (Drexler et al., Citation2017; Drexler et al., Citation2018; Merz et al., Citation2018). These findings appear to translate to clinical populations of PTSD patients (Inslicht et al., Citation2021), as well as to actual exposure therapy treatment settings (Meuret et al., Citation2016; Suris et al., Citation2010; Yehuda et al., Citation2010). In a first randomized controlled pilot study, which included 24 veterans affected by PTSD, clinical improvement after hydrocortisone augmentation of exposure therapy was associated with greater glucocorticoid sensitivity at baseline (Yehuda et al., Citation2015a). Importantly, recent work with rodents suggests that glucocorticoids not only enhance the strength of safety memory, but also increase its generalization to other contexts (Roozendaal & Mirone, Citation2020). A study including healthy males correspondingly indicated that stress induction before extinction learning enhanced the context independence of safety memory (Drexler et al., Citation2018). These findings show additional potential for glucocorticoids to enhance trauma-focused therapy outcome, as it is common for PTSD patients to experience the return of fear and relapse outside the therapeutic context.
Glucocorticoids such as cortisol provide a mechanism for enhancing extinction processes by acting on brain regions that play key roles in the emotional modulation of memory processes (de Quervain et al., Citation2017; Roozendaal & McGaugh, Citation2011). Previous studies have suggested that activation of the dorsal anterior cingulate cortex and anterior insular cortex – brain regions importantly associated with fear expression – at late extinction may indicate incomplete extinction of fear memory, whereas activations in the dorsolateral prefrontal cortex and ventromedial prefrontal cortex – brain regions importantly associated with fear extinction – would indicate a regulatory response that suppresses the expression of fear (Fullana et al., Citation2018; Picó-Pérez et al., Citation2019). Of note, effective extinction learning may also be signalled by patterns of functional connectivity between brain networks (Wen et al., Citation2022). Individuals with PTSD show increased activation in brain regions associated with fear expression and in the hippocampus, corresponding to the notion of weaker and more context-specific extinction learning (Suarez-Jimenez et al., Citation2019). Higher activations in fear-related networks and lower activations in the dorsolateral prefrontal cortex during emotion regulation tasks were also found to be predictive of poorer PTSD treatment outcome (Fonzo et al., Citation2017; Rooij et al., Citation2016). In correspondence, targeting prefrontal areas with transcranial magnetic stimulation can improve extinction learning outcomes (Raij et al., Citation2018).
Collectively, the aforementioned findings suggest a mechanistic model in which PTSD patients with dysfunctions in glucocorticoid signalling – potentially emerging from epigenetic programming by ELA – insufficiently benefit from PTSD treatments. This group of non-responding and relapsing individuals could potentially benefit from exogenous glucocorticoid administration during extinction learning (De Quervain et al., Citation2019). The current study integrates an observational study with a pharmacological intervention and functional resonance imaging (fMRI) study to provide a translational effort towards improved and personalized PTSD treatment. In our observational study, we investigate interaction effects of ELA and epigenetic programming of glucocorticoid signalling on HPA-axis regulation and their association with treatment outcome in a group of individuals with PTSD. The pharmacological intervention and fMRI study investigates glucocorticoid-induced enhancement of extinction learning in PTSD patients in a placebo-controlled clinical trial with a crossover design. By leveraging an experimental fear conditioning and extinction task within a virtual environment that uses houses as spatial contexts for the experiences, we will be able to investigate the effect of exogenous glucocorticoids on the context dependency of fear and extinction memories. Overall, we aim to provide proof of concept that HPA-axis dysregulation in individuals with PTSD is related to ELA and associated epigenetic markers of glucocorticoid signalling; that these individuals with PTSD show impairments in extinction learning and altered neural responses; and that this subgroup would particularly benefit from glucocorticoid augmentation of extinction learning through a mechanism of enhancing the strength and generalization of extinction memories.
1.1. Research objectives
Objectives of the observational study:
Replicate findings of HPA-axis dysregulation in PTSD patients relative to a control group of individuals without PTSD or history of early-life trauma.
Investigate associations between history of ELA and HPA-axis dysregulation.
Investigate whether epigenetic markers of glucocorticoid signalling (such as methylation status of glucocorticoid-related genes) mediate the association between history of ELA and HPA-axis dysregulation.
Exploratively, investigate whether HPA-axis dysregulation and glucocorticoid signalling are associated with treatment response.
Objectives of the pharmacological intervention study:
Investigate whether patients with HPA-axis dysregulation show impairments in extinction learning relative to patients without HPA-axis dysregulation, and whether this is accompanied by different neural responses during extinction learning in brain regions associated with fear expression and extinction.
Investigate whether the subgroup of PTSD patients with HPA-axis dysregulation particularly benefits from hydrocortisone administration (versus placebo) to improve the strength and generalization of extinction memories.
Investigate neural responses during extinction learning in brain regions associated with fear expression and extinction after hydrocortisone administration (versus placebo), that may be associated with improvements in strength and generalization of extinction learning.
2. Method
2.1. Design
This study consists of two integrated parts (). The first part, referred to as ‘part A’, is an observational study investigating HPA-axis characteristics. The second part, referred to as ‘part B’, is a randomized placebo-controlled pharmacological intervention and fMRI study with a crossover design, investigating the effect of hydrocortisone administration on extinction learning within a differential fear acquisition, extinction, and extinction recall task. Participants with PTSD can either participate in the entire study – that is, both parts, preferably sequentially, but alternatively in parallel – or only in part A. These PTSD group participants are recruited from collaborating mental healthcare institutions and the general population. A healthy, matched control group without exposure to early-life trauma is recruited from the general population and only participates in part A.
Figure 1. Overview of study procedures. This study consists of two integrated parts. For some participants, the order of study visits is changed to reduce the total duration of participation; these participants complete study visits B1.1 and B1.2 prior to study visits A1 and A2. *Only post-traumatic stress disorder (PTSD) group participants recruited from the general population complete a screening visit; participants recruited from one of the collaborating mental healthcare institutions are included based on a (suspected or preliminary) diagnosis of PTSD for which they received an indication for trauma-focused treatment. APM = Advanced Progressive Matrices; CAPS-5 = Clinician-Administered PTSD Scale for DSM-5; MINI = Mini International Neuropsychiatric Interview; MACE = Maltreatment and Abuse Chronology of Exposure; DST = dexamethasone suppression test; SECPT = socially evaluated cold-pressor test; fMRI = functional magnetic resonance imaging.
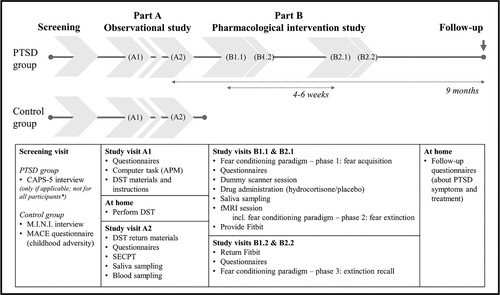
2.2. Population
This study aims to include n = 160 people with PTSD (part A), half of whom participate in the entire study (n = 80 for part B). In addition, n = 30 control group participants are recruited (part A). All participants must be aged 18–64 years old. Exclusion criteria for both groups are listed in . More details on criteria assessed during the screening visit and amendments during the trial are listed in the Supplementary Material (S1 and S2). Power calculations are also described in the Supplementary Material (S3).
Table 1. Exclusion criteria.
2.2.1. PTSD group
When recruited from one of the collaborating mental healthcare institutions, the participant should have received a (suspected or preliminary) diagnosis of PTSD for which they received an indication for trauma-focused treatment. Alternatively, participants should have previously received a diagnosis of PTSD and currently still experience considerable PTSD symptoms; current PTSD is then verified by means of a structured clinical interview. The aim is to achieve a prespecified gender distribution, with an acceptable (dis)balance ranging from 40–75% women; that is, 25–60% men and other gender identities. This range is asymmetrical given the disbalance in PTSD prevalence and because of hormonal contraceptive use by women, which may be investigated as a confounder variable.
2.2.2. Control group
The control group is screened for having no history of psychiatric disorders, no history of childhood trauma or maltreatment, and no current use of psychotropic medication (for details see Supplementary Material S1). Control participants will be selected based on age, gender, and education, with the aim of matching the distributions of these demographic variables to the (expected) distributions within the PTSD group.
2.3. Pharmacological intervention
In part B of the study, to investigate glucocorticoid effects on extinction learning and brain activity, participants ingest 20 mg hydrocortisone prior to extinction learning in one session, and placebo prior to extinction learning in the other session. The order of medication administration is double-blind and counterbalanced across participants (for details see Supplementary Material S4).
2.4. Materials
2.4.1. Clinical and questionnaire assessments
2.4.1.1. Classification of PTSD (PTSD group)
PTSD group participants who are invited by a clinician from a collaborating mental healthcare institution are included based on clinical judgement and according to local organizational methods of PTSD classification (without additional verification within the current study). However, for participants in the PTSD group from the general population, classification of PTSD is assessed with the Dutch version of the Clinician-Administered PTSD Scale for DSM-5 (CAPS-5), which is a structured clinical interview for PTSD (Boeschoten et al., Citation2018).
2.4.1.2. Screening for psychiatric disorders (control group)
In the control group, current psychiatric disorders are screened for with the Dutch translation of the Mini International Neuropsychiatric Interview (MINI) version 5, a structured clinical interview that is based on the Diagnostic and Statistical Manual of Mental Disorders, 4th Edition (DSM-IV) (Sheehan et al., Citation1998).
2.4.1.3. PTSD symptoms
PTSD symptoms are assessed with the Dutch version of the PTSD Checklist for DSM-5 (PCL-5), a 20-item self-report questionnaire that assesses symptoms in the past month according to the Diagnostic and Statistical Manual of Mental Disorders, Fifth Edition (DSM-5) (Blevins et al., Citation2015; Boeschoten et al., Citation2014).
2.4.1.4. Childhood trauma
Childhood traumatic experiences are assessed with the Dutch translation of Maltreatment and Abuse Chronology of Exposure (MACE), a 52-item self-report questionnaire that assesses exposure to 10 types of childhood trauma and neglect, as well as the ages of exposure (Teicher & Parigger, Citation2015).
2.4.1.5. Other questionnaires
All clinical and questionnaire assessments are described in the Supplementary Material (S5 and S6).
2.4.2. Biological materials
2.4.2.1. Saliva sampling
Saliva is sampled using Salivette collection devices (Sarstedt, Rommelsdorf, Germany). Each sample is stored at −20°C until biochemical analysis of cortisol levels.
2.4.2.2. Blood sampling
For assessment of DNA methylation levels and genotyping, blood is drawn into one (10 ml) K2EDTA-containing tube. In addition, blood is drawn into two (2.5 ml) PAXgene tubes for determination of mRNA levels. The samples are cooled at +4°C for approximately 45 minutes, then frozen at –20°C for 12–24 hours (overnight) and then stored at –80 to –70°C for long-term storage.
2.4.3. Dexamethasone suppression test
The low-dose dexamethasone suppression test (DST) measures suppressibility of the HPA axis caused by GR-mediated negative feedback by the administration of 0.5 mg dexamethasone (de Kloet et al., Citation2006). Participants collect saliva samples at waking time and 15, 30, 45, and 60 minutes and 8 hours after waking time on two days at home. In this study, dexamethasone is taken orally at 11 p.m. (23:00 h) on the evening before the second day of the test. Additional instructions ensure the quality of the saliva sample (for details, see Supplementary Material S6). More detailed information on instructional materials and procedures is provided in the Supplementary Material (S7).
2.4.4. Socially evaluated cold-pressor test
The socially evaluated cold-pressor test (SECPT) is a validated stress paradigm that induces robust increases in subjective stress ratings, blood pressure, and salivary cortisol levels (Schwabe et al., Citation2008; Schwabe & Schächinger, Citation2018). The SECPT protocol was adapted after consultation with our advice committee of experts – people with personal experience of PTSD – not to include a camera set-up, as this was considered too stressful for certain (social anxiety) subpopulations. For details about its implementation in the current study, see the Supplementary Material (S8).
2.4.5. Fear conditioning and extinction task
To assess the strength and generalization of safety learning within an experimental setting, the current study employs a 2 day differential fear acquisition, extinction, and extinction recall task in a virtual environment (VE) with contexts as occasion setters (). This task was adapted from previous studies and subsequently validated in a study with healthy young adults (de Nooij et al., Citation2021; de Voogd et al., Citation2020; Wirz et al., Citation2021). The task consists of two different VEs (natural scene and city scene) to allow repeated measurements within the crossover design of this study. Contexts for experiences are defined by three different houses within each VE. The conditioned stimuli (CSs) are simple geometric shapes and the unconditioned stimulus (US) is an electric shock to the fingers. The task consists of three phases: (1) fear acquisition, (2) fear extinction, and (3) recall. In each phase, the participant navigates within the three-dimensional VE using a joystick. After navigating to one of the houses, the participant is presented with a trial block of a two-dimensional task. During each trial, the participant provides a shock expectancy rating (SER) on a scale from 1 (‘certainly no shock’) to 5 (‘certainly a shock’). Primary outcomes consist of fear-related measures of autonomic nervous system response, particularly the skin conductance response (SCR) and pupil dilation response (PDR); these are assessed during each phase of the task. Further details on the task, experimental set-up, and counterbalancing are outlined in the Supplementary Material (S9).
Figure 2. Fear conditioning and extinction task. (a) The task consists of a fear acquisition, fear extinction, and extinction recall task. In this study, hydrocortisone or placebo is administered 45–55 minutes prior to the fear extinction task. This fear extinction task is completed during functional magnetic resonance imaging (fMRI) scanning. Outcome measures are psychophysiological measurements (pupil, skin conductance) and shock expectancy ratings during all tasks, and neuroimaging during the fear extinction task. (b) To accommodate the crossover design of this study, the task has two virtual environments (nature and city environment) with three houses each. These houses function as occasion setters, i.e., ‘contexts’ for the experiences (acquisition, extinction, novel). (c) Task procedures to start a task block. The participant is first instructed to enter a booth. Inside this booth, they are presented with the house to which they should navigate. They should also rate on a five-point scale whether they expect to receive at least one shock within that house in the upcoming block. Entering a booth within the correct house triggers the start of a block. (d) Task procedures within a block, per trial. The participant is presented with a fixation cross before presentation of a conditioned stimulus (CS). During CS presentation, they should rate on a five-point scale whether they expect to receive a shock based on the stimulus that is being presented. At the end of stimulus presentation, depending on the task and trial, negative reinforcement with an electric shock to the fingers may follow. (e) Two sets of three shapes that function as CSs within the paradigm.
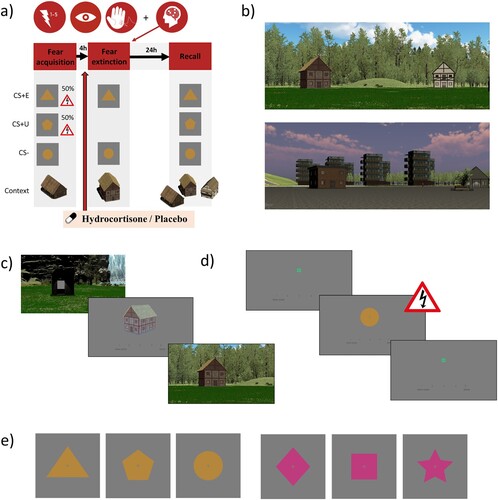
2.4.5.1. Task phases
Fear acquisition. Three different CSs are presented in four blocks. Each block consists of four trials per CS, totalling to 12 trials per block. One CS is never followed by shock (CS−), whereas two other CSs (CS+s) are sometimes followed by shock according to a 50% reinforcement schedule. All blocks are completed within the same house; this house is established as the ‘acquisition context’.
Fear extinction. The CS− and one of the two CS+s are presented in four blocks. Each block consists of four trials per CS, totalling to eight trials per block. Here, the included CS+ is being extinguished (CS+E) as no shocks are administered during this phase. The CS+ that is omitted from this phase remains unextinguished (CS+U). All blocks are completed within the same house, differently from the fear acquisition phase; this house is established as the ‘extinction context’.
Extinction recall. All three CSs (CS+E, CS+U, and CS−) are presented in six blocks. Each block consists of two trials per CS, totalling to six trials per block. The first two blocks are presented within the ‘extinction context' house, the next two blocks within the ‘novel context' house, and the last two blocks within the ‘acquisition context' house.
2.4.5.2. Practice tasks
In relation to the fear conditioning and extinction task, two practice tasks were developed: one to practise navigation with the joystick and for familiarization with the VE, and one to practise giving scores with the joystick and more generally to practise the required course of actions (for details, see Supplementary Material S9).
2.4.6. Neuroimaging
Details of magnetic resonance imaging (MRI) data acquisition are described in the Supplementary Material (S10).
2.4.6.1. Task-based fMRI: fear extinction
Neural responses associated with extinction learning are assessed during the fear extinction task, as previously described.
2.4.6.2. Task-based fMRI: emotion processing
Neural responses during implicit emotion processing are assessed using an adaptation of an emotional face matching task (Hariri et al., Citation2002). The current task version was implemented by the DynaMORE consortium (for details, see Wackerhagen et al., Citation2023). In short, grey-scale faces with angry and fearful expressions that should be matched are presented during the emotion condition, whereas in the shape condition, geometric shapes (circles, horizontal ellipses, and vertical ellipses) that should be matched are presented. The task consists of eight blocks of six trials that alternate between conditions.
2.4.6.3. Resting-state fMRI
For resting-state scans, participants are presented with a fixation cross (white cross on black background). They are instructed to keep their eyes open and have their thoughts flow freely, but refrain from repetitive mental activities such as counting.
2.5. Procedure
Additional information on study procedures can be found in the Supplementary Material (S11).
2.5.1. Recruitment
Participants from the PTSD group are recruited via Pro Persona and Kairos, which are collaborating mental healthcare institutions located in and around Nijmegen. In addition, following approval of a study amendment, participants from the PTSD group are also recruited from the general population via (social media) advertisements, distribution of posters and flyers, and personal and professional networks. PTSD group participants are directed to a study website with short informational videoclips and other information. They are personally contacted and complete a telephone screening to assess inclusion and exclusion criteria. Participants from the control group are recruited from the general population via (social media) advertisements and distribution of posters and flyers, and receive information and register via a study website for the control group.
2.5.2. Participant inclusion
All participants provide informed consent at their first visit. This is followed by verification and registration of inclusion and exclusion criteria, and collection of general information.
2.5.3. Screening visit
2.5.3.1. Screening visit for PTSD group
The screening visit only applies to PTSD patients who were recruited from the general population. Based on the preference of the participant and feasibility, this visit can be scheduled on location or as an online visit. During this visit, an online questionnaire and CAPS-5 clinical interview are administered to the participant. The participant is immediately informed about inclusion or exclusion in this study, depending on whether they meet classification criteria for PTSD or not.
2.5.3.2. Screening visit for control group
The participant completes the MACE as an online questionnaire, followed by a MINI assessment. Based on their results, the participant is immediately informed about inclusion in or exclusion from this study (for details, see Supplementary Material S1).
2.5.4. Observational study (Part A)
2.5.4.1. First visit (A1)
During this visit, the participant completes MACE (if not previously completed) and several other online questionnaires/assessments. Subsequently, they receive materials and in-person instructions for the DST procedure (for details, see Supplementary Material S7).
2.5.4.2. Second visit (A2)
This visit is scheduled in in the afternoon [SECPT from 1 p.m. (13:00 h)] to avoid any influence of higher morning cortisol levels. The researcher receives the materials for the DST procedure from the participant and evaluates the procedure. Then, the participant completes the PCL-5 and several other online questionnaires before participating in the SECPT procedure. Here, saliva samples are collected at 15, 30, 45, 60, 75, and 90 minutes after the onset of the stress procedure. Lastly, venous blood is sampled by a trained research nurse at the Radboud University Medical Center.
2.5.5. Pharmacological intervention and fMRI study (Part B)
Owing to the crossover design, this study part consists of two sessions that are scheduled with a 4–6 week interval. The sessions are identical, except for the type of drug administered (hydrocortisone or placebo) and the version of the fear conditioning and extinction task (different VE with different set of CSs). Each session includes two visits to the Donders Centre for Cognitive Neuroimaging on subsequent days (). Before each visit, participants receive instructions for quality assurance of data (for details, see Supplementary Material S6).
Figure 3. Timeline of study procedures per session in the pharmacological intervention and functional magnetic resonance imaging (fMRI) study. The first study visit typically starts around 10 or 11 a.m. (10:00 or 11:00 h), and the next-day visit typically starts around 3.15 or 4.15 p.m. (15:15 or 16:15 h).
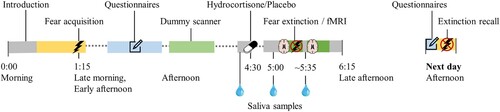
2.5.5.1. MRI visit (B1.1 and B2.1)
In the psychophysiology laboratory, the participant completes the two practice tasks (see Section 2.4.5) in the presence of the researcher. If more practice is needed, a practice test is repeated. The intensity of electric shocks is determined by a calibration procedure (for details, see Supplementary Material S9). The participant then performs the fear acquisition task with psychophysiological assessment (pupil, skin conductance, heart rate). After a lunch break, the participant completes several online questionnaires, followed by a practice session in the dummy MRI laboratory and a second break. Subsequently, they provide a baseline saliva sample, which is followed by the oral intake of the study medication (indistinguishable capsule with 20 mg hydrocortisone or placebo). During the experimental set-up for the MRI session, the participant provides another saliva sample 30 minutes after medication administration. Heart rate and respiratory data are collected throughout the MRI session. The first part of the MRI session consists of a field map and task-based fMRI during fear extinction (45–55 minutes after medication administration) with psychophysiological assessment (pupil, skin conductance, heart rate). This is followed by a structural scan (T1-weighted during the first session; diffusion tensor imaging during the second session). During a short break, another saliva sample is provided (60–75 minutes after medication administration). The second part of the MRI session consists of resting-state fMRI with field mapping, and task-based fMRI during emotion processing with field mapping.
2.5.5.2. Next-day visit (B1.2 and B2.2)
The participant completes online questionnaires and returns to the psychophysiology laboratory to complete the extinction recall task with psychophysiological assessment (pupil, skin conductance, heart rate). This visit is always scheduled the next day in the late afternoon, with an interval of at least 24 hours between the start of the fear extinction task and extinction recall task.
2.5.6. Follow-up survey
An online survey is sent out only to participants from the PTSD group 9 months after the last visit of the observational study (i.e. study visit A2) to assess, if applicable, their response to treatments (i.e. treatment as usual which is unrelated to the current study). This online survey includes the PCL-5 to assess PTSD symptomatology, and questions about receiving psychotherapy and/or pharmacological treatment for PTSD and other psychiatric disorders. The control group was screened to be from a healthy population and as such is not expected to participate in any form of psychiatric treatment; hence, they do not participate in the follow-up survey.
2.5.7. Additional patient data
Participants recruited from a collaborating mental healthcare institution provide consent that the study team may request specific types of information about them from their mental healthcare institution. This includes results from CAPS-5 assessments, which, according to harmonized clinical practice protocols, should be administered prior to trauma-related treatment.
2.5.8. Reimbursement
Participants are offered a financial reimbursement for their time investment and effort, of €92 for the observational study, €130 for the pharmacological intervention study, and €10 for completion of the follow-up survey. When a participant is excluded following a screening visit or discontinues their participation, they are offered a reimbursement equivalent to their contributions. In addition, participants receive compensation for travelling expenses.
2.6. Statistical analysis
Mitigation plans to adapt statistical analyses if extension of the data collection period is no longer feasible and the planned sample size is not achieved are described in the Supplementary Material (S12).
2.6.1. Observational study
2.6.1.1. Primary outcome measures
HPA-axis dysregulation is investigated with two procedures by repeated sampling of saliva. These saliva samples will be analysed for cortisol levels using a competitive electrochemiluminescence immunoassay. For the DST, the main parameter of interest is suppression of the cortisol awakening response (CAR) based on the area under the curve (AUC) of normal CAR and dexamethasone-suppressed CAR, calculated as (1 − AUCDEX/AUCCAR) × 100%. For the SECPT, the main parameter of interest is the AUC with respect to increase (AUCi) in the cortisol stress response (Pruessner et al., Citation2003). Other variables and parameters from both procedures will also be investigated. ELA is assessed with the MACE questionnaire, which provides scores on 10 subscales of abuse and neglect that add up to a total scaled score and a multiple trauma score. It also provides information on the ages of exposure. Blood samples will be analysed for DNA methylation status, genotyping, and mRNA levels. Glucocorticoid signalling is primarily indicated by methylation of glucocorticoid-related genes, including NR3C1 and FKBP5, but mRNA levels and genotype interactions will also be assessed. Treatment response is operationalized as the reduction of self-reported PTSD symptoms according to PCL-5 questionnaire assessments.
2.6.1.2. Statistical modelling
Replicate findings of HPA-axis dysregulation in PTSD patients relative to a control group of individuals without PTSD or history of early-life trauma.
Statistical analyses of HPA-axis dysregulation in the PTSD group versus the control group will be tested using analyses of variance (ANOVAs), with group as the predictor of interest, and inclusion of age and gender as covariates. False discovery rate (FDR) correction will be applied over the number ANOVA tests with different HPA-axis dysregulation outcome variables. Furthermore, model extensions will explore additional covariates such as lifestyle factors (smoking, alcohol use, and drug use), concurrent psychiatric symptoms, and (female) menstrual cycle.
2. | Investigate associations between history of ELA and HPA-axis dysregulation. |
Associations between ELA and HPA-axis dysregulation will be investigated in the PTSD group with linear regression models, with the inclusion of age and gender as covariates. FDR correction will be applied over the number of regression models with different HPA-axis dysregulation outcome variables and/or different ELA predictor variables. Additional linear regression models will explore additional covariates such as other relevant task variables (e.g. SECPT stress ratings, duration of hand in water), lifestyle factors (smoking, alcohol use, and drug use), concurrent psychiatric symptoms, and (female) menstrual cycle.
3. | Investigate whether epigenetic markers of glucocorticoid signalling (such as methylation status of glucocorticoid-related genes) mediate the association between history of ELA and HPA-axis dysregulation. |
As a validation check of the proposed epigenetic mechanism, it will first be ascertained that for both genes NR3C1 and FKBP5 (and potentially other genes of interest), DNA methylation inversely correlates with mRNA levels in the blood. This will be tested with Pearson or Spearman correlation tests. Linear regression models will then investigate the associations between ELA and DNA methylation at NR3C1 and FKBP5 in the PTSD group, with the inclusion of age and gender as covariates. For FKBP5, we will take into account polymorphisms of SNP rs1360780 (minor risk allele T versus homozygous C-allele carriers) by specification of an ELA-by-genotype interaction effect, because of previously reported allele-specific gene–childhood effects (Klengel et al., Citation2013). FDR correction will be applied over the number of regression models with different DNA methylation outcome variables and/or different ELA predictor variables. The association between DNA methylation status and HPA-axis dysregulation will also be investigated with linear regression models, with the inclusion of age and gender as covariates. FDR correction will be applied over the number of regression models with different HPA-axis dysregulation outcome variables and/or different DNA methylation predictor variables. Additional linear regression models will explore additional covariates, such as other relevant task variables (e.g. SECPT stress ratings, duration), lifestyle factors (smoking, alcohol use, and drug use), concurrent psychiatric symptoms, and (female) menstrual cycle. If appropriate, regression models will be followed up by mediation models to directly test mediation of the association between ELA and HPA-axis dysregulation by methylation status of glucocorticoid-related genes.
4. | Exploratively, investigate whether HPA-axis dysregulation and glucocorticoid signalling are associated with treatment response. |
In the PTSD group, functional markers of HPA-axis dysregulation associated with treatment response will be examined with mixed-effects linear regression models. Since the PTSD group will have experienced heterogeneous courses of treatment, a specific analysis plan will be drafted after exploration of the frequency and variety of treatments within the sample.
2.6.2. Pharmacological intervention and fMRI study
2.6.2.1. Primary outcome measures
Successful fear acquisition, as indicated by a higher SER for the CS+s in comparison to CS− in the last block of the fear acquisition task, is a prerequisite for the assessment of extinction learning. During the fear extinction task, fear extinction should be indicated by decreasing SER, showing no expectancy of shock in the last block of this task. Fear acquisition and fear extinction will also be signalled by psychophysiological outcome measures (SCR and PDR). Neuroimaging during the fear extinction task investigates pharmacological effects on neural responses associated with fear extinction learning. Ultimately, the strength and generalization of the fear and extinction memories are assessed in the extinction recall task during recall of these memories upon presentation of the CSs in different contexts. Here, the primary outcome variables are SCR, PDR, and SER. Details of psychophysiological analyses are described in Supplementary Material S13. More specifically, increased strength of extinction memory should be indicated by smaller differential responses within the extinction context, whereas increased generalization of extinction memory should be indicated by a context interaction effect showing transfer of extinction to novel and/or acquisition contexts. In addition, effects of HPA-axis dysregulation are investigated to investigate their role in glucocorticoid effects on extinction recall. Relative suppression of the CAR from the DST is prespecified as the primary outcome measure, and based on a median split, the PTSD group will be divided over two subgroups. Grouping based on other HPA-axis outcome variables will be regarded as secondary and subjected to appropriate multiple-comparison corrections. Planned MRI (preprocessing) analyses are discussed in the Supplementary Material (S14).
2.6.2.2. Statistical modelling
1. Investigate whether patients with HPA-axis dysregulation show impairments in extinction learning relative to patients without HPA-axis dysregulation, and whether this is accompanied by different neural responses during extinction learning in brain regions associated with fear expression and extinction.
This objective will be investigated with data from placebo sessions only. At the psychophysiological level, we expect HPA-axis dysregulation to be associated with lower strength of the extinction memory, indicated by lower differential responses for CS+U vs CS+E and/or higher differential responses for CS+E vs CS− during the extinction recall task. This will be modelled with a two-way ANOVA of averaged differential responses during the first block, which is within the extinction context and contains two presentations of each CS. Higher generalization of the extinction memory for the HPA-axis normalized group would be indicated by a context interaction effect that shows less renewal of fear, i.e. a lower increase of differential effects upon stimulus presentations within the novel and acquisition contexts. These comparisons may be affected by an overall attenuation of fear responses due to re-extinction over the course of the extinction recall task. This effect will be investigated with a 2 (group) by 3 (context) mixed ANOVA of averaged differential responses during the first block of each context. Both models will include the covariate order of medication administration, and, if appropriate, the covariates age and gender. Outcome measures SCR, PDR, and SER will be included in separate models, but since these measures of conditioning are complementary, no multiple comparisons correction will be applied. At the level of neuroimaging, differences in extinction learning signalled during the fear extinction task could be underlying the differences at the behavioural level. Here, we would expect different neural responses in brain regions and networks associated with (context-dependent) extinction learning for patients with HPA-axis dysregulation, which could also be associated with less successful recall of the extinction memory.
2. Investigate whether the subgroup of PTSD patients with HPA-axis dysregulation particularly benefits from hydrocortisone administration (versus placebo) to improve the strength and generalization of extinction memories.
To answer the main objective of the pharmacological intervention study, the aforementioned psychophysiological models will be extended with data from the hydrocortisone sessions (thus adding a within-subjects category for medication). Period effects may follow from the crossover design and will be accounted for by defining a medication-by-order interaction effect. Based on previous research, we hypothesize a group-by-medication interaction effect, indicating greater strength and/or generalization of the extinction memory following administration of hydrocortisone, that is most pronounced for the HPA-axis dysregulation group.
3. Investigate underlying neural mechanisms of glucocorticoid administration (versus placebo) to improve extinction learning.
Recruitment of neural networks associated with (context-dependent) extinction learning for patients with HPA-axis dysregulation (see point 1 above) would be expected to enhance following hydrocortisone administration. Pharmacological effects and their potential interactions with HPA-axis dysregulation are primarily investigated during the extinction learning task, but will also be supported by the following analyses: (1) amygdala (and salience network) reactivity to the emotion processing task, which we expect to be reduced after hydrocortisone administration; and (2) resting-state connectivity in networks associated with (context-dependent) extinction learning – ventromedial prefrontal cortex–amygdala connectivity, in particular – which we expect to be enhanced after hydrocortisone administration.
3. Discussion
3.1. Study progress
The start of this study was delayed as a consequence of the coronavirus disease 2019 (COVID-19) pandemic, after which its first participant was included in December 2021. Subsequently, the current study faced further delays in its progress owing to challenges in recruitment of the PTSD group that were exacerbated by long-term effects of the COVID-19 pandemic and institutional reorganizations at collaborating mental healthcare institutions. As of March 2024, the observational study has completed study visits for n = 55 participants from the PTSD group and n = 23 participants from the control group. Of the PTSD group, n = 19 participants have additionally completed the pharmacological intervention study. This study has undergone several amendments to manage these challenges. Most importantly, initially all participants were included via collaborating mental healthcare institutions; in order to increase inclusion rates, inclusion of PTSD group participants from the general population started in January 2023, followed by large advertising campaigns from May 2023.
3.2. Collaborations
The current project is being conducted by a multidisciplinary, multicentre team. First, close collaboration with a patient federation as well as a committee of people with personal experience of PTSD has provided invaluable insights and recommendations for conducting this research. Secondly, this project runs parallel to a fundamental research project with rodents, which is carried out by the same team and tackles these questions from a different approach. The research project includes two rat lines with differential HPA-axis regulation and employs a similar fear acquisition, fear extinction, and extinction recall task. Here, findings from the rodent studies may also inform novel hypotheses or lead to substantiated adaptations or additions to planned analyses. The patient and rodent research projects will have complementary strengths and weaknesses. Animal research is vital to understand the causal role of the neural mechanisms mediating the effects of enhanced HPA-axis feedback regulation and exogenous glucocorticoids on the strength and generalization of extinction memories.
3.3. Strengths and limitations
The current study protocol describes an integrated and comprehensive study, which is conducted by a multidisciplinary team. The application of experimental tasks and procedures as part of assessments to a clinical group of PTSD patients allows mechanistic insights with a high potential for translation to clinical practice. As such, this study may pave the way for future clinical studies that investigate personalized PTSD treatment. The current study also has some limitations. For example, self-report questionnaires are used to assess symptomatology at follow-up. These are considered less reliable than structured clinical interviews such as CAPS-5, but assess a greater range of symptoms while placing less burden on participants (and may thus also show increased response rates). The current study only includes a CAPS-5 screening visit for PTSD participants recruited from the general population. PTSD participants who are recruited from local health mental institutes do not participate in this screening visit; here, we rely on harmonized clinical practice protocols for PTSD classification and treatment indication that include the administration of CAPS-5. Although PTSD classification is not verified by the study team prior to participation (owing to logistical challenges), participants provide consent so that we may receive this information from the local health mental institutes at a later stage. Of note, necessary amendments during the collection of study data resulted in additional limitations. Inclusion of PTSD group participants from the general population may lead to a selection bias of participants with chronic/complex PTSD who have particular interest in our stress system dysregulation hypotheses. It also increases the subgroup of participants that receives no PTSD treatment shortly after study participation and otherwise increases variability in treatment trajectories. This complicates the utility of treatment outcome follow-up measurements, but was necessary to increase inclusion rates.
4. Conclusion
Proof of concept that exogenous hydrocortisone can improve safety learning for a subgroup of PTSD patients – which could be provided by this study – will pave the way for a personalized treatment approach for PTSD. In the future, HPA-axis dysregulation could be used as a biomarker for deficits in safety learning processes and may then indicate that treatment may benefit from a pharmacological treatment enhancer. In line with previously established effects of glucocorticoids on emotional learning, the outcomes of this study may corroborate the utility of using hydrocortisone as a safe pharmacological treatment enhancer in trauma-focused treatments such as exposure therapy (de Quervain et al., Citation2017; De Quervain et al., Citation2019).
Authors’ contributions
Laura de Nooij: conceptualization, methodology, investigation, writing – original draft, visualization, and project administration; Lisa Wirz: methodology, software, and writing – review and editing; Emma Heling: methodology, investigation, and writing – review and editing; Mariana Pais: methodology and writing – review and editing; Gert-Jan Hendriks and Robbert-Jan Verkes: conceptualization, methodology, resources, writing – review and editing, supervision, and funding acquisition; Benno Roozendaal: conceptualization, methodology, writing – review and editing, supervision, and funding acquisition; Erno Hermans: conceptualization, methodology, writing – review and editing, supervision, project administration, and funding acquisition.
Ethics approval and consent to participate
This research project was approved as a pharmacological intervention study by the medical ethical committee (METC Oost-Nederland) (dossier nr. 2020-6865, Protocol ID NL71432.091.20). All participants were provided with written and oral information, and provided written informed consent at the start of their first study visit.
20240419 CovoS protocol paper supplements v5.pdf
Download PDF (683 KB)Acknowledgements
First, we acknowledge the Dutch patient federation for anxiety, compulsion and phobia, ‘ADF Stichting’, for their support in the formation of an advisory committee of people with PTSD and their contributions to the development and distribution of recruitment materials. Secondly, we acknowledge everyone from the collaborating mental healthcare institutions who have helped to implement this project and show commitment to inviting individuals with PTSD for participation. Thirdly, we also acknowledge the practical help from trainees with starting up this study project: Bente Schippers, Lana Miedema, and Imke van de Coevering.
Disclosure statement
No potential conflict of interest was reported by the authors.
Data availability statement
The data sets generated during the current study will be made available in the Donders repository (www.data.donders.ru.nl).
Additional information
Funding
References
- American Psychiatric Association. (2013). Diagnostic and statistical manual of mental disorders, 5th edition: DSM-5. American Psychiatric Publishing.
- Bandelow, B., Seidler-Brandler, U., Becker, A., Wedekind, D., & Rüther, E. (2007). Meta-analysis of randomized controlled comparisons of psychopharmacological and psychological treatments for anxiety disorders disorders. The World Journal of Biological Psychiatry, 8(3), 175–187. https://doi.org/10.1080/15622970601110273
- Blevins, C. A., Weathers, F. W., Davis, M. T., Witte, T. K., & Domino, J. L. (2015). The Posttraumatic Stress Disorder Checklist for DSM-5 (PCL-5): Development and initial psychometric evaluation. Journal of Traumatic Stress, 28(6), 489–498. https://doi.org/10.1002/jts.22059
- Boeschoten, M. A., Bakker, A., Jongedijk, R. A., & Olff, M. (2014). PTSD Checklist for DSM-5 – Nederlandstalige versie. Stichting Centrum ‘45. Arq Psychotrauma Expert Groep.
- Boeschoten, M. A., Van der Aa, N., Bakker, A., Ter Heide, F. J. J., Hoofwijk, M. C., Jongedijk, R. A., Van Minnen, A., Elzinga, B. M., & Olff, M. (2018). Development and evaluation of the Dutch Clinician-Administered PTSD Scale for DSM-5 (CAPS-5). European Journal of Psychotraumatology, 9(1), 1546085. https://doi.org/10.1080/20008198.2018.1546085
- Bosch, J., Mackintosh, M.-A., Wells, S. Y., Wickramasinghe, I., Glassman, L. H., & Morland, L. A. (2020). PTSD treatment response and quality of life in women with childhood trauma histories. Psychological Trauma: Theory, Research, Practice, and Policy, 12(1), 55–63. https://doi.org/10.1037/tra0000468
- Bouton, M. E., Winterbauer, N. E., & Todd, T. P. (2012). Relapse processes after the extinction of instrumental learning: Renewal, resurgence, and reacquisition. Behavioural Processes, 90(1), 130–141. https://doi.org/10.1016/j.beproc.2012.03.004
- Bradley, R., Greene, J., Russ, E., Dutra, L., & Westen, D. (2005). A multidimensional meta-analysis of psychotherapy for PTSD. American Journal of Psychiatry, 162(2), 214–227. https://doi.org/10.1176/appi.ajp.162.2.214
- Cusack, K., Jonas, D. E., Forneris, C. A., Wines, C., Sonis, J., Middleton, J. C., Feltner, C., Brownley, K. A., Olmsted, K. R., Greenblatt, A., Weil, A., & Gaynes, B. N. (2016). Psychological treatments for adults with posttraumatic stress disorder: A systematic review and meta-analysis. Clinical Psychology Review, 43, 128–141. https://doi.org/10.1016/j.cpr.2015.10.003
- de Kloet, C. S., Vermetten, E., Geuze, E., Kavelaars, A., Heijnen, C. J., & Westenberg, H. G. M. (2006). Assessment of HPA-axis function in posttraumatic stress disorder: Pharmacological and non-pharmacological challenge tests, a review. Journal of Psychiatric Research, 40(6), 550–567. https://doi.org/10.1016/j.jpsychires.2005.08.002
- de Nooij, L., Deckers, N., Miedema, L., Wirz, L., Verkes, R. J., Roozendaal, B., Hendriks, G. J., & Hermans, E. (2021). Neural mechanisms underlying the recall of a fear extinction memory in different contexts.
- de Quervain, D., Schwabe, L., & Roozendaal, B. (2017). Stress, glucocorticoids and memory: Implications for treating fear-related disorders. Nature Reviews Neuroscience, 18(1), 7–19. https://doi.org/10.1038/nrn.2016.155
- De Quervain, D., Wolf, O. T., & Roozendaal, B. (2019). Glucocorticoid-induced enhancement of extinction — from animal models to clinical trials. Psychopharmacology, 236(1), 183–199. https://doi.org/10.1007/s00213-018-5116-0
- de Voogd, L. D., Murray, Y. P. J., Barte, R. M., van der Heide, A., Fernández, G., Doeller, C. F., & Hermans, E. J. (2020). The role of hippocampal spatial representations in contextualization and generalization of fear: Fear memory contextualization. Neuroimage, 206, 116308. https://doi.org/10.1016/j.neuroimage.2019.116308
- Dorrepaal, E., Thomaes, K., Hoogendoorn, A. W., Veltman, D. J., Draijer, N., & van Balkom, A. J. L. M. (2014). Evidence-based treatment for adult women with child abuse-related complex PTSD: A quantitative review. European Journal of Psychotraumatology, 5(1), 23613. https://doi.org/10.3402/ejpt.v5.23613
- Drexler, S. M., Hamacher-Dang, T. C., & Wolf, O. T. (2017). Stress before extinction learning enhances and generalizes extinction memory in a predictive learning task. Neurobiology of Learning and Memory, 141, 143–149. https://doi.org/10.1016/j.nlm.2017.04.002
- Drexler, S. M., Merz, C. J., & Wolf, O. T. (2018). Preextinction stress prevents context-related renewal of fear. Behavior Therapy, 49(6), 1008–1019. https://doi.org/10.1016/j.beth.2018.03.001
- Ehring, T., Welboren, R., Morina, N., Wicherts, J. M., Freitag, J., & Emmelkamp, P. M. G. (2014). Meta-analysis of psychological treatments for posttraumatic stress disorder in adult survivors of childhood abuse. Clinical Psychology Review, 34(8), 645–657. https://doi.org/10.1016/j.cpr.2014.10.004
- Fonzo, G. A., Goodkind, M. S., Oathes, D. J., Zaiko, Y. V., Harvey, M., Peng, K. K., Weiss, M. E., Thompson, A. L., Zack, S. E., Lindley, S. E., Arnow, B. A., Jo, B., Gross, J. J., Rothbaum, B. O., & Etkin, A. (2017). PTSD psychotherapy outcome predicted by brain activation during emotional reactivity and regulation. American Journal of Psychiatry, 174(12), 1163–1174. https://doi.org/10.1176/appi.ajp.2017.16091072
- Fullana, M. A., Albajes-Eizagirre, A., Soriano-Mas, C., Vervliet, B., Cardoner, N., Benet, O., Radua, J., & Harrison, B. J. (2018). Fear extinction in the human brain: A meta-analysis of fMRI studies in healthy participants. Neuroscience & Biobehavioral Reviews, 88, 16–25. https://doi.org/10.1016/j.neubiorev.2018.03.002
- Goenjian, A. K., Yehuda, R., Pynoos, R. S., Steinberg, A. M., Tashjian, M., Yang, R. K., Najarian, L. M., & Fairbanks, L. A. (1996). Basal cortisol, dexamethasone suppression of cortisol, and MHPG in adolescents after the 1988 earthquake in Armenia. American Journal of Psychiatry, 153(7), 929–934. https://doi.org/10.1176/ajp.153.7.929
- Grossman, R., Yehuda, R., New, A., Schmeidler, J., Silverman, J., Mitropoulou, V., Sta. Maria, N., Golier, J., & Siever, L. (2003). Dexamethasone suppression test findings in subjects with personality disorders: Associations with posttraumatic stress disorder and major depression. American Journal of Psychiatry, 160(7), 1291–1297. https://doi.org/10.1176/appi.ajp.160.7.1291
- Hariri, A. R., Mattay, V. S., Tessitore, A., Kolachana, B., Fera, F., Goldman, D., Egan, M. F., & Weinberger, D. R. (2002). Serotonin transporter genetic variation and the response of the human amygdala. Science, 297(5580), 400–403. https://doi.org/10.1126/science.1071829
- Hoeboer, C. M., Oprel, D. A. C., De Kleine, R. A., Schwartz, B., Deisenhofer, A.-K., Schoorl, M., Van Der Does, W. A. J., van Minnen, A., & Lutz, W. (2021). Personalization of treatment for patients with childhoodabuse-related posttraumatic stress disorder. Journal of Clinical Medicine, 10(19), 1–15. https://doi.org/10.3390/jcm10194522
- Hoffman, V., Middleton, J. C., Feltner, C., Gaynes, B. N., Weber, R. P., Bann, C., Viswanathan, M., Lohr, K. N., Baker, C., & Green, J. (2018). Psychological and pharmacological treatments for adults with Posttraumatic Stress Disorder: A systematic review update. Comparative Effectiveness Review No. 207.
- Houtepen, L. C., Vinkers, C. H., Carrillo-Roa, T., Hiemstra, M., van Lier, P. A., Meeus, W., Branje, S., Heim, C. M., Nemeroff, C. B., Mill, J., Schalkwyk, L. C., Creyghton, M. P., Kahn, R. S., Joëls, M., Binder, E. B., & Boks, M. P. M. (2016). Genome-wide DNA methylation levels and altered cortisol stress reactivity following childhood trauma in humans. Nature Communications, 7(1), 10967. https://doi.org/10.1038/ncomms10967
- Inslicht, S. S., Niles, A. N., Metzler, T. J., Lipshitz, S. L., Otte, C., Milad, M. R., Orr, S. P., Marmar, C. R., & Neylan, T. C. (2021). Randomized controlled experimental study of hydrocortisone and D-cycloserine effects on fear extinction in PTSD. Neuropsychopharmacology, 47, 1945–1952. https://doi.org/10.1038/s41386-021-01222-z
- Klaassens, E. R., Giltay, E. J., Cuijpers, P., van Veen, T., & Zitman, F. G. (2012). Adulthood trauma and HPA-axis functioning in healthy subjects and PTSD patients: A meta-analysis. Psychoneuroendocrinology, 37(3), 317–331. https://doi.org/10.1016/j.psyneuen.2011.07.003
- Klengel, T., Mehta, D., Anacker, C., Rex-Haffner, M., Pruessner, J. C., Pariante, C. M., Pace, T. W. W., Mercer, K. B., Mayberg, H. S., Bradley, B., Nemeroff, C. B., Holsboer, F., Heim, C. M., Ressler, K. J., Rein, T., & Binder, E. B. (2013). Allele-specific FKBP5 DNA demethylation mediates gene-childhood trauma interactions. Nature Neuroscience, 16(1), 33–41. https://doi.org/10.1038/nn.3275
- Koenig, A. M., Ramo-Fernández, L., Boeck, C., Umlauft, M., Pauly, M., Binder, E. B., Kirschbaum, C., Gündel, H., Karabatsiakis, A., & Kolassa, I.-T. (2018). Intergenerational gene × environment interaction of FKBP5 and childhood maltreatment on hair steroids. Psychoneuroendocrinology, 92, 103–112. https://doi.org/10.1016/j.psyneuen.2018.04.002
- Labonté, B., Azoulay, N., Yerko, V., Turecki, G., & Brunet, A. (2014). Epigenetic modulation of glucocorticoid receptors in posttraumatic stress disorder. Translational Psychiatry, 4(3), 1–7. https://doi.org/10.1038/tp.2014.3
- Meewisse, M. L., Reitsma, J. B., De Vries, G. J., Gersons, B. P. R., & Olff, M. (2007). Cortisol and post-traumatic stress disorder in adults: Systematic review and meta-analysis. British Journal of Psychiatry, 191(5), 387–392. https://doi.org/10.1192/bjp.bp.106.024877
- Mehta, D., Gonik, M., Klengel, T., Rex-Haffner, M., Menke, A., Rubel, J., Mercer, K. B., Pütz, B., Bradley, B., Holsboer, F., & Ressler, K. J. (2011). Using polymorphisms in FKBP5 to define biologically distinct subtypes of Posttraumatic Stress Disorder. Archives of General Psychiatry, 68(9), 901–910. https://doi.org/10.1001/archgenpsychiatry.2011.50
- Merz, C. J., Hamacher-Dang, T. C., Stark, R., Wolf, O. T., & Hermann, A. (2018). Neural underpinnings of cortisol effects on fear extinction. Neuropsychopharmacology, 43(2), 384–392. https://doi.org/10.1038/npp.2017.227
- Meuret, A. E., Rosenfield, D., Bhaskara, L., Auchus, R., Liberzon, I., Ritz, T., & Abelson, J. L. (2016). Timing matters: Endogenous cortisol mediates benefits from early-day psychotherapy. Psychoneuroendocrinology, 74, 197–202. https://doi.org/10.1016/j.psyneuen.2016.09.008
- Morris, M. C., Compas, B. E., & Garber, J. (2012). Relations among posttraumatic stress disorder, comorbid major depression, and HPA function: A systematic review and meta-analysis. Clinical Psychology Review, 32(4), 301–315. https://doi.org/10.1016/j.cpr.2012.02.002
- Oberlander, T. F., Weinberg, J., Papsdorf, M., Grunau, R., Misri, S., & Devlin, A. M. (2008). Prenatal exposure to maternal depression, neonatal methylation of human glucocorticoid receptor gene (NR3C1) and infant cortisol stress responses. Epigenetics, 3(2), 97–106. https://doi.org/10.4161/epi.3.2.6034
- Pan, X., Wang, Z., Wu, X., Wen, S. W., & Liu, A. (2018). Salivary cortisol in post-traumatic stress disorder: A systematic review and meta-analysis. BMC Psychiatry, 18(1), 1–10. https://doi.org/10.1186/s12888-018-1910-9
- Parent, J., Parade, S. H., Laumann, L. E., Ridout, K. K., Yang, B.-Z., Marsit, C. J., Seifer, R., & Tyrka, A. R. (2018). Dynamic stress-related epigenetic regulation of the glucocorticoid receptor gene promotor during early development: The role of child maltreatment. Development and Psychopathology, 29(5), 1635–1648. https://doi.org/10.1017/S0954579417001298
- Perry, R. E., Rincón-Cortés, M., Braren, S. H., Brandes-Aitken, A. N., Opendak, M., Pollonini, G., Chopra, D., Raver, C. C., Alberini, C. M., Blair, C., & Sullivan, R. M. (2019). Corticosterone administration targeting a hypo-reactive HPA axis rescues a socially-avoidant phenotype in scarcity-adversity reared rats. Developmental Cognitive Neuroscience, 40, 100716. https://doi.org/10.1016/j.dcn.2019.100716
- Picó-Pérez, M., Alemany-Navarro, M., Dunsmoor, J. E., Radua, J., Albajes-Eizagirre, A., Vervliet, B., Cardoner, N., Benet, O., Harrison, B. J., Soriano-Mas, C., & Fullana, M. A. (2019). Common and distinct neural correlates of fear extinction and cognitive reappraisal: A meta-analysis of fMRI studies. Neuroscience & Biobehavioral Reviews, 104, 102–115. https://doi.org/10.1016/j.neubiorev.2019.06.029
- Pitman, R. K., Rasmusson, A. M., Koenen, K. C., Shin, L. M., Orr, S. P., Gilbertson, M. W., Milad, M. R., & Liberzon, I. (2012). Biological studies of Posttraumatic Stress Disorder. Nature Reviews Neuroscience, 13(11), 769–787. https://doi.org/10.1038/nrn3339
- Pruessner, J. C., Kirschbaum, C., Meinlschmid, G., & Hellhammer, D. H. (2003). Two formulas for computation of the area under the curve represent measures of total hormone concentration versus time-dependent change. Psychoneuroendocrinology, 28(7), 916–931. https://doi.org/10.1016/S0306-4530(02)00108-7
- Raij, T., Nummenmaa, A., Marin, M.-F., Porter, D., Furtak, S., Setsompop, K., & Milad, M. R. (2018). Prefrontal cortex stimulation enhances fear extinction memory in humans. Biological Psychiatry, 84(2), 129–137. https://doi.org/10.1016/j.biopsych.2017.10.022
- Raymond, C., Marin, M. F., Majeur, D., & Lupien, S. (2018). Early child adversity and psychopathology in adulthood: HPA axis and cognitive dysregulations as potential mechanisms. Progress in Neuro-Psychopharmacology and Biological Psychiatry, 85, 152–160. https://doi.org/10.1016/j.pnpbp.2017.07.015
- Ressler, K. J., & Mayberg, H. S. (2007). Targeting abnormal neural circuits in mood and anxiety disorders: From the laboratory to the clinic. Nature Neuroscience, 10(9), 1116–1124. https://doi.org/10.1038/nn1944
- Rooij, S. V., Kennis, M., Vink, M., & Geuze, E. (2016). Predicting treatment outcome in PTSD: A longitudinal functional MRI study on trauma-unrelated emotional processing. Neuropsychopharmacology, 41(4), 1156–1165. https://doi.org/10.1038/npp.2015.257
- Roozendaal, B., & McGaugh, J. L. (2011). Memory Modulation. Behavioral Neuroscience, 125(6), 797–824. https://doi.org/10.1037/a0026187
- Roozendaal, B., & Mirone, G. (2020). Opposite effects of noradrenergic and glucocorticoid activation on accuracy of an episodic-like memory. Psychoneuroendocrinology, 114, 104588. https://doi.org/10.1016/j.psyneuen.2020.104588
- Rousseau, P. F., El Khoury-Malhame, M., Reynaud, E., Boukezzi, S., Cancel, A., Zendjidjian, X., Guyon, V., Samuelian, J.-C., Guedj, E., Chaminade, T., & Khalfa, S. (2019). Fear extinction learning improvement in PTSD after EMDR therapy: An fMRI study. European Journal of Psychotraumatology, 10(1), 1568132. https://doi.org/10.1080/20008198.2019.1568132
- Schottenbauer, M. A., Glass, C. R., Arnkoff, D. B., Tendick, V., & Gray, S. H. (2014). Nonresponse and dropout rates in outcome studies on PTSD: Review and methodological considerations. Psychiatry: Interpersonal and Biological Processes, 71(2), 134–168. https://doi.org/10.1521/psyc.2008.71.2.134
- Schwabe, L., Haddad, L., & Schachinger, H. (2008). HPA axis activation by a socially evaluated cold-pressor test. Psychoneuroendocrinology, 33(6), 890–895. https://doi.org/10.1016/j.psyneuen.2008.03.001
- Schwabe, L., & Schächinger, H. (2018). Ten years of research with the Socially Evaluated Cold Pressor Test: Data from the past and guidelines for the future. Psychoneuroendocrinology, 92, 155–161. https://doi.org/10.1016/j.psyneuen.2018.03.010
- Sheehan, D. V., Lecrubier, Y., Sheehan, K. H., Amorim, P., Janavs, J., Weiller, E., Hergueta, T., Baker, R., & Dunbar, G. C. (1998). The Mini-International Neuropsychiatric Interview (M.I.N.I.): The development and validation of a structured diagnostic psychiatric interview for DSM-IV and ICD-10. Journal of Clinical Psychiatry, 59, 22–33.
- Stein, M. B., Yehuda, R., Koverola, C., & Hanna, C. (1997). Enhanced dexamethasone suppression of plasma cortisol in adult women traumatized by childhood sexual abuse. Biological Psychiatry, 42(8), 680–686. https://doi.org/10.1016/S0006-3223(96)00489-1
- Suarez-Jimenez, B., Albajes-Eizagirre, A., Lazarov, A., Zhu, X., Harrison, B. J., Radua, J., Neria, Y., & Fullana, M. A. (2019). Neural signatures of conditioning, extinction learning, and extinction recall in posttraumatic stress disorder: A meta-analysis of functional magnetic resonance imaging studies. Psychological Medicine, 1–10. https://doi.org/10.1017/S0033291719001387
- Suris, A., North, C., Adinoff, B., Powell, C. M., & Greene, R. (2010). Effects of exogenous glucocorticoid on combat-related PTSD symptoms. Annals of Clinical Psychiatry, 22, 274–279.
- Teicher, M. H., & Parigger, A. (2015). The “Maltreatment and Abuse Chronology of Exposure” (MACE) scale for the retrospective assessment of abuse and neglect during development. PLoS One, 10(2), 1–37. https://doi.org/10.1371/journal.pone.0117423
- Turecki, G., & Meaney, M. J. (2016). Effects of the social environment and stress on glucocorticoid receptor gene methylation: A systematic review. Biological Psychiatry, 79(2), 87–96. https://doi.org/10.1016/j.biopsych.2014.11.022
- van Zuiden, M., Geuze, E., Willemen, H. L. D. M., Vermetten, E., Maas, M., Heijnen, C. J., & Kavelaars, A. (2011). Pre-existing high glucocorticoid receptor number predicting development of posttraumatic stress symptoms after military deployment. American Journal of Psychiatry, 168(1), 89–96. https://doi.org/10.1176/appi.ajp.2010.10050706
- Vukojevic, V., Kolassa, I.-T., Fastenrath, M., Gschwind, L., Spalek, K., Milnik, A., Heck, A., Vogler, C., Wilker, S., Demougin, P., Peter, F., Atucha, E., Stetak, A., Roozendaal, B., Elbert, T., Papassotiropoulos, A., & de Quervain, D. J.-F. (2014). Epigenetic modification of the glucocorticoid receptor gene is linked to traumatic memory and Post-traumatic Stress Disorder risk in genocide survivors. Journal of Neuroscience, 34(31), 10274–10284. https://doi.org/10.1523/JNEUROSCI.1526-14.2014
- Wackerhagen, C., Veer, I. M., van Leeuwen, J. M. C., Reppmann, Z., Riepenhausen, A., Bögemann, S. A., Mor, N., Puhlmann, L. M. C., Uściƚko, A., Zerban, M., Mituniewicz, J., Lerner, A., Yuen, K. S. L., Köber, G., Marciniak, M. A., Pooseh, S., Weermeijer, J., Arias-Vásquez, A., Binder, H., … Walter, H. (2023). Study protocol description: Dynamic Modelling of Resilience - Observational Study (DynaM-OBS). JMIR Research Protocols, 12, e39817. https://doi.org/10.2196/39817
- Wagenmans, A., Van Minnen, A., Sleijpen, M., & De Jongh, A. (2018). The impact of childhood sexual abuse on the outcome of intensive trauma-focused treatment for PTSD. European Journal of Psychotraumatology, 9(1), 1430962. https://doi.org/10.1080/20008198.2018.1430962
- Weaver, I. C. G., D’Alessio, A. C., Brown, S. E., Hellstrom, I. C., Dymov, S., Sharma, S., Szyf, M., & Meaney, M. J. (2007). The transcription factor nerve growth factor-inducible protein a mediates epigenetic programming: Altering epigenetic marks by immediate-early genes. The Journal of Neuroscience, 27(7), 1756–1768. https://doi.org/10.1523/JNEUROSCI.4164-06.2007
- Wen, Z., Seo, J., Pace-Schott, E. F., & Milad, M. R. (2022). Abnormal dynamic functional connectivity during fear extinction learning in PTSD and anxiety disorders. Molecular Psychiatry, 27(4), 2216–2224. https://doi.org/10.1038/s41380-022-01462-5
- Wessa, M., & Flor, H. (2007). Failure of extinction of fear responses in Posttraumatic Stress Disorder: Evidence from second-order conditioning. American Journal of Psychiatry, 164(11), 1684–1692. https://doi.org/10.1176/appi.ajp.2007.07030525
- Wicking, M., Steiger, F., Nees, F., Diener, S. J., Grimm, O., Ruttorf, M., Schad, L. R., Winkelmann, T., Wirtz, G., & Flor, H. (2016). Deficient fear extinction memory in posttraumatic stress disorder. Neurobiology of Learning and Memory, 136, 116–126. https://doi.org/10.1016/j.nlm.2016.09.016
- Wirz, L., Hermans, E., Pützer, A., & Wolf, O. (2021). Neural mechanisms of noardrenaline and cortisol effects on memory accuracy versus generalization over time.
- World Health Organization. (2018). International statistical classification of diseases and related health problems (11th ed).
- Yehuda, R. (2009). Status of glucocorticoid alterations in posttraumatic stress disorder. Annals of the New York Academy of Sciences, 1179(1), 56–69. https://doi.org/10.1111/j.1749-6632.2009.04979.x
- Yehuda, R., Bierer, L. M., Pratchett, L. C., Lehrner, A., Koch, E. C., Van Manen, J. A., Flory, J. D., Makotkine, I., & Hildebrandt, T. (2015a). Cortisol augmentation of a psychological treatment for warfighters with posttraumatic stress disorder: Randomized trial showing improved treatment retention and outcome. Psychoneuroendocrinology, 51, 589–597. https://doi.org/10.1016/j.psyneuen.2014.08.004
- Yehuda, R., Bierer, L. M., Pratchett, L., & Malowney, M. (2010). Glucocorticoid augmentation of prolonged exposure therapy: Rationale and case report. European Journal of Psychotraumatology, 1(1), 5643. https://doi.org/10.3402/ejpt.v1i0.5643
- Yehuda, R., Boisoneau, D., Lowy, M. T., & Giller, E. L. (1995). Dose-response changes in plasma cortisol and lymphocyte glucocorticoid receptors following dexamethasone adminisatration in combat veterans with and without Posttraumatic Stress Disorder. Archives of General Psychiatry, 52(7), 583–593. https://doi.org/10.1001/archpsyc.1995.03950190065010
- Yehuda, R., Flory, J. D., Bierer, L. M., Henn-Haase, C., Lehrner, A., Desarnaud, F., Makotkine, I., Daskalakis, N. P., Marmar, C. R., & Meaney, M. J. (2015b). Lower methylation of glucocorticoid receptor gene promoter 1F in peripheral blood of veterans with Posttraumatic Stress Disorder. Biological Psychiatry, 77(4), 356–364. https://doi.org/10.1016/j.biopsych.2014.02.006
- Yehuda, R., Golier, J. A., Halligan, S. L., Meaney, M., & Bierer, L. M. (2004). The ACTH response to dexamethasone in PTSD. American Journal of Psychiatry, 161(8), 1397–1403. https://doi.org/10.1176/appi.ajp.161.8.1397
- Yehuda, R., Halligan, S. L., Grossman, R., A. Golier, J., & Wong, C. (2002). The cortisol and glucocorticoid receptor response to low dose dexamethasone administration in aging combat veterans and holocaust survivors with and without posttraumatic stress disorder. Biological Psychiatry, 52(5), 393–403. https://doi.org/10.1016/S0006-3223(02)01357-4
- Yehuda, R., Southwick, S. M., Krystal, J. H., Bremner, D., Charney, D. S., & Mason, J. W. (1993). Enhanced suppression of cortisol following dexamethasone administration in posttraumatic stress disorder. American Journal of Psychiatry, 150(1), 83–86. https://doi.org/10.1176/ajp.150.1.83
- Zhang, T. Y., Labonté, B., Wen, X. L., Turecki, G., & Meaney, M. J. (2013). Epigenetic mechanisms for the early environmental regulation of hippocampal glucocorticoid receptor gene expression in rodents and humans. Neuropsychopharmacology, 38(1), 111–123. https://doi.org/10.1038/npp.2012.149
- Zoladz, P. R., & Diamond, D. M. (2013). Current status on behavioral and biological markers of PTSD: A search for clarity in a conflicting literature. Neuroscience & Biobehavioral Reviews, 37(5), 860–895. https://doi.org/10.1016/j.neubiorev.2013.03.024