ABSTRACT
Background: Individuals with posttraumatic stress disorder (PTSD) are at heightened risk for cardiovascular disease (CVD) compared to the general population. Inflammation and autonomic dysfunction are candidate mechanisms of CVD risk in PTSD; however, these mechanisms have not been well-characterised in the PTSD-CVD link. Further, these mechanisms may operate through altered stress-related neural activity (SNA). Yet, it remains unknown if changes in PTSD are associated with changes in CVD risk mechanisms.
Objective: This manuscript describes the design and procedures of a pilot randomised controlled trial to assess the impact of a first-line treatment for PTSD (Cognitive Processing Therapy; CPT) versus waitlist control on mechanisms of CVD risk. Further, this study will test the hypothesis that CPT reduces CVD risk through its effects on inflammation and autonomic function and that these changes are driven by changes in SNA.
Methods: Adults with PTSD and CVD risk (N = 30) will be randomised to CPT or waitlist control. Participants complete two laboratory visits (baseline and post-treatment) that include surveys, brain and peripheral imaging via 18F-fluorodeoxyglucose positron emission tomography (FDG-PET), and resting measures of autonomic function. Primary outcomes include arterial inflammation and heart rate variability. Secondary outcomes include leukopoiesis (bone marrow uptake), heart rate, and blood pressure. The indirect effects of PTSD treatment on changes in inflammation and autonomic function through SNA will also be examined.
Conclusions: This study seeks to characterise candidate neuroimmune mechanisms of the PTSD-CVD link to identify treatment targets and develop personalised interventions to reduce CVD events in PTSD populations.
Trial registration: ClinicalTrials.gov identifier: NCT06429293..
HIGHLIGHTS
Individuals with posttraumatic stress disorder (PTSD) have greater risk for cardiovascular disease (CVD) than the general population.
Autonomic dysfunction and inflammation are candidate mechanisms of the PTSD-CVD link, which may be driven by changes in neural activity.
This pilot randomised controlled trial will test the impact of a first-line PTSD treatment on autonomic dysfunction and inflammation, and whether neural alterations are associated with changes in these mechanisms.
Antecedentes: Las personas con trastorno de estrés postraumático (TEPT) tienen un mayor riesgo de enfermedad cardiovascular (ECV) en comparación con la población general. La inflamación y la disfunción autonómica son mecanismos candidatos de riesgo de ECV en el TEPT; sin embargo, estos mecanismos no han sido bien caracterizados en la conexión entre TEPT y ECV. Además, estos mecanismos pueden operar a través de una actividad neuronal relacionada con el estrés (SNA) alterada. Aún se desconoce si los cambios en el TEPT están asociados con cambios en los mecanismos de riesgo de ECV.
Objetivo: Este manuscrito describe el diseño y los procedimientos de un ensayo piloto aleatorizado y controlado para evaluar el impacto de un tratamiento de primera línea para el TEPT (Terapia de Procesamiento Cognitivo; CPT, por sus siglas en inglés) versus un grupo control en lista de espera, sobre los mecanismos de riesgo de ECV. Además, este estudio probará la hipótesis de que la CPT reduce el riesgo de ECV a través de sus efectos sobre la inflamación y la función autonómica, y que estos cambios son impulsados por cambios en el SNA.
Métodos: Adultos con TEPT y riesgo de ECV (N = 30) serán asignados aleatoriamente a CPT o a un grupo control en lista de espera. Los participantes completarán dos visitas de laboratorio (inicial y post-tratamiento) que incluyen encuestas, imágenes cerebrales y periféricas mediante tomografía por emisión de positrones con 18F-fluorodesoxiglucosa (FDG-PET) y medidas en reposo de la función autonómica. Los resultados primarios incluyen inflamación arterial y variabilidad de la frecuencia cardíaca. Los resultados secundarios incluyen leucopoyesis (captación de médula ósea), frecuencia cardíaca y presión arterial. También se examinarán los efectos indirectos del tratamiento del TEPT sobre los cambios en la inflamación y la función autonómica a través del SNA.
Conclusiones: Este estudio busca caracterizar los mecanismos neuroinmunes candidatos en la conexión entre TEPT y ECV para identificar objetivos de tratamiento y desarrollar intervenciones personalizadas que reduzcan los eventos de ECV en poblaciones con TEPT.
1. Introduction
Cardiovascular disease (CVD) is the leading cause of death in the US (Centers for Disease Control and Prevention, National Center for Health Statistics, Citation2021) and individuals with posttraumatic stress disorder (PTSD) have greater CVD risk compared to the general population (Edmondson & Cohen, Citation2013; Edmondson & Känel, Citation2017). After experiencing a traumatic event (e.g. assault, combat), those who do not recover naturally will develop mental health disorders including PTSD (Chen et al., Citation2024), which is a debilitating disorder that involves intrusive memories, flashbacks, nightmares, hypervigilance, and an exaggerated startle response (Association AP, Citation2013). Trauma exposure is common (Kessler et al., Citation1995) and PTSD affects over 12 million individuals per year (Goldstein et al., Citation2016); thus, increased CVD risk in PTSD has become a major public health concern. As such, there is a significant need to characterise CVD risk mechanisms in PTSD and to determine whether existing treatments for PTSD improve CVD risk mechanisms and, ultimately, the incidence of CVD events in this at-risk population.
PTSD is characterised by chronic activation of the stress response, such as increased heart rate (HR) (Buckley & Kaloupek, Citation2001; Ehlers et al., Citation2010; Jovanovic et al., Citation2009) and blood pressure (BP) (Seligowski et al., Citation2021), decreased heart rate variability (HRV) (Ge et al., Citation2020; Moon et al., Citation2013; Schneider & Schwerdtfeger, Citation2020; Seligowski et al., Citation2021), and increased norepinephrine levels (Hendrickson & Raskind, Citation2016). The sympathetic branch of the autonomic nervous system is responsible for this stress response (‘fight or flight’) that helps individuals contend with threat (e.g. pupils dilate, HR and BP increase, norepinephrine is released, heightened inflammatory response) (McCarty, Citation2016). This system is overactive in those with PTSD, such that individuals with PTSD exhibit chronically heightened HR and BP versus controls (Buckley & Kaloupek, Citation2001; Ehlers et al., Citation2010; Jovanovic et al., Citation2009). This is coupled with lowered activity of the parasympathetic nervous system in PTSD (Ge et al., Citation2020; Schneider & Schwerdtfeger, Citation2020; Seligowski et al., Citation2021; Moon et al., Citation2013), which is responsible for rest, digestion, and self-regulation. Specifically, HRV is a validated measure of autonomic balance that is lower in PTSD compared to controls (Ge et al., Citation2020; Moon et al., Citation2013; Schneider & Schwerdtfeger, Citation2020; Seligowski et al., Citation2021), and our recent work demonstrated that HRV mediated the association between PTSD and CVD events in a large hospital biobank sample (Seligowski et al., Citation2024). Because prolonged activation of the stress response leads to elevated BP (conferring risk for hypertension) and the release of inflammatory cytokines (Brudey et al., Citation2015; Kim et al., Citation2020; O’Donovan et al., Citation2012; Seligowski et al., Citation2024), autonomic dysfunction is a candidate mechanism increasing CVD risk in PTSD.
Increased CVD risk in PTSD may also be driven by systemic inflammation (Brudey et al., Citation2015; Kim et al., Citation2020; O’Donovan et al., Citation2012; Seligowski et al., Citation2024), which is associated with activation of the stress response. The stress response triggers cytokine release (e.g., interleukin-6; C-reactive protein) into circulation, leading to heightened activity in the bone marrow (leukopoiesis) and increased cellular release. Ultimately, this response results in arterial inflammation, a highly salient predictor of CVD events (Tawakol et al., Citation2017). Prior studies have found that PTSD is associated with higher cytokine levels (Brudey et al., Citation2015; Kim et al., Citation2020; O’Donovan et al., Citation2012), and our recent work demonstrated that increased high sensitivity C-reactive protein mediated the PTSD-CVD link (Seligowski et al., Citation2024). Further, we recently showed that PTSD was associated with worse arterial inflammation on FDG-PET imaging (Gharios et al., Citation2024; Lima et al., Citation2019; O’Donovan et al., Citation2012) compared to healthy and trauma-exposed controls. Accordingly, inflammation is another promising candidate mechanism in the PTSD-CVD link.
Alterations in neural activity have repeatedly been shown in PTSD (Shin et al., Citation2004, Citation2005; Stevens et al., Citation2013; Williams et al., Citation2006) and the brain regions involved are inherently linked to CVD risk mechanisms (). Specifically, individuals with PTSD exhibit increased amygdala activity (i.e. greater fear) and relatively lower ventromedial prefrontal cortex (vmPFC) activity (i.e. worse fear regulation) compared to controls (Shin et al., Citation2004, Citation2005; Stevens et al., Citation2013; Williams et al., Citation2006). These brain regions project to the hypothalamus and brainstem, where they ultimately regulate autonomic activity (e.g., HR, BP). Consistent with prior research in PTSD, we have shown that stress-associated neural activity (SNA), as the ratio of amygdala to vmPFC metabolic activity on FDG-PET imaging, is higher in PTSD (Gharios et al., Citation2024) and that it mediates the PTSD-CVD link (Seligowski et al., Citation2024). Further, SNA has been shown to predict CVD in several populations (e.g., atherosclerosis, myocardial infarction, stroke) (Gharios et al., Citation2024; Osborne et al., Citation2021; Tawakol et al., Citation2017) including trauma-exposed individuals with and without PTSD (Gharios et al., Citation2024). Thus, SNA represents another important mechanism in the PTSD-CVD relationship.
Figure 1. Mechanistic model of CVD risk in PTSD.
Note. BP = blood pressure; HR = heart rate; HRV = heart rate variability; NTS = solitary nucleus; RVLM = rostral ventrolateral medulla; SNA = stress-associated neural network activity; vmPFC = ventromedial prefrontal cortex. PTSD leads to alterations in stress-related neural activity, leading to increased inflammation and autonomic dysfunction, which both lead to cardiovascular disease.
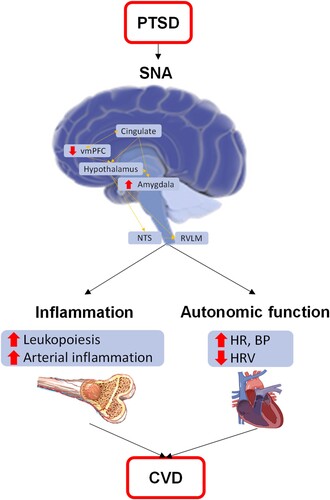
There is preliminary evidence that PTSD treatment confers neural changes in SNA-relevant regions (e.g. vmPFC) (Abdallah et al., Citation2019; Aupperle et al., Citation2013; Helpman et al., Citation2016; Manthey et al., Citation2021; Santarnecchi et al., Citation2019; Zhu et al., Citation2018) and improves HRV (Watkins et al., Citation2023). For example, alterations in the amygdala- and hippocampal-PFC pathways have been found following a first-line PTSD treatment (Prolonged Exposure Therapy [Foa et al., Citation2007; VA/DoD Clinical Practice Guideline, Citation2023]), and notably, these alterations appeared to resolve pre-treatment neural differences between those with PTSD and trauma-exposed controls (Zhu et al., Citation2018). Similar findings have been observed in other cognitive-behavioural PTSD treatments, as detailed in a recent review of neural changes following PTSD treatment (k = 24) (Manthey et al., Citation2021). Improvements in autonomic function after PTSD treatment, primarily HRV, have also been shown (see Bourassa et al. for review [Bourassa et al., Citation2021]), but less is known about the impact of PTSD treatment on inflammation and other CVD markers (Sumner et al., Citation2020). Recent evidence from a clinical trial of another first-line PTSD treatment (Cognitive Processing Therapy; CPT) (Resick et al., Citation2017; VA/DoD Clinical Practice Guideline, Citation2023) supports the effect of PTSD treatment on HRV; however, no treatment effect was found for changes in inflammation and endothelial function compared to waitlist control (Watkins et al., Citation2023). Given that participants were not recruited based on elevated CVD risk and excluded if they had coronary heart disease, sample characteristics may have contributed to the null findings in CVD risk mechanisms (Watkins et al., Citation2023). As such, a critical gap in this research is the comprehensive testing of how PTSD treatment affects inflammation and autonomic function in those with elevated CVD risk, and whether neural activity associates with change in these peripheral mechanisms.
This manuscript describes a pilot study that will address these gaps by testing the impact of CPT on mechanisms proposed in the PTSD-CVD link. Our central hypothesis is that CPT reduces CVD risk through its effects on inflammation and autonomic function, which are driven by changes in SNA. The first aim is to determine the effects of CPT on inflammation. We hypothesise that following CPT, participants will demonstrate reduced inflammation measured as arterial inflammation and leukopoiesis on FDG-PET compared to waitlist controls, and that changes in inflammation will associate with changes in SNA. The second aim is to determine the effects of CPT on autonomic function. We hypothesise that following CPT, participants will demonstrate improved autonomic function as indicated by increased HRV and decreased HR and BP compared to waitlist controls, and that changes in autonomic function will be related to changes in SNA.
2. Materials and method
This project is funded by the American Heart Association (23SCISA1143491). The trial is registered on clinicaltrials.gov (NCT06429293). All procedures described below were approved by the Massachusetts General Brigham Institutional Review Board.
2.1. Overview of study design and recruitment
This study is a randomised controlled trial of CPT compared to waitlist control that is testing the effects of CPT on mechanisms of the PTSD-CVD link. Enrolment began in 2023 and is projected to continue through 2026. Participants include individuals with PTSD and CVD risk recruited from the Boston area (N = 30). Treatment assignment is randomised and stratified by sex. Participants are randomised to CPT (n = 15) or waitlist control (n = 15). Potentially eligible participants complete a screening visit to confirm inclusion/exclusion criteria. Upon confirmation of eligibility, participants are scheduled for a baseline session, where they complete surveys, brain and peripheral imaging, and resting measures of autonomic function. Following the baseline visit, participants are randomised into CPT or the waitlist control group. Those randomised to CPT complete sessions via telehealth. Following a 12-week treatment period, participants attend the post-treatment visit, consisting of the same assessments administered at baseline. Participants randomised to waitlist are offered CPT upon completion of the post-treatment visit. See for an overview of study design and flow.
Participants will be recruited through multiple pathways. First, participants may self-refer based on recruitment flyers posted on approved bulletin boards at the hospital (including online boards). Second, eligible participants may be identified via the hospital-wide registry of patients interested in research and be directly invited. Third, participants may be referred by primary care physicians and physician specialists. Interested subjects are contacted by phone and screened to confirm initial eligibility criteria. If eligible, a virtual consent visit is scheduled, followed by psychiatric assessments to confirm additional eligibility criteria (e.g., substance use).
2.1.1. Inclusion/exclusion
Inclusion criteria for the study are as follows: (1) age 18–65 years (upper limit chosen to optimise changes in brain activation that diminish with age); (2) criterion A trauma exposure and PTSD symptoms (clinically significant symptoms in at least two symptom clusters [Klein et al., Citation2024]); (3) subclinical atherosclerotic CVD (e.g., coronary, cerebrovascular, or peripheral arterial plaque or calcifications on imaging), clinical atherosclerotic CVD (e.g. myocardial infarction or revascularization), or increased risk for atherosclerotic CVD (i.e. ≥2 of hypertension, diabetes mellitus, hyperlipidemia, and active smoking); (3) ability to understand and sign informed consent; (4) fluent English speaker.
Exclusion criteria include: (1) history of stroke, brain surgery, seizure (2) use of certain CVD medications (e.g. beta-blockers, high-intensity statins [e.g., rosuvastatin 20/40 mg and atorvastatin 40/80 mg], PCSK-9 inhibitors); (3) psychiatric or cardiovascular medication change within 4 weeks (i.e. stable regimen is allowed); (4) currently in PTSD therapy; (5) neurological or systemic inflammatory disease/current anti-inflammatory therapy; (6) moderate/severe alcohol/substance use disorder; (7) current mania/psychosis; (8) weight >300 lbs., claustrophobia, pregnancy, metal implants that are incompatible with magnetic resonance imaging (MRI), or uncontrolled hyperglycemia (for imaging); (9) significant radiation exposure (>2 nuclear tests, computed tomography images, or fluoroscopic procedures) during the preceding 12-months.
2.2. Diagnostic interviews and surveys
Participants are administered the Clinician Administered PTSD Scale (CAPS-5) (Weathers, Blake, et al., Citation2013) to assess PTSD symptoms following consent. In addition, participants complete self-report measures at baseline and post-treatment visits (). These evaluations include demographics, the Life Events Checklist (Weathers et al., Citation2013) to assess trauma history, the PTSD Checklist (Weathers, Litz, et al., Citation2013), the Beck Depression Inventory (Beck et al., Citation1996), the State-Trait Anxiety Inventory (Spielberger, Citation1983), the Pittsburgh Sleep Quality Index (Buysse et al., Citation1989), the International Physical Activity Questionnaire (Booth, Citation2000), and questionnaires to assess other health information (e.g. smoking, diet) used in our prior work (Gharios, Van Leent, et al., Citation2024; Tawakol et al., Citation2017).
Table 1. Schedule of study assessments.
2.3. Imaging
2.3.1. Brain FDG-PET
Simultaneous PET/MRI is performed on a Siemens PET/MR 3 T Biograph scanner (). FDG-PET imaging is used to assess the metabolic activity of the amygdala and vmPFC, which will be used to calculate SNA (Tawakol et al., Citation2017). The activity of each region will be quantified as the mean and maximum bilateral standardised uptake value (SUV) of the target tissue compared to the regulatory activity of cerebral tissue (mean vmPFC uptake value). SNA will then be calculated as a ratio of amygdala to vmPFC activity (Radfar et al., Citation2021).
Figure 3. Overview of imaging session.
Note. BP = Blood Pressure; ECG = electrocardiogram; FDG = 18F-fluorodeoxyglucose; PET/MRI = positron emission tomography/ magnetic resonance imaging; fMRI = functional magnetic resonance imaging; DTI = diffusion tensor imaging; all neural imaging is performed at rest besides fMRI which is performed with eyes opened with fixation cross and the Hariri task. Timeline of procedures for the 155-minute imaging session. The first 60 minutes of the session includes consent, measure of autonomic function, participant preparation and set-up followed by FDG-PET/MRI brain and peripheral imaging.
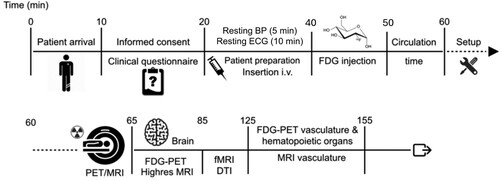
2.3.2. Arterial and bone marrow FDG-PET
The uptake of FDG, a radioactive glucose analogue that accumulates in metabolically active tissues (Osborne et al., Citation2021), relates to the degree of inflammatory activity in peripheral tissues in the absence of malignancy (Osborne et al., Citation2021). Importantly, the imaging measurements of FDG activity in the bone marrow and arteries are highly reproducible (Osborne et al., Citation2021). Furthermore, the arterial FDG signal predicts plaque expansion and CVD events (Tawakol et al., Citation2017). Measurements of arterial inflammation include: (1) the ratio of the mean of the maximum whole vessel SUV, an average of the maximum SUV measured every 3 mm spanning the vessel, to the background venous activity to yield a mean maximum arterial tissue-to-background ratio (TBR) and (2) the ratio of the maximally diseased segment SUV, an average of the maximum SUV from the segment with the highest SUV and the maximum SUV of two neighbouring segments, to venous background activity to yield a maximally diseased segment TBR. These measurements are performed for the aorta and carotid arteries. A validated measurement of leukopoiesis with FDG-PET imaging includes bone marrow FDG uptake as measured in the lumbar spine and compared to background venous FDG uptake to calculate a TBR (Emami et al., Citation2015).
2.3.3. Brain MRI
Non-contrast brain structural MRI, diffusion tensor imaging, and functional MRI are also performed. Participants will be imaged for fMRI at rest (eyes open, fixation cross) and during a commonly used fMRI measure of emotional reactivity (the Hariri task [Hariri et al., Citation2000]). Resting fMRI data will be used to measure SNA. The Hariri task asks participants to match fearful or angry faces, threatening images, or geometric shapes (control). The paradigm consists of nine randomised blocks, with of two blocks of faces, two blocks of images, and five control blocks (Hariri et al., Citation2000). The Hariri task will allow us to evaluate PTSD-relevant neural centre activation (e.g. amygdala [Shin et al., Citation2005; Williams et al., Citation2006]) in response to various emotional stimuli. PET imaging of the brain will only be performed at rest. This is a stable measure over months based on our prior work and the work of others and therefore is more reflective of a chronic rather than acute state (Schaefer et al., Citation2000). This imaging protocol facilitates assessments of brain volume, activation in response to emotional triggers, connectivity, and axonal integrity to complement the assessment of SNA.
2.3.4. Arterial MRI
To minimise scan duration and artifacts, 3-dimensional black blood MRI is used to assess plaque burden in the aorta and carotid arteries. Measurements include wall area/thickness and plaque volume. Plaque components are also evaluated (Osborne et al., Citation2021).
2.4. Autonomic measures
Biopac MP150 for Windows is used to collect resting electrocardiogram (ECG) data at a sampling rate of 1 kHz. The ECG is measured using two disposable Ag/AgCl electrodes placed in the Lead I position (right clavicle and left forearm, with a ground on the left clavicle) and data will be processed using MindWare software. Average HR in beats per minute will be calculated for 10 min at rest. Data will include ECG R-R intervals (the time interval between heart beats), which is used to derive high frequency HRV. Settings for the high frequency band will be based on standard recommendations for HRV data (0.12–0.40 Hz) (Task Force of the European Society of Cardiology the North A E, Citation1996). Average HRV will be calculated for the 10-minute resting period. BP is measured during a five-minute resting period (average of three measurements) with an Omron HEM-907XL sphygmomanometer. The measurement of BP and ECG will be conducted separately prior to imaging.
2.5. Primary outcomes
Primary outcomes were chosen based on the strength of the prior evidence while maximising power for this small pilot trial. The primary outcome for Aim 1 (inflammation) is arterial inflammation, given that arterial inflammation represents an independent predictor of CVD risk (Cecelja & Chowienczyk, Citation2012) and offers greater specificity than other commonly used markers of inflammation (e.g. CRP [Ruparelia et al., Citation2017]). As described above, arterial inflammation is obtained at baseline and post-treatment visits through FDG-PET imaging. The primary outcome for Aim 2 (autonomic function) is HRV, as HRV is the dominant autonomic indicator implicated in PTSD (Bourassa et al., Citation2021; Watkins et al., Citation2023). Average resting HRV collected at baseline and post-treatment visits will be calculated.
2.6. Secondary outcomes
A secondary outcome for Aim 1 (inflammation) is leukopoiesis, which is measured as bone marrow activity at baseline and post-treatment via FDG-PET imaging. Secondary outcomes for Aim 2 (autonomic function) include resting HR and BP, which are measured at baseline and post-treatment. Additional exploratory outcomes include data derived from MRI imaging of the brain and arteries, including emotional reactivity data from the Hariri task.
2.7. Treatment
The active intervention is Cognitive Processing Therapy (CPT). CPT is a gold-standard cognitive behavioural therapy for PTSD (Finley et al., Citation2019; Richards et al., Citation2017). The CPT intervention consists of 12 60-minute sessions teaching skills to challenge trauma-relevant cognitions that are distorted or unhelpful. Trauma-relevant cognitions fall into five themes that are highlighted during treatment: safety, trust, power/control, esteem, and intimacy. The empirical base for CPT is strong with numerous studies demonstrating that it results in significant reduction of PTSD symptoms regardless of trauma type (Asmundson et al., Citation2019; Kline et al., Citation2018; Watts et al., Citation2013) and that it is 89% more effective than control treatment (Watts et al., Citation2013). CPT has been successfully implemented in virtual formats with comparable efficacy levels to that of in-person CPT (Held, Coleman, et al., Citation2022; Held, Kovacevic, et al., Citation2022). For feasibility purposes, including the increased incidence of CPT training in the United States (Finley et al., Citation2019; Richards et al., Citation2017) and efficacy of telehealth provision (Held, Coleman, et al., Citation2022; Held, Kovacevic, et al., Citation2022), CPT was chosen over other first-line PTSD treatments. CPT sessions for this study will be conducted virtually by a CPT-trained clinician.
3. Data analytic plan
3.1. Data analysis plan
Analyses for primary endpoints will focus on between-group differences (CPT vs. waitlist control) in baseline adjusted change in inflammation (arterial inflammation; Aim 1) and autonomic function (HRV; Aim 2). We will use a superiority trial design with two-sided alpha = 0.05. All analyses will use the intent-to-treat sample. We hypothesise that there will be greater reduction in inflammation among participants randomised to CPT vs. waitlist controls (Aim 1), as indicated by greater reduction in arterial inflammation. We will use a one-way analysis of covariance (ANCOVA) to compare baseline adjusted change in arterial inflammation among those in CPT (group 1) vs. waitlist controls (group 2). Secondary analyses will include one-way ANCOVAs to compare baseline adjusted decreases in leukopoiesis, which we hypothesise will be greater among those in CPT vs. waitlist controls. Similarly, we hypothesise that there will be greater improvement in autonomic function among participants randomised to CPT vs. waitlist controls (Aim 2), as indicated by a greater increase in HRV. We will use a one-way ANCOVA to compare baseline adjusted changes in HRV among those in CPT (group 1) vs. waitlist controls (group 2). Secondary analyses will include one-way ANCOVAs to compare baseline adjusted decreases in HR and BP, which we hypothesise will be greater among those in CPT vs. waitlist controls. The ANCOVAs for both aims will include age and sex as covariables, as well as any health-related behaviours found to be associated with outcomes. Exploratory analyses will examine the effect of CPT on MRI endpoints using ANCOVAs, as described for primary endpoints. DTI data will be used to understand the axonal integrity of connections between important brain regions before and after the intervention. For example, in a recent manuscript we saw that individuals with PTSD had decreased axonal integrity of the uncinate (connection between the amygdala and frontal cortex) (Gharios, Van Leent, et al., Citation2024).
3.1.1. Indirect effects of SNA
Given our hypothesis that CPT will reduce CVD risk mechanisms via alterations in SNA, we will test the indirect effect of SNA on the associations between CPT and inflammation and autonomic function using the Process Macro in SPSS (IBM Corporation, Armonk, NY, USA). Age and sex will be included as covariables. The difference in SNA (resting fMRI data) from baseline to post-treatment will be calculated. We expect that SNA will mediate CPT-related changes in inflammation and autonomic function. Given the small sample size, these estimates will be used to inform effect sizes for future studies.
3.2. Power
Sample size was determined based on the amalgamation of power analyses for primary outcomes. For inflammation, the average baseline value of arterial inflammation is expected to be 2.24 (as a TBR of arterial SUV to background venous SUV) based on data from a prior FDG-PET study (Tawakol et al., Citation2013), and a reduction of 12% is expected based on prior researchFootnote1 indicating the effect statin intensification over 12-weeks (Tawakol et al., Citation2013). The assumed standard deviation of change in arterial inflammation is 0.23 (Tawakol et al., Citation2013). Accordingly, a sample size of 26, with 13 in each group, will provide >80% power to detect a between-groups difference of 0.27 (12% reduction from baseline of 2.24). For autonomic function, the average baseline value of high frequency HRV is expected to be approximately 157.56 ms2 with a standard deviation of 31.99 ms2 based on data from a PTSD sample (Bourassa et al., Citation2021; Seligowski et al., Citation2021). If high frequency HRV increases by 25% with CPT (healthy control high frequency HRV: 332.83 ms2), a sample size of 24 (12 in each group) will ensure >80% power to detect a between-groups difference. Using a study dropout rate of 10%, a total sample size of 30 (15 in each group) will provide >80% power to detect between-group differences for both primary outcomes in the current study.
4. Discussion
The elevated rate of CVD among individuals with PTSD is of significant public health concern and highlights the need for greater understanding of the PTSD-CVD link. The prior literature and our preliminary work (Osborne et al., Citation2021; Seligowski et al., Citation2021; Seligowski et al., Citation2024; Tawakol et al., Citation2017) support the scientific premise that autonomic function (HRV, HR, BP) and inflammation (leukopoiesis, arterial inflammation) are integral mechanisms that contribute to the link between PTSD and elevated CVD risk. Further, there is evidence that SNA mediates the PTSD-CVD link via connecting stress-responsive neural centres to the periphery (Seligowski et al., Citation2024). Accordingly, it would logically follow that reductions in PTSD symptoms would be associated with neural alterations that confer changes in CVD risk mechanisms; however, this hypothesis has yet to be empirically tested. The current study seeks to assess the impact of a first-line treatment for PTSD on inflammation and autonomic function, and to determine whether changes in these mechanisms are driven by changes in SNA among a sample with PTSD and elevated CVD risk.
A major strength of this study is that it is the first to leverage state-of-the-art neural and peripheral FDG-PET/MRI to determine how CVD risk mechanisms are influenced by existing PTSD treatment. While neural alterations in PTSD are well-characterised (Shin et al., Citation2004; Shin et al., Citation2005; Stevens et al., Citation2013; Williams et al., Citation2006), there is a paucity of research showing how they are affected by gold-standard PTSD treatments. Further, no prior study to date has tested how alterations in the brain impact markers of CVD risk in PTSD. This study will use state-of-the-art FDG-PET/MRI brain and peripheral imaging to assess whether (1) the neural regions involved in SNA are affected by PTSD treatment, and (2) determine how these changes in SNA following PTSD treatment affect peripheral mechanisms that contribute to CVD risk. Given the high metabolic activity of inflammatory cells and neural tissues and their potential roles in this pathway, FDG-PET is uniquely suited to characterise the relationships between metabolic activities of disparate organs (e.g., brain, arteries) (Osborne et al., Citation2021). Furthermore, inflammation of the bone marrow (leukopoiesis) and arteries on FDG-PET strongly predict CVD events, and SNA measured via FDG-PET provides an objective measure that associates with stress, inflammation, and CVD (Osborne et al., Citation2021). Moreover, MRI allows for the assessment of brain structure, connectivity, activation, and axonal integrity, as well as arterial structure to complement these other assessments. As such, our validated FDG-PET/MRI protocol provides a state-of-the-art imaging evaluation that facilitates a uniquely broad evaluation of the brain, bone marrow, and arteries, and the impact of an intervention on these measures. Other strengths of this study include the use of a gold-standard, first-line treatment for PTSD, and the inclusion of men and women with diverse trauma histories, subthreshold PTSD, and elevated CVD risk factors that are frequently excluded from clinical trials in PTSD (e.g., hypertension, smoking).
There are limitations of the current study design. First, although the study is adequately powered for primary outcomes, an assessment of SNA’s role in the changes observed will need to be replicated in a larger sample. Second, the current study does not include follow-up beyond 12-weeks and, therefore, cannot draw long-term conclusions about the maintenance of treatment effects on mechanisms of CVD risk or future CVD events. Third, inclusion and exclusion criteria may limit generalizability of findings to other populations with known PTSD and CVD risk (e.g., those with moderate or severe substance use disorders [Gan et al., Citation2021; Kessler et al., Citation1995] and older adults [Benjamin et al., Citation2019; Byers et al., Citation2014]). Fourth, results may require replication in populations meeting full criteria for PTSD as the inclusion of subthreshold PTSD may lead to lower overall PTSD severity in the sample; however, the inclusion of these participants is also a strength given subthreshold PTSD is associated with significant functional impairment (Pietrzak et al., Citation2011) and comparable treatment response to those with PTSD (Dickstein et al., Citation2013). Similarly, given the small sample, heterogeneity in CVD risk severity could result in null findings. As such, results will inform CVD risk-related eligibility for future studies and will require replication in a larger sample. Finally, the use of a waitlist control will provide preliminary evidence for the effects of CPT on CVD risk and SNA, however, direct comparison to other interventions for CVD (e.g. exercise, medication) will be needed to determine the relative efficacy of CPT for CVD risk. Future research will also be needed to evaluate the impact of other frontline-PTSD treatments on CVD risk mechanisms.
Because CVD represents the leading cause of death in the US and individuals with PTSD demonstrate elevated CVD risk, research characterising PTSD-CVD mechanisms is critically needed. This study will test whether CPT, a gold-standard treatment for PTSD, associates with changes in CVD risk mechanisms, and whether these changes are driven by neural alterations. These results have the potential to better characterise the nature of the PTSD-CVD link and identify potential targets for intervention with the goal of reducing the incidence of CVD events in this at-risk population.
Data availability statement
Data sharing not applicable – no new data generated.
Disclosure statement
Michael Osborne declared unrelated consultation for WCG Clinical.
Additional information
Funding
Notes
1 Preliminary data from a medication trial conducted by our group was used to estimate a clinically meaningful intervention effect for arterial inflammation within the timeframe of CPT.
References
- Abdallah, C. G., Averill, C. L., Ramage, A. E., Averill, L. A., Alkin, E., Nemati, S., Krystal, J. H., Roache, J. D., Patricia, A. R., Young-McCaughan, S., Peterson, A. L., & Fox, P. (2019). Reduced salience and enhanced central executive connectivity following PTSD treatment. Chronic Stress, 3, 247054701983897. https://doi.org/10.1177/2470547019838971
- Asmundson, G. J. G., Thorisdottir, A. S., Roden-Foreman, J. W., Baird, S. O., Witcraft, S. M., Stein, A. T., Smits, J. A. J., & Powers, M. B. (2019). A meta-analytic review of cognitive processing therapy for adults with posttraumatic stress disorder. Cognitive Behaviour Therapy, 48(1), 1–14. https://doi.org/10.1080/16506073.2018.1522371
- Association AP. (2013). DSM-5 the diagnostic and statistical manual of mental disorders. (5th ed.). American Psychiatric Publishing.
- Aupperle, R. L., Allard, C. B., Simmons, A. N., Flagan, T., Thorp, S. R., Norman, S. B., Paulus, M. P., & Stein, M. B. (2013). Neural responses during emotional processing before and after cognitive trauma therapy for battered women. Psychiatry Research: Neuroimaging, 214(1), 48–55. https://doi.org/10.1016/j.pscychresns.2013.05.001
- Beck, A. T., Steer, R. A., & Brown, G. (1996). Beck Depression Inventory–II. PsycTESTS Dataset. Published online 1996.
- Benjamin, E. J., Muntner, P., Alonso, A., Bittencourt, M. S., Callaway, C. W., Carson, A. P., Chamberlain, A. M., Chang, A. R., Cheng, S., Das, S. R., Delling, F. N., Djousse, L., Elkind, M. S. V., Ferguson, J. F., Fornage, M., Jordan, L. C., Khan, S. S., Kissela, B. M., Knutson, K. L., … Virani, S. S. (2019). Heart disease and stroke statistics—2019 update: A report from the American Heart Association. Circulation, 139(10), e56–e528. https://doi.org/10.1161/CIR.0000000000000659
- Booth, M. (2000). Assessment of physical activity: An international perspective. Research Quarterly for Exercise and Sport, 71(Suppl 2), 114–120. https://doi.org/10.1080/02701367.2000.11082794
- Bourassa, K. J., Hendrickson, R. C., Reger, G. M., & Norr, A. M. (2021). Posttraumatic stress disorder treatment effects on cardiovascular physiology: A systematic review and agenda for future research. Journal of Traumatic Stress, 34(2), 384–393. https://doi.org/10.1002/jts.22637
- Brudey, C., Park, J., Wiaderkiewicz, J., Kobayashi, I., Mellman, T. A., & Marvar, P. J. (2015). Autonomic and inflammatory consequences of posttraumatic stress disorder and the link to cardiovascular disease. American Journal of Physiology-Regulatory, Integrative and Comparative Physiology, 309(4), R315–R321. https://doi.org/10.1152/ajpregu.00343.2014
- Buckley, T. C., & Kaloupek, D. G. (2001). A meta-analytic examination of basal cardiovascular activity in posttraumatic stress disorder. Psychosomatic Medicine, 63(4), 585–594. https://doi.org/10.1097/00006842-200107000-00011
- Buysse, D. J., Reynolds, C. F., Monk, T. H., Berman, S. R., & Kupfer, D. J. (1989). The Pittsburgh Sleep Quality Index: A new instrument for psychiatric practice and research. Psychiatry Research, 28(2), 193–213. https://doi.org/10.1016/0165-1781(89)90047-4
- Byers, A. L., Covinsky, K. E., Neylan, T. C., & Yaffe, K. (2014). Chronicity of posttraumatic stress disorder and risk of disability in older persons. JAMA Psychiatry, 71(5), 540–546. https://doi.org/10.1001/jamapsychiatry.2014.5
- Cecelja, M., & Chowienczyk, P. (2012). Role of arterial stiffness in cardiovascular disease. JRSM Cardiovascular Disease, 1(4), 1–10. https://doi.org/10.1258/cvd.2012.012016
- Centers for Disease Control and Prevention, National Center for Health Statistics. (2021). About multiple cause of death, 1999–2020. CDC WONDER Online Database website.
- Chen, Y., Shen, Q., Lichtenstein, P., Gradus, J. L., Arnberg, F. K., Larsson, H., D’Onofrio, B. M., Fang, F., Song, H., & Valdimarsdottir, U. A. (2024). Incidence trajectories of psychiatric disorders after assault, injury, and bereavement. JAMA Psychiatry, 81(4), 374–385. https://doi.org/10.1001/jamapsychiatry.2023.5156
- Dickstein, B. D., Walter, K. H., Schumm, J. A., & Chard, K. M. (2013). Comparing response to cognitive processing therapy in military veterans with subthreshold and threshold posttraumatic stress disorder. Journal of Traumatic Stress, 26(6), 703–709. https://doi.org/10.1002/jts.21869
- Edmondson, D., & Cohen, B. E. (2013). Posttraumatic stress disorder and cardiovascular disease. Progress in Cardiovascular Diseases, 55(6), 548–556. https://doi.org/10.1016/j.pcad.2013.03.004
- Edmondson, D., & Känel, R. (2017). Post-traumatic stress disorder and cardiovascular disease. The Lancet Psychiatry, 4(4), 320–329. https://doi.org/10.1016/S2215-0366(16)30377-7
- Ehlers, A., Suendermann, O., Boellinghaus, I., Vossbeck-Elsebusch, A., Gamer, M., Briddon, E., Martin, M. W., & Glucksman, E. (2010). Heart rate responses to standardized trauma-related pictures in acute posttraumatic stress disorder. International Journal of Psychophysiology, 78(1), 27–34. https://doi.org/10.1016/j.ijpsycho.2010.04.009
- Emami, H., Singh, P., & MacNabb, M. (2015). Splenic metabolic activity predicts risk of future cardiovascular events. JACC: Cardiovascular Imaging, 8(2), 121–130. https://doi.org/10.1016/j.jcmg.2014.10.009
- Finley, E. P., Mader, M., Haro, E. K., Noël, P. H., Bernardy, N., Rosen, C. S., Bollinger, M., Garcia, H. A., Sherrieb, K., & Pugh, M. J. V. (2019). Use of guideline-recommended treatments for PTSD among community-based providers in Texas and Vermont: Implications for the veterans choice program. The Journal of Behavioral Health Services & Research, 46(2), 217–233. https://doi.org/10.1007/s11414-018-9613-z
- Foa, E. B., Hembree, E., & Rothbaum, B. (2007). Prolonged exposure therapy for PTSD: Therapist guide: Emotional processing of traumatic experiences. Oxford University Press. https://doi.org/10.1093/med:psych/9780195308501.001.0001
- Gan, W., Buxton, J., Scheuermeyer, F., Palis, H., Zhao, B., Desai, R., Janjua, N. Z., & Slaunwhite, A. K. (2021). Risk of cardiovascular diseases in relation to substance use disorders. Drug and Alcohol Dependence, 229, 109132. https://doi.org/10.1016/j.drugalcdep.2021.109132
- Ge, F., Yuan, M., Li, Y., & Zhang, W. (2020). Posttraumatic stress disorder and alterations in resting heart rate variability: A systematic review and meta-analysis. Psychiatry Investigation, 17(1), 9–20. https://doi.org/10.30773/pi.2019.0112
- Gharios, C., Van Leent, M. M. T., Chang, H. L., Abohashem, S., O’Connor, D., Osborne, M. T., Tang, C. Y., Kaufman, A. E., Robson, P. M., Ramachandran, S., Calcagno, C., Mani, V., Trivieri, M. G., Seligowski, A. V., Dekel, S., Mulder, W. J. M., Murrough, J. W., Shin, L. M., Tawakol, A., & Fayad, Z. A. (2024). Cortico-limbic interactions and carotid atherosclerotic burden during chronic stress exposure. European Heart Journal, 45(19), 1753–1764. https://doi.org/10.1093/eurheartj/ehae149
- Goldstein, R. B., Smith, S. M., Chou, S. P., Saha, T. D., Jung, J., Zhang, H., Pickering, R. P., Ruan, W. J., Huang, B., & Grant, B. F. (2016). The epidemiology of DSM-5 posttraumatic stress disorder in the United States: Results from the National Epidemiologic Survey on Alcohol and Related Conditions-III. Social Psychiatry and Psychiatric Epidemiology, 51(8), 1137–1148. https://doi.org/10.1007/s00127-016-1208-5
- Hariri, A. R., Bookheimer, S. Y., & Mazziotta, J. C. (2000). Modulating emotional responses. NeuroReport, 11(1), 43. https://doi.org/10.1097/00001756-200001170-00009
- Held, P., Coleman, J. A., & Petrey, K. (2022). A case series examining PTSD and depression symptom reductions over the course of a 2-week virtual intensive PTSD treatment program for veterans. Psychological Trauma: Theory, Research, Practice, and Policy, 14(4), 615–623. https://doi.org/10.1037/tra0001106
- Held, P., Kovacevic, M., & Petrey, K. (2022). Treating posttraumatic stress disorder at home in a single week using 1-week virtual massed cognitive processing therapy. Journal of Traumatic Stress, 35(4), 1215–1225. https://doi.org/10.1002/jts.22831
- Helpman, L., Marin, M. F., Papini, S., Zhu, X., Sullivan, G. M., Schneier, F., Neria, M., Shvil, E., Malaga Aragon, M. J., Markowitz, J. C., Lindquist, M. A., Wager, T. D., Milad, M. R., & Neria, Y. (2016). Neural changes in extinction recall following prolonged exposure treatment for PTSD: A longitudinal fMRI study. NeuroImage: Clinical, 12, 715–723. https://doi.org/10.1016/j.nicl.2016.10.007
- Hendrickson, R. C., & Raskind, M. A. (2016). Noradrenergic dysregulation in the pathophysiology of PTSD. Experimental Neurology, 284, 181–195. https://doi.org/10.1016/j.expneurol.2016.05.014
- Jovanovic, T., Norrholm, S. D., Sakoman, A. J., Esterajher, S., & Kozarić-Kovačić, D. (2009). Altered resting psychophysiology and startle response in Croatian combat veterans with PTSD. International Journal of Psychophysiology, 71(3), 264–268. https://doi.org/10.1016/j.ijpsycho.2008.10.007
- Kessler, R. C., Sonnega, A., Bromet, E., Hughes, M., & Nelson, C. B. (1995). Posttraumatic stress disorder in the National Comorbidity Survey. Archives of General Psychiatry, 52(12), 1048–1060. https://doi.org/10.1001/archpsyc.1995.03950240066012
- Kim, T. D., Lee, S., & Yoon, S. (2020). Inflammation in Post-Traumatic Stress Disorder (PTSD): A review of potential correlates of PTSD with a neurological perspective. Antioxidants, 9(2), 107. https://doi.org/10.3390/antiox9020107
- Klein, A. B., Schnurr, P. P., Bovin, M. J., Friedman, M. J., Keane, T. M., & Marx, B. P. (2024). An empirical investigation of definitions of subthreshold posttraumatic stress disorder. Journal of Traumatic Stress, 37(1), 113–125. https://doi.org/10.1002/jts.22987
- Kline, A. C., Cooper, A. A., Rytwinksi, N. K., & Feeny, N. C. (2018). Long-term efficacy of psychotherapy for posttraumatic stress disorder: A meta-analysis of randomized controlled trials. Clinical Psychology Review, 59, 30–40. https://doi.org/10.1016/j.cpr.2017.10.009
- Lima, B. B., Hammadah, M., Wilmot, K., Pearce, B. D., Shah, A., & Levantsevych, O. (2019). Posttraumatic stress disorder is associated with enhanced interleukin-6 response to mental stress in subjects with a recent myocardial infarction. Brain, Behavior, and Immunity, 75, 26–33. https://doi.org/10.1016/j.bbi.2018.08.015
- Manthey, A., Sierk, A., Brakemeier, E. L., Walter, H., & Daniels, J. K. (2021). Does trauma-focused psychotherapy change the brain? A systematic review of neural correlates of therapeutic gains in PTSD. European Journal of Psychotraumatology, 12(1), 1929025. https://doi.org/10.1080/20008198.2021.1929025
- McCarty, R. (2016). Chapter 4 – the fight-or-flight response: A cornerstone of stress research. In G. Fink (Ed.), Stress: Concepts, cognition, emotion, and behavior (pp. 33–37). Academic Press. https://doi.org/10.1016/B978-0-12-800951-2.00004-2
- Moon, E., Lee, S. H., Kim, D. H., & Hwang, B. (2013). Comparative study of heart rate variability in patients with schizophrenia, bipolar disorder, post-traumatic stress disorder, or major depressive disorder. Clinical Psychopharmacology and Neuroscience, 11(3), 137–143. https://doi.org/10.9758/cpn.2013.11.3.137
- O’Donovan, A., Neylan, T. C., Metzler, T., & Cohen, B. E. (2012). Lifetime exposure to traumatic psychological stress is associated with elevated inflammation in the Heart and Soul Study. Brain, Behavior, and Immunity, 26(4), 642–649. https://doi.org/10.1016/j.bbi.2012.02.003
- Osborne, M. T., Abohashem, S., Zureigat, H., Abbasi, T. A., & Tawakol, A. (2021). Multimodality molecular imaging: Gaining insights into the mechanisms linking chronic stress to cardiovascular disease. Journal of Nuclear Cardiology, 28(3), 955–966. https://doi.org/10.1007/s12350-020-02424-6
- Pietrzak, R. H., Goldstein, R. B., Southwick, S. M., & Grant, B. F. (2011). Prevalence and Axis I comorbidity of full and partial posttraumatic stress disorder in the United States: Results from Wave 2 of the National Epidemiologic Survey on Alcohol and Related Conditions. Journal of Anxiety Disorders, 25(3), 456–465. https://doi.org/10.1016/j.janxdis.2010.11.010
- Radfar, A., Abohashem, S., Osborne, M. T., Wang, Y., Dar, T., Hassan, M. Z. O., Ghoneem, A., Naddaf, N., Patrich, T., Abbasi, T., Zureigat, H., Jaffer, J., Ghazi, P., Scott, J. A., Shin, L. M., Pitman, R. K., Neilan, T. G., Wood, M. J., & Tawakol, A. (2021). Stress-associated neurobiological activity associates with the risk for and timing of subsequent Takotsubo syndrome. European Heart Journal, 42(19), 1898–1908. https://doi.org/10.1093/eurheartj/ehab029
- Resick, P. A., Monson, C. M., & Chard, K. M. (2017). Cognitive processing therapy for PTSD: A comprehensive manual. Guilford Press.
- Richards, L. K., Bui, E., Charney, M., Hayes, K. C., Baier, A. L., Rauch, P. K., Allard, M., & Simon, N. M. (2017). Treating veterans and military families: Evidence based practices and training needs among community clinicians. Community Mental Health Journal, 53(2), 215–223. https://doi.org/10.1007/s10597-016-0013-7
- Ruparelia, N., Chai, J. T., Fisher, E. A., & Choudhury, R. P. (2017). Inflammatory processes in cardiovascular disease: A route to targeted therapies. Nature Reviews Cardiology, 14(3), 133–144. https://doi.org/10.1038/nrcardio.2016.185
- Santarnecchi, E., Bossini, L., Vatti, G., Fagiolini, A., La Porta, P., Di Lorenzo, G., Siracusano, A., Rossi, S., & Rossi, A. (2019). Psychological and brain connectivity changes following trauma-focused CBT and EMDR treatment in single-episode PTSD patients. Frontiers in Psychology, 10, 129. https://doi.org/10.3389/fpsyg.2019.00129
- Schaefer, S. M., Abercrombie, H. C., Lindgren, K. A., Larson, C. L., Ward, R. T., Oakes, T. R., Holden, J. E., Perlman, S. B., Turski, P. A., & Davidson, R. J. (2000). Six-month test-retest reliability of MRI-defined PET measures of regional cerebral glucose metabolic rate in selected subcortical structures. Human Brain Mapping, 10(1), 1–9. https://doi.org/10.1002/(SICI)1097-0193(200005)10:1<1::AID-HBM10>3.0.CO;2-O
- Schneider, M., & Schwerdtfeger, A. (2020). Autonomic dysfunction in posttraumatic stress disorder indexed by Heart Rate Variability: A meta-analysis. Psychological Medicine, 50(12), 1937–1948. https://doi.org/10.1017/S003329172000207X
- Seligowski, A. V., Grewal, S. S., Abohashem, S., Zureigat, H., Qamar, I., Aldosoky, W., Gharios, C., Hanlon, E., Alani, O., Bollepalli, S. C., Armoundas, A., Fayad, Z. A., Shin, L. M., Osborne, M. T., & Tawakol, A. (2024). PTSD increases risk for major adverse cardiovascular events through neural and cardio-inflammatory pathways. Brain, Behavior, and Immunity, 117, 149–154. https://doi.org/10.1016/j.bbi.2024.01.006
- Seligowski, A. V., Steuber, E. R., Hinrichs, R., Reda, M. H., Wiltshire, C. N., Wanna, C. P., Winters, S. J., Phillips, K. A., House, S. L., Beaudoin, F. L., An, X., Stevens, J. S., Zeng, D., Neylan, T. C., Clifford, G. D., Linnstaedt, S. D., Germine, L. T., Bollen, K. A., Guffanti, G., … Jovanovic, T. (2021). A prospective examination of sex differences in posttraumatic autonomic functioning. Neurobiology of Stress, 15, 100384. https://doi.org/10.1016/j.ynstr.2021.100384
- Shin, L. M., Orr, S. P., Carson, M. A., Rauch, S. L., Macklin, M. L., Lasko, N. B., Peters, P. M., Metzger, L. J., Dougherty, D. D., Cannistraro, P. A., Alpert, N. M., Fischman, A. J., & Pitman, R. K. (2004). Regional cerebral blood flow in the amygdala and medial prefrontal cortex during traumatic imagery in male and female Vietnam veterans With PTSD. Archives of General Psychiatry, 61(2), 168–176. https://doi.org/10.1001/archpsyc.61.2.168
- Shin, L. M., Wright, C. I., Cannistraro, P. A., Wedig, M. M., McMullin, K., Martis, B., Macklin, M. L., Lasko, N. B., Cavanagh, S. R., Krangel, T. S., Orr, S. P., Pitman, R. K., Whalen, P. J., & Rauch, S. L. (2005). A functional magnetic resonance imaging study of amygdala and medial prefrontal cortex responses to overtly presented fearful faces in posttraumatic stress disorder. Archives of General Psychiatry, 62(3), 273–281. https://doi.org/10.1001/archpsyc.62.3.273
- Spielberger, C. D. (1983). State-Trait Anxiety Inventory for adults. PsycTESTS Dataset. Published online 1983.
- Stevens, J. S., Jovanovic, T., Fani, N., Ely, T. D., Glover, E. M., Bradley, B., & Ressler, K. J. (2013). Disrupted amygdala-prefrontal functional connectivity in civilian women with posttraumatic stress disorder. Journal of Psychiatric Research, 47(10), 1469–1478. https://doi.org/10.1016/j.jpsychires.2013.05.031
- Sumner, J. A., Nishimi, K. M., Koenen, K. C., Roberts, A. L., & Kubzansky, L. D. (2020). Posttraumatic stress disorder and inflammation: Untangling issues of bidirectionality. Biological Psychiatry, 87(10), 885–897. https://doi.org/10.1016/j.biopsych.2019.11.005
- Task Force of the European Society of Cardiology the North A E. (1996). Heart rate variability. Circulation, 93(5), 1043–1065. https://doi.org/10.1161/01.CIR.93.5.1043
- Tawakol, A., Fayad, Z. A., Mogg, R., Alon, A., Klimas, M. T., Dansky, H., Subramanian, S. S., Abdelbaky, A., Rudd, J. H. F., Farkouh, M. E., Nunes, I. O., Beals, C. R., & Shankar, S. S. (2013). Intensification of statin therapy results in a rapid reduction in atherosclerotic inflammation. Journal of the American College of Cardiology, 62(10), 909–917. https://doi.org/10.1016/j.jacc.2013.04.066
- Tawakol, A., Ishai, A., Takx, R. A., Figueroa, A. L., Ali, A., Kaiser, Y., Truong, Q. A., Solomon, C. J., Calcagno, C., Mani, V., Tang, C. Y., Mulder, W. J., Murrough, J. W., Hoffmann, U., Nahrendorf, M., Shin, L. M., Fayad, Z. A., & Pitman, R. K. (2017). Relation between resting amygdalar activity and cardiovascular events: A longitudinal and cohort study. The Lancet, 389(10071), 834–845. https://doi.org/10.1016/S0140-6736(16)31714-7
- VA/DoD Clinical Practice Guideline. (2023). Management of posttraumatic stress disorder and acute stress disorder work group. U.S. Government Printing Office.
- Watkins, L. L., LoSavio, S. T., Calhoun, P., Resick, P. A., Sherwood, A., Coffman, C. J., Kirby, A. C., Beaver, T. A., Dennis, M. F., & Beckham, J. C. (2023). Effect of cognitive processing therapy on markers of cardiovascular risk in posttraumatic stress disorder patients: A randomized clinical trial. Journal of Psychosomatic Research, 170, 111351. https://doi.org/10.1016/j.jpsychores.2023.111351
- Watts, B. V., Schnurr, P. P., Mayo, L., Young-Xu, Y., Weeks, W. B., & Friedman, M. J. (2013). Meta-analysis of the efficacy of treatments for posttraumatic stress disorder. The Journal of Clinical Psychiatry, 74(6), e541–e550. https://doi.org/10.4088/JCP.12r08225
- Weathers, F. W., Blake, D. D., Schnurr, P. P., Kaloupek, D. G., Marx, B. P., & Keane, T. M. (2013). The Life Events Checklist for DSM-5 (LEC-5). Instrument available from the National Center for PTSD. www.ptsd.va.gov.
- Weathers, F. W., Blake, D. D., Schnurr, P. P., Kaloupek, D. G., Marx, B. P., & Keane, T. M. (2013). The Clinician-Administered PTSD Scale for DSM-5 (CAPS-5) [Assessment]. Published online 2013. www.ptsd.va.gov.
- Weathers, F. W., Litz, B. T., Keane, T. M., Palmieri, P. A., Marx, B. P., & Schnurr, P. P. (2013). The PTSD checklist for DSM-5 (PCL-5). Published online 2013. www.ptsd.va.gov.
- Williams, L. M., Kemp, A. H., Felmingham, K., Barton, M., Olivieri, G., Peduto, A., Gordon, E., & Bryant, R. A. (2006). Trauma modulates amygdala and medial prefrontal responses to consciously attended fear. Neuroimage, 29(2), 347–357. https://doi.org/10.1016/j.neuroimage.2005.03.047
- Zhu, X., Suarez-Jimenez, B., Lazarov, A., Helpman, L., Papini, S., Lowell, A., Durosky, A., Lindquist, M. A., Markowitz, J. C., Schneier, F., Wager, T. D., & Neria, Y. (2018). Exposure-based therapy changes amygdala and hippocampus resting-state functional connectivity in patients with posttraumatic stress disorder. Depression and Anxiety, 35(10), 974–984. https://doi.org/10.1002/da.22816