ABSTRACT
Background
Childhood adversity has been associated with greater risk of developing psychopathology, altered processing of emotional stimuli, and changes in neural functioning. Although the neural correlates of rumination have been previously described, little is known about how adverse childhood experiences are related to brain functioning during rumination.
Objective
This study explored differences in neural functional connectivity between participants with and without histories of childhood adversity, controlling for tendency to ruminate, during resting-state and induction of rumination.
Method
A total of 86 adults (51 women) took part. Based on a diagnostic clinical interview, participants were divided into groups with and without adverse childhood experiences. All participants underwent resting-state imaging and a functional magnetic resonance imaging scan where they performed a rumination induction task.
Results
Individuals with childhood adversities differed from those without adverse experiences in seed-based functional connectivity from right angular gyrus and left superior frontal gyrus during the rumination task. There were also group differences during resting-state in seed-based functional connectivity from the right angular gyrus, left middle temporal gyrus, and left superior frontal gyrus.
Conclusions
Childhood adversity is associated with altered brain functioning during rumination and resting-state, even after controlling for tendency to ruminate. Our results shed light on the consequences of early adversity. People who experienced childhood adversities differ from those with no adverse experiences in brain functional connectivity when engaged in negative repetitive self-referential thinking.
HIGHLIGHTS
This study focuses on the relationship between the presence of childhood adversity and brain activation during rumination in adults.
Childhood adversity is associated with aberrant functional connectivity during rumination as well as resting-state
Antecedentes: La adversidad en la infancia se ha asociado con un mayor riesgo de desarrollar psicopatología, alteración del procesamiento de estímulos emocionales y cambios en el funcionamiento neuronal. Aunque los correlatos neuronales de la rumiación se han descrito previamente, se sabe poco acerca de cómo las experiencias adversas en la infancia se relacionan con el funcionamiento del cerebro durante la rumiación.
Objetivo: Este estudio exploró las diferencias en la conectividad neuro funcional entre participantes con y sin antecedentes de adversidad en la infancia, controlando la tendencia a rumiar, durante el estado de reposo y la inducción de la rumiación.
Método: Participaron un total de 86 adultos (51 mujeres). Basado en una entrevista clínica de diagnóstico, los participantes se dividieron en grupos con y sin experiencias adversas en infancia. Todos los participantes se sometieron a imágenes en estado de reposo y a una resonancia magnética funcional en la que realizaron una tarea de inducción de la rumiación.
Resultados: Los individuos con adversidades en la infancia diferían de aquellos sin experiencias adversas en la conectividad funcional basada en semillas de la circunvolución angular derecha y la circunvolución frontal superior izquierda durante la tarea de rumiación. También hubo diferencias de grupo durante el estado de reposo en la conectividad funcional basada en semillas de la circunvolución angular derecha, la circunvolución temporal media izquierda y la circunvolución frontal superior izquierda.
Conclusiones: La adversidad en la infancia se asocia con un funcionamiento cerebral alterado durante la rumiación y el estado de reposo, incluso después de controlar la tendencia a rumiar. Nuestros resultados aclaran las consecuencias de la adversidad temprana. Las personas que experimentaron adversidades en la infancia difieren de aquellas que no tuvieron experiencias adversas en la conectividad funcional del cerebro cuando se dedican al pensamiento autorreferencial repetitivo negativo.
背景:童年逆境与发展精神病理学、情绪刺激处理改变和神经功能变化的风险增加有关。尽管之前已经描述了反刍的神经相关性,对于童年的不良经历与反刍期间的大脑功能之间的关系知之甚少。
目的:本研究探讨了有无童年逆境史的参与者之间神经功能连接的差异,控制了反刍倾向、静息状态和反刍诱导。
方法:共有 86 名成年人(51 名女性)参加。根据一项诊断性临床访谈,参与者被分为有、无不良童年经历组。所有参与者都接受了静息状态成像和执行反刍诱导任务的功能性磁共振成像扫描。
结果:在反刍任务期间,有童年逆境的个体与没有不良经历的个体在来自右角回和左额上回的基于种子的功能连接不同。在静息状态下,右侧角回、左侧颞中回和左侧额上回的基于种子的功能连接也存在组间差异。
结论:童年逆境与反刍和静息状态下大脑功能的改变有关,即使在控制了反刍倾向之后也是如此。我们的结果揭示了早期逆境的后果。经历过童年逆境的人与那些没有不良经历的人在进行消极重复的自我参照思维时大脑功能连接不同。
PALABRAS CLAVE:
1. Introduction
Adverse childhood experiences (ACEs) refer to a broad range of negative childhood experiences that result in actual or potential harm to a child (Butchart, Harvey, Mian, Fürniss, & Kahane, Citation2006). ACEs include, but are not limited to, neglect, physical, sexual and emotional abuse, parental death or loss, bullying, and poverty. It is well established that ACEs increase the risk of emotional disorders in short- and long-term perspectives (Bellis et al., Citation2019; Kalmakis & Chandler, Citation2015; Nelson et al., Citation2020; Nurius, Green, Logan-Greene, & Borja, Citation2015). Early stressors, particularly those that are traumatic, interpersonal, and chronic, are associated with greater rates of depression, anxiety, posttraumatic stress disorder (PTSD), suicidal behaviours, and substance use disorders (e.g. Carrion, Weems, Ray, & Reiss, Citation2002; Ford, Stockton, Kaltman, & Green, Citation2006; Widom, DuMont, & Czaja, Citation2007). Given the range of adverse life outcomes, exposure to ACEs is considered a transdiagnostic risk factor for various forms of psychopathology later in life (De Bellis & Zisk, Citation2014).
Literature on biological mechanisms that link ACEs and adverse life outcomes suggests that stress triggers a cascade of physiological events leading to enduring neurobiological alterations in susceptible individuals (De Bellis & Zisk, Citation2014; Nelson et al., Citation2020; Teicher, Samson, Anderson, & Ohashi, Citation2016). Outcomes are associated with differences in biological stress symptoms as well as brain structure, function, and connectivity. Numerous neuroimaging studies have demonstrated that childhood trauma affects brain development in multiple brain regions, negatively impacting emotional and behavioural regulation, motivation, and cognitive function (e.g. Heany et al., Citation2018; Hein & Monk, Citation2017; Lim, Radua, & Rubia, Citation2014). Teicher et al. (Citation2016) described several structural and functional alterations that follow childhood maltreatment. Adversity negatively correlates with resting-state connectivity between subcortical and cortical regions, for example, amygdala – anterior cingulate cortex (ACC) coupling. Connectivity of neural networks in maltreated individuals is characterised by increased importance of the anterior insula and precuneus but decreased engagement of the ACC, middle frontal pole, and temporal pole (Teicher et al., Citation2016). De Bellis and Zisk (Citation2014) note that trauma-related alterations reflect executive, attentional, and affective emotional dysregulation similar to both children and adolescents experiencing adversities as well as adults with histories of ACEs. For example, one study on adults with PTSD related to childhood maltreatment (Bremner et al., Citation1999) revealed that they had decreased activation in the dorsal control networks with corresponding increased activation of the amygdala and hippocampus and other structures of the affective emotional networks during emotional challenge tasks. This indicates not only decreased executive and attentional function, but also suggests dorsal control network deficits in adult PTSD secondary to childhood trauma. Fadel et al. (Citation2021) in a resting-state study indicated that childhood abuse correlates with increased salience network (SN) connectivity. There was a relationship between childhood neglect and decreased connectivity within SN but increased coupling between SN and default mode network (DMN). On the other hand, Marusak, Etkin, and Thomason (Citation2015) suggest that childhood trauma is associated with reduced connectivity between SN and DMN.
Interestingly, similar alterations are indicated as related to rumination. Nolen-Hoeksema and Watkins (Citation2011) emphasised that rumination, defined as the tendency to constantly focus on negative thoughts, problems, or states, and on the possible causes and implications of negative feelings, is a transdiagnostic risk factor for psychopathology. Many studies confirm that internalising disorders (mainly, but not exclusively, depression) are also strongly associated with rumination (cf. Olatunji, Naragon-Gainey, & Wolitzky-Taylor, Citation2013). Studies on neural correlates of rumination suggest the DMN, related to self-referential processing, plays a central role. Araujo, Kaplan, and Damasio (Citation2013) indicated that rumination is specifically associated with activations of the core regions and the dorsal medial prefrontal cortex (dmPFC) subsystem of DMN. Hamilton, Farmer, Fogelman, and Gotlib (Citation2015) suggested a model of rumination in major depression, indicating that the key to producing rumination is increased functional integration between the DMN and subgenual prefrontal cortex. Recently, Zhou et al. (Citation2020) suggested that rumination and self-referential processing are associated with enhanced activity of cortical midline structures (see also Makovac, Fagioli, Rae, Critchley, & Ottaviani, Citation2020; Nejad, Fossati, & Lemogne, Citation2013; Northoff et al., Citation2006). In another recent study on patients with internalising conditions, Feurer et al. (Citation2021) focused on rumination and worry as forms of repetitive negative thinking (RNT); in worry, as in rumination, there is evidence of DMN involvement. Results of whole-brain analyses showed that greater rumination and worry jointly correspond with greater positive resting-state functional connectivity (rsFC) between the amygdala and prefrontal regions (i.e. middle and inferior frontal gyrus).
Although neuroimaging research on mechanisms underlying rumination (or RNT) is developing, there are few studies comparing individuals with and without the tendency to ruminate. Piguet et al. (Citation2014) showed that people not prone to rumination have greater activation of the visual cortex during rest and activation of the insula when performing an easy task, whereas those prone to ruminate activate the left entorhinal region in both conditions. Kowalski, Wypych, Marchewka, and Dragan (Citation2019) compared participants with high and low intensities of symptoms of cognitive-attentional syndrome, the core aspect of which is rumination. They found differences in functional connectivity during an induced negative thinking task and resting state fMRI. The first group of participants showed disrupted patterns of connectivity within and between the DMN, SN, and central executive network. The impairments of self-referential, task-oriented, and emotional neural processing are similar to abnormalities reported in studies on different emotional disorders (Ding et al., Citation2011; Liu et al., Citation2015; Mulders, van Eijndhoven, Schene, Beckmann, & Tendolkar, Citation2015; Peters, Dunlop, & Downar, Citation2016; Zhang et al., Citation2011), supporting the idea that rumination is a key transdiagnostic factor underlying these disorders and their neural correlates.
Many self-report studies show that rumination is a mediator in the link between ACEs and adult psychopathology (e.g. Dragan & Kowalski, Citation2020; Kim, Jin, Jung, Hahn, & Lee, Citation2017; Raes & Hermans, Citation2008). Peters, Burkhouse, Kinney, and Phan (Citation2019) noticed that there is substantial overlap between the functional correlates of ruminative thought patterns and emotion processing in early life adversity, including in the amygdala, anterior and posterior cingulate, medial and dorsolateral prefrontal cortex, (para-) hippocampus, medial and inferior temporal gyri, and inferior parietal lobule. They compared groups of anxious and depressed patients with healthy individuals when performing an emotional face-matching task during fMRI; thus, the study focused on neural correlates of facial emotion processing in patients with heterogeneous internalising disorders, with or without exposure to early life adversity and controlled for rumination (brooding). The findings show that a history of maltreatment augmented engagement of brain regions involved in emotion processing beyond what was accounted for by current symptoms. The authors hypothesised that adverse experiences may heighten cortico-limbic sensitivity to negative emotional cues, in turn promoting increased attention to symptoms and possible causes of one’s own distress (i.e. ruminative brooding).
Given that there is little neuroimaging research examining both ACEs and rumination, this study aimed to explore differences in neural functioning between participants with and without histories of childhood adversity, controlling for tendency to ruminate (i.e. cognitive-attentional syndrome). The main task we focused on was RNT induction, which has been employed in some previous fMRI studies comparing depressed or anxious patients with healthy controls (Berman et al., Citation2014; Cooney, Joormann, Eugène, Dennis, & Gotlib, Citation2010; Paulesu et al., Citation2010). However, we decided to include a non-clinical sample, hypothesising that participants with a history of ACEs would tend to ruminate more often and demonstrate similar patterns of brain activations to those found in studies on neural correlates of both ACEs and rumination. Our study was designed as exploratory; however, taking into account the results of previous studies, we focused on data-driven brain regions associated with tendency to ruminate. In particular, we focused on activity of the DMN, a network associated with rumination and self-reference processing. Similarly to previous research (e.g. Feurer et al., Citation2021), we focused on commonly examined nodes in the DMN, SN, affective, and executive control networks. We hypothesised that a history of ACEs and rumination would correlate with more posterior cingulate cortex (PCC) engagement based on theory and meta-analytic findings (Makovac et al., Citation2020), though we did not make specific hypotheses as to rsFC connectivity patterns, given the inconsistent results.
2. Material and methods
2.1. Participants and procedure
A total of 86 adults (51 women) aged 19–43 years old (Mage = 31.83; SD = 6.45) took part in the study. Participants were recruited by an external company from a community sample (N = 1225). Exclusion criteria included a history of neurological disorders, substance abuse, and any contraindications to the MRI procedure. All participants were right handed. They were also recruited based on scores on scales measuring tendency to ruminate, constituting two extreme groups: low- and high-ruminators; group affiliation was blinded at subsequent stages of the study. Next, all selected participants underwent a diagnostic clinical interview that included questions on adverse childhood experiences. Based on qualitative analysis, participants were divided into two groups, regardless of their tendency for RNT: with and without a history of ACEs. The two groups differed in terms of their high and low tendency to ruminate (χ = 4.24; p < .05). Hence, the tendency to ruminate was used as a covariate in the analyses. None of the participants in the no-ACEs group had a current clinical diagnosis. 37% of participants from the ACEs group had a current clinical diagnosis (such as GAD, MDD, PTDS, social phobia, dysthymia, agoraphobia, etc.). Importantly, almost all participants with a clinical diagnosis had a high tendency to ruminate (89%), which is already accounted for in the analysis. Descriptive statistics for both groups are presented in alongside information on the three main categories of ACEs (abuse, neglect, and other adversities, including things like serious arguments between parents or bullying).
Table 1. Descriptive statistics.
The procedure was approved by the local ethics committee. All participants provided informed consent and were paid the equivalent of 50 EUR in local currency. The study was conducted in accordance with the guidelines of the Declaration of Helsinki.
2.2. Assessment
Adverse childhood experiences were assessed during the interview with the Structured Clinical Interview for DSM-IV-TR, research version (SCID-I/NP; First, Spitzer, Gibbon, & Williams, Citation2002; Polish version: Popiel, Pragłowska, & Zawadzki, Citation2014). This assesses past and current psychiatric diagnoses limited to Axis I psychological disorders and mental illnesses, based on DSM-IV-TR criteria (American Psychiatric Association [APA], Citation2000). The part concerning post-traumatic stress disorder (module F in SCID-I DSM-IV-TR), which includes a question about traumatic events throughout life, allows for asking additional questions, including ones about being a victim of violence. This allowed the interview to be extended with the same questions for childhood (i.e. prior to the age of 16). The answers were coded as a qualitative variable to enable further qualitative analysis and then re-coded as a dichotomous variable (0–no ACEs, 1–ACEs) for the purposes of this neuroimaging study.
Tendency to ruminate was assessed with several measures to create a single binary index which indicated either high- or low-levels of tendency to ruminate. We employed the Cognitive-Attentional Syndrome questionnaire (CAS-1; Kowalski & Dragan, Citation2019; Wells, Citation2009), which measures tendency to RNT along with co-occurring symptoms, like attention to threat, maladaptive coping behaviours, and underlying dysfunctional metacognitive beliefs. Other measures were the Brooding subscale from the Ruminative Responses Scale (Treynor, Gonzalez, & Nolen-Hoeksema, Citation2003) and two subscales from the Metacognitions-30 questionnaire: Need to Control Thoughts and Uncontrollability and Dangerousness of Thoughts (Wells & Cartwright-Hatton, Citation2004). The participants who scored above the 66th percentile in all four questionnaires were considered as having a high tendency to ruminate and those who scored below 33rd percentile as having a low tendency to ruminate. The rationale for and detailed description of this procedure are provided in previous works (Dragan & Kowalski, Citation2020; Kowalski et al., Citation2019; Kowalski & Dragan, Citation2019).
2.3. Experimental task
A modified version of the rumination induction task of Cooney et al. (Citation2010) with ruminative/worrying and abstract sentences was used to measure brain activity during rumination. The sentences involved the participant’s emotions, experiences, and appraisals. Participants thought about the sentences and were asked to clear their minds when a fixation cross was displayed. Sentences were presented for 30s, interleaved with a 10s fixation cross. There were 4 blocks with 5 sentences presented in non-consecutive order. The total duration of the task was about 15 min. The sentences used are provided in the supplementary material of Kowalski et al. (Citation2019).
2.4. MRI data acquisition and preprocessing
First structural images were acquired, followed by a resting-state and task-based functional scans. Whole-brain functional and structural images were acquired using a 3T MRI scanner (Trio TIM, Siemens, Germany) equipped with a 12-channel head coil. High-resolution T1-weighted images were obtained: TR/ TE = 2530/3.32 ms; flip angle = 7°; FoV = 256 mm; voxel dimensions = 1 mm isotropic. Functional images were acquired using a T2-weighted, gradient-echo echo planar imaging (EPI) pulse sequence. A total of 364 whole-brain volumes were recorded for the rumination task with the following parameters: TR/TE = 2500/28 ms; flip angle = 80°; FoV = 216 mm; 3 × 3 × 3 mm vox size. 200 volumes were acquired during resting state with parameters: TR/TE = 2000/28 ms; flip angle = 80°; FoV = 216 mm; 3 × 3 × 3 mm vox size.
Analyses were performed using Statistical Parametric Mapping (SPM12; Wellcome Department of Cognitive Neurology, London, UK), implemented in MATLAB (2019; The MathWorks Inc., Natick, MA, USA). Images were spatially realigned, slice-time corrected, co-registered to the first functional image, segmented, normalised to the standard Montreal Neurological Institute (MNI) template, and spatially smoothed with a 6-mm isotropic Gaussian kernel.
2.5. First-level analysis
Data were modelled using a general linear model (GLM) for each subject. All task conditions and fixation crosses were entered into the model: (i) rumination (Rum), (ii) abstract (Abs), (iii) fixation cross (Fix). Regressors of no interest (fixation crosses and motion parameters) were included in the model. Additionally, the Artificial Detection Tool (ART) toolbox was used to determine motion-affected volumes. Any EPI that deviated from previous EPI by 3SD, 1.6 mm, or 0.04 rad was regressed out in the model; 4.19% (SD = 3.1) of scans from the rumination task and 4.79% (SD = 4) from the resting-state scans were regressed out.
2.6. Second-level analysis
Single-subject contrasts (Rum > Abs) underwent second-level analyses. To test whether groups with and without ACEs differed in brain activation during rumination, a two-sample whole-brain model was used. Age, sex, and tendency for RNT were included as covariates. Family-wise error correction (FWE < .05) was used to control for multiple comparisons. The Harvard-Oxford Atlas was used to label the results.
2.7. Functional connectivity analysis
2.7.1. Seed definition
Seeds for functional connectivity were based on whole-brain task activation. A single sample t test was performed to examine brain activation during rumination using the ([(Rum > Fix)>(Abs > Fix)]) contrast. The MarsBar toolbox was used to build spheres with 6 mm radii around peak activations. Results are given in . The following seeds were defined (coordinates in MNI space): right precuneus [4 −52 28], left SFG (dmPFC) [−2 56 40], right PCC [2 −20 36], left MTG [−62 −14 −10], right thalamus [12 −34 8], left [−50 −60 26] and right [50 −56 34] angular gyri.
Table 2. Whole-brain activation for main effect of task across all participants.
2.7.2. gPPI and resting state
Generalised psychophysiological interaction (gPPI) analysis was performed to examine the task-dependent relationship between neural activity and rumination. The CONN functional connectivity toolbox (v.18b; http://www.nitrc.org/projects/conn) with FWE correction was used to conduct the functional connectivity analyses. The analyses were corrected for multiple comparisons for all seven seeds with p ≤ .001, FWE ≤ .007 (p-value for cluster). Functional data were denoised with the respective T1-weighted images normalised to MNI space, with five regressors for white matter, five regressors for cerebrospinal fluid, and movement parameters from the ART toolbox. The acceptance threshold for the denoised signal (voxel to voxel correlation) was on average r < .1. All regressors of interest (i.e. Rum and Abs) and no interest (i.e. Fix, motion parameters) were entered into a gPPI model, and a group-level seed-based connectivity analysis was performed for the Rum > Abs contrast. Similarly to whole-brain analyses, age, sex, and tendency for RNT were entered as covariates. Functional connectivity scores between seeds and whole clusters were extracted using the CONN toolbox for each participant. Fisher’s Z (i.e. Fisher-transformed correlation coefficients) represents connectivity strength. Additionally, functional connectivity during resting state was analysed using the same seeds and parameters as in the gPPI model. Resting state fMRI was acquired with a fixation cross in an eyes open condition. Participants were asked to fix their gaze on the cross for the entire scan (10 min).
3. Results
3.1. Whole-brain analyses
Whole-brain analysis was performed for the rumination vs abstract thinking contrast. First, analysis was performed across all participants. Rumination-related activation was revealed in medial brain regions (SFG, FP, PCC, PCun, thalamus), bilateral angular gyri, and left MTG. Deactivation was found in bilateral frontal cortex (IFG, MFG), temporal cortex (MTG and STG), SMG, and left insula. Results are given in and . Group comparison between participants with and without ACEs did not yield significant results.
Figure 1. Rumination-related brain activation across all participants. Warm colours indicate stronger activation during rumination; cool colours indicate stronger activation during control condition. Abbreviations: AnG: angular gyrus; FP: frontal pole; Ins: insula; IFG: inferior frontal gyrus; MCC: middle cingulate cortex; MFG: middle frontal gyrus; MTG: middle temporal gyrus; PCun: precuneus; SFG: superior frontal gyrus; SMG: supramarginal gyrus; STG: superior temporal gyrus; Th: thalamus.
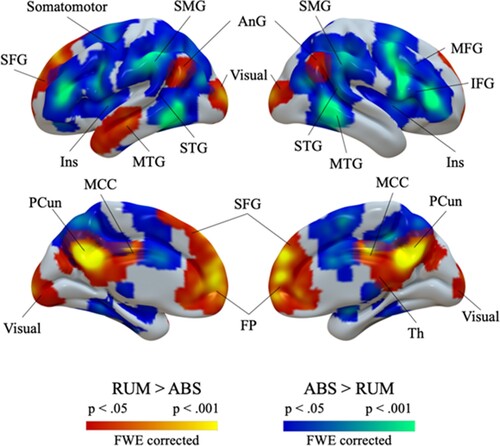
3.2. Functional connectivity
Seed-based connectivity during the rumination task showed that, compared to ACE participants, no ACE participants exhibited stronger functional connectivity between right angular gyrus and left occipital pole, left superior frontal gyrus and right inferior frontal gyrus, and between left and right superior frontal gyri. Results are reported in and .
Figure 2. Seed-based functional connectivity during the rumination task. Green arrows indicate stronger connectivity in the group without ACEs. Fisher’s Z is displayed on the arrows. AnG: angular gyrus; IFG: inferior frontal gyrus; OP: occipital pole; PaC: paracingulate cortex; SFG: superior frontal gyrus.
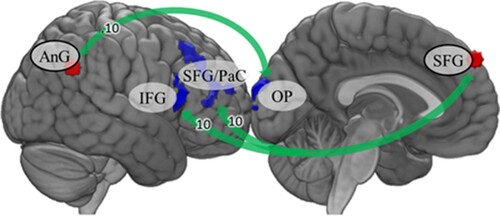
Table 3. Group differences in functional connectivity during the rumination task.
Seed-based connectivity during resting state showed that ACE participants exhibited stronger functional connectivity between right angular gyrus and left cerebellum than no ACE participants. Reverse contrast indicated that the no ACE group was characterised by stronger connectivity between left middle temporal gyrus and inferior frontal gyrus as well as between left superior frontal gyrus and right planum temporale and left inferior frontal gyrus. Results are reported in and .
Figure 3. Seed-based functional connectivity during resting state. Red arrows indicate stronger connectivity in the ACE group, green arrows indicate stronger connectivity in the no ACE group. Fisher’s Z is displayed on the arrows. AnG: angular gyrus; Cb: cerebellum; IFG: inferior frontal gyrus; MTG: middle temporal gyrus; PT: planum temporale; SFG: superior frontal gyrus; TP: temporal pole.
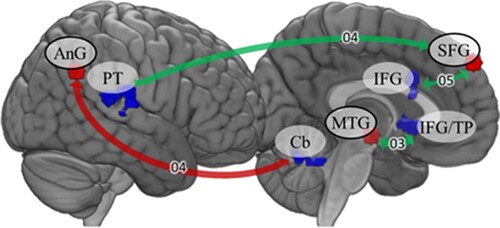
Table 4. Group differences in functional connectivity during resting state.
4. Discussion
4.1. Results of whole-brain analyses
This study focused on the relationship between adverse childhood experiences (ACEs) and neural activity during rumination induction and abstract thinking. Whole-brain analysis revealed expected brain activation associated with the main effect of rumination mainly in the medial prefrontal cortex, precuneus, and angular gyrus. These regions overlap with the DMN, which is highly engaged in mental self-processing, a subtype of more general self-referential processing (Northoff et al., Citation2006; Qin, Wang, & Northoff, Citation2020). The angular gyrus may be responsible for switching between one’s and others’ perspectives and is involved in processing one’s own social and mental features (Northoff et al., Citation2006). The regions found to be active in our study have also been associated with rumination. The medial prefrontal cortex and angular gyri are involved in processing self-relevant affective information (Andrews-Hanna, Reidler, Sepulcre, Poulin, & Buckner, Citation2010). The medial prefrontal cortex is also involved in mentalizing, metacognitive processing, and attention to the mental states of oneself and others (Frith & Frith, Citation2003). Midline regions, including posterior cingulate cortex and precuneus, are engaged during recalling autobiographical information and simulate mental states based on previous experience (Zhou et al., Citation2020). The posterior cingulate cortex integrates self-relational information using spatial and temporal context (Hamilton et al., Citation2015). Interestingly, the engagement of the aforementioned regions in perseverative cognition has been reported in both task-based and resting-state studies (Makovac et al., Citation2020).
4.2. Functional connectivity during induction of rumination
Individuals with ACEs were characterised by increased functional connectivity during the rumination task between the right angular gyrus and left occipital pole. Deming et al. (Citation2018) reported that the occipital cortex is involved in processing information related to oneself, suggesting that this connection supports differentiating focusing on one’s own features from abstract thinking.
ACEs were also related to stronger functional connectivity between the left SFG and regions including the right IFG, SFG, and paracingulate gyrus. The medial PFC is engaged in repetitive cognition, suggesting higher rumination and worry in those with ACEs. Makovac et al. (Citation2020) in their meta-analysis (including task-related studies on rumination) indicated that the mPFC is highly involved in perseverative cognition. Importantly, this region is a part of the DMN, which has been linked to self-processing (Qin et al., Citation2020). The mPFC also facilitates recalling autobiographical information and referring to one’s own previous experiences (Zhou et al., Citation2020). Increased connectivity within mPFC related to ACEs suggests that these individuals may tend to focus on previous negative experiences and engage in negative repetitive self-referential thinking (i.e. rumination; Nolen-Hoeksema, Wisco, & Lyubomirsky, Citation2008).
Our results extend to the paracingulate gyrus and ACC. The ACC has been shown to be engaged in rumination compared to neutral thinking as well as worrying about the future, suggesting higher self-referential processing and inward attention (Steinfurth, Alius, Wendt, & Hamm, Citation2017). Importantly, the ACC and mPFC are involved in self-referential processing of negative but not positive emotional stimuli (Yoshimura et al., Citation2009). These regions are crucial for emotion regulation (Etkin, Büchel, & Gross, Citation2015). Our study shows that individuals with ACEs have increased activation of regions responsible for emotional response regulation.
Childhood adversity is a risk factor for psychopathology (Kessler et al., Citation2010). The ACC is particularly affected by stress (Cohen et al., Citation2006). Goodkind et al. (Citation2015) reported decreased dACC volume across various psychiatric diagnoses, which was related to cognitive and dysexecutive symptoms. Thus, ACC alterations may constitute transdiagnostic correlates of psychiatric symptom occurrence (cf. Kowalski, Wypych, Marchewka, & Dragan, Citation2022). Considering the aforementioned role of ACC in rumination (Makovac et al., Citation2020) and emotion regulation (Etkin et al., Citation2015), individuals with ACEs may be characterised by structural and functional changes in ACC that could result in decreased cognitive control and emotion regulation in the form of stress-related and affective disorders.
4.3. Resting-state functional connectivity
Resting-state analysis revealed decreased functional connectivity between the left MTG and IFG extending to the temporal pole as well as between the left SFG and left IFG, right parietal operculum, and planum temporale in individuals with ACEs. These regions overlap with the cingulo-opercular network (CON; Ji et al., Citation2019). This task positive network is responsible for implementing goal-directed behaviour, maintaining task strategy, and engaging executive functions (Dosenbach et al., Citation2007). Importantly, CON overlaps with the SN (cf. Ji et al., Citation2019; Power et al., Citation2011). When we consider the role of the SN in switching between other large-scale networks (Goulden et al., Citation2014; Menon & Uddin, Citation2010), one interpretation of our results may be that ACEs are related to alterations in switching between intrinsic connectivity networks. This is in line with Fadel et al. (2021) who showed that there is a relationship between the level of childhood neglect and connectivity between task-positive and task-negative networks (SN and DMN, respectively). They also found decreased SN connectivity, however that was significant only for childhood neglect, not childhood abuse. This suggests that ACEs are not homogeneous and may have different impacts on brain functioning.
Changes in CON functioning are present across various psychiatric disorders, which is in line with the role of dACC, as this structure constitutes the core region of the CON (Wu et al., Citation2016). Yu et al. (Citation2019) reported that patients with depression were characterised by decreased within-network connectivity in the CON compared to controls. A history of ACEs was associated with changes in connectivity between CON and sensory networks.
Both task-based and resting state functional connectivity showed altered coupling between SFG and IFG, although in a different direction. ACEs are related to increased connectivity to the right IFG during rumination but decreased connectivity to the left IFG in resting state. A recent meta-analysis revealed functional decrease in the left superior frontal gyrus in healthy participants who experienced ACEs (Kraaijenvanger et al., Citation2020). Structural and functional alterations in the bilateral IFG are related to rumination (Kühn, Vanderhasselt, De Raedt, & Gallinat, Citation2012; Kühn, Vanderhasselt, De Raedt, & Gallinat, Citation2014). The IFG is crucial for affective control, being engaged in the inhibition of repetitive thoughts (Weber-Goericke & Muehlhan, Citation2019). Our results indicate that, in terms of functional connectivity effects, the resting state is not a valid approximation of rumination (cf. Berman et al., Citation2014), at least in comparison to induced rumination. Perhaps this ambiguity reflects forced disinhibition of ruminative thoughts in the task condition vs mind wandering with habitual attempts at thought control in the resting state. Future studies could further explore this difference.
5. Conclusions and limitations
Our study has some limitations. We studied overall childhood adversity without investigating the impact of different types of stressful experiences. ACEs are qualitatively different and may have distinct impacts on brain functioning. Moreover, we did not use any additional measure of ACEs. Although there is no consensus on how best to measure ACEs, and using a clinical interview as a generally reliable assessment method was sufficient for this study, it could lead to an underestimation of adverse experiences (Brewin, Andrews, & Gotlib, Citation1993; Saini, Hoffmann, Pantelis, Everall, & Bousman, Citation2019). Our analyses were not controlled for the presence of a clinical diagnosis. Clinical diagnoses are highly related to both rumination and presence of ACEs. At least some part of the results could be explained by the clinical diagnosis and the severity of symptoms, not the presence of ACEs alone. The relationship between the presence of clinical diagnoses and rumination have been previously described in Dragan and Kowalski (Citation2020). Another limitation is the lack of control of participants’ thoughts during the resting state. A simple questionnaire after the scanning session could reveal whether the groups differed in repetitive thoughts even when no instruction is given.
However, the obtained results prompt reflection on the sequelae of early adversity. Participants who experienced childhood adversities differed from those who didn’t in brain functional connectivity when engaged in negative repetitive self-referential thinking. It is worth mentioning that there are two recent models of the impact of adversity on brain development: dimensional and stress acceleration models. The first assumes that threat and deprivation are common dimensions of various adversities. These two features impact neurodevelopment differently. The latter model assumes that adversity may accelerate neurodevelopment, resulting in earlier maturation of neural networks (McLaughlin, Weissman, & Bitrán, Citation2019). Although our study doesn’t determine which approach better describes stress consequences, we argue the two models are not necessarily mutually exclusive. We can see effects that can be attributed to threat (altered mPFC connectivity supporting emotional processing) and deprivation (altered CON functioning supporting executive functions). This doesn’t exclude the possibility that circuits engaged in emotional processing could have matured early. Future studies (preferably longitudinal) are needed to disentangle the complex relationship between childhood adversities and their consequences.
The obtained findings also confirm previous results indicating that early adverse experiences might promote patterns of cognitive-emotional processing based on self-referential negative thinking. The results are potentially important for the prevention of emotional disorders and support models of psychotherapy that focus on disturbances in self-regulation and maladaptive styles of cognitive-emotional processing. They indicate that early trauma is an important factor for the development of disorders, because it increases the risk of disturbed emotional regulation, including the tendency toward negative self-referential thinking.
Disclosure statement
No potential conflict of interest was reported by the author(s).
Data availability statement
The data that support the findings of this study are available on request from the corresponding author, MD. The data are not publicly available due to their containing information that could compromise the privacy of research participants.
Additional information
Funding
References
- American Psychiatric Association (APA). (2000). Diagnostic and statistical manual of mental disorders – revised (DSM-IV-TR). Washington, DC: American Psychiatric Association.
- Andrews-Hanna, J. R., Reidler, J. S., Sepulcre, J., Poulin, R., & Buckner, R. L. (2010). Functional-anatomic fractionation of the brain's default network. Neuron, 65(4), 550–562. doi: 10.1016/j.neuron.2010.02.005
- Araujo, H. F., Kaplan, J., & Damasio, A. (2013). Cortical midline structures and autobiographical-self processes: An activation-likelihood estimation meta-analysis. Frontiers in Human Neuroscience, 7, 548. doi: 10.3389/fnhum.2013.00548
- Bellis, M. A., Hughes, K., Ford, K., Rodriguez, G. R., Sethi, D., & Passmore, J. (2019). Life course health consequences and associated annual costs of adverse childhood experiences across Europe and North America: A systematic review and meta-analysis. The Lancet Public Health, 4(10), e517–e528. doi: 10.1016/S2468-2667(19)30145-8
- Berman, M. G., Misic, B., Buschkuehl, M., Kross, E., Deldin, P. J., Peltier, S., … Jonides, J. (2014). Does resting-state connectivity reflect depressive rumination? A tale of two analyses. Neuroimage, 103, 267–279. doi: 10.1016/j.neuroimage.2014.09.027
- Bremner, J. D., Narayan, M., Staib, L. H., Southwick, S. M., McGlashan, T., & Charney, D. S. (1999). Neural correlates of memories of childhood sexual abuse in women with and without posttraumatic stress disorder. American Journal of Psychiatry, 156(11), 1787–1795.
- Brewin, C. R., Andrews, B., & Gotlib, I. H. (1993). Psychopathology and early experience: A reappraisal of retrospective reports. Psychological Bulletin, 113(1), 82–98. doi: 10.1037/0033-2909.113.1.82
- Butchart A., Harvey A. P., Mian M., Fürniss T., & Kahane T. (2006) Preventing child maltreatment: A Guide to taking action and generating evidence / World Health Organization and International Society for Prevention of Child Abuse. Geneva: WHO Press.
- Carrion, V. G., Weems, C. F., Ray, R., & Reiss, A. L. (2002). Toward an empirical definition of pediatric PTSD: The phenomenology of PTSD symptoms in youth. Journal of the American Academy of Child & Adolescent Psychiatry, 41(2), 166–173. doi: 10.1097/00004583-200202000-00010
- Cohen, R. A., Grieve, S., Hoth, K. F., Paul, R. H., Sweet, L., Tate, D., … Williams, L. M. (2006). Early life stress and morphometry of the adult anterior cingulate cortex and caudate nuclei. Biological Psychiatry, 59(10), 975–982. doi: 10.1016/j.biopsych.2005.12.016
- Cooney, R. E., Joormann, J., Eugène, F., Dennis, E. L., & Gotlib, I. H. (2010). Neural correlates of rumination in depression. Cognitive, Affective, & Behavioral Neuroscience, 10(4), 470–478. doi: 10.3758/CABN.10.4.470
- De Bellis, M. D., & Zisk, A. (2014). The biological effects of childhood trauma. Child and Adolescent Psychiatric Clinics of North America, 23(2), 185–222. doi: 10.1016/j.chc.2014.01.002
- Deming, P., Philippi, C. L., Wolf, R. C., Dargis, M., Kiehl, K. A., & Koenigs, M. (2018). Psychopathic traits linked to alterations in neural activity during personality judgments of self and others. NeuroImage: Clinical, 18, 575–581. doi: 10.1016/j.nicl.2018.02.029
- Ding, J., Chen, H., Qiu, C., Liao, W., Warwick, J. M., Duan, X., … Gong, Q. (2011). Disrupted functional connectivity in social anxiety disorder: A resting-state fMRI study. Magnetic Resonance Imaging, 29(5), 701–711. doi: 10.1016/j.mri.2011.02.013
- Dosenbach, N. U., Fair, D. A., Miezin, F. M., Cohen, A. L., Wenger, K. K., Dosenbach, R. A., … Petersen, S. E. (2007). Distinct brain networks for adaptive and stable task control in humans. Proceedings of the National Academy of Sciences, 104(26), 11073–11078. doi: 10.1073/pnas.0704320104
- Dragan, M., & Kowalski, J. (2020). Childhood adversities and psychopathology in participants with high and low severity of cognitive-attentional syndrome symptoms. European Journal of Trauma & Dissociation, 4(4), 100112. doi: 10.1016/j.ejtd.2019.05.005
- Etkin, A., Büchel, C., & Gross, J. J. (2015). The neural bases of emotion regulation. Nature Reviews Neuroscience, 16(11), 693–700. doi: 10.1038/nrn4044
- Fadel, E., Boeker, H., Gaertner, M., Richter, A., Kleim, B., Seifritz, E., … Wade-Bohleber, L. M. (2021). Differential alterations in resting state functional connectivity associated with depressive symptoms and early life adversity. Brain Sciences, 11(5), 591. doi: 10.3390/brainsci11050591
- Feurer, C., Jimmy, J., Chang, F., Langenecker, S. A., Phan, K. L., Ajilore, O., & Klumpp, H. (2021). Resting state functional connectivity correlates of rumination and worry in internalizing psychopathologies. Depression and Anxiety, 38(5), 488–497. doi: 10.1002/da.23142
- First, M. B., Spitzer, R. L., Gibbon, M., & Williams, J. B. W. (2002). Structured clinical interview for DSM-IV-TR axis I disorders, research version, patient edition (SCID-I/P). New York: Biometrics Research, New York State Psychiatric Institute.
- Ford, J. D., Stockton, P., Kaltman, S., & Green, B. L. (2006). Disorders of extreme stress (DESNOS) symptoms are associated with type and severity of interpersonal trauma exposure in a sample of healthy young women. Journal of Interpersonal Violence, 21(11), 1399–1416. doi: 10.1177/0886260506292992
- Frith, U., & Frith, C. D. (2003). Development and neurophysiology of mentalizing. Philosophical Transactions of the Royal Society of London. Series B: Biological Sciences, 358(1431), 459–473. doi: 10.1098/rstb.2002.1218
- Goodkind, M., Eickhoff, S. B., Oathes, D. J., Jiang, Y., Chang, A., Jones-Hagata, L. B., … Etkin, A. (2015). Identification of a common neurobiological substrate for mental illness. JAMA Psychiatry, 72(4), 305–315. doi: 10.1001/jamapsychiatry.2014.2206
- Goulden, N., Khusnulina, A., Davis, N. J., Bracewell, R. M., Bokde, A. L., McNulty, J. P., & Mullins, P. G. (2014). The salience network is responsible for switching between the default mode network and the central executive network: Replication from DCM. Neuroimage, 99, 180–190. doi: 10.1016/j.neuroimage.2014.05.052
- Hamilton, J. P., Farmer, M., Fogelman, P., & Gotlib, I. H. (2015). Depressive rumination, the default-mode network, and the dark matter of clinical neuroscience. Biological Psychiatry, 78(4), 224–230. doi: 10.1016/j.biopsych.2015.02.020
- Heany, S. J., Groenewold, N. A., Uhlmann, A., Dalvie, S., Stein, D. J., & Brooks, S. J. (2018). The neural correlates of childhood trauma questionnaire scores in adults: A meta-analysis and review of functional magnetic resonance imaging studies. Developmental Psychopathology, 30(4), 1475–1485. doi: 10.1017/S0954579417001717
- Hein, T. C., & Monk, C. S. (2017). Research review: Neural response to threat in children, adolescents, and adults after child maltreatment – a quantitative meta-analysis. Journal of Child Psychology and Psychiatry, 58(3), 222–230. doi: 10.1111/jcpp.12651
- Ji, J. L., Spronk, M., Kulkarni, K., Repovš, G., Anticevic, A., & Cole, M. W. (2019). Mapping the human brain's cortical-subcortical functional network organization. Neuroimage, 185, 35–57. doi: 10.1016/j.neuroimage.2018.10.006
- Kalmakis, K. A., & Chandler, G. E. (2015). Health consequences of adverse childhood experiences: A systematic review. Journal of the American Association of Nurse Practitioners, 27(8), 457–465. doi: 10.1002/2327-6924.12215
- Kessler, R. C., McLaughlin, K. A., Green, J. G., Gruber, M. J., Sampson, N. A., Zaslavsky, A. M., … Williams, D. R. (2010). Childhood adversities and adult psychopathology in the WHO world mental health surveys. The British Journal of Psychiatry, 197(5), 378–385. doi: 10.1192/bjp.bp.110.080499
- Kim, J. S., Jin, M. J., Jung, W., Hahn, S. W., & Lee, S. H. (2017). Rumination as a mediator between childhood trauma and adulthood depression/anxiety in non-clinical participants. Frontiers in Psychology, 8, 1597. doi: 10.3389/fpsyg.2017.01597
- Kowalski, J., & Dragan, M. (2019). Cognitive-attentional syndrome – the psychometric properties of the CAS-1 and multi-measure CAS-based clinical diagnosis. Comprehensive Psychiatry, 91, 13–21. doi: 10.1016/j.comppsych.2019.02.007
- Kowalski, J., Wypych, M., Marchewka, A., & Dragan, M. (2019). Neural correlates of cognitive-attentional syndrome: An fMRI study on repetitive negative thinking induction and resting state functional connectivity. Frontiers in Psychology, 10, 648. doi: 10.3389/fpsyg.2019.00648
- Kowalski, J., Wypych, M., Marchewka, A., & Dragan, M. (2022). Brain structural correlates of cognitive-attentional syndrome – a voxel-based morphometry study. Brain Imaging and Behavior, https://doi.org/10.1007/s11682-022-00649-2
- Kraaijenvanger, E. J., Pollok, T. M., Monninger, M., Kaiser, A., Brandeis, D., Banaschewski, T., & Holz, N. E. (2020). Impact of early life adversities on human brain functioning: A coordinate-based meta-analysis. Neuroscience & Biobehavioral Reviews, 113, 62–76. doi: 10.1016/j.neubiorev.2020.03.008
- Kühn, S., Vanderhasselt, M. A., De Raedt, R., & Gallinat, J. (2012). Why ruminators won't stop: The structural and resting state correlates of rumination and its relation to depression. Journal of Affective Disorders, 141(2-3), 352–360. doi: 10.1016/j.jad.2012.03.024
- Kühn, S., Vanderhasselt, M. A., De Raedt, R., & Gallinat, J. (2014). The neural basis of unwanted thoughts during resting state. Social Cognitive and Affective Neuroscience, 9(9), 1320–1324. doi: 10.1093/scan/nst117
- Lim, L., Radua, Q., & Rubia, K. (2014). Gray matter abnormalities in childhood maltreatment: A voxel-wise meta–analysis. American Journal of Psychiatry, 171(8), 854–863. doi: 10.1176/appi.ajp.2014.13101427
- Liu, F., Guo, W., Fouche, J. P., Wang, Y., Wang, W., Ding, J., … Chen, H. (2015). Multivariate classification of social anxiety disorder using whole brain functional connectivity. Brain Structure and Function, 220(1), 101–115. doi: 10.1007/s00429-013-0641-4
- Makovac, E., Fagioli, S., Rae, C. L., Critchley, H. D., & Ottaviani, C. (2020). Can't get it off my brain: Meta-analysis of neuroimaging studies on perseverative cognition. Psychiatry Research: Neuroimaging, 295, 111020. doi: 10.1016/j.pscychresns.2019.111020
- Marusak, H. A., Etkin, A., & Thomason, M. E. (2015). Disrupted insula-based neural circuit organization and conflict interference in trauma-exposed youth. NeuroImage: Clinical, 8, 516–525. doi: 10.1016/j.nicl.2015.04.007
- McLaughlin, K. A., Weissman, D., & Bitrán, D. (2019). Childhood adversity and neural development: A systematic review. Annual Review of Developmental Psychology, 1, 277–312. doi: 10.1146/annurev-devpsych-121318-084950
- Menon, V., & Uddin, L. Q. (2010). Saliency, switching, attention and control: A network model of insula function. Brain Structure and Function, 214(5-6), 655–667. doi: 10.1007/s00429-010-0262-0
- Mulders, P. C., van Eijndhoven, P. F., Schene, A. H., Beckmann, C. F., & Tendolkar, I. (2015). Resting-state functional connectivity in major depressive disorder: A review. Neuroscience & Biobehavioral Reviews, 56, 330–344. doi: 10.1016/j.neubiorev.2015.07.014
- Nejad, A. B., Fossati, P., & Lemogne, C. (2013). Self-referential processing, rumination, and cortical midline structures in major depression. Frontiers in Human Neuroscience, 7, 666. doi: 10.3389/fnhum.2013.00666
- Nelson, C. A., Scott, R. D., Bhutta, Z. A., Harris, N. B., Danese, A., & Samara, M. (2020). Adversity in childhood is linked to mental and physical health throughout life. BMJ, 371, m3048. doi: 10.1136/bmj.m3048
- Nolen-Hoeksema, S., & Watkins, E. R. (2011). A heuristic for developing transdiagnostic models of psychopathology: Explaining multifinality and divergent trajectories. Perspectives on Psychological Science, 6(6), 589–609. doi: 10.1177/1745691611419672
- Nolen-Hoeksema, S., Wisco, B. E., & Lyubomirsky, S. (2008). Rethinking rumination. Perspectives on Psychological Science, 3(5), 400–424. doi: 10.1111/j.1745-6924.2008.00088.x
- Northoff, G., Heinzel, A., De Greck, M., Bermpohl, F., Dobrowolny, H., & Panksepp, J. (2006). Self-referential processing in our brain—a meta-analysis of imaging studies on the self. Neuroimage, 31(1), 440–457. doi: 10.1016/j.neuroimage.2005.12.002
- Nurius, P. S., Green, S., Logan-Greene, P., & Borja, S. (2015). Life course pathways of adverse childhood experiences toward adult psychological well-being: A stress process analysis. Child Abuse & Neglect, 45, 143–153. doi: 10.1016/j.chiabu.2015.03.008
- Olatunji, B. O., Naragon-Gainey, K., & Wolitzky-Taylor, K. B. (2013). Specificity of rumination in anxiety and depression: A multimodal meta-analysis. Clinical Psychology: Science and Practice, 20(3), 225.
- Paulesu, E., Sambugaro, E., Torti, T., Danelli, L., Ferri, F., Scialfa, G., … Sassaroli, S. (2010). Neural correlates of worry in generalized anxiety disorder and in normal controls: A functional MRI study. Psychological Medicine, 40(1), 117–124. doi: 10.1017/S0033291709005649
- Peters, A. T., Burkhouse, K. L., Kinney, K. L., & Phan, K. L. (2019). The roles of early-life adversity and rumination in neural response to emotional faces amongst anxious and depressed adults. Psychological Medicine, 49(13), 2267–2278. doi: 10.1017/S0033291718003203
- Peters, S. K., Dunlop, K., & Downar, J. (2016). Cortico-striatal-thalamic loop circuits of the salience network: A central pathway in psychiatric disease and treatment. Frontiers in Systems Neuroscience, 10, 104. doi: 10.3389/fnsys.2016.00104
- Piguet, C., Desseilles, M., Sterpenich, V., Cojan, Y., Bertschy, G., & Vuilleumier, P. (2014). Neural substrates of rumination tendency in non-depressed individuals. Biological Psychology, 103, 195–202. doi: 10.1016/j.biopsycho.2014.09.005
- Popiel, A., Pragłowska, E., & Zawadzki, B. (2014). Ustrukturalizowany wywiad kliniczny do badania zaburzeń z osi I DSM-IV-TR. [SCID-I]. Pracownia Testów, Warsaw.
- Power, J. D., Cohen, A. L., Nelson, S. M., Wig, G. S., Barnes, K. A., Church, J. A., … Petersen, S. E. (2011). Functional network organization of the human brain. Neuron, 72(4), 665–678. doi: 10.1016/j.neuron.2011.09.006
- Qin, P., Wang, M., & Northoff, G. (2020). Linking bodily, environmental and mental states in the self—A three-level model based on a meta-analysis. Neuroscience & Biobehavioral Reviews, 115, 77–95. doi: 10.1016/j.neubiorev.2020.05.004
- Raes, F., & Hermans, D. (2008). On the mediating role of subtypes of rumination in the relationship between childhood emotional abuse and depressed mood: Brooding versus reflection. Depression and Anxiety, 25(12), 1067–1070. doi: 10.1002/da.20447
- Saini, S. M., Hoffmann, C. R., Pantelis, C., Everall, I. P., & Bousman, C. A. (2019). Systematic review and critical appraisal of child abuse measurement instruments. Psychiatry Research, 272, 106–113. doi: 10.1016/j.psychres.2018.12.068
- Steinfurth, E. C., Alius, M. G., Wendt, J., & Hamm, A. O. (2017). Physiological and neural correlates of worry and rumination: Support for the contrast avoidance model of worry. Psychophysiology, 54(2), 161–171. doi: 10.1111/psyp.12767
- Teicher, M. H., Samson, J. A., Anderson, C. M., & Ohashi, K. (2016). The effects of childhood maltreatment on brain structure, function and connectivity. Nature Reviews Neuroscience, 17(10), 652–666. doi: 10.1038/nrn.2016.111
- Treynor, W., Gonzalez, R., & Nolen-Hoeksema, S. (2003). Rumination reconsidered: A psychometric analysis. Cognitive Therapy and Research, 27(3), 247–259. doi: 10.1023/A:1023910315561
- Weber-Goericke, F., & Muehlhan, M. (2019). A quantitative meta-analysis of fMRI studies investigating emotional processing in excessive worriers: Application of activation likelihood estimation analysis. Journal of Affective Disorders, 243, 348–359. doi: 10.1016/j.jad.2018.09.049
- Wells, A. (2009). Metacognitive therapy for anxiety and depression. London: Guilford Press.
- Wells, A., & Cartwright-Hatton, S. (2004). A short form of the metacognitions questionnaire: Properties of the MCQ-30. Behaviour Research and Therapy, 42(4), 385–396. doi: 10.1016/S0005-7967(03)00147-5
- Widom, C. S., DuMont, K., & Czaja, S. J. (2007). A prospective investigation of major depressive disorder and comorbidity in abused and neglected children grown up. Archives of General Psychiatry, 64(1), 49–56. doi: 10.1001/archpsyc.64.1.49
- Wu, X., Lin, P., Yang, J., Song, H., Yang, R., & Yang, J. (2016). Dysfunction of the cingulo-opercular network in first-episode medication-naive patients with major depressive disorder. Journal of Affective Disorders, 200, 275–283. doi: 10.1016/j.jad.2016.04.046
- Yoshimura, S., Ueda, K., Suzuki, S. I., Onoda, K., Okamoto, Y., & Yamawaki, S. (2009). Self-referential processing of negative stimuli within the ventral anterior cingulate gyrus and right amygdala. Brain and Cognition, 69(1), 218–225. doi: 10.1016/j.bandc.2008.07.010
- Yu, M., Linn, K. A., Shinohara, R. T., Oathes, D. J., Cook, P. A., Duprat, R., … Sheline, Y. I. (2019). Childhood trauma history is linked to abnormal brain connectivity in major depression. Proceedings of the National Academy of Sciences, 116(17), 8582–8590. doi: 10.1073/pnas.1900801116
- Zhang, J., Wang, J., Wu, Q., Kuang, W., Huang, X., He, Y., & Gong, Q. (2011). Disrupted brain connectivity networks in drug-naive, first-episode major depressive disorder. Biological Psychiatry, 70(4), 334–342. doi: 10.1016/j.biopsych.2011.05.018
- Zhou, H. X., Chen, X., Shen, Y. Q., Li, L., Chen, N. X., Zhu, Z. C., … Yan, C. G. (2020). Rumination and the default mode network: Meta-analysis of brain imaging studies and implications for depression. Neuroimage, 206, 116287. doi: 10.1016/j.neuroimage.2019.116287