ABSTRACT
Background: E-cigarette use has been shown to have short-term acute effects among active users but less is known of the acute passive effects, particularly among individuals with existing respiratory diseases.
Objective: To investigate local and systemic effects of short-term passive vape exposure among patients with mild or moderate chronic obstructive pulmonary disease (COPD).
Methods: In a double-blinded crossover study 16 non-smoking COPD-patients (mean age 68) were randomly exposed for 4 h to passive vape (median PM2.5: 18 µg/m3 (range: 8–333)) and clean air (PM2.5 < 6 µg/m3) separated by 14 days. Particles were measured using an ultrafine particle counter (P-TRAK) and a scanning mobility particle sizer (SMPS). Health effects including Surfactant Protein-A (SP-A) and albumin in exhaled air, spirometry, FeNO, and plasma proteins were evaluated before, right after, and 24 hours after exposure. Participants reported symptoms throughout exposure sessions. Data were analyzed using mixed models.
Results: SP-A in exhaled air was negatively affected by exposure to vape and several plasma proteins increased significantly. Throat irritation was more pronounced during passive vape exposure, while FVC and FEV1 decreased, however, not significantly.
Conclusions: SP-A in exhaled air and some plasma proteins were affected by passive vape in patients with COPD indicating inflammation, showing that passive vape exposure is potentially harmful.
Introduction
As e-cigarettes are by some parties considered a healthier alternative to conventional cigarettes, they are promoted as an aid to decrease or quit tobacco smoking [Citation1]. However, their use as an effective smoking cessation device is questionable [Citation2,Citation3] and so is the idea that they are safe to use [Citation4,Citation5]. There is concern that e-cigarettes may have adverse long-term health effects and serve as a gateway product to cigarettes [Citation6,Citation7]. Several studies focusing on short-term health effects among e-cigarette users indicate that e-cigarettes are not harmless. Evidence show that e-cigarettes are affecting the cardiovascular as well as the respiratory system including respiratory symptoms such as cough, sore throat and dry mouth, reduced lung function, increase in impedance, increased oxidative stress biomarkers, decrease in exhaled NO (FeNO), signs of possible vascular damage, and increased blood pressure and heart rate in users [Citation4,Citation8–11].
E-cigarettes produce an aerosol often referred to as ‘vapor’ that is inhaled by the user. Unlike conventional cigarettes, e-cigarettes produce no secondary or side-stream emissions; therefore, passive exposure (i.e. exposure to air exhaled by vapers) consists only of what the user exhales. As the number of e-cigarette users is increasing, so is exposure to passive vape. Also, the use of e-cigarettes is often permitted in otherwise smoke-free areas causing passive vape exposure for individuals present [Citation12]. Passive vape exposure remain a concern as previous studies have demonstrated that vape from e-cigarettes can contain toxic chemicals that are harmful to health [Citation13]. A WHO-commissioned review found that while there are a limited number of studies in this area, it can be concluded that e-cigarette aerosol is a new air contamination source for particulate matter, which includes fine and ultrafine particles, as well as 1,2-propanediol, VOCs, heavy metals, and nicotine [Citation14]. It is reasonable to assume that the increased concentrations of toxicants from passive vape over background levels poses an increased risk for the health of all bystanders [Citation14] – and, bystanders with respiratory disease might be even more sensitive.
More research is needed to better understand potential health effects to passive bystanders, especially among vulnerable populations, including individuals with existing respiratory disease, known to be frail to environmental exposure [Citation15]. Thus, the objective of the present study was to investigate acute local and systemic effects of short-term passive exposure to vape from e-cigarettes among individuals with mild or moderate Chronic Obstructive Lung Disease (COPD). The hypothesis was that passive vape exposure would lead to inflammation in lungs and blood.
Materials and methods
Details on recruitment, exposures, clinical testing, and statistical analyses are provided in supplemental online material.
Design
In a randomized double-blind, crossover trial participants were exposed to two exposure sessions (). Each session lasted 4 h; either air mixed with aerosol from e-cigarette users (median PM2.5: 18 µg/m3 (range: 8–333)) or clean filtered air (PM2.5 < 6 µg/m3) at least two weeks apart in order to eliminate carry-over effects. The filtered clean air and e-cigarette vape sessions were identical except for the air quality.
Figure 1. CONSORT (consolidated standards of reporting trials) flow diagram: Number of participants from enrollment to analysis
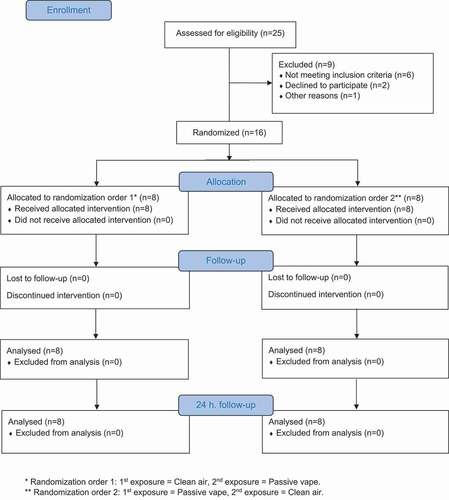
Participants
Non-smoking patients with mild or moderate COPD were recruited. Patients had a known diagnosis of COPD determined by symptoms and spirometry using FEV1/FVC below lower limit of normal and MRC score≥2 and CAT (COPD Assessment Test) score≥10. If patients were on long-acting bronchodilators and inhaled corticosteroids, it was converted to short-acting bronchodilators one week prior to participation. Before any exposure session, participants were required to be without signs of infections or airway symptoms for at least one week, and not to have taken any medicine during at least 48 hours. Additionally, participants were asked by a doctor whether they had been abstaining from smoking/vaping, which had to be affirmed. We aimed to include 30 participants according to our power calculation.
Exposure
Exposure sessions took place in an exposure chamber, while exposure generation took place in an adjacent chamber. Three participants were exposed at a time. The aerosol was generated by two or three vapers who, in turn, vaped on e-cigarettes. The most popular brand of e-cigarettes in Denmark was examined; Joyetech eGo AIO (with a 2 ml tank, standard battery capacity of 1500 mAh and a standard Cubis BF coil (0.6 ohm)). We chose the two most commonly sold e-juices in Denmark at the time of the study; The included e-juices were pre-made ‘Tobacco’ and ‘Strawberry’ flavour (70% propylene glycol/30% vegetable glycerine) from ‘InSano’ containing 6 mg of nicotine acquired in containers of 10 ml. All participants were exposed to both flavours in combination. A controlled flow of aerosol was transferred from the vaping chamber to the exposure chamber by a pipe connection using a negative pressure of 10 Pascal. During clean air sessions, vapers were present in the vaping chamber; however, they did not use e-cigarettes. Instead, they were chewing gum (Nicotinell® Fruit) with 4 mg nicotine and fruit taste in order to mask the exposure. The specific vape exposure levels used in this study were chosen to obtain levels comparable with real-life scenarios.
Data collection
Particle size range (7–500 nm) as well as gravimetric measurements were determined using particle counters and filters. Prior to, right after, and 24 hours following exposure each participant underwent a series of health examinations including sampling of Particles in Exhaled Air (PExA), spirometry, Fraction of Exhaled Nitrogen Oxide (FeNO), and a blood sample. During each exposure session, a questionnaire assessing symptoms was completed prior to exposure, every 30 min during exposure, and at the end of the exposure session.
Statistics
Mixed models based on the univariate repeated measurements ANOVA were performed, taking into account the different design variables corresponding to the crossover design [Citation16]. Model 1 included a time-exposure interaction. If the interaction was non-significant, model 2 without interaction was performed. Surfactant Protein-A (SP-A) and albumin in exhaled air were the primary outcomes of interest. Secondary outcomes were spirometry, FeNO, plasma proteins, and self-reported symptoms.
Ethics
The Ethical Committee in Central Denmark Region approved the study protocol (ref.no. 1–10-72-273-16) and the project was conducted in accordance with The Declaration of Helsinki. Participants had received written and oral information about the project and provided written consent prior to participation.
Results
Sixteen patients (6 female; 10 male) with moderate severe COPD and a mean age of 67.6 years participated (See ).
Table 1. Participant characteristics for the study population
Exposures
As shown in , the peak number of particles on days with vape exposure was approximately in the size range of 30–40 nm. Another peak, although minor, was seen for larger particles (200–500 nm), which is coinciding with information from the vapers on the coil overheating. Hence, these particles could derive from combustion. The particle mass peaked when the particle size was around 300–500 nm. On exposure days with clean air particle concentrations were very low (see for average concentrations). Figure E1 in the online data supplement shows the individual measurements during each exposure day.
Figure 2(a). Average SMPS size distribution of particles and corresponding mass distributions during a) days with passive vape, b) days with clean air. Mean particle size distribution (orange curve (dN/dlogDp (particle number (#)/cm3))) and particle mass (green curve (dM/dlogDp (µg/m3))) ± SD. In Figure 2(a) colored symbols are an average of six measurement series, grey symbols from at least two measurement series. In Figure 2(b) colored symbols are an average of four measurement series, grey symbols from at least one measurement series
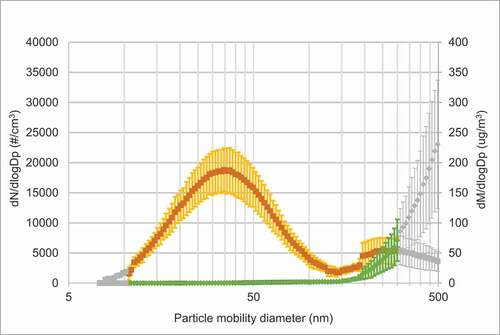
Formaldehyde, acetone, and acetaldehyde
Average concentrations of formaldehyde, acetaldehyde and acetone in the exposure chamber were similar between experiments with or without vaping (see Figure E2 in the online data supplement). Measurements in the adjacent room with vapers and at the outlet during one exposure session, showed higher concentrations of acetaldehyde (mean ± SD) (3.2 ± 0.2 μg m−3) and acetone (15.2 ± 1.2 μg m−3) in the air from the adjacent room than in the exposure chamber (2.0 ± 0.2 μg m−3 and 7.2 ± 0.2 μg m−3, respectively), while formaldehyde was observed at similar levels (3.3 ± 0.1 μg m−3 in adjacent room and 4.1 ± 0.2 μg m−3 in exposure chamber). In addition, an analysis method for nicotine in particle filter samples was developed, but, unfortunately, the method was not sensitive enough to detect nicotine in all but one of the analyzed samples.
Health outcomes
PExA
All participants performed PExA-samples, however, data are only analyzed for nine out of 16 participants as samples from seven participants were ‘below limit of detection’ (<40 ng). Among those nine participants having enough sample material, three participants contributed with only one measure and one person only contributed to measures on one of the exposure days – therefore only five participants are included in the mixed effect analyses. There was a significant increase in percent of SP-A in PExA-samples 24 hours after exposure start compared to before exposure start (p = 0.021), also the time-exposure interaction was significant for percent SP-A (p = 0.029) showing a decrease after passive vape exposure taking into account the diurnal effect (). Percent of SP-A in the PExA-sample increased after exposure to passive vape (0.579 (95% CI −0.483; 1.641)), however insignificant. Exposure to passive vape did not affect percent of Albumin in PExA-samples or the Albumin/SP-A ratio.
Table 2. Change in particles in exhaled air comparing passive vape exposure to clean air exposure (reference)
Spirometry
Minor, however, borderline-significant reductions in FEV1 and FVC were observed for passive vape compared to clean air (−0.046 l and −0.071 l, respectively) (see ).
Table 3. Change in lung outcomes comparing passive vape exposure to clean air exposure (reference)
FeNO
As seen from , exposure to passive vape did not significantly affect FeNO when compared to exposure to clean air (−1.60 (95% CI −5.14; 1.94)).
Blood plasma
The time-exposure interaction was significant for several of the analyzed proteins (see ), indicating that a differential change in the plasma occurred during the two exposure sessions. Albumin (p = 0.006) and Acetoacetate (p = 0.014) were highly significant, while other markers – in particular cholesterol and lipoproteins – were less pronounced, however, still significant. Citrate, free cholesterol in very large HDL plus triglycerides in medium HDL, in plasma were positively associated by exposure to vape alone, though the majority of the measured proteins did not show any variations related to exposure (model 2). Time also had a significant influence on several of the proteins indicating diurnal or post-prandial variation (results not shown).
Table 4. Significant change in plasma proteins comparing passive vape exposure to clean air exposure (ref.)
Symptoms from eyes, nose, and throat
Data on symptoms were registered by all participants every 30 minutes during the 4-h exposure sessions including 0 min of exposure, resulting in nine time points. As seen from , differences in participants’ symptoms were mild when comparing days with passive vape to days with clean air ranging from 6–20% of maximum on the scale. However, throat irritation was significantly higher on days with passive vape compared to days with clean air at time 180 and 210 min, showing that throat irritation worsened over time on days with passive vape. There were no differences on eye and nose irritation when comparing passive vape to clean air, except for eye irritation that became significantly higher as time went by when exposed to passive vape.
Figure 3. Mean symptoms (95% CI) as % of max1 (y-axis) experienced over time (0 min to 240 min) during the two exposure scenarios: Eye irritation, throat irritation, and nose irritation
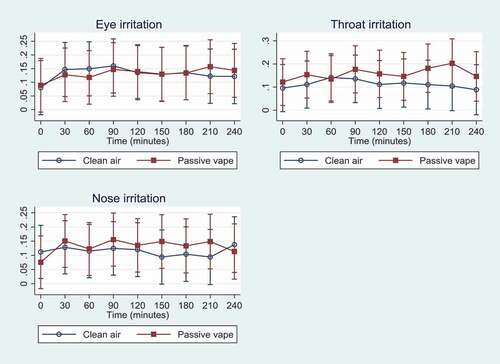
Discussion
In the present study, we have shown that exposure to passive vape from e-cigarettes resulted in symptoms and systemic health effects among COPD-patients. We found that SP-A in exhaled air was affected by time and exposure to vape concentrations of median 18 µg/m3 (range: 8–333) indicating a negative effect of passive vape on SP-A the morning after exposure. Furthermore, several plasma proteins increased significantly indicating inflammation caused by vape exposure. We found a borderline significant decline in lung function, but no effect on FeNO among the 16 COPD-patients exposed to passive vape.
Average concentrations of formaldehyde, acetaldehyde and acetone were similar between days with and without vaping, which is in contrast to previous studies where emission of carbonyl compounds from thermal degradation of e-liquid constituents has been observed [Citation17–19]. In our study, the air exchange was high (2.1 ± 0.2), and it seems that levels of carbonyl compounds were also affected by other factors than vaping, such as emission of carbonyl compounds from participants. This might explain why no particular difference in carbonyls between exposure days was seen [Citation20]. We measured particles along their way from the small chamber through the pipe connection and into the large chamber using a P-TRAK. We found that we only lost very few particles during the transportation. However, the exposure was purposely mixed with clean inlet air and diluted when entering the large chamber.
Our results regarding SP-A in the lungs after passive vape exposure are novel. The significant time-exposure interaction showed a differential change in SP-A in exhaled particles during the two different exposure scenarios; a decrease was observed after passive vape exposure compared to clean air. One reason for the low 24-h level might be depletion of SP-A due to inflammation caused be the passive vape exposure. Similarly, a study by McKenzie et al. found decreasing SP-A levels after acute exposure to nanoparticles [Citation21]. SP-A is a pivotal part of the respiratory immune system; it has the ability to opsonize or bind pathogens and other invading micro-organisms to enhance phagocytic removal from the airways [Citation22]. It seems likely that SP-A decrease after acute exposure to particles, as they perish after fighting invading micro-organisms. The clinical implications of decreasing SP-A levels are unclear, however, it has been shown that low levels increase the risk of infections, e.g. virus infections [Citation22]. Therefore, in COPD-patients depleted levels of SP-A might increase the risk of exacerbations. We also observed a minor, however insignificant, increase after vape exposure alone (not taking time into account). This might be because SP-A initially increases to protect the lungs from, e.g. inhaled particles [Citation23], followed by a depletion after having fought the invading micro-organisms.
While SP-A and albumin in exhaled air were the only primary outcomes in our study, several secondary outcomes were analyzed, however, they have to be viewed upon as hypothesis generating. Our findings of borderline significant negative changes in FEV1 and FVC following passive vape exposure is differing from other experimental studies. In a chamber study, Flouris and colleagues exposed 15 never-smokers to machine-generated e-cigarette aerosol for a single hour after which they measured lung function. Never-smokers also underwent a control session with no emissions and a passive tobacco cigarette session. The assessment of lung function demonstrated that a one-hour passive e-cigarette vaping session did not significantly interfere with normal lung function, and the same result applied to active e-cigarette users [Citation24]. A lack of change in lung function was also found in some of our previous exposure studies where we observed airway inflammation [Citation25], indicating that lung function measurements may be less sensitive than other measurements. However, COPD-patients might be more sensitive with regard to changes in lung function than healthy volunteers. An important consideration in the present study was participant’s discontinuation of corticosteroids seven days before exposure start possibly affecting the level of hyper-responsiveness in the lung. Increased responsiveness would render the participants more frail to passive vape, and we did see an indication of an effect. A possible explanation for the decline in lung function not reaching significance is low power, as we expected SD of 1.5 in our power calculation. Importantly, absence of significant short-term changes in spirometry does not mean that e-cigarettes are harmless [Citation26].
In our study, we observed a minor, but insignificant, decrease in FeNO concentrations following exposure to passive vape. Tzortzi et al. examined the effects of passive exposure to e-cigarette emissions on respiratory mechanics in a crossover experimental study with 40 young healthy non-smokers [Citation27]. All participants underwent a 30-min control session with no emissions and two experimental sessions (0.5 and 1.5 ohm exposure). In their study, FeNO decreased significantly post exposure in the 0.5-ohm session indicating immediate alterations [Citation27]. A decrease in nitric oxide (NO) is opposite to the findings in some air pollution studies, where NO production has been reported to increase with high levels of air pollution indicating airway inflammation [Citation28,Citation29], however, in accordance with studies on exposure to active and passive smoking of conventional cigarettes and active use of e-cigarettes [Citation30–33]. A decrease in NO after exposure to passive vape could be explained by a negative feedback mechanism as the NO in vape downregulates NO synthases in the lungs through the nitric oxide it contains – similar to the mechanism observed by conventional cigarette smoking [Citation27,Citation34].
In the present study, we found increased levels of several plasma proteins, including albumin, acetoacetate, and citrate. Citrate is known for its activation potential for innate immune reactions and it plays a critical role in many normal physiological activities. Dysregulation can lead to several consequences such as impaired blood coagulability [Citation35]. We found increased levels of free and esterified cholesterols, which are biomarkers associated with cardiovascular inflammation related to PM exposure [Citation36].
We found no evident change in self-reported symptoms of eye and nose irritation, however, throat irritation was more pronounced on days with passive vape exposure, although to a limited extent. To our knowledge, no previous exposure study on passive vape has examined self-reported symptoms, however, our results complement studies with other designs and/or other subgroups of the population. Dicpinigaitis and colleagues found in their exposure study among 30 healthy volunteers a significant inhibition of cough reflex sensitivity after a single session of active e-cigarette use [Citation37]. In a cross-sectional study among youths with asthma, Bayly and colleagues found that passive vape exposure was associated with higher odds of reporting an asthma attack, i.e. shortness of breath, chest tightness, cough, and/or wheezing in the past 12 months [Citation38].
Strengths and limitations
The crossover design, the randomization, and double-blinding were the major strengths of the present study. In addition, we used an up-to-date exposure chamber in which all conditions other than the exposures were kept constant. Another strength was using human vapers, which gives a more realistic exposure than using a vaping machine, as the aerosol released to the surroundings are as in real-life with regard to composition of chemicals and particles exhaled from the user [Citation39]. It is questionable whether smoking machines are able to replicate human vaping behavior, as the inhaled aerosol undergoes changes in the human lung that is assumed to be attributed to deposition and evaporation, and it is therefore uncertain whether results from studies relying on smoking machines are trustworthy [Citation4,Citation39]. However, using real vapers resulted in variability in the exposure, as the vapers did not use a standardized vape procedure, since they were only instructed to vape in shifts. From previous studies, we know that there is a large degree of variability in user exposure to these aerosol constituents across patterns of e-cigarette use among other things [Citation40]. We feel that the blinding by using fruit gum as placebo worked well, however, we did not perform a systematic analyses on the effect of blinding.
Our study had limitations such as low power due to few participants, and furthermore, not all participants were able to complete all health examinations resulting in missing data. We found it difficult to recruit mildly to moderately affected COPD-patients, who were able to take the time to participate and at the same time cope with the discontinuation of corticosteroids even for a period of seven days. The activities of participants in the hours and days before the exposure sessions could not be standardized or completely controlled for and were likely to cause random effects. Low and varying levels of aerosol was another limitation in our study. The difference between median particle counts for exposure days with clean air and days with passive vape was not pronounced and neither were levels of formaldehyde, acetaldehyde or acetone. On two out of eight exposure days with passive vape, the level of particle counts were quite high as some very experienced vapers were present in the small chamber inhaling and exhaling forcefully. Previous studies show that composition of the aerosol that is generated depends on the ingredients of the e-liquid, the e-liquid levels left, the characteristics of the e-cigarette including the electrical characteristics of the heating element, and that production of harmful substances is influenced by both battery voltage output and temperature reached, which complicates research in this field [Citation4,Citation40].
Although nicotine-free liquids are available, the use of liquids containing nicotine is more common [Citation41], why we chose e-liquids with 6 mg nicotine. This was the lowest amount possible, and one might expect worse health effects with higher nicotine levels [Citation8]. The low amount of nicotine was chosen in order for the vapers not to become unwell during vaping for several hours.
In summary, we believe that a true, but very mild effect of passive vape occurred in this study, although chance is an alternative explanation, and therefore interpretation should be made with caution. The findings of this study do not necessarily pertain to the background population; however, they might be generalized to other people with chronic respiratory disease.
Conclusion
In conclusion, the results of this study indicate that passive vape is capable of exerting acute small inflammatory responses in lungs and blood as well as throat irritation. Despite the study being modest in its size with a narrow scope, it offers new findings on the potential harm of e-cigarettes. Although more research is required, it is clear that e-cigarette emissions are not merely harmless aerosol. In the future, we recommend more studies on passive vape exposure in sensitive subgroups, and studies of people chronically exposed to passive vaping, as such studies are virtually non-existent.
Author’s contributions to the study
KRL was responsible for conducting the study, she analyzed the health outcomes, and wrote the first draft of the article. JHB came up with the proposal to the study, designed the study in collaboration with TS and he contributed to the manuscript. EB helped design the study and recruit participants, and she contributed to the manuscript. MB and MG planned and supervised the chemical and particle analysis and contributed to the manuscript. SM and PR measured particle exposure and contributed to the manuscript. PR also controlled the exposure chamber and analyzed the particle data. VHG and KØ helped conduct the study and contributed to the manuscript. ACO helped performing the PExA measurements and she contributed to the manuscript. TS was the principal investigator in designing and conducting the study, and he contributed to the manuscript. All authors read and approved the final manuscript before submission.
Supplemental Material
Download MS Word (3.1 MB)Acknowledgments
We are thankful to PExA AB (Göteborg, Sweden) for lending us the PExA® instrument and performing the analysis, and to Nightingale Health Ltd (Helsinki, Finland) for analyzing the blood samples. We would like to thank K.V. Kristensen for skillful assistance in measuring the particle exposure and R.B. Madsen and J.K. Christensen (Dept. of Chemistry, Aarhus University) for their contributions to chemical analyses. We particularly thank the subjects for their participation in the study.
Disclosure statement
Anna-Carin Olin is a board member and shareholder of PExA AB, and has a patent (wo2009045163) licensed to PExA AB. No potential competing interests was reported by the other authors.
Supplementary material
Supplemental data for this article can be accessed here
Correction Statement
This article has been republished with minor changes. These changes do not impact the academic content of the article.
Additional information
Funding
Notes on contributors
Karin Rosenkilde Laursen
Karin Rosenkilde Laursen is a PhD-student at Department of Public Health, Aarhus University & Danish Ramazzini Center. Her research is centered on experimental research within the field of indoor air pollution and related health effects.
Jakob Hjort Bønløkke
Jakob Hjort Bønløkke is a Medical Doctor and Associate Professor at Department of Occupational and Environmental Medicine, Aalborg University Hospital & Danish Ramazzini Center. His research interests are within the field of occupational and environmental health effects.
Elisabeth Bendstrup
Elisabeth Bendstrup is a Medical Doctor, consultant and Professor at Department of Respiratory Diseases and Allergy at Aarhus University Hospital. Her research is focusing on pulmonary disease – especially it centers on IPF and other rare lung diseases.
Merete Bilde
Merete Bilde is a Professor at Department of Chemistry, Aarhus University. Her research covers atmospheric chemistry with a focus on aerosols and their role in air quality and climate.
Marianne Glasius
Marianne Glasius is an Associate Professor at Department of Chemistry, Aarhus University, where she is developing chemical analysis methods to study chemical processes from molecular to global scales in topics such as air pollution and climate change.
Vibeke Heitmann Gutzke
Vibeke Heitmann Gutzke is a Laboratory technician and Project coordinator at Department of Public Health, Aarhus University & Danish Ramazzini Center. She is a part of the team conducting randomized controlled exposure trials, where her focus is on coordinating trials and examining the health of the participants.
Shamjad Puthukkadan Moosakutty
Shamjad P. Moosakutty is a former Postdoctoral Fellow at Department of Chemistry, Aarhus University. Currently, he is working at the Clean Combustion Research Center, King Abdullah University of Science and Technology in Saudi Arabia.
Anna-Carin Olin
Anna-Carin Olin is a Medical Doctor and Professor at Department of Public Health and Community Medicine, University of Gothenburg, where she is doing research in respiratory medicine and discovery of early biomarkers.
Peter Ravn
Peter Ravn is a Technician at the exposure chambers at Department of Public Health, Aarhus University & Danish Ramazzini Center. He is a part of the team conducting randomized controlled exposure trials, where he establishes, controls, and measure exposures.
Kirsten Østergaard
Kirsten Østergaard is a Laboratory technician at Department of Public Health, Aarhus University & Danish Ramazzini Center. She is a part of the team conducting randomized controlled exposure trials, where her focus is on health examination of the participants and analyzation of biomarkers in collected samples.
Torben Sigsgaard
Torben Sigsgaard is a Professor at Department of Public Health, Aarhus University & Danish Ramazzini Center. He is conducting research within the field of ambient and indoor air pollution and related health effects, including gene-environment interaction. Especially, his focus is on respiratory disease and allergy.
References
- The Lancet. E-cigarettes: time to realign our approach? Lancet. 2019;394(10206):1297.
- Pisinger C, Dagli E, Filippidis FT, et al. ERS and tobacco harm reduction. Eur Respir J. 2019;54(6):1–11.
- National Academies of Sciences, Engineering, and Medicine. Public health consequences of E-cigarettes. Washington, DC: The National Academies Press; 2018.
- Pisinger C, Døssing M. A systematic review of health effects of electronic cigarettes. Prev Med. 2014;69:248–260.
- Layden JE, Ghinai I, Pray I, et al. Pulmonary illness related to e-cigarette use in Illinois and Wisconsin - final report. N Engl J Med. 2020;382(10):903–916.
- Bhatta DN, Glantz SA. Association of e-cigarette use with respiratory disease among adults: a longitudinal analysis. Am J Prev Med. 2020;58(2):182–190.
- Soneji S, Barrington-Trimis JL, Wills TA, et al. Association between initial use of e-cigarettes and subsequent cigarette smoking among adolescents and young adults a systematic review and meta-analysis. JAMA Pediatr. 2017;171(8):788–797.
- Kennedy CD, van Schalkwyk MCI, McKee M, et al. The cardiovascular effects of electronic cigarettes: A systematic review of experimental studies. Prev Med. 127 (2019) 105770. DOI:https://doi.org/10.1016/j.ypmed.2019.105770.
- Skotsimara G, Antonopoulos AS, Oikonomou E, et al. Cardiovascular effects of electronic cigarettes: A systematic review and meta-analysis. Eur J Prev Cardiol. 2019;26(11):1219–1228.
- Chun LF, Moazed F, Calfee CS, et al. Pulmonary toxicity of e-cigarettes. Am J Physiol - Lung Cell Mol Physiol. 2017;313(2):L193–206.
- Pisinger C, WHO. A systematic review of health effects of electronic cigarettes. 2015.
- World Health Organization. Exposure to aerosol from smoking-proxy electronic inhaling systems: a systematic review. World Health Organization, Barcelona, 2016. https://www.who.int/tobacco/industry/product_regulation/BackgroundPapersENDS1_4november.pdf?ua=1.
- Cheng T. Chemical evaluation of electronic cigarettes. Tob Control. 2014;23(SUPPL. 2):ii11–ii17EMJEEEMEEE.
- WHO Framework Convention on Tobacco Control. Electronic nicotine delivery systems and electronic non-nicotine delivery systems (ENDS/ENNDS). In Delhi, India: WHO; 2016. p. 1–11.
- Postma DS, Kerstjens HAM. Characteristics of airway hyperresponsiveness in asthma and chronic obstructive pulmonary disease. Am J Respir Crit Care Med. 1998;158(5 III). DOI:https://doi.org/10.1164/ajrccm.158.supplement_2.13tac170
- Twisk JWR. Applied longitudinal data analysis for epidemiology. A practical guide. 2nd ed. Cambridge: Cambridge University Press; 2013.
- Khlystov A, Samburova V. Flavoring compounds dominate toxic aldehyde production during E‑cigarette vaping. Environ Sci Technol. 2016;50(23):13080–13085.
- Sleiman M, Logue JM, Montesinos VN, et al. Emissions from electronic cigarettes: key parameters affecting the release of harmful chemicals. Environ Sci Technol. 2016;50(17):9644–9651.
- Klager S, Vallarino J, MacNaughton P, et al. Flavoring chemicals and aldehydes in e-cigarette emissions. Environ Sci Technol. 2017;51(18):10806–10813.
- Visser WF, Klerx WN, Cremers HWJM, et al. The health risks of electronic cigarette use to bystanders. Int J Environ Res Public Health. 2019;16:9.
- McKenzie Z, Kendall M, Mackay RM, et al. Surfactant protein A (SP-A) inhibits agglomeration and macrophage uptake of toxic amine modified nanoparticles. Nanotoxicology. 2015;9(8):952–962.
- Nathan N, Taytard J, Duquesnoy P, et al. Surfactant protein A: A key player in lung homeostasis. Int J Biochem Cell Biol. 2016;81:151–155.
- Wright JR, Borron P, Brinker KG, et al. Surfactant protein A: regulation of innate and adaptive immune responses in lung inflammation. Am J Respir Cell Mol Biol. 2001;24(5):513–517.
- Flouris AD, Chorti MS, Poulianiti KP, et al. Acute impact of active and passive electronic cigarette smoking on serum cotinine and lung function. Inhal Toxicol. 2013;25(2):91–101.
- Bønløkke JH, Stridh G, Sigsgaard T, et al. Upper-airway inflammation in relation to dust spiked with aldehydes or glucan. Scand J Work Environ Heal. 2006;32(5):374–382.
- Gotts JE, Jordt SE, McConnell R, et al. What are the respiratory effects of e-cigarettes? BMJ. 2019;366:1–16.
- Tzortzi A, Teloniatis S, Matiampa G. et al. Passive exposure to e-cigarette emissions: minor respiratory effects. Tob Prev Cessat. 2018;4(Supplement):1–8.
- Jansen KL, Larson TV, Koenig JQ, et al. Associations between health effects and particulate matter and black carbon in subjects with respiratory disease. Environ Health Perspect. 2005;113(12):1741–1746.
- Steerenberg PA, Snelder JB, Fischer PH, et al. Increased exhaled nitric oxide on days with high outdoor air pollution is of endogenous origin. Eur Respir J. 1999;13(2):334–337.
- Marini S, Buonanno G, Stabile L, et al. Short-term effects of electronic and tobacco cigarettes on exhaled nitric oxide. Toxicol Appl Pharmacol. 2014;278(1):9–15.
- Malinovschi A, Janson C, Holmkvist T, et al. Effect of smoking on exhaled nitric oxide and flow-independent nitric oxide exchange parameters. Eur Respir J. 2006;28(2):339–345.
- Vardavas CI, Anagnostopoulos N, Kougias M, et al. Short-term pulmonary effects of using an electronic cigarette: impact on respiratory flow resistance, impedance, and exhaled nitric oxide. Chest. 2012;141(6):1400–1406.
- Meo SA, Ansary MA, Barayan FR, et al. Electronic cigarettes: impact on lung function and fractional exhaled nitric oxide among healthy adults. Am J Mens Health. 2019;13:1.
- Hoyt JC, Robbins RA, Habib M, et al. Cigarette smoke decreases inducible nitric oxide synthase in lung epithelial cells. Exp Lung Res. 2003;29(1):17–28.
- Costello L, Franklin RB. Plasma citrate homeostasis: how it is regulated; and its physiological and clinical implications. an important, but neglected, relationship in medicine. HSOA J Hum Endocrinol. 2016;1(1):1–19.
- Ito F, Ito T. High-density lipoprotein (Hdl) triglyceride and oxidized HDL: new lipid biomarkers of lipoprotein-related atherosclerotic cardiovascular disease. Antioxidants. 2020;9(5). DOI:https://doi.org/10.3390/antiox9050362
- Dicpinigaitis PV, Chang AL, Dicpinigaitis AJ, et al. Effect of e-cigarette use on cough reflex sensitivity. Chest. 2016;149(1):161–165.
- Bayly JE, Bernat D, Porter L, et al. Secondhand exposure to aerosols from electronic nicotine delivery systems and asthma exacerbations among youth with asthma. Chest. 2019;155(1):88–93.
- Schripp T, Markewitz D, Uhde E, et al. Does e-cigarette consumption cause passive vaping? Indoor Air. 2013;23(1):25–31.
- Dinakar C, O’Connor GT. The health effects of electronic cigarettes. N Engl J Med. 2016;375(14):1372–1381.
- Morean ME, Kong G, Cavallo DA, et al. Nicotine concentration of e-cigarettes used by adolescents. Drug Alcohol Depend. 2016;167(167):224–227.