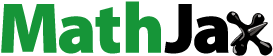
Abstract
Ports are complex systems that play a crucial role in the transportation of maritime-related products and services. Since ports face various safety issues in design, installation, operation and maintenance processes, there are many aspects open for improvement in Occupational Health and Safety risk assessment and it is required to deal with risks, reduce them to an acceptable level and control. To struggle with emerging risks and manage this process better, a risk assessment framework is proposed for ports in this study. A case study is performed in a Turkish international port authority using the proposed framework. Fuzzy Analytic Hierarchy Process (FAHP), which is a commonly used fuzzy multi-criteria decision-making method, was used in weighting two risk factors: likelihood and severity. The orders of priority of hazards were then determined by a fuzzy-based VIKOR method based on the output of FAHP. The proposed framework overcomes some drawbacks, including a lack of assignment on the weight to two risk assessment factors and lack of the high dependence of the prioritisation on the experts’ opinions. To validate the results, a sensitivity analysis was conducted. In conclusion, the study contributes to the applicability of the proposed framework to assess the risks in ports and provides a distinct risk prioritisation.
1. Introduction
Ports are important facilities that play key roles in the transportation of goods and people (Antão et al. Citation2016; Mokhtari et al. Citation2011; Mokhtari et al. Citation2012). The demand for ports, terminals and other service-related infrastructures is influenced by the growth of container transportation network (Celik et al. Citation2009). According to the figures of Port Operators Association of Turkey, the compound annual growth rate of the total volume of container traffic in Turkish ports has reached an increase of more than 10% after 2009 (Marine Industry Report-Citation2014, Citation2015). However, besides its significance and installed capacity, port operations and processes involve various risks during their design, installation, operation and maintenance phases (Alyami et al. Citation2014). The sector workers are exposed to risks that can result in loss of lives and serious injuries during the various phases of a port. To create a safe and healthy work environment and ensure sustainability in ports, the determination of existing and external hazard sources and the management of the risks occurred gain great importance (Alyami et al. Citation2016).
OHS risk assessment (OSHRA) methods are commonly used to find the causes and characteristics of accidents. Recently, new quantitative methods have been proposed against classical OSHRA approaches to uncover occupational risks. Multi-criteria decision-making (MCDM)-based risk assessment methods are one of the recently emerged quantitative OHSRA methods. In MCDM methods, decision makers frequently face difficulty in the evaluation of giving a precise rating to a hazard with respect to the risk factors. Therefore, classical risk assessment methods cannot give satisfactory results due to the incomplete risk data or the availability of high level of vagueness. In that case, fuzzy-based MCDM is adopted to model this uncertainty. Evaluating the relative importance of risk factors, using fuzzy numbers instead of crisp numbers, is one of the most important advantages of fuzzy MCDM-based OHSRA methods. In this paper, we apply fuzzy MCDM in the assessment of potential port hazards. A number of attempts for MCDM approach in OHSRA have appeared in the literature for various sectors (Gul et al. Citation2019; Yucesan and Kahraman Citation2019; Yazdi Citation2018; Mete Citation2018; Gul Citation2018a, Citation2018b; Ak and Gul Citation2018; Oz et al. Citation2018; Gul, Guneri, Nasirli Citation2018; Gul, Guneri, Baskan Citation2018; Gul, Guven, et al. Citation2018; Yazdi et al. Citation2018; Yazdi Citation2017; Gul et al. Citation2017; Ozdemir et al. Citation2017; Sahin and Kum Citation2015; Mahdevari et al. Citation2014).
In this study, a combined framework is proposed for port occupational risk assessment. The current study provides some contributions from both methodological and application point of view. Unlike classical OHSRA methods, decision makers assign risk factor weights by the fuzzy linguistic scale and pairwise comparison manner of Buckley’s FAHP. Several classical OHSRA methods neglect the assignment of a relative importance weight to the related risk factors. For example, in the decision matrix method, Fine-Kinney method and Failure Mode and Effects Analysis (FMEA), weights of risk factors are not considered in risk priority value computation. The proposed framework also includes integration of experts’ opinions on risk prioritisation using fuzzy-based VIKOR. To the best of our knowledge, this is the first attempt in OHSRA context in assessing the risks for an international port authority that uses a combined fuzzy approach.
The remainder of this study proceeds as follows. The next section presents a related review of literature and reveals the research gap that this study addresses. Section 3 presents the research methods. In Section 4, an application case study is presented. Some concluding remarks and future recommendations are provided in the last section.
2. Review of the literature
Risk assessment is an important concept for almost all sectors. So, the port industry contains high-risk operations, much attention is paid to the risk assessment process. The International Maritime Organization suggested a process consisted of five steps, which are hazards identification, risk analysis, development of control measures to reduce risks, cost–benefit analysis and conclusions and recommendation (Zhang et al. Citation2016; Kristiansen Citation2013; Wang Citation2006). The last step of conclusions and recommendation corresponds to ‘Monitoring & Review’ in classical OHSRA. The fertility in risk assessment methods indicates the importance of selection of the appropriate one (Guneri et al. Citation2015). Several methods are developed to assess risks up to now. These methods are mostly examined under two main groups as qualitative and quantitative (Tixier et al. Citation2002; Reniers et al. Citation2005; Marhavilas et al. Citation2011). Quantitative risk assessments, which incorporate historical data and expert judgements, have attracted much more attention than qualitative approaches and they have been applied to the port operations. Many studies are available in the OHS risk management literature related to the ports with different risk assessment approaches. Table shows a comparative summary about a number of recent studies for port OHS risk management.
Table 1 Previous OHSRA studies for port operations.
Regarding risk analysis for container port safety evaluation and the safety performance of a container terminal operational system, Alyami et al. (Citation2016) and Alyami et al. (Citation2014) constitute the most important two studies. In the first study, they proposed a new method for the application of FMEA in assessing the safety performance of container terminal operational system. They incorporated a Fuzzy Rule-Based Bayesian Network (FRBN) with Evidential Reasoning (ER) in a complementary manner. The FRBN provided input failure information for risk estimates of individual hazardous events (HEs) at the bottom level of a risk assessment hierarchy. The ER was used to aggregate HE safety estimates collectively. In the second study, they only benefited from FRBN to evaluate the criticality of the HEs in a container terminal. Mokhtari et al. (Citation2011) integrated generic bow-tie framework with the risk assessment phase as a backbone of the phase of the proposed risk management framework used for the purpose of seaports and offshore terminal operations and management. They applied Fault Tree Analysis (FTA) and Event Tree Analysis (ETA) to analyse the risk factors associated within the ports and offshore terminals and used fuzzy sets to deal with the vagueness of the data. On conclusion of their study, port professionals and port risk managers benefitted from the identified risk factors more in detail. In another study by Mokhtari et al. (Citation2012), they used Fuzzy Set Theory to describe and evaluate the associated risk factors within the ports and terminal operations and management and used ER to synthesise the information produced. They carried out a case study in three Southern Iranian ports by using an illustrative operational risk hierarchy. Bayesian Belief Networks (BBN) and Fuzzy Evidential Reasoning (FER) methods were considered in the risk evaluation of port operations. Zhang et al. (Citation2016) used BBN to express the dependencies between the indicator variables and accident consequences in the Tianjin port, China. They first made a statistical analysis of historical accident data of six years from 2008 to 2013 prior to applying BBN. They also carried out a sensitivity analysis to see the influence of several indicator variables on the consequences including navigational area, ship type and time of the day. They reached a conclusion that the navigational risk of the Tianjin port is at the acceptable level. Yang et al. (Citation2014) dealt with FER to facilitate the quantitative analysis of port facility security assessment.
In addition to the above-explained studies, many contributions are made to the literature by some studies, such as John et al. (Citation2018); Ozturkoglu et al. (Citation2019); Cao and Lam (Citation2019); Efe (Citation2019). John et al. (Citation2018) applied FAHP to analyse the complex structure of the seaport security system and determine the weights of security systems/measures while used ER to synthesise the risk analysis. Ozturkoglu et al. (Citation2019) defined risks in the ship recycling industry by using triple bottom line approach and developed a risk-based conceptual model, including with potential risks and proposed future state with preventative actions for risks. Fuzzy Decision-Making Trial and Evaluation Laboratory is used due to its ability to solve complex problems by providing causal relations between factors, in addition to the order of importance. In Cao and Lam (Citation2019), the vulnerability of the four assessment units of the Tianjin Port was assessed the under the background of 2015 explosion. A fast reaction-based port vulnerability assessment framework integrating Fuzzy Technique for Order Preference by Similarity Ideal Solution and FER was proposed. Efe (Citation2019) proposed a flexible and suitable model for FMEA-based risk evaluation using intuitionistic fuzzy numbers. In that study, intuitionistic fuzzy numbers-based quality function deployment approach determines the weights of design requirements for each FMEA criterion (occurrence, severity and detection). The intuitionistic fuzzy numbers-based VIKOR approach then ranks the occupational accidents-related failure modes in shipbuilding.
From the overview of previously related work, it is concluded that the use of MCDM methods in OHSRA of ports is indeed new and proper. The majority of recent studies have been developed to deal with classical methods such as FMEA, FTA, ETA, BBN and ER. Therefore, the use of this combined fuzzy-based framework can further help the stakeholders determine national- and macroscale-risk control policies for ports.
3 Research methods
In the OHSRA literature, experts often distinguish between risk analysis and risk assessment. Risk analysis is the process of calculating the risk for the identified hazards quantitatively or qualitatively. Risk assessment is the process of using the results obtained in the risk analysis to improve the safety of a workplace through risk reduction (Kristiansen Citation2013). This involves the introduction of safety measures, also known as risk control options. A usual flow chart for the process of risk analysis and risk assessment is demonstrated in Figure . The framework proposed in this study concerns the risk analysis phase of a usual risk assessment. It starts with a hazard identification and then continues by a risk estimation, selection of risk control options, reducing risks and monitoring and review. The risk analysis phase is to calculate the risk value based on two parameters of decision matrix method, risk likelihood and risk severity (Acuner and Cebi Citation2016). The risk reduction step enables the process to become more efficient so that significant risks are eliminated fast by using hazard control hierarchy. In order to reduce the risks of hazards to as low a level as reasonably practicable (ALARP), the introduction of safety measures into the system will be necessary.
3.1. Proposed methodology
The structure of the proposed framework we followed in this study is given in Figure . The steps of the proposed framework are presented in the following sections.
3.1.1. Hazard identification
First, a risk assessment team, consisting of port OHS experts with different maritime-related background, is established. Then, potential hazards that emerged in four different workplaces of the port are identified: (1) Dock, (2) Offices, (3) The whole port and (4) Workshop. This kind of classification makes the risk assessment easier. Through this step, 40 potential hazards are identified by the expert team, as provided in Table .
Table 2 Hazards list and their descriptions.
3.1.2. Assessing hazards
The specific merit of this paper is inside this step. In this step, Buckley’s FAHP is used in weighting two risk factors by taking into consideration pairwise comparison and fuzzy linguistic ratings. In the literature, classic OHSRA methods mostly consider equal weights to two (e.g. likelihood and severity in decision matrix method) or three (e.g. likelihood, severity and frequency in Fine-Kinney method and likelihood, severity and detection in FMEA method) risk factors. Moreover, different combinations of judgements on the parameters may lead to a completely different meaning. For example, hazards with high likelihood and low severity could be classified at the same level as hazards with low likelihood and high severity. These minuses are expressed in the literature (Gul and Ak Citation2018). So, this paper considers weighting of the two parameters of decision matrix method by Buckley’s FAHP calculations. The priority orders of 40 different hazards with respect to these parameters are then determined by using a fuzzy-based VIKOR (FVIKOR) (see Figure ).
The first phase of assessing hazards step is regarding FAHP calculations. FAHP is one of the most used fuzzy-based MCDM methods. Since classical analytic hierarchy process (AHP) cannot provide the subjective thinking manner, FAHP is proposed in order to solve hierarchical problems under fuzzy environment (Kubler et al. Citation2016). Since some FAHP methods have limitations, Buckley's (Citation1985) method was used in the application of this paper. For instance, the extent analysis method (Chang Citation1996) could not make full use of all the fuzzy comparison matrix information and might cause an irrational zero weight to the selection criteria (Chan and Wang Citation2013). Sahin and Yip (Citation2017) proposed a Gaussian fuzzy number-based AHP to eliminate the case of zero weights. In another study, consistency control and expert consistency prioritisation are investigated by using Chang’s extent analysis method of trapezoidal FAHP (Sahin Citation2017). In Radionovs and Uzhga-Rebrov (Citation2017)’s study, Chang’s and Buckley’s methods are considered as the best for the risk assessment. In the study, the main advantages and disadvantages of FAHP in the context of risk assessment are also emphasised.
In the current study, we have applied Buckley’s approach under triangular fuzzy numbers. Our study does not aim to find a solution for a selection problem as Sahin and Yip (Citation2017)’s study. There are only two criteria to compare pairwisely in the current study (risk factors of likelihood and severity). The aim is to weigh these two factors. The non-secondary method that will determine the prioritisation essentially is FVIKOR. Also, the problem is not so huge to check consistency. As the extent analysis has abovementioned pitfalls, Buckley’s approach under triangular fuzzy numbers is applied. The process of Buckley’s FAHP method was presented as in the following (Tzeng and Huang Citation2011; Gul and Guneri Citation2016):
Step 1: Pairwise comparisons are constructed among decision criteria. Linguistic terms are assigned by asking which is more important of the two risk factors, such as likelihood and severity. The linguistic terms and corresponding fuzzy values for the evaluation of risk factors in this study are provided in Table .
(1)
(1)
(2)
(2)
Table 3 Linguistic terms and corresponding fuzzy values for risk factor weighting.
Step 2: Fuzzy geometric mean matrix is defined using the geometric mean technique.
(3)
(3)
Step 3: Fuzzy weights of each criterion are obtained by equation (4).
(4)
(4) Here,
is the fuzzy weight of criterion i. And
.
Here, ,
,
justify lower, middle and upper value of the fuzzy weight of criterion i, respectively.
Step 4: Centre of area (CoA) method is used to find the best non-fuzzy performance, as in Equation (5)
(5)
(5) The second phase of assessing hazard step is FVIKOR process. VlseKriterijumska Optimizacija I Kompromisno Resenje (VIKOR; that means multi-criteria optimisation and compromise solution) is one of the MCDM methods developed by Opricovic (Citation1998) for multi-criteria optimisation problems and compromise solutions. It ranks alternatives and determines the compromise solution that is the closest to the ‘ideal’. The fuzzy version of this method FVIKOR involves fuzzy assessments of criteria and alternatives in VIKOR. For a recent literature review on VIKOR-based applications and their fuzzy extensions, see Gul et al. (Citation2016). The steps of FVIKOR method can be described as follows.
Step 1: Defuzzification of the elements of fuzzy decision matrix for the criteria weights and the alternatives into crisp values are carried out. A fuzzy number can be converted into a crisp number a by Equation (6):
(6)
(6) The expert team members use the linguistic rating variables shown in Table to evaluate the ratings of alternatives (hazards) with respect to each criterion (risk factor).
Table 4 Linguistic terms and corresponding fuzzy values for hazard rankings (Chen Citation2000).
Step 2: The best and worst values of all criteria ratings (j = 1, 2, … , n) and alternatives (i = 1, 2, … , m) are determined using Equations (7)–(8).
(7)
(7)
(8)
(8) Step 3: Siand Ri values are calculated using Equations (9)–(10).
(9)
(9)
(10)
(10) Step 4: Qi values are calculated using Equation (11).
(11)
(11) where
. v is the weight for the strategy of maximum group utility and 1−v is the weight of the individual regret.
Step 5: Alternatives are ranked sorting by the values S, R and Q in an ascending order.
Step 6: As a compromise solution the alternative (A(1)), which is best ranked by the measure Q (minimum), is proposed if the following two conditions are satisfied.
Condition 1:
‘Acceptable advantage’: Adv ≥ DQ
where is the advantage rate of the alternative A(1) ranked first, A(2) is the alternative with the second position in {A}Q and the threshold
.
Condition 2:
‘Acceptable stability in decision making’:
The alternative A(1) must also be best ranked by S or/and R. The compromise solution is stable within a decision-making process, which could be the strategy of maximum group utility (when v > 0.5 is needed), or ‘‘by consensus v ≅ 0.5’, or ‘‘with veto’ (v < 0.5). Please note that v is the weight of the decision-making strategy of maximum group utility. If one of the conditions is not satisfied, then a set of compromise solutions is proposed, which consists of:
Alternatives A(1) and A(2) if only the condition 2 is not satisfied or
Alternatives A(1); A(2); … ; A(M) if the condition 1 is not satisfied; A(M) is determined by the relation for maximum M (the position of these alternatives are in closeness).
The remained three steps of the proposed methodology are relating with the ranking of risks from highest to lowest based on their risk score and selection of the best control option and documentation. At this step, Acuner and Cebi (Citation2016) proposed control options such as eliminating hazards at their source, replacing a source of hazard with a less dangerous source of hazard, taking engineering controls on the source, taking organisational administrative controls on the source and using personal proactive equipment.
4 Case study
In this paper, the risk assessment of an international port authority, which is situated in the Marmara Region, was examined in order to illustrate the proposed framework. After hazard identification step, by utilising Buckley’s FAHP method, evaluations of expert team members (ETMs) in linguistic variables were used to determine the importance of two risk factors (Likelihood and Severity) by a pairwise comparison.
In the current study, three experts participated in rating and analysing occupational hazard risks in relation to the port. All members are OHS experts with C class certificate. They have enough experience in maritime and port operations. Class C experts are eligible to work in the less dangerous worksites than those with class B experts. Experts with B certificates work in dangerous worksites. Experts with Class A certificates can work in all hazardous worksite types. It is necessary to work for at least 4 years as a Class B OHS expert in order to be certified as a Class A OHS expert. Since the experience levels (year of experience regarding port operations) are close to each other, we have assumed equal importance to them. In the literature, there exists some novel methods for expertise coefficient determination (Şahin and Yazır Citation2019; Yazdi Citation2019). These methods consider job experience, education level, status/title and so on.
The evaluation in linguistic form is presented in Table . For instance, when comparing the risk factors ‘likelihood’ and ‘severity’, the responses of three members are slightly weak (SW), very weak (VW) and very weak (VW). After applying FAHP the weights of risk factors are obtained as (0.379 and 0.621) for likelihood and severity, respectively.
Table 5 Evaluations of expert team members in linguistic variables and weights of the risk factors.
After determining the weights of two risk factors by Buckley’s FAHP, the fuzzy evaluations of each risk factor with respect to hazards in the observed port, FVIKOR is applied. In the paper, the ETMs made all evaluations of the most important hazards using linguistic variables using the scale, as shown in Table . The evaluations of the ETMs in linguistic variables for the risk factors with respect to 40 different hazards are expressed, as in Table .
Table 6 Linguistic assessment for the most important hazards in the observed port.
For example, the first ETM evaluated the hazard D1 as medium poor (MP) and medium poor (MP) respectively for likelihood and severity. The linguistic ratings are converted into fuzzy triangular numbers and then aggregate ratings are generated, as explained in Awasthi and Kannan (Citation2016). The fuzzy ratings for the hazard sources are converted into aggregated crisp ratings using Equation (6). For example, for hazard D1 with respect to likelihood parameter as (1, 3.67, 7) is converted to a crisp value of (1 + 4*3.67 + 7)/6 = 3.78. The results for aggregated crisp ratings of the 40 hazards with respect to two parameters are presented in Table . Based on these values, the best and the worst values
of the three parameters are computed using Equations (7) and (8), respectively (Table ). Si, Ri and Qi values for the hazards are calculated using Equations (9)–(11), respectively. The values of
,
,
,
are obtained using related formulas.
Table 7 Aggregated crisp ratings, the best and the worst values of likelihood and severity (for risk assessment of the observed port).
Figure ranks the hazards, sorting by the values Si, Ri and Qi in an ascending order. The minimum values are ranked highest risk, while risks having Si, Ri and Qi values closest from 1 is ranked lowest risk. Results show that the most important hazards in the dock of the observed port are stemmed from working without helmet (D2), working at height (D3), standing under load (D4) and not opening of magnesite vessel covers (D7). The first three hazards occur during loading and unloading activities, while the last one occurs under working with magnesite. In Sahin and Kum (Citation2015)’s study, which is focused on navigational risk factors, some similar results were obtained. Human–machine interface problems and faults in ergonomics, design and operation of the devices are determined as the most important risks with higher weights. Regarding the hazards in office buildings, electric shock, while the usage of computer and other machines such as printer, copier and fax (O5 and O7), is determined as the most important. In the whole port operations, the risk of hitting and to be hit while working with forklift (P4) and lack of dual carriageways and non-determination of speed limits in the case of passenger car traffic (P10) are obtained as the most critical hazards. Regarding workshop hazards, overthrowing and collapsing during stacking materials (W4), air tank explosion in compressor usage (W6) and oxygen cylinders in working with the pressure tube (W8) have given a Qi value of zero that means the most serious hazards in this station. The overall risk assessment and management cannot eliminate risks altogether but can only suggest suitable control measures. Therefore, after completing each stages of risk assessment, each risk should be controlled or eliminated. If this is not possible, they should be reduced to an acceptable level (Mahdevari et al. Citation2014).
The compromised ranking of the hazards is shown in Figure . In this case of dock of the port, the compromised rank 1 (first cluster consisting of D2, D3, D4 and D7) represents the hazard value closest to the ideal solution which means it has the most serious risk, and similarly the highest rank 4 (fourth cluster consisting of only the hazard D1) represents those hazards which are having least risk associated with them. The second cluster (D9 and D10) and third cluster (D8, D5 and D6) represent the moderate risk levels. Similar analyses have been fulfilled in the remaining three stations in the observed port authority, as shown in Figure .
4.1 Potential control measures
In this section, we provide discussions on the measures that should be taken to control risks in each station of the observed port. Regarding the hazards in the dock, Table shows the potential control measures. For the most serious hazards placed at Cluster-1, measures, such as providing the use of helmets for all employees, ensuring the use of appropriate scaffolding, platforms and mobile vehicles during works at height, hanging of a warning sign as ‘Do not stand under suspended loads’ in the workplaces and providing the magnesite cap gains open easily by making consultation with the manufacturer, should be taken into consideration. For the least serious hazards placed at Cluster-4, all employees should be provided wear steel-based steel-toed work shoes.
Table 8 Control measures for the hazards in the dock of the observed port.
Regarding the hazards in the offices, Table shows the potential control measures. For the most serious hazards placed at Cluster-1, a crucial measure of 30 mA leakage current relay installation and periodic monitoring of grounding suitability of all electrical installations for each year should be taken into consideration. For the least serious hazards placed at Cluster-3, several measures should be suggested as follows: (1) performing periodic control of electrical installations, implementation of fire alarm systems and detection systems and training of employees, (2) providing a non-slippery ground against fallings, (3) taking periodic eye examinations and providing a minimum illumination of 500 lux, (4) providing ergonomic chairs and completing employee ergonomics training, (5) fixing the furniture longer than 150 cm, (6) completing the training of employees, (7) using protective gloves and (8) ensuring the use of gloves preventing the liquid touch.
Table 9 Control measures for the hazards in the offices of the observed port.
The measures against the most critical risks obtained from the risk assessment through the whole port are as follows: (1) Forklifts should not be loaded by blocking the operator’s view and pallets should be loaded individually. (2) Parking area of passenger cars in the workplaces must be determined. It is not allowed to leave the vehicles chaotically. The speed limit should be determined for vehicles with loading and subcontractors must be notified. Areas where pedestrians can be in the dock area should be designed so as not to enter the vehicle and must be separated. For the least serious hazard placed at Cluster-3 through the whole port (P1), training should be provided for manual handling. A worker should not lift more than 25 kg and loads more than 50 kg should be transported by lift trucks.
Regarding the hazards in the workshop of the observed port, three of the most important hazards (Cluster-1) are emerged as W4, W6 and W8. To reduce the risks related to W4, materials in the workplaces must be stacked on the ground which sustain on load. The height of the packing material should be such that it does not exceed 3 m. Workers should be trained in this regard. Regarding W6, hydraulic control of the air tank and periodic pressure test of the compressor must be performed by technical staff at least annually. So, an explosion that may arise due to material fatigue, corrosion and so on reasons should be avoided. Periodic controls should not be postponed or neglected for reasons such as work intensity. Regarding W8, oxygen cylinders-related hazards required notices and warnings must be put into practice in order to prevent touching of workers to the oxygen tubes with oily hands considering oxygen is burning. Tables – also show the summary of control measures for the hazards in the whole port and workshop of the observed port, respectively.
Table 10 Control measures for the hazards in the whole port.
Table 11 Control measures for the hazards in the workshop of the observed port.
4.2 Sensitivity analysis
One of the most popular validation methods is conducting sensitivity analysis (Alyami et al. Citation2014). In order to test the accuracy of the result performance of the proposed FAHP and FVIKOR combined methodology, the weights gained from the FAHP are changed. Three cases are realised during the sensitivity analysis as in Table . More different weight changes can be applied to expand the sensitivity analysis. Thus, the methodology result changes can be seen, and this helps the decision maker to determine the priorities and make the evaluation process easier (Gul and Guneri Citation2016). The results of the sensitivity analysis can be seen from Figure .
Table 12 Weights of the risk factors with respect to the considered cases.
Sensitivity analysis shows that the ranking among the hazards is quite sensitive to the changes considering the weights of the two risk factors. In all cases (Case 1, Case 2 and Case 3), the first three most important hazards of each station in the observed port have not been changed excluding in the workshop. In Case 2, as the weight of severity is highest, the most important hazard types remained the same for each station. The least important hazard type in the workshop is W5 for all cases. In the whole port and dock, the least critical hazard has not been changed for Case 1 and Case 2 but varied in Case 3. According to the analysis above, this paper finds that the proposed framework can produce reasonable results and provide suitable information to assist management in the risk assessment problems.
A second sensitivity analysis is carried out by varying the value of v which in this study is set to 0.5. Ten values of v are run (from 0.0 to 1.0 increasing by 0.1) to assess the results. The outputs of the second sensitivity analysis are demonstrated in Figure . D2, D3, D4 and D7 have an equal Q value of zero. It means that they have the best ranking in all ten cases regarding v value. They are the most serious and riskiest hazards for the dock operations. This type of sensitivity analysis (sensitivity analysis based on v value) also confirms that the results of the ranking orders are consistent. The results prove that the proposed framework yields reasonable results and presents suitable outcomes to support stakeholders in OHS decision-making.
5. Conclusion
There is an unavoidable need for comprehensive methods for identifying and assessing OHS risks and for improving the working conditions in ports. Having considered that ports are complex workplaces, many occupational risks and accidents may emerge regarding the worksite-, worker and work-related issues. These can cause excessive costs. Therefore, decision makers in ports need to use new and proper safety risk assessment frameworks to avoid life and monetary losses. Hence, this study presents a two-stage fuzzy MCDM-based OHSRA framework. The reason to merge these two methods in the proposed framework was to overcome some drawbacks of classical OSHRA methods. To that end, Buckley’s FAHP was used in weighting two risk factors (likelihood and severity). Then, risk prioritisation of hazards was determined by using FVIKOR method. The proposed framework was applied through an international port authority in Turkey to make a distinct prioritisation among hazards in each station of the observed port and suggest potential control measures.
Implementation of the proposed framework to the observed international port indicates that the risk prioritisation according to this new framework is closer to the reality and provides a more accurate ranking to the decision makers than classical methods. Outputs of the case study show that working at height, working without helmet, standing under load and not opening of magnesite vessel covers in the dock of the port; electric shock in the offices; lack of dual carriageways and non-determination of speed limits and leakage current in the whole port were emerged as the most serious hazards. In each station of the observed port, control measures are overtaken for the hazards and a risk re-evaluation is performed. In this regard, the risks regarding dock operations assessed by the proposed framework can be classified in four categories. The risks regarding the offices, the whole port and the workshop can be in three categories. Since the least important risk cluster in the dock of the port includes only one hazard (D1), it can be merged with Cluster-3. Therefore, according to the scores of FVIKOR and experts’ opinions, a three-class categorisation(High (Not acceptable), Medium (Generally acceptable) and Low (Acceptable)) can be created. However, control measures suggested all these three classes, the ones for the low-class risks need not be applied. The ones for high- and medium-class risks are required to be planned and implemented. When comparing the outputs of the proposed framework to those of the classical 5 × 5 matrix method, it can be easily inferred that the proposed prioritisation provides more accurate classification. This inference has been supported by the decision makers of the observed port authority. Additionally, two different sensitivity analyses were carried out to test the accuracy of the result performance of the proposed framework. First one the sensitivity to the changes of weight vector of the two risk factors. Second one is performed by varying the value of v (maximum group utility) which in this study is set to 0.5. Both sensitivity analyses confirm that the results of the ranking orders are consistent.
This study contributes to the context of OHSRA from two sides. From the methodological point of view, (1) a FAHP-based method to risk factor weighting is proposed. Apart from the classical OHSRA methods, decision makers assign criteria weights by pairwise comparison manner of Buckley’s FAHP. (2) Benefiting from the compromised rankings of FVIKOR method, hazards in four different worksites of the observed port were examined under clusters. These clusters present a new viewpoint regarding taking control measures in terms of time period and responsibility. From the application point of view, this study is expected to provide a basis for decisions and policies that must be taken by port authorities in their maritime process. This is the first study in OHSRA in assessing the risks for a port that uses a two-stage (FAHP-FVIKOR) framework. This will further lead the stakeholders in the determination of national and macroscale port risk control policies.
The current study has some limitations. For the second stage of the framework presented in this paper-prioritisation of hazards, it is important to keep in mind that the other MCMD methods (fuzzy TOPSIS, fuzzy ELECTRE, etc.) and/or their combinations can also be used as effective solutions. If any potential difficulty in capturing the decision maker’s judgement with respect to the risk factors using a single set of fuzzy linguistic terms has been emerged, one can consider the application of various versions of fuzzy sets theory (intuitionistic, hesitant, Pythagorean, spherical, etc.) for resolving this kind of problem.
Disclosure statement
No potential conflict of interest was reported by the author.
ORCID
Muhammet Gul http://orcid.org/0000-0002-5319-4289
References
- Acuner O, Cebi S. 2016. An effective risk-preventive model proposal for occupational accidents at shipyards. Brodogradnja. 67(1):67–84.
- Ak MF, Gul M. 2018. AHP-TOPSIS integration extended with Pythagorean fuzzy sets for information security risk analysis. Complex Intell Syst. 5(2):113–126.
- Alyami H, Lee PTW, Yang Z, Riahi R, Bonsall S, Wang J. 2014. An advanced risk analysis approach for container port safety evaluation. Marit Policy Manag. 41(7):634–650.
- Alyami H, Yang Z, Riahi R, Bonsall S, Wang J. 2016. Advanced uncertainty modelling for container port risk analysis. Accid Anal Prev. doi:10.1016/j.aap.2016.08.007.
- Antão P, Calderón M, Puig M, Michail A, Wooldridge C, Darbra RM. 2016. Identification of occupational health, safety, security (OHSS) and environmental performance indicators in port areas. Saf Sci. 85:266–275.
- Awasthi A, Kannan G. 2016. Green supplier development program selection using NGT and VIKOR under fuzzy environment. Comput Ind Eng. 91:100–108.
- Buckley JJ. 1985. Fuzzy hierarchical analysis. Fuzzy Sets Syst. 17(3):233–247.
- Cao X, Lam JSL. 2019. A fast reaction-based port vulnerability assessment: Case of Tianjin port explosion. Transp Res A Policy Pract. 128:11–33.
- Celik M, Cebi S, Kahraman C, Er ID. 2009. Application of axiomatic design and TOPSIS methodologies under fuzzy environment for proposing competitive strategies on Turkish container ports in maritime transportation network. Expert Syst Appl. 36(3):4541–4557.
- Chan HK, Wang X. 2013. Fuzzy extent analysis for food risk assessment. In: Fuzzy hierarchical model for risk assessment. London: Springer; p. 89–114.
- Chang DY. 1996. Applications of the extent analysis method on fuzzy AHP. Eur J Oper Res. 95(3):649–655.
- Chen CT. 2000. Extensions of the TOPSIS for group decision-making under fuzzy environment. Fuzzy Sets Syst. 114(1):1–9.
- Efe B. 2019. Analysis of operational safety risks in shipbuilding using failure mode and effect analysis approach. Ocean Eng. 187:106214.
- Gul M. 2018a. A review of occupational health and safety risk assessment approaches based on multi-criteria decision-making methods and their fuzzy versions. Hum Ecol Risk Assess Int J. 24(7):1723–1760.
- Gul M. 2018b. Application of Pythagorean fuzzy AHP and VIKOR methods in occupational health and safety risk assessment: The case of a gun and rifle barrel external surface oxidation and colouring unit. Int J Occup Saf Ergon. doi:10.1080/10803548.2018.1492251.
- Gul M, Ak MF. 2018. A comparative outline for quantifying risk ratings in occupational health and safety risk assessment. J Cleaner Prod. 196:653–664.
- Gul M, Ak MF, Guneri AF. 2017. Occupational health and safety risk assessment in hospitals: A case study using two-stage fuzzy multi-criteria approach. Hum Ecol Risk Assess Int J. 23(2):187–202.
- Gul M, Ak MF, Guneri AF. 2019. Pythagorean fuzzy VIKOR-based approach for safety risk assessment in mine industry. J Saf Res. 69:135–153.
- Gul M, Celik E, Aydin N, Gumus AT, Guneri AF. 2016. A state of the art literature review of VIKOR and its fuzzy extensions on applications. Appl Soft Comput. 46:60–89.
- Gul M, Guneri AF. 2016. A fuzzy multi criteria risk assessment based on decision matrix technique: A case study for aluminum industry. J Loss Prev Process Ind. 40:89–100.
- Gul M, Guneri AF, Baskan M. 2018. An occupational risk assessment approach for construction and operation period of wind turbines. Glob J Environ Sci Manag. 4(3):281–298.
- Gul M, Guneri AF, Nasirli SM. 2018. A fuzzy-based model for risk assessment of routes in oil transportation. Int J Environ Sci Technol. 1–16.
- Gul M, Guven B, Guneri AF. 2018. A new Fine-Kinney-based risk assessment framework using FAHP-FVIKOR incorporation. J Loss Prev Process Ind. 53:3–16.
- Guneri AF, Gul M, Ozgurler S. 2015. A fuzzy AHP methodology for selection of risk assessment methods in occupational safety. Int J Risk Assess Manag. 18(3–4):319–335.
- John A, Yang Z, Riahi R, Wang J. 2018. A decision support system for the assessment of seaports’ security under fuzzy environment. In: Modeling, computing and data handling methodologies for maritime transportation. Cham: Springer; p. 145–177.
- Kristiansen S. 2013. Maritime transportation: safety management and risk analysis. Vol. 16. London: Routledge; p. 4671–4686.
- Kubler S, Robert J, Derigent W, Voisin A, Le Traon Y. 2016. A state-of the-art survey & testbed of fuzzy AHP (FAHP) applications. Expert Syst Appl. 65:398–422.
- Mahdevari S, Shahriar K, Esfahanipour A. 2014. Human health and safety risks management in underground coal mines using fuzzy TOPSIS. Sci Total Environ. 488:85–99.
- Marhavilas PK, Koulouriotis D, Gemeni V. 2011. Risk analysis and assessment methodologies in the work sites: On a review, classification and comparative study of the scientific literature of the period 2000–2009. J Loss Prev Process Ind. 24(5):477–523.
- Maritime Chambers of Commerce of Istanbul and Marmara, Aegean, Mediterranean, Black Sea Region. 2015. Marine Industry Report-2014. www.denizticaretodasi.org.tr/Shared%20Documents/sektorraporu/2014_sektor_tr.pdf [accessed 2016 Jul 1].
- Mete S. 2018. Assessing occupational risks in pipeline construction using FMEA based AHP-MOORA integrated approach under Pythagorean fuzzy environment. Hum Ecol Risk Assess Int J. doi:10.1080/10807039.2018.1546115.
- Mokhtari K, Ren J, Roberts C, Wang J. 2011. Application of a generic bow-tie based risk analysis framework on risk management of sea ports and offshore terminals. J Hazard Mater. 192(2):465–475.
- Mokhtari K, Ren J, Roberts C, Wang J. 2012. Decision support framework for risk management on sea ports and terminals using fuzzy set theory and evidential reasoning approach. Expert Syst Appl. 39(5):5087–5103.
- Opricovic S. 1998. Multicriteria optimization of civil engineering systems. Faculty of Civil Engineering. Belgrade. 2(1):5–21.
- Oz NE, Mete S, Serin F, Gul M. 2018. Risk assessment for clearing & grading process of a natural gas pipeline project: An extended TOPSIS model with Pythagorean fuzzy sets for prioritizing hazards. Hum Ecol Risk Assess Int J. doi:10.1080/10807039.2018.1495057.
- Ozdemir Y, Gul M, Celik E. 2017. Assessment of occupational hazards and associated risks in fuzzy environment: A case study of a university chemical laboratory. Hum Ecol Risk Assess Int J. 23(4):895–924.
- Ozturkoglu Y, Kazançoğlu Y, Ozkan-Ozen YD. 2019. A sustainable and preventative risk management model for ship recycling industry. J Cleaner Prod. 238:117907.
- Radionovs A, Uzhga-Rebrov O. 2017. Comparison of Different Fuzzy AHP Methodologies in Risk Assessment. In Proceedings of the 11th International Scientific and Practical Conference. Volume II (Vol. 137), p. 142.
- Reniers GLL, Dullaert W, Ale BJM, Soudan K. 2005. Developing an external domino accident prevention framework: Hazwim. J Loss Prev Process Ind. 18(3):127–138.
- Sahin B. 2017. Consistency control and expert consistency prioritization for FFTA by using extent analysis method of trapezoidal FAHP. Appl Soft Comput. 56:46–54.
- Sahin B, Kum S. 2015. Risk assessment of Arctic navigation by using improved fuzzy-AHP approach. Int J Marit Eng. 157(4):241.
- Şahin B, Yazır D. 2019. An analysis for the effects of different approaches used to determine expertise coefficients on improved fuzzy analytical hierarchy process method. J Fac Eng Archit Gazi Univ. 34(1):89–102.
- Sahin B, Yip TL. 2017. Shipping technology selection for dynamic capability based on improved Gaussian fuzzy AHP model. Ocean Eng. 136:233–242.
- Tixier J, Dusserre G, Salvi O, Gaston D. 2002. Review of 62 risk analysis methodologies of industrial plants. J Loss Prev Process Ind. 15(4):291–303.
- Tzeng GH, Huang JJ. 2011. Multiple attribute decision making: methods and applications. Florida: CRC Press.
- Wang J. 2006. Maritime risk assessment and its current status. Qual Reliab Eng Int. 22(1):3–19.
- Yang Z, Ng AK, Wang J. 2014. A new risk quantification approach in port facility security assessment. Transp Res A Policy Pract. 59:72–90.
- Yazdi M. 2017. Hybrid probabilistic risk assessment using fuzzy FTA and fuzzy AHP in a process industry. J Fail Anal Prev. 17(4):756–764.
- Yazdi M. 2018. Risk assessment based on novel intuitionistic fuzzy-hybrid-modified TOPSIS approach. Saf Sci. 110:438–448.
- Yazdi M. 2019. Footprint of knowledge acquisition improvement in failure diagnosis analysis. Qual Reliab Eng Int. 35(1):405–422.
- Yazdi M, Korhan O, Daneshvar S. 2018. Application of fuzzy fault tree analysis based on modified fuzzy AHP and fuzzy TOPSIS for fire and explosion in the process industry. Int J Occup Saf Ergon. 1–17. doi:10.1080/10803548.2018.1454636.
- Yucesan M, Kahraman G. 2019. Risk evaluation and prevention in hydropower plant operations: A model based on Pythagorean fuzzy AHP. Energy Policy. 126:343–351.
- Zhang J, Teixeira ÂP, Guedes Soares C, Yan X, Liu K. 2016. Maritime transportation risk assessment of Tianjin port with Bayesian belief networks. Risk Anal. 36(6):1171–1187.