Abstract
Applications of magnetic and fluorescent poly(ε-caprolactone) (PCL) is increased rapidly in the biomedical engineering field as a bioimaging probe. This urged us to do the present work. In the present investigation, the role of Fe3O4–AR dye nanohybrid on the ring opening polymerization (ROP) of CL was studied at different experimental conditions such as variation in [M] and [M/I]. Prior to ROP of CL, the nanohybrid was characterized by various spectral methods like FTIR spectroscopy, UV–visible spectroscopy, fluorescence spectroscopy, VSM and FESEM like analytical techniques. The Fe3O4–AR nanohybrid is used as an initiator for the ROP of CL. After the formation of PCL/Fe3O4–AR nanocomposite again the systems were characterized by FTIR spectroscopy, UV–visible spectroscopy, fluorescence spectroscopy, DSC, VSM and FESEM like methods. The interesting point noted in the present investigation is the size of Fe3O4 nanoparticle was found to be reduced after the nanohybrid and nanocomposite formation. The three functional groups of AR dye were involved in the ROP of CL. The binding constant and number of binding sites were determined.
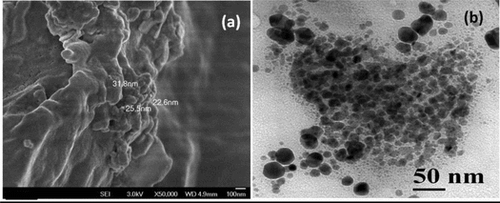
Introduction
Poly(ε-caprolactone) (PCL) is an important biomedical plastic material that holds the potential to reduce the waste associated with the disposal of conventional polymers. The material scientists expect that synthesis of PCL by a bulk and single-step method without using any hazardous solvents and with luminescence and fluorescence properties since it is a biomedical candidate. This will increase the applications of PCL in the biomedical field. Various initiators are used for the synthesis of PCL. For example, in 2010 Ireland and co-workersCitation1 reported about the cationic organomagnesium-initiated ROP of ε-CL. The biomedical PCL can be synthesized using a functionalized initiators such as amine, hydroxyl, carboxyl, thiol, and amide.Citation2–10 The biomedically valuable PCL can be synthesized using isobutyl alumoxane.Citation11 Kinetics and mechanism of anionic ROP of ε-CL were explained in the literature.Citation12 Hydroxy butyl vinyl ether functionalized PCL was reported by Lipik et al.Citation13 A novel Yttrium complex initiated ROP of ε-CL was done by Wu and research team.Citation14 MWCNT-initiated PCL was synthesized and characterized by NMR and WAXS.Citation15 Azemi et al.Citation16 synthesized the solventless enzyme catalyzed ROP of ε-CL. Thiol functionalized,Citation17 heteronuclear base functionalized,Citation18 perfluoroalkane sulfonamide functionalized,Citation19and phosphoester functionalizedCitation20 PCL report is available in the literature. By thorough literature survey, we could not find any report based on the Alizarin red S (AR)-functionalized Fe3O4 initiated ROP of ε-CL and its drug release activity. The present methodology offers a solvent free ROP of ε-CL. Above all; PCL is an effective drug carrier material.
Magnetic nanoparticle particularly Fe3O4, has been effectively studied by various research team.Citation21–23 Unfortunately, the application of Fe3O4 was restricted due to the absence of the functional group on the Fe3O4 surface. Recently, our research team reported about the functionalization of Fe3O4 by different functionalities.Citation24 Synthesis of water soluble cyclic amide functionalized Fe3O4 was reported by Li and co-workers.Citation25 The literature survey reveals that AR-functionalized Fe3O4 report is not available. Recently, the polymer scientists are doing research with targeted drug delivery using Fe3O4, particularly in the photodynamic therapy (PDT) field. This motivated us to do the present investigation because, after the utilization, it is not necessary to remove the iron from the system since it is a biometal.
An organic dye is an important key element in the PDT for the production of reactive oxygen species (ROS) towards the apoptosis of cancer cells. In 2009, Kayrak et al.Citation26 studied the optimization of synthetic method for the production of fluorescent and conductive wool. In 2014, Kohila and research teamCitation27 studied the poly(congored) grafted silk fiber. On going through the literature, we could not find any report based on the AR-functionalized Fe3O4 nanohybrid initiated ROP of CL. In the present investigation, we chose AR dye because of hydroxyl and –SO3Na like functional groups. The present investigation was done towards the synthesis and characterization of fluorescent dye functionalized magnetic nanohybrid. The novelty of the present methodology is an eco-friendly, biocompatible, economically cheaper and single-step preparation without any hazardous solvents.
Chemically conjugated drug delivery systems can be used in an effective way rather than the simply loaded drug delivery systems towards the sustainable release of drug and elimination of side effects in the pharmaceutical field. In this case, the rate of drug release is controlled by the rate of hydrolysis. In 2013, Khazaoi and co-workersCitation28 reported the drug release behavior of poly(styrene-alt-maleicanhydride) system. Sultana et alCitation29 studied the ciprofloxacin hydrochloride release from Povidone K30 matrix. Drug release activity of aspirin loaded PLGA-PEG-PLGA/montmorillonite microparticles was reported in the literature.Citation30 The other authors also reported about the drug release study.Citation31,32 By thorough literature survey, we could not find any report on the Rif. loaded drug release activity of fluorescent and magnetic PCL. Hence, the drug release study is the main aim of the present investigation. In order to avoid the photodegradation of dye molecule during the PDT process, the dye is chemically conjugated with the PCL (as an initiator). Moreover, during the PDT application, lesser amount of dye is injected into the system in order to avoid the side effects and cytotoxic effects. For this purpose, we have considered the AR dye-functionalized Fe3O4 nanohybrid as a chemical initiator for the ROP of ε-CL.
Experimental
Materials
ε-caprolactone (CL, Across chemicals, USA), stannous-2-ethyl hexanoate (Sn(Oct)2, Across chemicals), FeCl3, FeSO4 and NaOH were freshly purchased from s.d fine chemicals, India and used as such. Alizarinred S (AR) was purchased from Spectrum chemicals, India. Diethylether, non-solvent, was purchased from Aldrich chemicals and used as received. CHCl3 solvent was purchased from Aldrich chemicals, India. Rifambin (Rif, Ranbaxy Chemicals, India) was purchased in tablet form and used as such. Double distilled (DD) water was used for the preparation of reaction solutions.
Synthesis of AR-functionalized Fe3O4 nanohybrid system
The novelty of the present investigation is the synthesis of AR-functionalized Fe3O4 and the same is used as an initiator for the ROP of CL. The procedure in brief is mentioned here (Scheme ). About 1 g AR dye was dissolved in 50 mL of DD water, taken in a 500 mL beaker under vigorous stirring conditions. About 2.5 g FeSO4 and 5 g FeCl3 were added inside the beaker under N2 atmosphere at room temperature. Here, the Fe2+ to Fe3+ ratio was maintained at 1:2.Citation33 Further, 100 mL of DD water was added and mixed for 10 min. 10 g NaOH in 100 mL DD water was prepared separately. Thus, prepared NaOH solution was added to the reaction mixture under vigorous stirring condition till the content became dark black. Once the permanent dark black color was obtained, further addition of NaOH solution was stopped. The reaction was allowed further for two hours with stirring. The added NaOH nucleated the formation of Fe3O4. After 2 h of mixing the excess NaOH was removed by placing the beaker on a magnetic bar. Under the influence of magnetic field the AR decorated Fe3O4 molecules settled down, whereas the unreacted NaOH remained in the aqueous medium. The top aqueous layer was removed with the help of a pipette. The precipitate is washed with excess amount of DD water and placed on the magnetic bar. Under the influence of both gravitational and magnetic force, the AR-functionalized Fe3O4 settled down and the liquid was removed. This washing process was continued till the supernatant liquid attains the pH 7.0 and colorless. Finally, the precipitate was freeze dried, weighed, and stored in a zipper lock cover. The same procedure was followed for the synthesis of pure Fe3O4 in the absence of AR molecules. In the present investigation, AR contains two –OH groups and one –SO3Na group. Hence, the AR physically adsorbed on the surface of the ferrite molecules. In 2013, Demin et alCitation29 explained the interaction between the ferrite and alkoxysilane compound. But in the present study, already there are lot of –OH groups present on the surface of ferrite due to NaOH medium, the –OH group of AR may not interact with the ferrite surface. Hence, the only possibility is the interaction of –SO3Na with the metal surface.
Synthesis of PCL/Fe3O4–AR nanocomposites
A two-way necked 25 mL capacity round bottomed (RB) flask was taken and dried well. One neck acts as a sulfur free N2 inlet, whereas the second one acts as a N2 outlet. About 1 g CL was charged in the above said two way necked RB flask. With this 0.001 g Sn(Oct)2 (catalyst) was charged. Here, the [M/C] = 1000. ROP of CL was carried out under different experimental conditions such as [M/I] and monomer variation. Required amount of AR-functionalized Fe3O4 was accurately weighed and mixed with the two-way necked RB flask. The contents were mixed for 10 min under N2 purging at room temperature. After uniform mixing the two-way necked RB flask was kept in an oil bath at 160 °C. The ROP was allowed for 2 h under mild stirring condition.Citation34 At the end of the reaction the N2 purging was stopped and the highly viscous liquid was dissolved in 25-mL CHCl3 solvent. Thus, obtained polymer was purified by re-precipitation with the addition of diethylether. After the drying process for 6 h under fume hood, the Fe3O4/AR nanohybrid end capped PCL was obtained as a white crystalline powder. Thus, obtained white crystalline powder was weighed and stored in a zipper lock cover.
Characterization
Magnetic measurements were carried out with a superconducting quantum interference device magnetometer (Lakesore-7410-VSM, USA) with magnetic fields up to 7 T at 32 °C. FTIR spectra were recorded with the help of Shimadzu 8400 S, Japan model instrument by KBr pelletization method from 400 to 4000 cm−1. Three milligram of polymer sample was ground with 200 mg of spectral grade KBr and made into a disk under the pressure of 7 tons. 1H and 13C-NMR spectra of the polymer samples were recorded by using Bruker Biospin High Resolution Digital 300 MHz NMR spectrometer, USA. Dueterated chloroform (CDCl3) was used as a solvent, and tetramethyl silane (TMS) served as an internal standard. UV–visible spectrum was measured by dissolving the sample in chloroform solvent. The instrument used here is Shimadzu UV-3600, Japan model instrument from 200 to 800 nm. The same sample solution was subjected to fluorescence emission measurement from 350 to 700 nm using Elico SL174 (India) instrument. The melting temperature (Tm) and the degradation temperature (Td) of the polymer samples were determined by using Dupont Thermal Analyst 2000 Differential Scanning Calorimeter 910S, USA model instrument (simultaneous DSC and TGA analyzer). All the measurements were done under N2 atmosphere in a temperature range of RT to 100 °C with 10 °C/min heating rate. Field emission scanning electron microscopy (FESEM) was used to examine the morphological behavior of the polymer with the help of FESEM – Hitachi S4800 Japan, instrument.(1)
where A1726 is corrected peak area of a peak at 1726 cm−1 and A730 is corrected peak area at 730 cm−1. A Waters 2690 GPC instrument was used to determine the Mw of the polymer samples using THF as an eluent at room temperature at the flow rate of 1 mL min−1 against polystyrene (PS) standards.
Drug release study
The ultimate aim of the present investigation is the drug release activity of PCL. The drug release study was carried out for the above synthesized materials and the procedure in brief is given below: 0.50 g PCL was taken in a disk form prepared under 7 tons of pressure. About 500 mL of gastric pH solution was prepared and the tablet suspended in the medium with stirring. Citation31 While stirring, the drug is slowly released and the same can be quantitatively measured at different intervals of time by pipetting 2 mL of aliquot by using UV–visible spectrophotometer. From the calibration curve, the % cumulative drug release (% CDR) was calculated as follows:(2)
Various possible models were dried and the best fit models are explained in the present investigation. The drug release models were done in a universal standard model.
Results and discussion
Characterization of Fe3O4/AR nanohybrid system
The FTIR spectrum of pure Fe3O4 is given in Figure a. The spectrum shows two peaks. A broad peak around 3466 cm−1 is due to the –OH stretching of Fe3O4. During the Fe3O4 synthesis some amount of water molecules are intercalated. The metal oxide stretching appeared at 603 cm−1.Citation35 Figure b represents the FTIR spectrum of AR decorated Fe3O4 nanohybrid. The –OH stretching appeared at 3444 cm−1. The aromatic symmetric and anti-symmetric stretching was observed at 2889 and 2959 cm−1, respectively. The bending vibration of –OH from AR appeared at 1637 cm−1. The SO2 stretching from AR appeared at 1216 cm−1. The aromatic C–H stretching was observed at 870 and 920 cm−1. The C–H out of plane bending vibration (OPBV) appeared at 719 cm−1. The metal oxide stretching also appeared at 632 and 444 cm−1. Hence, the appearance of new peaks such as –OH bending, SO2 stretching, aromatic CH stretching, and C–H OPBV confirms the surface functionalization of Fe3O4 by AR dyes.
Figure 1 FTIR spectrum of a Fe3O4 and b Fe3O4–AR nanohybrid, UV–visible spectrum of c AR dye and d Fe3O4–AR nanohybrid, Fluorescence emission spectrum of e AR dye and f Fe3O4–AR nanohybrid, VSM of g Fe3O4 and h Fe3O4–AR nanohybrid, FESEM image of i Fe3O4 and j Fe3O4–AR nanohybrid system
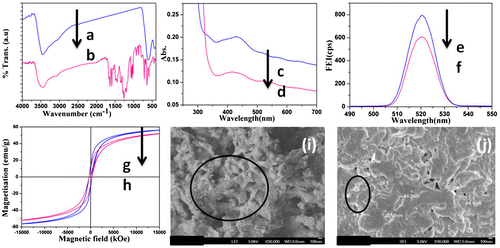
The UV–visible spectrum of AR before and after functionalization reaction confirms the interaction between Fe3O4 and AR dye. A broad peak at 418.7 nm (Figure c) is responsible for the AR dye. After the functionalization reaction the same peak was red shifted to 431.9 nm (Figure d). The red shift in the peak after the functionalization reaction confirms the existence of chemical interaction between dye and Fe3O4. The SO2 group of AR dye might interact with the Fe3O4 through the secondary forces of attraction. As a result, the peak was red shifted. The red shift in the peak after the nanohybrid formation was explained in our earlier communication.Citation34
The peculiar property of AR dye before and after surface functionalization reaction was tested by fluorescence spectroscopy. The fluorescence emission spectrum of pristine AR dye is shown in (Figure e) with the intensity of 806 cps. After the surface functionalization with Fe3O4 the FEI was suppressed to 606 cps (Figure f) without any shift in the peak. This confirms the quenching nature of Fe3O4.
The magnetic property of Fe3O4 was studied by VSM technique and is shown in Figure g with the magnetization value 55 emu/g. But after the surface functionalization reaction the VSM value of Fe3O4 was suppressed to 49.9 emu/g (Figure h). The decrease in VSM value confirms the existence of chemical interaction between the Fe3O4 and AR dye. In the case of AR dye, three types of functional groups such as –OH, –SO3Na, and C=O were observed and the –SO3Na group is found to be more active towards the metal oxide surface rather than the –OH and C=O groups. The –SO2 group of AR dye might interact with the metal surface through the weak secondary forces.Citation36
The surface morphology, size, and shape of Fe3O4 nanoparticles before and after surface functionalization reactions were analyzed by FESEM technique. Before the surface functionalization reaction, a nanospherical, and nanorod shape was observed (Figure i). The size of the sphere was calculated as 85 nm whereas the size of the nanorod was calculated as 300–500 nm. But after the surface functionalization reaction (Figure j), the nanorod shape of Fe3O4 disappeared and one can see a nanosphere-like morphology of Fe3O4 with the size of less than 50 nm. This indicates that after the surface functionalization reaction both the size and shape were altered. Due to the mild thermal force and mechanical force the size and shape of Fe3O4 were altered. Anbarasan et alCitation37 reported about the conversion of Au nanosphere into nanorod during the insitu polymerization N-isopropylacrylamide. In the present investigation, the observation is just reversed.
Characterization of PCL/Fe3O4–AR nanocomposite system
The surface catalytic effect of nanohybrid towards the ROP of CL was tested at different [M/I]. While increasing the [M/I] from 10–400 the corrected peak area of carbonyl stretching of PCL was increased. The FTIR spectrum of PCL synthesized at various [M/I] is given in Figure a–e. The important peaks are characterized below: a broad peak around 3500 cm−1 explains the –OH stretching of PCL. The aliphatic C–H symmetric and anti-symmetric stretching was noted at 2860 and 2942 cm−1, respectively. The C=O stretching of PCL was noted at 1717 cm−1.Citation34 The ester C–O–C linkage of PCL was identified at 1185 cm−1. The C–H OPBV could be seen at 729 cm−1. The M–O stretching was noted at 448 cm−1 as a small hump. From the FTIR spectrum it was found that, while increasing the [M/I], the ratio of FTIR-RI[C=O/C–H OPBV] was linearly increased. This confirms the ROP of CL by the nanohybrid. In order to find out the efficiency of the nanohybrid towards the ROP of CL a log–log plot was made between [M/I] and RI(C=O/CH) (Figure f). This plot shows two regions. It means initially the ROP of CL was very fast and later it was found to be slow. While increasing the [M/I] the number of initiating sites were reduced. As a result the rate of ROP of CL was lowered. At the same time the molecular weight of the polymer was increased. From the plot, the slope value was determined as 0.25 which confirmed the 0.25 order of ROP CL with respect to the nanohybrid. (i.e.) R(ROP) α [nanohybrid]0.25. This indicates that 0.25 mol of initiator is required to form 1 mol of PCL. The order of the reaction proved the initiating efficiency of nanohybrid towards the ROP of CL. The MWCNT-NIR dye system exhibited 0.50 order of reaction towards the ROP of CL.Citation38 When compared to the literature; the present system exhibited a good result.
Figure 2 FTIR spectrum of PCL/Fe3O4–AR nanocomposites synthesized at [M/I] of a 10, b 50, c 100, d 200, e 400 and f plot of log(RI[C=O/C–H]) vs. log[M/I]
![Figure 2 FTIR spectrum of PCL/Fe3O4–AR nanocomposites synthesized at [M/I] of a 10, b 50, c 100, d 200, e 400 and f plot of log(RI[C=O/C–H]) vs. log[M/I]](/cms/asset/fc4b2c46-5bf3-4805-91b2-218992affb64/ynan_a_1207009_f0002_oc.gif)
The chemical structure of PCL was confirmed by NMR spectroscopy. Figure a represents the 1H-NMR spectrum of PCL/Fe3O4–AR nanocomposite system. Peaks corresponding to standard TMS and solvent appeared at 0 and 7.3 ppm, respectively. A signal at 4.2 ppm is corresponding to the –OCH2 proton. This is in accordance with our earlier publication.Citation39 The –CO2–CH2 proton signal appeared at 2.4 ppm. The remaining methylene protons of PCL appeared between 1 and 2 ppm. The peak integration value confirmed the chemical structure and composition of PCL. Peaks corresponding to AR dye are not appeared due to non-solvation effect or lesser amount of AR dye used as an initiator (M/I = 100). The structure of PCL was further confirmed by 13C-NMR spectroscopy (Figure b). Solvent peak appeared at 78 ppm. A signal due to the carbonyl carbon appeared at 173 ppm.Citation28 Here, the aromatic and aliphatic carbonyl carbons are merged with each other and appeared as a single peak. Moreover, the quantity of AR taken was low (M/I = 100). The –OCH2 and –CO2–CH2 signals appeared at 62 and 34 ppm, respectively. The methylene carbon signals appeared between 20 and 30 ppm. Thus, the NMR spectra confirmed the chemical structure of PCL.
The UV–visible spectrum was recorded for PCL, synthesized at various [M] is shown in Figure a–e. The spectrum shows a peak at 446.4 nm. It was found that while increasing the [M], the absorbance at 446.4 nm was found to be reduced. This can be explained as follows: While increasing the [M] the number of monomers were increased at constant initiator concentration. The number of initiator shared by the monomer was reduced. As a result, the absorbance at 446.4 nm was reduced. Using the UV–visible spectroscopy one can find out the binding constant by plotting 1/[CL] vs. A0/A–A0. Figure f indicates the same with a linear plot. The binding constant was determined from the [I/S] as 0.28 × 105 M−1. The binding constant value again confirms the existence of strong interaction between AR dye and PCL units. In comparison with the literature,Citation34 we found that while changing the size and shape of the nanohybrid system, automatically the binding constant was changed.
Figure 4 UV–visible spectrum of PCL/Fe3O4–AR nanocomposites synthesized at [M] of a 0.50 g, b 1.5 g, c 2.0 g, d 2.5 g, e 3.0 g and f plot of 1/[CL] vs. A0/[A−A0]
![Figure 4 UV–visible spectrum of PCL/Fe3O4–AR nanocomposites synthesized at [M] of a 0.50 g, b 1.5 g, c 2.0 g, d 2.5 g, e 3.0 g and f plot of 1/[CL] vs. A0/[A−A0]](/cms/asset/a5aa8671-44aa-45dd-b175-b7e1bb7098c8/ynan_a_1207009_f0004_oc.gif)
While using a dye it is very important to find out the fluorescence property. Fluorescence emission spectrum was recorded for PCL samples synthesized at various [M]. It was found that while increasing [M], the FEI was decreased and the spectrum is shown in Figure a–e. This is due to the increase in [M], ultimately quenches the FEI of AR dye. In order to find out the number of binding sites involved in the ROP of CL and binding constant, the plot of log(weight of monomer) vs. log[I0−I/I] (Figure f) was made and the slope value was determined as 1.06 and the intercept value was noted as 2.64. The slope value is the binding constant, whereas intercept value indicates the number of binding sites. This is in accordance with our earlier publication.Citation34 In the case of AR dye; 2–OH and 1–SO3Na groups are available. During the ROP of CL, the –OH group was actively involved in the ROP of CL.Citation34 The –SO3Na group was binded with ferrite through the physical forces. This physical force is very weak and it is cleaved when it is heated to higher temperature. Now the –SO3Na group is attached with the PCL chain via secondary forces of attraction. The involvement of these 3 functional groups towards the ROP of CL was confirmed by FE spectroscopy.
Figure 5 Fluorescence emission spectrum of PCL/Fe3O4–AR nanocomposites synthesized at [M] of a 0.50 g, b 1.5 g, c 2.0 g, d 2.5 g, e 3.0 g and f plot of log(weight of monomer) vs. log[I0−I/I]
![Figure 5 Fluorescence emission spectrum of PCL/Fe3O4–AR nanocomposites synthesized at [M] of a 0.50 g, b 1.5 g, c 2.0 g, d 2.5 g, e 3.0 g and f plot of log(weight of monomer) vs. log[I0−I/I]](/cms/asset/8f369378-0eb4-454e-9381-04e957f1f2e2/ynan_a_1207009_f0005_oc.gif)
The phase transition of PCL during heating was analyzed by DSC. Figure a–e indicates the DSC thermogram of PCL synthesized at various [M/I]. While increasing the [M/I], the Tm of PCL is also proportionally increased. The Tm value was varied between 60.6 and 63.9 °C. The increase in Tm is due to the increase in molecular weight of PCL.Citation34 Another one important point noted here is while increasing [M/I] the area of the melting peak is also increased. This confirms the increase in molecular weight of PCL while increasing the [M/I] variation. The molecular weight of PCL is discussed in the forth coming session. The thermal stability of PCL/Fe3O4–AR nanocomposite systems was studied by TGA at the heating rate of 10 °C/min under nitrogen atmosphere (Figure f–j). Generally, the thermogram exhibited a two-step degradation process. The first minor weight loss below 200 °C is associated with the removal of moisture and cleavage of dye units from the PCL backbone. The second major weight loss around 310 °C is ascribed to the degradation of PCL backbone. The interesting point noted here is after the major degradation, the % weight residue remained above 400 °C is increased with the decrease of [M/I] ratio (i.e.) increase of Fe3O4–AR nanohybrid concentration. This confirmed that the nanohybrid was effectively initiated the ROP of CL under nitrogen atmosphere at 160 °C. Thus, both DSC and TGA explained the thermal properties of the PCL/Fe3O4–AR nanocomposite.
Figure 6 DSC thermogram a–e and TGA thermogram f–j of PCL/Fe3O4–AR nanocomposites synthesized at [M/I] of a f 10, b,g 50, c,h 100, d,i 200 and e,j 400
![Figure 6 DSC thermogram a–e and TGA thermogram f–j of PCL/Fe3O4–AR nanocomposites synthesized at [M/I] of a f 10, b,g 50, c,h 100, d,i 200 and e,j 400](/cms/asset/27b2883c-454a-4023-b1d0-b6d81fe32e95/ynan_a_1207009_f0006_oc.gif)
In order to confirm the ROP of CL in the presence of Fe3O4–AR nanohybrid system the GPC analysis was used. Figure a and b indicates the GPC images of PCL synthesized at the [M/I] of 10 and 400, respectively. When the [M/I] was maintained at 10, the Mw, Mn, and polydispersity (PD) values were determined as 7535 g/mol, 3717 and 2.02, respectively. The PCL synthesized at the [M/I] value of 400, the Mw, Mn, and PD values were determined as 16412 g/mol, 9067 and 1.81, respectively. This indicates that while increasing the [M/I] ratio, the Mw of the PCL was increased. This is due to the decrease in initiator concentration (i.e.) the number of initiating centers were reduced. The PD inferred that at higher [M/I] ratio, the polymer yielded a narrow molecular weight without any branching or cross linking. Hence, the GPC results proved that the Fe3O4–AR nanohybrid is an effective initiator towards the ROP of CL. This is in accordance with the literature report.Citation34
Figure 7 GPC traces of PCL/Fe3O4–AR nanocomposite systems synthesized at a [M/I] = 10 and b [M/I] = 400
![Figure 7 GPC traces of PCL/Fe3O4–AR nanocomposite systems synthesized at a [M/I] = 10 and b [M/I] = 400](/cms/asset/dcb65011-f120-420e-a9bd-18e713f0c382/ynan_a_1207009_f0007_b.gif)
Figure shows the VSM loop of PCL/Fe3O4–AR nanocomposite system synthesized at [M/I] = 100. The VSM value was determined as 24.57emu/g. The VSM value of pristine Fe3O4 and surface-functionalized Fe3O4 were greater than that of the present system. The decrease in VSM value is due to the encapsulation of Fe3O4 by PCL chains. In our earlier publication.Citation34 we explained the decrease in VSM after the insitu polymerization by four factors. The same four factors are suitable for the present investigation too.
Figure a shows the FESEM image of PCL/Fe3O4–AR nanocomposite synthesized at [M/I] = 100. The broken stone like morphology confirms the presence of PCL. The nanosized Fe3O4 was dispersed on the PCL backbone. The size of Fe3O4 nanoparticles was determined as approximately 25 nm. This is definitely smaller than the size of pristine Fe3O4 and nanohybrid system. The decrease in particle size in the present system is associated with the effective utilization of high thermal energy. Hence, this shows that thermal treatment is one of the ways to produce nanosized materials. The HRTEM image of PCL/Fe3O4–AR nanocomposite system is given in Figure b. The image indicates the presence of both agglomerated and non-agglomerated Fe3O4 nanoparticles. The size of Fe3O4 is varied between 10 and 40 nm. The darkened area confirmed the agglomerated Fe3O4 nanoparticle. Thus, the HRTEM image confirmed the dispersion of Fe3O4 nanoparticle on the surface of PCL.Citation34
Figure shows the size of Fe3O4 nanoparticles, I indicate the size of pristine Fe3O4, II indicates the size of Fe3O4 in nanohybrid and III indicates the size of Fe3O4 in PCL/Fe3O4–AR nanocomposite system. The nanocomposite preparation not only reduced the size but also controlled the shape of Fe3O4 nanoparticles.Citation37
The drug release activity of PCL nanocomposite was studied in the presence of Rif. as a model drug. The drug release study was carried out at gastric pH and the same was confirmed by UV–visible spectroscopy. It was found that while increasing the time the λmax value at 262.7 nm was increased (Figure a–h). Various drug release models like zero-order reaction, first-order reaction, Higuchi model, Korsmeyer and Peppas model and Hixson model were tried among which the first-order reaction exhibited the maximum R2 value of 0.91. Figure i indicates the plot of log (%CDR retained) vs. Time. This indicates that the drug was slowly released into the medium via dissolution process in the gastric pH condition. The drug release mechanism was determined by using Korsmeyer and Peppas model (Figure j). From the plot, the slope value was calculated as 0.492 which is less than n (i.e.) 0.89. This confirms the non-Fickian transport mechanism. In our previous communication, we reported that the folic acid conjugated PCL indicated the Fickian-type mechanism,Citation38 but in the present system, the drug is not chemically conjugated with PCL.
Figure 11 a–h UV–visible spectrum of Rif. released at different interval of time and i plot of (Time) vs. (log(% CDR retained) and i plot of log(Time) vs. log(%CDR)
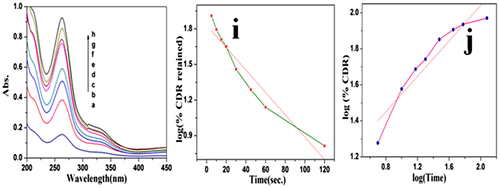
For the sake of comparison, the drug release mechanism of PCL nanocomposite was carried out by considering AR dye as a model drug. Here, also various drug models were tried. The UV–visible spectrum was recorded for different interval of time (Figure a–l). Among the models, the Korsmeyer and Peppas model exhibited the maximum R2 value of 0.97. Figure m indicates the plot of log (%CDR) vs. log (Time). The slope value of the Korsmeyer and Peppas model was determined as 0.29, which is less than 0.5. Again this confirms the non-Fickian transport mechanism of drug release. This study indicates that the drug release followed the non-Fickian transport mechanism while using PCL as a drug carrier and the drug is chemically conjugated or not.
Conclusions
From the above kinetic study the niche points are presented here as conclusion. The initiating capability of nanohybrid was tested by FTIR-RI method and further co-supported by 0.25 order of reaction with respect to [M/I]. The binding constant was determined from UV–visible spectroscopy as 0.28 × 105 M−1. The number of binding sites was determined as 2.64 from FE spectroscopy. The Tm value of PCL was increased with the increase of [M/I]. The VSM value was found to be reduced after the nanohybrid and nanocomposite formation through the surface functionalization and encapsulation reactions, respectively. The size of Fe3O4 nanoparticle was also reduced after the nanohybrid and nanocomposite formation. The drug release followed the non-Fickian transport mechanism. The present investigation inferred that one can reduce the size of the material via thermal and mechanical treatment. In overall comparison, the present investigation yielded somewhat better results when compared with the literature particularly with the GPC results.
Disclosure statement
No potential conflict of interest was reported by the authors.
Acknowledgment
Mrs. G. Vijaylakshmi, Assistant Professor, Department of English is gratefully acknowledged for her valuable help during this manuscript preparation work.
References
- B. J. Ireland, C. A. Wheaton and P. G. Hayes: ‘Cationic organo magnesium complexes as highly active initiators for the ring opening polymerization of ε-caprolactone’, Organometallics, 2010, 29, 1079–1084.10.1021/om900669y
- M. L. Gou, Z. Y. Qian, H. Wang, Y. B. Tang and M. J. Huang: ‘Preparation and characterization of magnetic poly (ε-caprolactone)-poly (ethyleneglycol) -poly (ε-caprolactone) microspheres’, J. Mater. Sci. Mater. Med., 2008, 19, 1033–1041.10.1007/s10856-007-3230-3
- X. Deliang and J. Bian: ‘Study on the ring opening polymerization of ε-caprolactone initiated by hydroxyl acids’, Acta Polym. Sinica., 2000, 5, 532–537.
- H. Liu, Z. Shen, S. E. Stiriba and Y. Chen: ‘Coreshell type multiarm star PEI-b-PCL: synthesis and guest encapsulation potential’, J. Polym. Sci. Part A Polym. Chem., 2006, 44, 4165–4173.10.1002/(ISSN)1099-0518
- R. S. Lee, W. H. Chen and J. H. Lin: ‘Polymer grafted MWCNT through surface initiated ring opening polymerization and click reaction’, Polymer, 2001, 52, 2180–2188.
- A. Sowkath, A. Mansur and R. Anbarasan: ‘Synthesis, characterization and application of a novel Schiff base’, J. Chem. Bio. Phy. Sci. Sec A., 2014, 4, 1930–1940.
- B. Meenarathi, S. Palanikumar, L. Kannammal and R. Anbarasan: ‘Synthesis and characterizations of Fe3O4-acidfuchsin tagged poly (ε-caprolactone) nanocomposites’, Mater. Res. Exp., 2014, 1, 1–16.
- L. Kannammal, S. Palanikumar, B. Meenarathi, A. Yelilarasi and R. Anbarasan: ‘Synthesis, characterization and band gap energy of poly(ε-caprolactone)/Sr-MSA nanocomposite’, J. Phys. D: Appl. Phys., 2014, 47, 1–10.
- K. Agathian, P. Siva, B. Meenarathi and R. Anbarasan: ‘Luminol intiated ring opening polymerization of ε-caprolactone’, Ind. J. Sci., 2013, 5, 37–40.
- B. Meenarathi, H. H. Chen, P. H. Chen and R. Anbarasan: ‘Near infrared dye functionalized MWCNT as an effective initiator for the ring opening polymerization of ε-caprolactone’, J. Polym. Res., 2013, 20, 118–130.10.1007/s10965-013-0118-4
- B. Wu, R. W. Lenz and B. Hazer: ‘Synthesis, characterization and drug delivery activity of poly (anthranilicacid) based triblock copolymer’, Macromolecules, 1999, 32, 6856–6859.10.1021/ma990166o
- B. A. Rozenberg: ‘Kinetics and mechanism of ε-caprolactone anionic polymerization under the influence of amines’, Pure Appl. Chem., 1981, 53, 1715–1727.
- V. T. Lipik and M. J. M. Asadie: ‘Process optimization of poly(ε-caprolactone) synthesizing ring opening polymerization’, Iran. Polym. J., 2010, 19, 885–893.
- G. Wu, J. Liu, W. Sun and Z. Shen: ‘Ring opening polymerization of ε-caprolactone by a new yuttium complex’, Polym. Int., 2010, 59, 431–436.10.1002/pi.v59:4
- A. Kiersnowski, P. Dąbrowski, H. Budde and J. Kressler: ‘Synthesis and structure of poly(caprolactone)/synthetic montmorillonite nanointercalates’, Eur. Polym. J., 2004, 40, 2591–2598.10.1016/j.eurpolymj.2004.06.014
- T. F. Al-Azemi, L. Kondaveti and K. S. Bisht: ‘Solventless enantioselective ring opening polymerization of substituted ε-caprolactone by enzymatic catalysis’, Macromolecules, 2002, 35, 3380–3386.10.1021/ma012016v
- G. Carrot, J. G. Hilborn and M. Trollsås: ‘Two general methods for the synthesis of thiol functionalized poly (ε-caprolactone)’, Macromolecules, 1999, 32, 5264–5269.10.1021/ma990198b
- I. Lin, C. G. Cheng, Y. -C. Yen and F. C. Chang: ‘Synthesis and assembly behavior of heteronucleobase-functionalized of poly (ε-caprolactone)’, Macromolecules, 2010, 43, 1245–1252.10.1021/ma9026614
- M. Oshimura and A. Takasu: ‘Controlled ring opening polymerization of ε-caprolactone by rare earth perfluoroalkanesulfonates and perfluoroalkanesulfonimides’, Macromolecules, 2010, 43, 2283–2290.10.1021/ma902557t
- Y. C. Wang, X. Q. Liu, T. M. Sun, M. H. Xiong and J. Wang: ‘Functionalized micelles from block co polymer of polyphosphoester and poly(ε-caprolactone) for receptor mediated drug delivery’, J. Contr. Rel., 2008, 128, 32–40.10.1016/j.jconrel.2008.01.021
- M. J. Kim, D. H. Jang and Y. H. Choa: ‘Preparation and characterization of carboxyl functionalization of magnetic nanoparticles for oligo nucleotide immobilization’, Phys. Ser., 2010, 139, 1–4.
- H. Lida, K. Takayanagi, T. Nakanishi and T. Osaka: ‘Synthesis of Fe3O4 nanoparticles with various sizes and magnetic properties by controlled hydrolysis’, J. Colloid Inter. Sci., 2007, 314, 274–280.
- S. Ahmad, U. Riaz, A. Kaushik and J. Alam: ‘Soft template synthesis of superparamagnetic Fe3O4 nanoparticles a novel technique’, J. Inorg. Organomet. Polym., 2009, 19, 355–360.10.1007/s10904-009-9276-6
- S. Palanikumar, L. Kannammal, B. Meenarathi and R. Anbarasan: ‘Effect of folic acid functionalized magnetic fluorescent nanoparticles on the sedimentation of starch molecules’, Int. Nano Lett., 2014, 4, 104–113.10.1007/s40089-014-0104-9
- Z. Li, H. Chen, H. Bao and M. Gao: ‘One pot reaction to synthesize water soluble magnetic nanocrystals’, Chem. Mater., 2004, 16, 1391–1393.10.1021/cm035346y
- A. Kayrak, R. C. Foitzik and M. Pfeffer: ‘Fluorescence and conductivity studies on wool’, Mater. Chem. Phys., 2009, 113, 480–484.
- V. Kohila, M. G. Sribala, L. Kannammal, B. Meenarathi, T. Dhanalakshmi and R. Anbarasan: ‘Synthesis and spectral characterization of poly(congored) grafted silk fibre: A kinetic Approach’, Ind. J. Fibre. Text. Res., 2014, 9, 172–179.
- A. Khazaoi, S. Saedina, M. K. Borazjgani and F. Abbasi: ‘Synthesis and characterization of novel polymer drug conjugates based on the poly(Styrene-alt-maleic anhydride) as a potential method for drug release’, Acta Chim. Slov., 2013, 60, 724–731.
- J. Sultana, M. S. Hossaih, S. M. A. Islam and M. S. Islam: ‘Synthesis and characterizations of Fe3O4-acidfuchsin tagged poly (ε- caprolactone) nanocomposites’, Ind. J. Novel Drug Del., 2012, 4, 145–150.
- H. J. Liu, H. C. Chu, L. H. Lin and S. Y. Hsu: ‘Effect of MWCNT and Au nanoparticle on the structure-property relationship of poly (N-isopropylacrylamide)’, Int. J. Polym. Mater. Polym. Biomater., 2015, 64, 7–14.10.1080/00914037.2014.886238
- A. C. Salome, C. O. Godswil and I. O. Ikecha: ‘Kinetics and mechanisms of drug release from swellable and non-swellable matrices: a review’, Res. J. Pharm. Biol. Chem. Sci., 2013, 4, 97–103.
- M. Olukman, O. Şanlı and E. K. Solak: ‘Release of anticancer drug 5-fluorouracil form different ionically cross linked alginate beads’, J. Biomater. Nanobiotechnol., 2012, 3, 469–479.10.4236/jbnb.2012.34048
- S. Palanikumar, B. Meenarathi, L. Kannammal and R. Anbarasan: ‘Effect of Fe3O4 on the sedimentation and structure-property relationship of starch under different pHs’, Int. J. Biol. Macromol., 2014, 67, 91–98.10.1016/j.ijbiomac.2014.03.012
- B. Meenarathi, S. Palanikumar, L. Kannammal and R. Anbarasan: ‘Synthesis, characterization and catalytic activity of Ag-acidfuchsin nanohybrid system towards the ring opening polymerization of ɛcaprolactone’, Spectrochim. Acta Part A, 2015, 135, 93–100.10.1016/j.saa.2014.06.136
- R. Rahimi, A. Tadjarodi, M. Imani, M. Rabbani, S. Safalou and H. Kerdari: ‘Synthesis of tetrakis(carboxyphenyl)porphyrin coated paramagnetic iron oxide nanoparticles via amino acid for photodegradation of methylene blue’, Turk. J. Chem., 2013, 37, 879–888.10.3906/kim-1204-19
- A. M. Demin, V. P. Krasnov and V. N. Charushin: ‘Covalent surface modification of Fe3O4 magnetic nanoparticles with alkoxy silanes and amino acids’, Mendaleev Commun., 2013, 23, 14–16.10.1016/j.mencom.2013.01.004
- R. Anbarasan and C. A. Peng: ‘Effect of MWCNT and Au nanoparticle on the structure-property relationship of poly (N-isopropylacrylamide)’, J. Appl. Polym. Sci., 2012, 124, 3996–4006.10.1002/app.v124.5
- S. Kailash, B. Meenarathi, L. Kannammal, S. Palanikumar and R. Anbarasan: ‘Synthesis, characterization, drug delivery, and splinting activity of folic acid bridged poly(ε-caprolactone-co-tetrahydrofuran)’, Int. J. Polym. Mater. Polym. Biomater., 2015, 64, 620–627.10.1080/00914037.2014.996711
- B. Meenarathi, H. H. Chen, P. H. Chen and R. Anbarasan: ‘Synthesis and characterization of fluorescent bio-degradable poly(ε-caprolactone)’, Int. J. Plast. Technol., 2014, 18, 135–145.10.1007/s12588-014-9070-3