Abstract
In recent years, polymer nanocomposites (PNCs) have attracted the attention of scientists and technologists in water purification due to improved processability, surface area, stability, tunable properties, and cost effectiveness. PNCs showed fast decontamination ability with high selectivity to remove various pollutants. This review provides up-to-date information about the importance of PNCs in the removal of metal ions, dyes, and microorganism from polluted water. The general methodology for preparation and properties of nanocomposites with reference to PNCs is given. Different adsorption isotherm and kinetic models along with thermodynamic parameters for adsorption have been discussed.
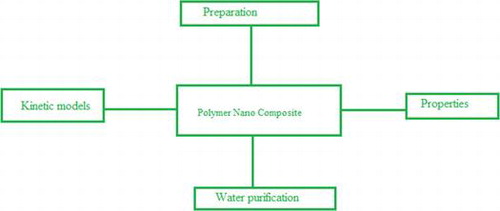
Introduction
Water is a precious natural resource and its quality availability is essential for the survival of living creatures on the earth. However, rapid industrialization is continuously degrading the quality of water due to addition of large amounts of pollutants into the water bodies.Citation1,2 Water pollutants have appeared as threats to entire biosphere, so their removal has become essential. The different types of water pollutants along with their sources and impact are given in Table .
Table 1 Different water pollutants, examples, sources and their effects
According to recent UN report,Citation3,4 reliable access of clean and affordable water is one of the most basic humanitarian goals, and is a major global challenge for the 21st century. The fundamental requirements for water purification is appropriate materials with high separation capacity, low cost, porosity, and reusability.Citation5,6 In this regard, nanotechnology provides, an opportunity to develop advanced materials for effective water purification by optimizing their properties like hydrophillicity, hydrophobicity, porosity, mechanical strength, and dispersibility.Citation7 Nano particles having high surface area, can contribute a lot in water purification but its agglomeration restricts its use.Citation8 However, agglomeration can be minimized by converting nanomaterials to nanocomposites. In this review, different types of nanocomposites, their preparation and properties have been discussed. Emphasis has been given on polymer nanocomposite and their use for water purification.
Nanocomposite
Nanocomposites are multi-phasic materials, in which at least one of the phases shows dimensions in the nano range (10–100 nm). Now-a-days nanocomposite materials have emerged as suitable alternatives to overcome limitations of different engineering materials. They are reported to be the materials of 21st century. Nanocomposite materials can be classified, according to their dispersed matrix and dispersed phase materials.Citation9 The classification of nanocomposite is illustrated in Figure .
Among different nanocomposites, polymer-based nanocomposite (PNCs) have become a prominent area of current research and development. PNCs have lot of advantageous properties such as film forming ability, dimensional variability, and activated functionalities.Citation10,11 The importance of materials can be recognized from the continuous increasing rate of publications in leading journals, which is presented in Figure .
Figure 2 Publication trends in polymer nanocomposite (source scifinder on 22 June 2016 with search term polymer nanocomposite for water purification)
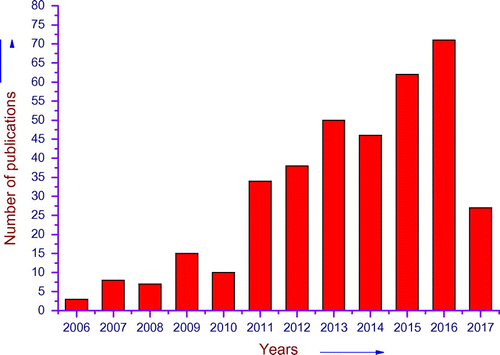
Preparative methods
Number of methods has been used for the preparation of polymer nanocomposites. A brief summary of different methods and their comparison for the preparation of nanocomposites are given in Table . However, the designing of correct preparation technique is very critical to obtain nanocomposite with desirable properties.
Table 2 Different methods used for preparation of nanocomposites
The PNC prepared from inorganic materials (metals and metal oxide nano-particles) using in situ polymerization and composite formation shows excellent sorbent properties and are also suitable as catalysts, sensors, reducing agents, and bactericides. Shukla et al.Citation23 has synthesized zinc oxide polyaniline nanocomposite with improved interface. The resulting hybrid nanocomposites exhibited thousand times better electrical conductivity due to synergistic effects. The integration of dispersed phase into a dispersed medium was done by both approaches (ex-situ or in-situ). Many times nano particles were also functionalized prior to dispersion in the matrix. The basic objective was to improve the compatibility, ion exchange, and surface interaction. Luo et al.Citation24 prepared ionic liquid coated Fe3O4@chitosan@graphene oxide (GOIL-Fe3O4@CS@GO) by dissolving one gram of tetraoctylammoniumbromide in 15 mL methanol. Further, 1.0 g of Fe3O4@CS@GO was taken in a round bottom flask, dissolved in ionic liquids and sonicated for 2 h with a 15 min intermittent time interval. The resulting solution was filtered and washed with methanol. Thus, obtained ionic liquid impregnated Fe3O4@CS@GO was found suitable for dye removal. The maximum adsorption capacity for methylene blue was 262 mg/g. The preparation and application of Ionic liquid-Fe3O4@CS@GO for removal of MB is illustrated in Scheme . [Citation24]
A semiconductor–conductor tubular nanocomposite in a 60 mm thick alumina template membrane with 200 nm diameter pores were prepared. TiO2 tubules were synthesized within the pores of the alumina membrane by sol–gel process before they were subjected to thermal treatment. Polypyrrole wires were then grown inside the semiconductor tubules by using chemical polymerization method. The conducting polymer enhanced the electrical conductivity of the material, and increased the photo-efficiency of TiO2-polypyrrole nanocomposites as a photocatalyst.Citation25
Characterization of nanocomposite
The important techniques used for the characterizations of PNCs are thermal analysis (TGA, DTA, DSC, TMA, and DMA), microscopic techniques (SEM, TEM, and AFM), spectroscopic techniques (FT–IR, Raman) and X-ray diffraction techniques. The presence of polymer matrix improves the processibility of a non-polymeric constituent and the thermal stability of polymer is also improved significantly. Thermal analytical techniques have frequently been used for this purpose.Citation26
Scanning electron microscopic methods provide images of surface, which are associated with sample properties like homogeneity, roughness, porosity, etc. The images are also used to get information about compatibility and lattice mismatch. The other microscopic techniques are scanning probe microscopy (SPM) and scanning tunneling microscopy (STM). They are indispensable in characterizing PNCs.Citation27 The AFM uses a sharp tip to scan across the sample and appropriate to evaluate roughness of morphology.Citation28,29 The transmission electron microscopy (TEM) allows qualitative understanding about the internal structure, spatial distribution of the various phases, and views of the defective structure through direct visualization of PNCs, in some cases of individual atoms.Citation30 Another important tool is wide angle X-ray diffraction pattern, which provides the crystallinity and latice structure of nanocomposite. Small-angle X-ray scattering (SAXS) is typically used to observe structures of the order of 10 Å or larger. The wide angle X-ray diffraction (WAXD) patterns and corresponding TEM images of three different types of nanocomposites are presented in Figure .Citation31 A closer observation of the micrograph at higher magnification revealed that each dark line often corresponds to several clay layers.
Figure 3 WAXD patterns and TEM images of three different types of nanocomposites (Intercalated, Intercalated and Flocculated, Exfoliated)
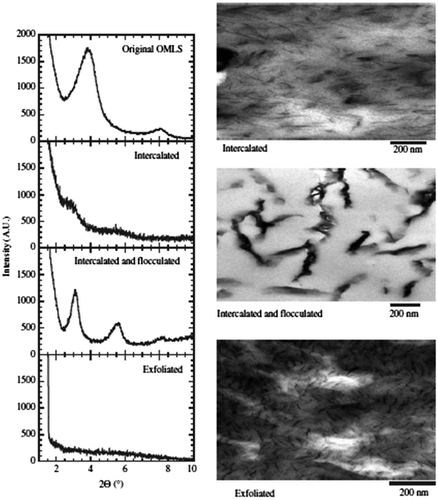
PNCs exhibit newer physical and chemical properties than the individual constituents. It depends on interactions between dispersed phase and matrix, here polymers are considered to be a good host material due to processibility.Citation32 FT–IR and Raman spectra are widely used to investigate these types of interactions.Citation33 Figure clearly indicates that as the concentration of Ag in Ag/PMMA nanocomposites increases, the peak shifted to lower wave numbers indicating strong interactions between the constituents.
Figure 4 FTIR spectra of Ag/PMMA nanocomposites at various concentrations at 120 °C (a) ~6% (b) ~8% (c) ~10%Citation34
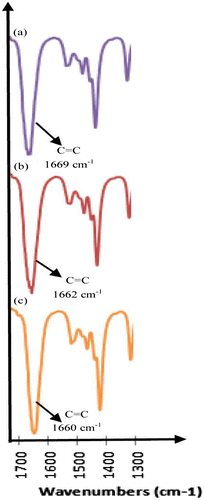
Properties
Addition of nanoparticles in polymer matrix improves the polymer properties and produce PNCs with desired properties. Catalytic, adsorption, and mechanical properties of PNCs are generally used for the purification of water. These properties of PNCs are briefly discussed below.
Catalyst
Large number of metal, metal oxide, and sulfides have been used as catalysts for purification of water and waste water both in the presence and absence of light.Citation35 Number of catalytic degradation of different pollutants like nitrogen contain compounds, dyes, and residual organic compounds have been reported.Citation36,37 Some catalysts also produce reactive oxygen species (ROS), which enhances the catalytic rate. Heterogeneous catalysis among different metal compounds like TiO2, ZnO, Fe2O3, CdS, GaP, and ZnS are reported for water decontamination due to their ionic surface interaction and modified surface tension.Citation38 Among different catalysts, titanium dioxide and zinc oxide (ZnO) have received the greatest importance due to their low cost, high photo catalytic activity, and stability.Citation39,40 They have been used as a photocatalyst for speeding up many redox reactions on their surface. An example of photocatalytic activity of ZnO/PMMA nanocomposite has been discussed by Mauro et al.Citation41 for degradation of phenol and methylene blue. The result illustrated in Figure indicates drastic enhancement in catalytic properties due to incorporation of ZnO in PMMA.
Figure 5 Photolytic degradation methylene blue over ZnO/PMMA composites as a function of the irradiation timeCitation41
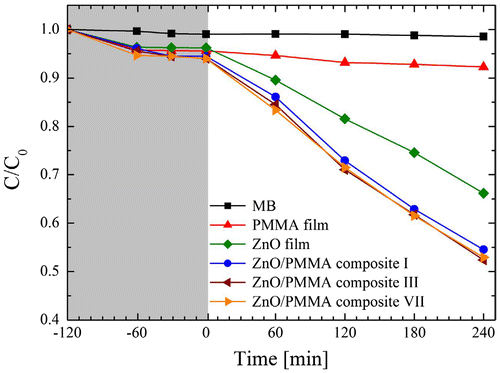
Generally, on irradiation with UV light, these oxides generated holes and released electrons, which reacted with H2O and O2 molecules, and adhered on the surface. In result, it produces highly reactive oxygen species (ROS) such as peroxides, superoxide, hydroxyl radicals, and singlet oxygen. ROS are capable to degrade organic water pollutants like dyes efficiently.Citation42 Generation of ROS also has antibacterial effect due to oxydative stress in an aqueous condition, for example, the hydroxyl radical is responsible for inactivation of Escherichia coli.Citation43 However, there are limitations because of the effects on human health and ecosystems due to their release, stability, dissolution, and retention time. In this regards PNCs have attracted maximum attention. Titania/PMMA nanocomposite has been prepared by electrochemical anodization. The nanocomposite has been tested for inactivation of Escherichia coli as a model organism. Sankar et al.Citation44 studied antimicrobial effect of silver embedded aluminum oxyhydroxide–chitosan nanocomposite. This composite is capable to control sustained release of silver ions (40 ± 10 ppb) in natural drinking water for an extended volume of water passing through it due the formation of abundant –O and –OH functional groups on chitosan surface. A new class of photocatalyst is g-C3N4 which increase the photocatalytic activity of semiconductor.Citation45 Beside this g-C3N4 has large surface area to adsorb large variety of synthetic organic pollutant and toxic ions.Citation46–48 Therefore, carbon nano sheet (CNS) nanocomposites with multiadaptable features is a good technique to increase the application of semiconductor nanomaterials in the area of photocatalyst. Many semiconductor photocatalysts such as BiOCl,Citation49 BiVO4,Citation50 Bi2MoO6,Citation51 Bi2WO6,Citation52 In2S3,Citation53 CuO2,Citation54 SrTiO3,Citation55 TiO2,Citation56 WO3,Citation57 ZnO,Citation58 and Ag/AgBrCitation59 have been used to couple with g-C3N4 for the synthesis of CNS nanocomposite.Citation60 TiO2 has been widely used for waste treatment, water splitting, air purification, and self cleaning of surfaces because of its unique photocatalutic property. TiO2 has also been incorporated in various membrane matrixes to provide photocatalytic activities.Citation61,62 Rahimpour et al.Citation63 studied the effect of UV radiation on the performance of TiO2/PES nanocomposite membrane and found that UV irradiated TiO2/PES membrane had higher flux and enhanced fouling resistance when compared the system without UV radiation. They attributed this enhancement to the photocatalysis of TiO2 under UV radiation. Damodar et al.Citation64 obtained a superior membrane with enhanced permeability and antibacterial activity.
Adsorption behavior
Polymer nanocomposites are known for highly tunable adsorption behavior due to the presence of nano particles with high surface area in the polymer matrix. Optimized adsorption behavior of nanocomposites make them suitable for different technical applications like chemical sensor, water purification, drug delivery, and fuel cell technology. PNCs have extensively been used for adsorptive removal of various toxic metal ions, dyes, and microorganism from water/waste water. Khare et al.Citation65 has prepared chitosan, carbon nanofibers (CNFs)-supported iron (Fe)-oxide nanoparticles (NPs) and polyvinyl alcohol nanocomposite film with improved adsorption capacity. The materials show a high metal uptake (80 mg per g of chitosan/Fe-CNF composite), and explored for efficient removal Cr(VI) from water under dynamic conditions (Figure ).
Figure 6 Shematic illustration for chromium adsorption on Chitosan/Fe-Carbon nanofibers and polyvinyl alcohol nanocompositeCitation65
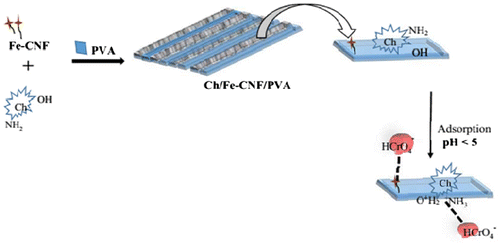
However, in the course of adsorption many secondary pollutants are also generated. Thus, in order to overcome the problem of secondary pollutants, PNCs have been used as adsorbents for water purifications. A series of binary, tertiary, and even quaternary PNCs have been made to develop appropriate level of adsorption behavior. Mittal et al.Citation66 have reported that addition of nano SiO2 in acrylamide hydrogel improves the monolayer adsorption capacity of acrylamide hydrogel to 1408.67 mg g−1. They prepared nano silica and gum karaya grafted with poly (acrylic acid acrylamide) (GK-cl-P(AA-co-AAM) nanocomposite containing hydrogel for adsorptive removal of methylene blue (MB) from aqueous solutions. Their findings indicated that, grafted copolymerized gum karaya hydrgel could be used as an eco friendly and efficient adsorbent for the removal of methylene blue dye from industrial wastewater (Figure ). However, the complete separation of the adsorbent particles from the treated water still remains a challenge in batch mode of purification.
Figure 7 Illustration of MB removal by gum karaya grafted with poly(acrylic acid acrylamide) nanocomposite hydrogelCitation66
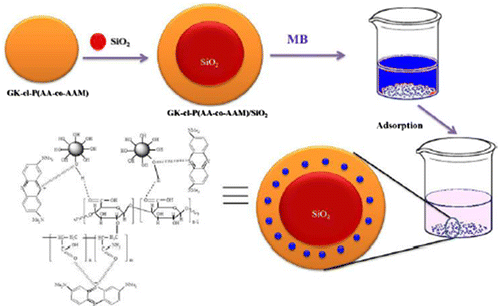
Another important strategy to optimize adsorption behavior is to synchronize hydrophobic and hydrophilic behavior in composite matrix. A novel hydrophilic–hydrophobic optimized magnetic interpenetrating polymer networks (IPNs) of poly (methyl acryloyldiethylenetriamine)/polydivinylbenzene (PMADETA/PDVB) was synthesized by interpenetration of polydivinylbenzene (PDVB) networks in the pores of the magnetic poly (glycidyl methacrylate) (PGMA) networks. Initially, PGMA networks were transformed to poly (methyl acryloyl diethylenetriamine) (PMADETA) networks by an amination reaction.Citation67 The adsorption of a molecule depends on ionic or surface interaction, which needs selective interaction site. PNCs are most appropriate substrate for this purpose. Poly (acrylamide–styrene sodium sulfonate) containing titanium oxide [TiO2/(P(AAm–SSS)] obtained by in-situ intercalative polymerization of co-poly acrylamide (PAAm) and styrene sodium sulfonate (SSS) in the presence of TiO2 nanoparticles were prepared, which showed improved metal ions (Cs+, Co2+, and Eu3+) adsorption with sorption capacity upto 120, 100.9 and 85.7 mg/g, respectively. Another important aspect about the use of PNCs in water purification is biodegradation. In this regard, biopolymers like polysacchride has enormous potentials due to its world wide availability. Sun et al.Citation68 has prepared biodegradable polysaccharide-based nanocomposite adsorbents. Wheat xylan/poly(acrylic acid) nanocomposite hydrogel containing Fe3O4 nanoparticles has been reported for 90% removal of MB by adsorption. The interpenetrating nature and super magnetic behavior of hydrogel is further responsible for better adsorption. The materials are having environmental importance and widely covered in green chemistry and also referred as green nanocomposites.
Mechanical properties
Processability, stability, and end use of a PNCs depend on its mechanical strength. The interaction among polymer and non-polymeric constituents strongly influences this property. The interaction between constituents particles of composite not only influences mechanical behavior of the neat resin but also increases the value-added properties, which were not present in the pure polymer. Another important characteristics of nanocomposite is anisotropy, which aligns the mechanical properties in composites. In this regards, carbon-based composite have played significant role in the development of PNC.Citation69 The dispersion of carbon nanotube in PMMA matrix increases the mechanical properties of PMMA.Citation70 The results have indicated that the improvement depends on surface interaction between carbon nanotube and polymer chain. The young modulus of PMMA changes from 2.86 to 3.99 GPa; yield stress from 2.86 to 35.06 MPa due to addition of CNT in PMMA matrix. Carbon nanotubes (CNTs) have also attracted intense attention of scientists working in various disciplines due to its exceptional electrical, mechanical, and thermal properties. These properties have made CNTs as outstanding materials for a range of technical applications including water purifications.Citation71 The ultra-high mechanical properties like young’s modulus and tensile strength of nanotubes in polymer-matrix composites are promising.Citation72 The PNCs with optimized porosity have capability of mass transfer, liquid retention, and lighter weight. The porous materials have applications in water purification but limitation of porous materials are low mechanical strength and stiffness. Arash et al. introduced CNTs in PMMA matrix to improve the mechanical properties.Citation73 Balasubramaniam et al.Citation74 has prepared three sets of MWCNT/PMMA nanocomposite thin films with various weight percentage of MWCNT using solvent casting method employing dichloromethane as a solvent. The result revealed that addition of 10 weight percent of multi-wall carbon nanotube yielded a considerable increase in mechanical properties and porosity. Generally, incorporation of CNT brings improvement in porosity and relative affinity of solvent and solute. This synchronization brings better selectivity and rejectivity of ions present in water. These type of nanocomposites are used for adsorptive separations. The current findings indicated that the property profiles of nanocomposite are unexpected. The crystallization rate and degree of crystallinity influenced the crystal confined spaces. The size of spherulitic growth can be confined if the primary nuclei are not present during crystallization and homogeneous nucleation proceed. This have subsequent effects on the properties of nanocomposites. Nanomaterials change the glass transition temperature of the polymer matrix. The mechanical properties of polymer hydrogels obtained via crosslinking of polyacrylamide (PAM) and chromium acetate has been significantly enhanced due to addition of silica nano particles. It is understood that addition of nano-silica into polymer/chromium acetate crosslinking systems produces composite polymeric network through coordination polymerization. The silica particles are dispersed into the polymer matrix due to small size, and produces high-toughness, wear-resisting, and good-stability to enhance mechanical strength and deformation reversibility of nanocomposites.Citation75 The mechanical properties are directly related to concentration of silica, while hydrogel without silica is brittle. The compression strength of composite hydrogel was seven time higher than that of pure hydrogel.
Application of polymer nanocomposites for water purifications
Different types of PNCs are currently being explored for usage in purification of water. The prime reason for the use of PNCs in any applications lies in their unique properties, which is different from their counterparts. Different PNCs used in water purifications technology are shown in Figure .
Methods of water purification
Different methods used for water purifications are recently been reviewed by Singh et al.Citation76 and are also tabulated in Table . These methods are mainly categorized on the basis of separation techniques like physical adsorption, chemical degradation, and biological treatment. All the methods have some advantages and disadvantages but no single process is able to purify the water adequately. Thus, combination of processes are recommended to insure adequate quality of water. The research findings have indicated that serious efforts are required to integrate different techniques like adsorption–biological treatments to enhance biodegradation of dye stuffs and reduce the sludge formation.
Table 3 Important water purification processes
Removal of heavy metal ions
Most of the surface and ground water are contaminated with many heavy and radioactive metals due to anthropogenic sources or geological reasons. These metal ions are accumulated in the biosphere and edible items. They also enter the human body through food chain and are also responsible for their biomagnification. Different toxic heavy metal ions and nanocomposites used as adsorbents are listed in Table .
Table 4 Different types of metallic pollutant and suitable adsorbents
Lim et al. has reviewed the development of economically suitable adsorbents for heavy metals removal from water and wastewater.Citation87 The different techniques used for metal removal are adsorption, chemical, precipitation, coagulation, flocculation, ion exchange, and membrane filtration. The requirement for these methods are suitable adsorbing materials with flexibility in design and operation to generate high-quality treated effluent. For the reversible nature of adsorption process, the adsorbents should be regenerated for multiple use through suitable desorption method at low maintenance cost, high efficiency, and ease of operation.Citation88,89 The interaction between the adsorbent and adsorbate molecules are both physical and chemical. Physical adsorption is reversible in nature. While, if the attraction forces is due to chemical bonding, it is difficult to desorbe the chemisorbed species from the adsorbent surface. Ion exchange techniqueCitation90 also shares various common features along with adsorption. Ion exchange is a reversible chemical process in which an ion from solution is exchanged for a similar charged ion attached to an immobile solid particle or composite film. The polymer-based nanocomposite bears superior properties for metal decontamination in different forms like candle, mat, membrane, beads, etc.Citation91 The nanocomposite-based adsorbents were prepared by infusing the inorganic nanoparticles onto the polymers such as alginate,Citation92 cellulose,Citation93 porous resins,Citation94 and ion-exchangers.Citation95 To avoid issues caused by the ultra-fine particle size such as transition loss and excessive pressure drops, porous PNCs adsorbents or ion exchangers have proved to be an ideal hybrid adsorbents, due to their excellent mechanical strength and adjustable surface chemistry which is because of polymeric support.Citation96 Qiu et al.Citation97 prepared nano iron oxide loaded polystyrene with strong sorption behavior. The presence of counter ion affects the adsorption behavior in the removal of metal ions from an aqueous solution. It is due to the formation of tertiary complex around the composite. Fe3O4 nanoparticles coated with polyethylenimine (PEI) polymer were intercalated between sodium rich montmorillonite (MMT) layersCitation98 under acidic conditions (pH 2). The composite was used as a magnetic sorbent for the adsorption of Cr(VI). At pH 2, amine groups of PEI were protonated and intercalated between MMT platelets by cationic exchange as shown in Scheme .
A superadsorbent composite was synthesized by copolymerization of acrylic acid on bentonitw powder. The compsoite was found suitable for removal of different heavy metals with qmax values 1666.67 for Pb+2, 270.27 for Ni+2, and 416.67 mg/g for Cd+2 (Figure ).
Figure 9 Adsorption isotherm for different heavy metal ions on PNCCitation99
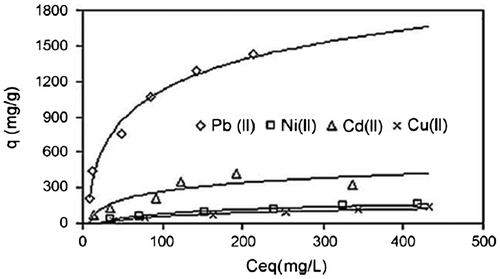
Further, basic problem of nanoparticles is their stabilization in appropriate oxidation state. Rastogi et al.Citation100 encapsulated nano size nickel (II) oxide prepared through eutectic melt in urea formaldehyde resins. Agglomeration free NiO and CuO encapsulated urea formaldehyde nanocomposite was formed and was found suitable for efficient removal of As(III) (80%). Chitosan and its nano derivatives are reported as a good adsorbents for the removal of water contaminants. An comprehensive review on the issue has been published by Shukla et al.Citation101 covering most of its technical and scientific aspects. Further, the addition of magnetic particles in composite make an advantageous feature for efficient purification of water. Chitosan-based magnetic composites show improved adsorption rate and better adsorption efficiency for removal of various pollutants. Their recovery process is also very simple and easy. Shi et al.Citation102 prepared magnetic chitosan nanocomposites on the basis of amine-functionalized magnetite nanoparticles. These nanocomposites were used to remove heavy metal ions. The interaction between chitosan and heavy metal ions are reversible, which means that those ions can be removed from chitosan in weak acidic deionized water with the assistance of ultrasound radiation. On the basis of the above referred reason, synthesized magnetic chitosan nanocomposites were used as a useful recyclable tool for heavy metal ions removal.
The inorganic particles immobilized membranes were found to remove toxic contaminants from water. Titania poly (ethersulfone) composite membranes showed higher fluxes and enhanced antifouling properties.Citation103 Other nanocomposite structures like lyotropic liquid crystals exhibited high flux and selective water transportation. For example, zeolite-polyamide based composite membranes offered new ways of designing nano filtration and reverse osmosis membranes with increased water permeability and high salt rejection.Citation104 Super magnetic sodium alginate supported tetrasodium thiacalix arene tetrasulfonate (TSTC[4]AS-s-SA) nanogel was prepared using sodium alginate nanoparticles and in situ generation of Fe3O4. The nanogel was found suitable for adsorptions of Cu(II), Cd(II), Pb(II), Co(II), Ni(II), and Cr(III) ions from aqueous solution at pH 7. The increase in adsorption capacity is due to incorporation of thiacalix[4]arene tetrasulfonate and Fe3O4 into sodium alginate nanoparticles and led to the magnetic property.Citation105 The nanofibers is an important nanostructure due to high specific surface area, uniaxial crystallinity, porosity and complex pore structures. The geometry, morphology, composition, and directional alignment of nanofibers can be easily maneuvered for specific applications. The nano-fiber based mat are reported to be suitable for nano filtration and adsorption with many advantageous features.
Other important metallic pollutants are radioactive waste (RW), which are discharged in huge amount during operations of nuclear plants and reprocessing of nuclear fuel from nuclear plants.Citation106 The major constituents of RW are cesium, neptunium, americium, uranium, and curium. Cesium radioisotopes stand as the most important fission products because of their high fission yield, long half-life, and serious environmental impacts.Citation107 The contamination caused by cesium is of serious social and environmental concerns. It causes severe risk to human health and environment, because Cs is a strong gamma emitter. Gamma radiation has high solubility and migration properties through groundwater to the biosphere.Citation108,109 In this concern, the processing of nuclear waste is important for industrial development and environmental problems. In this regard, solid phase extraction based on polymer nanocomposite ion exchangers has attracted wide interest due to their high selectivity, rapid separation, and high thermal and radiation stabilities.Citation110 It is reported that magnetic nanocomposite structures are useful for adsorbing different radioactive metal ions.Citation111,112 Some polymer nanocomposites used for removal of radioactive metals are potassium zinc hexacyanoferrate loaded polymer nanocomposite for CsCitation113, ammonium molybdophosphate–polyacrylonitrile (AMP–PAN) for removal of Co(II), Sr(II), and Cs(I).Citation114 The adsorption takes place due to both physical adsorption, ion exchange and has influence of competitive adsorption and presence of alkali metals. Ma et al.Citation115 has developed cellulose nano fiber through oxidation of wood pulp employing (2,2,6,6-tetramethylpiperidin-1-yl)oxyl (TEMPO)/NaBr/NaClO process followed by mechanical treatment. The presence of carboxylate groups on the surface of cellulose nanofibers bears negative charges, which is able to adsorb UO22+ in water with adsorption capacity 167 mg/g at least two times better than an adsorbent without carbonyl group.
Removal of dyes
The tunable surface and catalytic properties of PNCs also find huge potential to remove dyes, one of severe pollutants. Exponential discharge of dyes in water bodies, are creating alarming water problem and water born diseases.Citation116–118 Most of the dyes have detrimental effects on atmosphere and aquatic living organism and cause allergy, dermatitis, skin irritation as well as mutations in humans.Citation119,120 Various techniques such as adsorption, coagulation, filtration, photo, catalytic and biochemical degradation have been developed for removal of dyes. In this regards, polymer nanocomposites are used by many researchers for efficient removal of dyes. Some important polymer nanocomposites as dye adsorbents are listed in Table .
Table 5 Different types of adsorbents suitable for dyes removal
PNC shows several properties like high adsorption capacity, catalytic and magnetic properties for dyes removal. Addition of metal has developed functional sites and thus several hybrid structures have been used for removal of Dyes. Metal decorated polypyrole nanocomposite anode have been explored to degrade direct Red 80 upto 83–100% by electrochemical oxidation.Citation130 The rate of decomposition depends on various properties such as biocompatibility, surface area, conductivity, etc. The method is found to be effective for the removal of soluble and insoluble dyes. However, it reduces chemical oxygen demand. The other limitations are huge consumption of electric current, generation of sludge and formation of pollutants as byproducts. Thus, an advanced approach “Advanced Oxidation Processes” (AOPs) have been developed to insure simultaneous oxidation process of all components at ambient condition, because a single oxidation system is not enough for the total removal of dyes. AOPs involve accelerated production of the high reactive hydroxyl free radical, by use of Fenton’s reagent oxidation, ultra violet (UV), and sonolysis. The advantage of the method is the formation of CO2 after degradation of dyes.Citation131 Fe3O4 anisotropic nanostructures that exhibit excellent catalytic performance are rarely used to catalyze Fenton-like reactions because of the inevitable drawbacks resulting from traditional preparation methods. In this study, a facile, nontoxic, water-based approach is developed for directly regulating. A series of anisotropic morphologies of Fe3O4 nanostructures in a hydrogel matrix has been developed using facile method. The composite structure has advantages of both the catalytic activity of Fe3O4 and the adsorptive capacity of an anionic polymer network. The hybrid nanocomposites have the capability to effect the rapid removal of cationic dyes, such as methylene blue, from water samples. More interestingly, hybrid nanocomposite hydrogel loaded with Fe3O4 nanorods exhibit the highest catalytic activity compared to those composed of nanoneedles and nanooctahedra, revealing the important role of nanostructure morphology. Results revealed that Fe3O4 nanorods can efficiently catalyze H2O2 decomposition and thus generate more free radicals OH, HO2 for methylene blue degradation, which might account for their high catalytic activity. Bio-chemical treatment of dyes from wastewater is another effective technique for treatment of wide spectrum of dyes from various affluent water.Citation132,133 The method has significant advantages as it is inexpensive in nature and generates non-toxic and eco friendly degraded products. In this regard, researchers have designed various methods such as immobilization, modification, and genetic modification for the improvement in enzyme stability. Both natural and synthetic polymers (polysaccharides, polyacrylamides, alginates, resins, chitosan, etc.) have been used for bio-immobilization via loading or entrapment. For example, Amberlite MB 150 and chitosan beads were used by Tripathi et al. to immobilize amylase from mung beans (Vigna radiata).Citation134,135 The performance of free and immobilized enzymes was compared. The activity loss for free amylase after 100 days of storage at 4 °C was ~70%, whereas for Amberlite- and chitosan-based amylases, it were 45 and 55%, respectively, under the identical experimental conditions. Moreover, polymer-based amylase showed a residual activity of 43 and 27%, respectively, after 10 uses.
In addition to above methods, the adsorption technique is also widely used for dye removal.Citation136 The adsorption process indicates concentration of a material at a solid surface from its surrounding liquid or gaseous atmosphere.Citation137,138 Kayser for the first time introduced the term adsorption in 1881 to differentiate surface accumulation from intermolecular penetration. He suggested the basic feature and differentiated two types of adsorption (Physical and Chemical). Both type of adsorptions are grouped together as “sorption processes” for unified treatment of water. Ion exchange-based adsorption has been suitable for the removal of dyes. Many studies have been dedicated for dye removal exploring adsorption technique.Citation139,140 A relationship between the structure, mobility, and degree of crosslinking of these sorbents have been established. These crosslinked starch-based composites, containing tertiary amine groups are found suitable for the recovery of various dyes from aqueous solutions. The most important characteristics of the adsorbent is the quantity of adsorbate that can accumulate on its surface. It is usually estimated from the adsorption isotherms. The adsorption isotherms is a relationship between the quantity of adsorbate per unit of adsorbent (qe) and its equilibrium solution concentration (Ce) at constant temperature. The mechanistic steps of dyes adsorption on a polymer composite can be understood from Scheme . The adsorption process take place into three steps: (a) external mass transfer of impurities across the liquid boundary to exterior surface of the adsorbent surface, this can also be called film diffusion or boundary layer diffusion or outer diffusion (b) transfer of adsorbate from the adsorbent exterior surface to the bulk matrix (pores or capillaries of the adsorbent) or internal structure, which is referred as intra-particle diffusion or inner diffusion (c) the adsorption of adsorbate on top of the active sites in the inner and outer surfaces of the adsorbent.Citation141 The last step is considered to be very fast, and thus cannot be treated as a rate-limiting step.
The basic requirement of a good adsorbentCitation143 is porous structure and high surface area. The polymer nanocomposites are suitable for dye removal due to synergism between two compounds with large surface area, high adsorption capacity, suitable pore size, high mechanical strength, easy regeneration, biocompatibility, cost effectiveness, and high selectivity.Citation144 Some polymeric resins suitable for adsorbents through ion exchange mechanism are sulfonated polystyrene, sulfonated phenolic resin, phenolic resin, polystyrene phosphonate, polystyrene amidoxime, polystyrene-based trimethyl benzyl ammonium, epoxy-polyamine, and aminopolystyrene. These ion exchange resins have efficient dye removal capacity.Citation145,146 However, bio-compatibility is an persisting problem during its application. Therefore, a wide spectrum biopolymer-based nanocomposites are explored for dye removal. Chitosan, Alginate, starch based-nanocomposites have been widely used for the removal of different dyes. Particularly chitosan is a polysaccharide-based multifunctional biopolymer with primary and secondary hydroxyl groups, along with reactive amino groups. This makes it useful starting support materials for adsorption purpose. However, its chemical stability need to be optimized.Citation147 Graphene oxide (GO)-based polymer nanocomposites were fabricated in aq. solution through a hybrid novel strategy. These GO-based polymer nanocomposites were reported efficient absorbents for the removal of organic dyes from the aqueous solution. Furthermore, optimal adsorption time of GO-PDA-PSPSH nanocomposites toward MB was 58 min along with maximum adsorption efficiency at pH 7.Citation148 Similarly, starch-montmorillonite/polyaniline (St-MMT/PANI) nanocomposite was synthesized by chemical oxidative polymerization of aniline in the presence of starch-montmorillonite.Citation149 The prepared ternary nanocomposite (St-MMT/PANI) was used for the adsorption of a reactive dye. Results indicated that the removal mechanism was based on both the adsorption and electrostatic attraction between nanocomposite and dye molecules. All these results demonstrated the effectiveness of the hybrid system as an efficient adsorbent for removal of reactive dyes from textile effluents.
Removal of other pollutants
The other important water pollutants are microorganism, pesticides, pathogens, and other organic materials. The risks associated with them include the formation of disinfection by-products and multidrug resistant bacterial species and have prompted the exploration of advanced disinfection methods.Citation150 The nano structured composite kills pathogens by liberating toxic chemicals, conciliating cell membrane integrity on direct contact. In some cases, they also produces reactive oxygen species (ROS). The bactericidal effect of metals are known since ancient times, but advancements in nanotechnology have improved the efficiency and enabled its use as a viable disinfectant. However, the use of metal particles as disinfectant also poses serious health problems.Citation151 Although, disinfection mechanism is also not completely known but it is proposed that metal atom interacts with base pairs of DNA and disrupts the hydrogen bonding. Thus, it denatures the DNA molecule of the cell.Citation152 Some metals like silver, copper, zinc, iron, lead, aluminum, and gold have proved to be an efficient disinfectant. However, some of them are toxic for human, thus the non-toxic metals for mammalian cells are used. The advent of nanotechnology made nanocomposite a potential water decontaminator and replacement to current chemical disinfectants.Citation153 The metal bound copolymer beads are used efficiently for removing wide spectrum of bacterial strains upto 99.9%. The microscopic structure of Ag coated polyurathane foam used for water purificaton is shown in Figure .
Figure 10 Optical microscope image of pure polyurethane foam (A) and Ag-coated polyurethane foam(B)Citation153
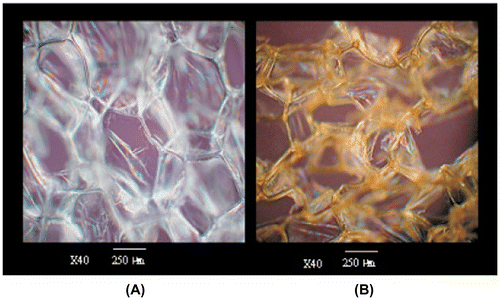
Cellulose acetate fibers embedded with Ag nanoparticles were found to be effective against bacteria.Citation154 The water filters manufactured by polyurethane’s foam coated with Ag nanofibers have efficient disinfectant properties against bacteria like Escherichia coli (E. coli). The examples of other materials to prepare low-cost potable water microfilters by incorporating Ag nanoparticles are reported to be used in remote areas in developing countries.Citation155 The results have indicated that disinfection efficiency of Ag embedded polymer filler decreased over a period of time. The different composites with carbon nano structuresCitation156 such as carbon nanotubes (CNTs), activated carbon fibers (ACFs) are also able to remove pathogenic microorganisms adequately.Citation157–160 However, efficiency of these composite need to be improved. Gunawan et al.Citation161 have investigated the immobilization of AgNP/CNTs coated on the surface of a polyacrylonitrile (PAN) hollow fiber membrane for water purification (Figure ).
Figure 11 Schematic representation of representative disinfection methodCitation161
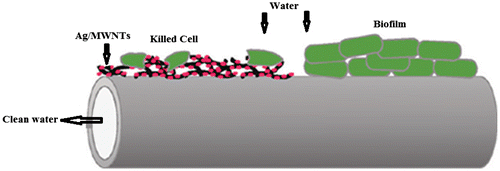
PAN alone and AgNP/CNT/PAN membranes have been used for filteration of E. Coli contaminated water. In the continuous filtration mode for E. coli feedwater, the relative flux drop over Ag/MWNTs/PAN was 6%, while over the pristine PAN it was 55% at 20 h of filtration. The results revealed that AgNP/CNT coating has significantly enhanced antimicrobial activity, as well as antifouling properties of the membranes. The microorganism removal capacity in wastewater filter systems depends on many factors.Citation162 These factors are biotic and abiotic in nature like moisture, pH, temperature, species of microorganism. In the context of improving the processes for water treatment containing organic and inorganic solutes, dendrite polymers are used as water-soluble ligands for purification purposes.Citation163,164
The use of nanofibers and composite nanostructure membranes can help in degrading a wide spectrum of organic and inorganic contaminants in real field applications as shown in Figure .
The better understanding of the formation of nanocomposite membranes will certainly be a step toward improving the performance of multifunctional nanocomposite membranes. The pattern of nanoparticles within the host matrices of membranes and change in the structures and properties of both nanomaterials and host matrices could be among the priority concerns in the real field applications of the nanofibrous membranes for water/wastewater treatment.
Quality monitoring or aquodiagnosis
Water contains significant concentrations of solids, dissolved and particulate matter, microorganisms, nutrients, heavy metals, and micro-pollutants.Citation165 The monitoring of pollution levels in water bodies like rivers, lakes, and coastal waters are also very important with the requirement to achieve good ecological and chemical status. Furthermore, constant examination of water pollutants and the ability to estimate the impact of effluent discharges in real-time, would preserve the high quality of water bodies and provide the most reliable and economical environmental protection. Thus, it is a current demand for accurate and reliable quality monitoring of pollutants concentration to allow real-time monitoring of water quality. Attempts have been made to develop different PNCs based electrochemical, photochemical devices for water testing. Some important devices are summarized in Table .
Table 6 Different types of sensor based on PNC
These devices are based on generation of different responsive signal(electrical or optical) after interaction between pollutants and PNC. The sensing signal are either adsorptions or catalytically oxidation or degradations. In this regard PNC optimizes both adsorption and catalytic behaviors in sensing substrate. Nanostructured photocatalysts with high photocatalytic activity, strong oxidative power, low cost, environmental benignity, and excellent stability have attracted extensive attention from scientific researchers, technology developers for development of different water sensor. These sensors can be used for lab-based analyzes, on-line and on-site determination of organic pollutants in wastewater. In electrochemical sensors, basic requirement is to design suitable electrode materials to measure current or voltages. A voltametric sensor was developed by using glassy carbon electrode modified with multi-walled carbon nanotube/poly(pyrocatechol violet)/bismuth film for determination of cadmium and lead as environmental pollutants.Citation181 In optimum conditions, the developed sensor showed a good linear response to Cd2+ and Pb2+ in the concentration range of 1.0–300.0 μg L−1 and 1.0–200.0 μg L−1, respectively. The limits of detection for Cd2+ and Pb2+ were 0.20 μg L−1 and 0.40 μg L−1, respectively. Sensor showed least interference from most of the common interfering ions, which may be due to specific and selective interaction of electrodes with sensed metal ions. The response of the electrode was constant for three weeks with successive operation. Similarly other electrochemical sensors are made by polypyrrole decorated graphene/β-cyclodextrin composite for mercury (II)Citation182; graphene oxide/molecularly imprinted polymer for 2,4-dichlorophenol,Citation183 graphene oxide, and conducting polymers for Organophosphate-based pesticides.Citation184
Sahu et al.Citation185 have developed one-pot synthesis for silver nanoparticle imprinted sodium alginate (SA)/polyvinyl alcohol (PVA) nanocomposite thin films. The composite film of SA–Ag/PVA thin films are found capable of detecting a very low concentration (ppb) of Hg2+ in aqueous solution by monitoring the decrease in intensity (Figure ).
Figure 13 Schematic representation of sensitive changes of SA–Ag/PVA nanocomposite thin films by Hg2+Citation185
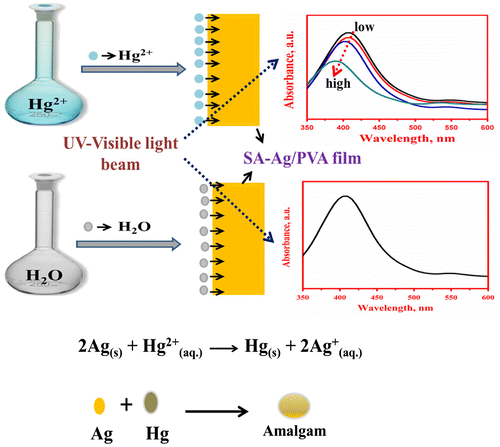
The quantitative presence of dibutyl phthalate(DBP) in tap water samples was monitored by fluorescence of molecularly imprinted polymer nanocomposites (SiO2@QDs@MIPs).Citation186 In optimized conditions, fluorescence intensity decreased linearly with increasing concentration of DBP in the range of 5–50 mmol L−1, with a correlation coefficient of 0.9974. The PNC has also been used for detection of different explosives with encouraging parameters.Citation187 Patil et al. has developed a piezoresistive ultra-sensitive polymer nanocomposite for explosive vapor detection.Citation188 The sensor promises to be used as a rugged portable, hand held device for rapid and sensitive detection of explosive vapors at different places.
Scheme 1 The preparation of ionic liquid-Fe3O4@CS@GOCitation24

Scheme 2 Illustration for the formation of polyethylenimine-based compsoite for Cr removalCitation99
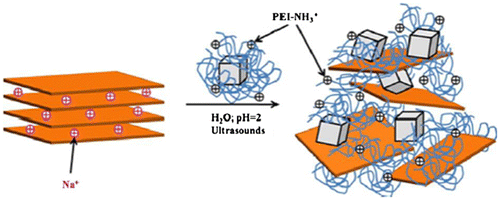
Scheme 3 Adsorption schematic diagram of MO on pHEMA-CS-f-MWCNTsCitation142
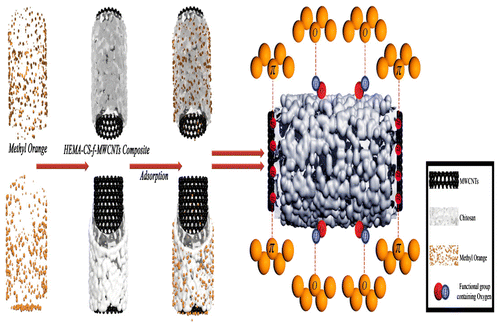
Equilibrium studies
Adsorption isotherm models
An adsorption isotherm is an equation relating equilibrium concentration of a solute on the surface of an adsorbent to the concentration of the solute in the liquid, with which it is in contact at a particular temperature and represented graphically. There are several models for predicting the equilibrium distribution. However, some widely accepted models are Langmuir, Freundlich, Dubinin–Radushkevich, Temkin, Flory–Huggins, Redlich–Peterson, Sips, Khan, BET, FHH, Halse, etc.Citation189–194
Kinetic equations
The kinetic studies for water purifications are of utmost importance for speculating the most favorable conditions to perform efficient adsorption of pollutants. The kinetics describe the solute uptake rate, which directly controls residence time of sorbate uptake at the solid–solution interface. The study also provides information about adsorption mechanisms and probable rate optimizing steps like mass transport, ionization, and chemical interactions. Several efforts have been made to formulate a general relation to describe the kinetics of pollutant adsorption on the surface of a solid adsorbent for liquid–solid phase sorption systems. The sorption kinetics can be both simple and complex. Number of kinetic models have been proposed in the literature.Citation195–205
Thermodyanic parameters
The thermodynamic parameters are required to understand the effect of temperature on the adsorption behavior of a pollutant on an adsorbent.Citation206 Some thermodynamic parameters related to adsorption process are changes in standard free energy (ΔG0), enthalpy (ΔH0), and entropy (ΔS0). These parameter can be obtained from experimental observation carried out at various temperatures.Citation207 It is reported that negative value of ΔG0 indicate spontaneity of adsorption and a positive ΔH0 value show that the process of adsorption is endothermic, however, a positive ΔS0 value show that randomness is enhanced at solid/liquid interface.Citation208
Future prospects
The long-term development of the global water situation is closely connected to the growth of the world population and global climate change. The demand for fresh water is growing dramatically. In recent years, the use of nanomaterials in water remediation has increased considerably. The nanomaterials have unique size-dependent properties and allow the development of novel high-tech materials for efficient water and wastewater treatment processes, namely membranes, adsorption materials, nanocatalysts, functionalized surfaces, coatings, and reagents. Because of agglomeration and instability, nanocomposites particularly polymer nanocomposites are now being preferred over nanomaterials. The potential of nanocomposites in various sectors of research and application is promising and attracting increasing investment from Governments and business in many parts of the world. While there are some niche applications where nanotechnology has penetrated the market, the major impact will be at least a decade away. There are certain magnetic polymer nanocomposites which have special features. Biodegradable polymer-based nanocomposites have a great deal of future promise for potential applications as high-performance biodegradable materials. These are entirely new type of materials based on plant and nature materials (organoclay). Inspite of lot of advantages of polymer nanocomposites in water remediation, there are still several drawbacks that have to be negotiated. Materials functionalized with nanoparticles incorporated or deposited on their surface have risk potential, since nanoparticles might release and emit to the environment where they can accumulate for long periods of time. Available information in the literature has revealed that several nanomaterials may have adverse effects on the environment and human health. Nevertheless, standards for assessing the toxicity of nanomaterials are relatively insufficient at present. Hence, comprehensive evaluation of the toxicity of nanomaterials is in urgent need to ensure their real applications. In order to make polymer nanocomposites an efficient and cost effective for water remediation, lot of work is to be done particularly interaction between polymer nanocomposites and the targeted pollutants or the substrates, choice of nanomaterials and polymers, optimizing conditions for water purification and toxic effect on human health and environment. Although these ideas can pave a way toward a sustainable tomorrow, in reality detailed technical study is required to bridge the gap between laboratory-scale and industrial applications.
Conclusions
This review provides up to date account of recent developments in the field of polymer nanocomposites used for water treatment. Methods for the preparation, characterization and properties of polymer nanocomposites have been reviewed. Efficiency of decontamination of metal ions, dyes, and microbes from water by nanocomposites, their regeneration possibility and cost effectiveness have made the nanocomposites as one of the most important material. Adsorption, kinetic, and thermodynamic models for removal of pollutants by adsorption have also been referred. Comparatively little information is available on the use of nanocomposite for removal of protozoa and radionucleides removal techniques. Despite various applications and advantages of polymer nanocomposites in water remediation several issues related to their use still remain to be addressed.
Disclosure statement
No potential conflict of interest was reported by the authors.
References
- M. A. Shannon, P. W. Bohn, M. Elimelech, J. G. Georgiadis, B. J. Mariñas and A. M. Mayes: ‘Science and technology for water purification in the coming decades’, Nature, 2008, 452, 301–310.10.1038/nature06599
- X. Qu, P. J. Alvarez and Q. Li: ‘Applications of nanotechnology in water and wastewater treatment’, Water Res., 2013, 47, 3931–3946.10.1016/j.watres.2012.09.058
- The United Nations World Water Development Reports: ‘Water for sustainable world’, 2015, United Nations Educational, Scientific and Cultural Organization, ISBN 978-92-3-100071-3.2. place de Fontenoy, 75352 Paris 07 SP, France.
- Application of Nanotechnology in Water Research, (ed. A. K. Mishra), Scrivener Publishing, Wiley-Scrivener; 2014. ISBN: 978-1-118-49630-5.
- P. Malaviya and A. Singh: ‘Physicochemical technologies for remediation of chromium-containing waters and wastewaters’, Crit. Rev. Environ. Sci. Technol., 2011, 41, 1111–1172.10.1080/10643380903392817
- A. Bhatnagar and M. Sillanpää: ‘Applications of chitin- and chitosan-derivatives for the detoxification of water and wastewater — A short review’, Adv. Colloid Interface Sci., 2009, 152, 26–38.10.1016/j.cis.2009.09.003
- S. Daer, J. Kharraz, A. Giwa and S. W. Hasan: ‘Recent applications of nanomaterials in water desalination: A critical review and future opportunities’, Desalination, 2015, 367, 37–48.10.1016/j.desal.2015.03.030
- P. Z. Ray and H. J. Shipley: ‘Inorganic nano-adsorbents for the removal of heavy metals and arsenic: a review’, RSC Adv., 2015, 5, 29885–29907.10.1039/C5RA02714D
- T. E Twardowski: Introduction to nanocomposite materials: properties, processing, characterization’, 2007, DEStech Publications. Pensylvania 17601 USA.
- J. Jordan, K. I. Jacob, R. Tannenbaum, M. A. Sharaf and I. Jasiuk: ‘Experimental trends in polymer nanocomposites – a review’, Mater. Sci. Eng. A, 2005, 393, 1–11.10.1016/j.msea.2004.09.044
- G. Lofrano, M. Carotenuto, G. Libralato, R. F. Domingos, A. Markus, L. Dini, R. K. Gautam, D. Baldantoni, M. Rossi, S. K. Sharma, M. C. Chattopadhyaya, M. Giugni and S. Meric: ‘Polymer functionalized nanocomposites for metals removal from water and wastewater: An overview’, Water Res., 2016, 92, 22–37.10.1016/j.watres.2016.01.033
- Z. Fu, C. He, H. Li, C. Yan, L. Chen, J. Huang and Y.-N. Liu: ‘A novel hydrophilic hydrophobic magnetic interpenetrating polymer networks (IPNs) and its adsorption towards salicylic acid from aqueous solution’, Chem. Eng. J., 2015, 279, 250–257.10.1016/j.cej.2015.04.146
- R. Surudžić, A. Janković, N. Bibić, M. Vukašinović-Sekulić, A. Perić-Grujić, V. Mišković- Stanković, S. J. Park and K. Y. Rhee: ‘Physico–chemical and mechanical properties and antibacterial activity of silver/poly(vinyl alcohol)/graphene nanocomposites obtained by electrochemical method’, Compos. Part B, 2016, 85, 102–112.10.1016/j.compositesb.2015.09.029
- F. Sun, M. Lin, Z. Dong, J. Zhang, C. Wang, S. Wang and F. Song: ‘Nanosilica-induced high mechanical strength of nanocomposite hydrogel for killing fluids’, Colloid Interface Sci., 2015, 458, 45–52.10.1016/j.jcis.2015.07.006
- H. A. Shawky, S.-R. Chae, S. Lin and M. R. Wiesner: ‘Synthesis and characterization of a carbon nanotube/polymer nanocomposite membrane for water treatment’, Desalination, 2011, 272, 46–50.10.1016/j.desal.2010.12.051
- M. Rahmat and P. Hubert: ‘Carbon nanotube–polymer interactions in nanocomposites: A review’, Compos. Sci. Technol., 2011, 72, (1), 72–84.10.1016/j.compscitech.2011.10.002
- B. Arash, H. S. Park and T. Rabczuk: ‘Mechanical properties of carbon nanotube reinforced polymer nanocomposites: A coarse-grained model’, Compos. Part B, 2015, 80, 92–100.10.1016/j.compositesb.2015.05.038
- F. Hussain, M. Hojjati, M. Okamoto and R. E. Gorga: ‘Review article: Polymer-matrix nanocomposites, processing, manufacturing, and application: An overview’, J. Compos. Mater., 2006, 40, 1511–1575.10.1177/0021998306067321
- S. K. Shukla, S. K. Shukla, P. P. Govender and E. S. Agorku: ‘A resistive type humidity sensor based on crystalline tin oxide nanoparticles encapsulated in polyaniline matrix’, Microchem. Acta, 2016, 183, (2), 573–580.10.1007/s00604-015-1678-2
- H. Dong, Y. R. Sliozberg, J. F. Snyder, J. Steele, T. L. Chantawansri, J. A. Orlicki, S. D. Walck, R. S. Reiner and A. W. Rudie: ‘Highly Transparent and Toughened Poly(methyl methacrylate) nanocomposite films containing networks of cellulose nanofibrils’, ACS Appl. Mater. Interfaces, 2015, 7, (45), 25464–25472.10.1021/acsami.5b08317
- G. David, M. Drobota and B. C. Simionescu: ‘Preparation approach effect on polyurethane/montmorillonite nanocomposites characteristics’, High Perform. Polym., 2015, 27, 555–562.10.1177/0954008315584184
- C. Li, H. Bai and G. Shi: ‘Conducting polymer nanomaterials: electrosynthesis and applications’, Chem. Soc. Rev., 2009, 38, 2397–2409.10.1039/b816681c
- S. K. Shukla, N. B. Singh and R. P. Rastogi: ‘Efficient ammonia sensing over zinc oxide /polyaniline nanocomposite’, Ind. J. Eng. Mater. Sci., 2013, 20, 319–324.
- L. Li, H. Duan, X. Wang and C. Wang: ‘Fabrication of novel magnetic nanocomposite with a number of adsorption sites for the removal of dye’, Int. J. Biol. Macromol., 2015, 78, 17–22.
- M. Cantarella, R. Sanz, M. A. Buccheri, L. Romano and V. Privitera: ‘PMMA/TiO2 nanotubes composites for photocatalytic removal of organic compounds and bacteria from water’, Mater. Sci. Semicond. Process., 2016, 42, 58–61.10.1016/j.mssp.2015.07.053
- P. Meneghetti and S. Qutubuddin: ‘Synthesis, thermal properties and applications of polymer–clay nanocomposites’, Thermochim. Acta, 2006, 442, 74–77.10.1016/j.tca.2006.01.017
- A. Cobut, H. Sehaqui and L. A. Berglund: ‘Cellulose nanocomposites by melt compounding of TEMPO-treated wood fibers in thermoplastic starch matrix’, Bio. Res., 2014, 9, (2), 3276–3289.
- Y. Wan, C. Wu, G. Xiong, G. Zuo, J. Jin, K. Ren, Y. Zhu, Z. Wang and H. Luo: ‘Mechanical properties and cytotoxicity of nanoplate-like hydroxyapatite/polylactide nanocomposites prepared by intercalation technique’, J. Mech. Behav. Biomed. Mater., 2015, 47, 29–37.10.1016/j.jmbbm.2015.03.009
- S. N. Magonov and D. H. Reneker: ‘Characterization of polymer surfaces with atomic force microscopy’, Annu. Rev. Mater. Sci., 1997, 27, 175–222.10.1146/annurev.matsci.27.1.175
- S. L. Kim, F. Fornasiero, H. G. Park, J. B. E. In, Eric Meshot, G. Giraldo, M. Stadermann, M. Fireman, J. Shan and C. P. Grigoropoulos, Fabrication of flexible, aligned carbon nanotube/polymer composite membranes by in-situ polymerization, J. Memb. Sci., 2014, 460, 91–98.10.1016/j.memsci.2014.02.016
- http://repositorium.sdum.uminho.pt/bitstream/1822/26120/1/Chapter.pdf.
- M. Bahmanyar, S. Sedaghat, A. Ramazani and H. Baniasadi: ‘Preparation of ethylene vinyl acetate copolymer/graphene oxide nanocomposite films via solution casting method and determination of the mechanical properties’, Poly. Technol. Eng., 2015, 54, 218–222.10.1080/03602559.2014.958772
- J. R. Capadona, O. V. Den Berg, L. A. Capadona, M. Schroeter, S. J. Rowan, D. J. Tyler and C. Weder: ‘A versatile approach for the processing of polymer nanocomposites with self-assembled nanofibre templates’, Nat. Nanotechnol., 2007, 29, 765–769.10.1038/nnano.2007.379
- N. D. Singho, N. Akmal, C. Lah, M. R. Johan and R. Ahmad: ‘FTIR Studies on silver-poly(methylmethacrylate) nanocomposites via in-situ polymerization technique’, Int. J. Electrochem. Sci., 2012, 7, 5596–5603.
- F. Petronella, A. Truppi, C. Ingrosso, T. Placido, M. Striccoli, M. L. Curri, A. Agostiano and R. Comparelli: ‘Nanocomposite materials for photocatalytic degradation of pollutants’, Catal. Today, 2017, 281, 85–100.10.1016/j.cattod.2016.05.048
- Y. Harada and K. Yamasaki: ‘Treatment of wastewater and sludge by a catalytic wet oxidation process’, Desalination, 1994, 98, 27–39.10.1016/0011-9164(94)00129-4
- C. Peng, B.-H. Yao, J.-X. Li and Jin-fen Niu: ‘Preparation and characterization of conjugated microspheres FeTCPP-SSA-TiO2’, Mater. Sci. Forum, 2016, 852, 244–251.10.4028/www.scientific.net/MSF.852
- S. Singh, K. C. Barick and D. Bahadu: ‘Functional oxide nanomaterials and nanocomposites for the removal of heavy metals and dyes’, Nanomater. Nanotechnol., 2013, 3, 20.
- S. Baruah, S. K. Pal and J. Dutta: ‘Nanostructured zinc oxide for water treatment’, Nanosci. Nanotechnol. Asia, 2012, 2, 90–102.
- M. A. Lazar, S. Varghese and S. S. Nair: ‘Photocatalytic water treatment by titanium dioxide: Recent updates’, Catalysts, 2012, 2, 572–601.10.3390/catal2040572
- A. Di Mauro, M. Cantarella, G. Nicotra, G. Pellegrino, A. Gulino, M. V. Brundo, V. Privitera and G. Impellizzeri: ‘Novel synthesis of ZnO/PMMA nanocomposites for photocatalytic applications’, Sci. Rep., 2017, 7, 40895.10.1038/srep40895
- E. Brillas and C. A. Martínez-Huitle: ‘Decontamination of wastewaters containing synthetic organic dyes by electrochemical methods. An updated review’, Appl. Catal. B Environ., 2015, 166–167, 603–643.10.1016/j.apcatb.2014.11.016
- C. Han, J. Lalley, N. Devi, K. Cromer and N. N. Mallikarjuna: ‘Titanium dioxide-based antibacterial surfaces for water treatment’, Curr. Opin. Chem. Eng., 2016, 11, 46–51.10.1016/j.coche.2015.11.007
- M. U. Sankar, S. Aigal, S. M. Maliyekkal, A. Chaudhary, A. Anshup, A. Kumar, K. Chaudhari and T. Pradeep: ‘Biopolymer-reinforced synthetic granular nanocomposites for affordable point-of-use water purification’, PNAS, 2013, 110, 8459–8464.10.1073/pnas.1220222110
- J. S. Zhang, X. F. Chen , K. Takanabe, K. Maeda, K. Domen, J. D. Epping, X. Z. Fu, M. Antonietti and X. C. Wang: ‘Synthesis of a carbon nitride structure for visible-light catalysis by copolymerization’, Chem. Int. Ed., 2010, 49, 441–444.10.1002/anie.200903886
- Y. Cui, Z. Ding, P. Liu, M. Antonietti, X. Fu and X. Wang: ‘Metal-free activation of H2O2 by g-C3N4 under visible light irradiation for the degradation of organic pollutants’, Phys. Chem. Chem. Phys., 2012, 14, 1455–1462.10.1039/C1CP22820J
- Y. L. Tian, B. B. Chang, J. L. Lu, J. Fu, F. N. Xi and X. P. Dong: ‘Hydrothermal synthesis of graphitic carbon nitride–Bi2WO6 heterojunctions with enhanced visible light photocatalytic activities’, ACS Appl. Mater. Interfaces, 2013, 5, 7079–7085.10.1021/am4013819
- R. Hao, G. Wang, H. Tang, L. Sun, C. Xu and D. Han: ‘Template-free preparation of macro/mesoporous g-C3N4/TiO2 heterojunction photocatalysts with enhanced visible light photocatalytic activity’, Appl. Catal. B: Environ., 2016, 187, 47–58.10.1016/j.apcatb.2016.01.026
- X. J. Wang, Q. Wang, F. T. Li, W. Y. Yang, Y. Zhao, Y. J. Hao and S. J. Liu: ‘Novel BiOCl–C3N4 heterojunction photocatalysts: In situ preparation via an ionic-liquid assisted solvent-thermal route and their visible-light photocatalytic activities’, Chem. Eng. J., 2013, 234, 361–371.10.1016/j.cej.2013.08.112
- Y. X. Ji, J. F. Cao, L. Q. Jiang, Y. H. Zhang and Z. G. Yi: ‘G-C3N4/BiVO4 composites with enhanced and stable visible light photocatalytic activity’, J. Alloy. Compd., 2014, 590, 9–14.10.1016/j.jallcom.2013.12.050
- Y. L. Tian, F. X. Cheng, X. Zhang, F. Yan, B. C. Zhou, Z. Chen, J. Y. Liu, F. N. Xi and X. P. Dong: ‘Solvothermal synthesis and enhanced visible light photocatalytic activity of novel graphitic carbon nitride–Bi2MoO6 heterojunctions’, Powder Tech., 2014, 267, 126–133.10.1016/j.powtec.2014.07.021
- L. Ge, C. C. Han and J. Liu: ‘Novel visible light-induced g-C3N4/Bi2WO6 composite photocatalysts for efficient degradation of methyl orange’, Appl. Catal. B: Environ., 2011, 108–109, 100–107.10.1016/j.apcatb.2011.08.014
- C. S. Xing, Z. D. Wu, D. L. Jiang and M. Chen: ‘Hydrothermal synthesis of In2S3/g-C3N4 heterojunctions with enhanced photocatalytic activity’, J. Colloid Interface Sci., 2014, 433, 9–15.10.1016/j.jcis.2014.07.015
- Y. L. Tian, B. B. Chang, J. Fu, B. C. Zhou, J. Y. Liu, F. N. Xi and X. P. Dong: ‘Graphitic carbon nitride/Cu2O heterojunctions: Preparation, characterization, and enhanced photocatalytic activity under visible light’, J. Solid State Chem., 2014, 212, 1–6.
- X. Chen, P. F. Tan, B. H. Zhou, H. G. Dong, J. Pan and X. Xiong: ‘A green and facile strategy for preparation of novel and stable Cr-doped SrTiO3/g-C3N4 hybrid nanocomposites with enhanced visible light photocatalytic activity’, J. Alloy. Compd., 2015, 647, 456–462.10.1016/j.jallcom.2015.06.056
- L. A. Gu, J. Y. Wang, Z. J. Zou and X. J. Han: ‘Graphitic-C3N4-hybridized TiO2 nanosheets with reactive {0 0 1} facets to enhance the UV- and visible-light photocatalytic activity’, J. Hazard. Mater., 2014, 268, 216–223.10.1016/j.jhazmat.2014.01.021
- J. L. Zhao, Z. Y. Ji, X. P. Shen, H. Zhou and L. B. Ma: ‘Facile synthesis of WO3 nanorods/g-C3N4 composites with enhanced photocatalytic activity’, Ceram. Int., 2015, 41, 5600–5606.10.1016/j.ceramint.2014.12.140
- Y. P. Zhu, M. Li, Y. L. Liu, T. Z. Ren and Z. Y. Yuan: ‘Carbon-doped ZnO hybridized homogeneously with graphitic carbon nitride nanocomposites for photocatalysis’, J. Phys. Chem. C, 2014, 118, 10963–10971.10.1021/jp502677h
- A. Akhundi and A. Habibi-Yangjeh: ‘Novel magnetically separable g-C3N4/AgBr/Fe3O4 nanocomposites as visible-light-driven photocatalysts with highly enhanced activities’, Ceram. Int., 2015, 41, 5634–5643.10.1016/j.ceramint.2014.12.145
- L. Y. Ng, A. W. Mohammad, C. P. Leo and N. Hilal: ‘Polymeric membranes incorporated with metal/metal oxide nanoparticles: A comprehensive review’, Desalination, 2013, 308, 15–33.10.1016/j.desal.2010.11.033
- A. Mills and S. Le Hunte: ‘An overview of semiconductor photocatalysis’, J. Photochem. Photobiol. A: Chem., 1997, 108, 1–35.10.1016/S1010-6030(97)00118-4
- Y. Paz: ‘Application of TiO2 photocatalysis for air treatment: Patents’ overview’, Appl. Catal. B: Environ., 2010, 99, 448–460.10.1016/j.apcatb.2010.05.011
- A. Rahimpour, S. S. Madaeni, A. H. Taheri and Y. Mansourpanah: ‘Coupling TiO2 nanoparticles with UV irradiation for modification of polyethersulfone ultrafiltration membranes’, J. Membr. Sci., 2008, 313, 158–169.10.1016/j.memsci.2007.12.075
- R. A. Damodar, S. J. You and H. H. Chou: ‘Study the self cleaning, antibacterial and photocatalytic properties of TiO2 entrapped PVDF membranes’, J. Hazard. Mater., 2009, 172, 1321–1328.10.1016/j.jhazmat.2009.07.139
- P. Khare, A. Yadav, J. Ramkumar and N. Verma: ‘Microchannel-embedded metal–carbon–polymer nanocomposite as a novel support for chitosan for efficient removal of hexavalent chromium from water under dynamic conditions’, Chem. Eng. J., 2016, 293, 44–54.10.1016/j.cej.2016.02.049
- H. Mittal, A. Maity and S. S. Ray: ‘Synthesis of co-polymer-grafted gum karaya and silica hybrid organic–inorganic hydrogel nanocomposite for the highly effective removal of methylene blue’, Chem. Eng. J., 2015, 279, 166–179.10.1016/j.cej.2015.05.002
- H. Faghihian, M. Iravani, M. Moayed and M. Ghannadi-Maragheh: ‘Preparation of a novel PAN–zeolite nanocomposite for removal of Cs+ and Sr2+ from aqueous solutions: Kinetic, equilibrium, and thermodynamic studies’, Chem. Eng. J., 2013, 222, 41–48.10.1016/j.cej.2013.02.035
- X. F. Sun, B. Liu and Z. Jing: ‘Preparation and adsorption property of xylan/poly(acrylic acid) magnetic nanocomposite hydrogel adsorbent’, Carbohydr. Polym., 2015, 118, 16–23.10.1016/j.carbpol.2014.11.013
- G. Mittal, V. Dhand, K. Y. Rhee, S.-J. Park and W. R. Lee: ‘A review on carbon nanotubes and graphene as fillers in reinforced polymer nanocomposites’, J. Ind. Eng. Chem., 2015, 21, 11–25.10.1016/j.jiec.2014.03.022
- B. Arash, Q. Wang and V. Varadan: ‘Mechanical properties of carbon nanotube/polymer composites’, Sci. Rep., 2014, 4, 6479.
- Z. Sui, Q. Meng, X. Zhang, R. Ma and B. Cao: ‘Green synthesis of carbon nanotube–graphene hybrid aerogels and their use as versatile agents for water purification’, J. Mater. Chem., 2012, 22, 8767–8771.10.1039/c2jm00055e
- G. Mittal, V. Dhand, K. Y. Rhee, S.-J. Park and W. R. Lee: ‘A review on carbon nanotubes and graphene as fillers in reinforced polymer nanocomposites’, J. Ind. Eng. Chem., 2015, 21, 11–25.10.1016/j.jiec.2014.03.022
- B. Arash, H. S. Park and T. Rabczuk: ‘Mechanical properties of carbon nanotube reinforced polymer nanocomposites: A coarse-grained model’, Compos. Part B., 2015, 80, 92–100.10.1016/j.compositesb.2015.05.038
- S. Makireddi, S. Shivprasad, V. Francis, G. Varghese and K. Balasubramanium: ‘Electro-elastic properties of MWCNT/PMMA nanocomposite thin films’, J. Mater. Sci. Mech. Eng., 2015, 2, (1), 24–28.
- F. Sun, M. Lin, Z. Dong, J. Zhang, C. Wang, S. Wang and F. Song: ‘Nanosilica-induced high mechanical strength of nanocomposite hydrogel for killing fluids’, J. Colloid. Interface Sci., 2015, 458, 45–52.10.1016/j.jcis.2015.07.006
- N. B. Singh and S. Agarwal: ‘Nanocomposites: an overview’, Emerg. Mater. Res., 2016, 5, (1), 5–43.10.1680/jemmr.15.00025
- A. Riccardo and A. Muzzarelli: ‘Potential of chitin/chitosan-bearing materials for uranium recovery: An interdisciplinary review’, Carbohydrate Polymers, 2011, 84, 54–63.
- T. Sreenivasan, R. Sreenivasan and A. R. Tharunl: ‘Adsorptive removal of thorium(IV) from aqueous solutions using poly(methacrylic acid)-grafted chitosan/bentonite composite matrix: Process design and equilibrium studies’, Colloids Surf. A, 2010, 368, 13–22.
- Y.-Z. Wei, H. Hoshi, M. Kumagai, T. Asakura and Y. Morita: ‘Separation of Am(III) and Cm(III) from trivalent lanthanides by 2,6-bistriazinylpyridine extraction chromatography for radioactive waste management’, J. Alloys Comp., 2004, 374, 447–450.10.1016/j.jallcom.2003.11.059
- S. Abbasizadeh, A. R. Keshtkar and M. A. Mousavian: ‘Preparation of a novel electrospun polyvinyl alcohol/titanium oxide nanofiber adsorbent modified with mercapto groups for uranium(VI) and thorium(IV) removal from aqueous solution’, Chem. Eng. J., 2013, 220, 161–171.10.1016/j.cej.2013.01.029
- A. R. Keshtkar, M. Irani and M. A. Moosavian: ‘Removal of uranium (VI) from aqueous solutions by adsorption using a novel electrospun PVA/TEOS/APTES hybrid nanofiber membrane: comparison with casting PVA/TEOS/APTES hybrid membrane’, J. Radioanal. Nucl. Chem., 2013, 295, 563–571.10.1007/s10967-012-2110-6
- M. S. Gasser and H. G. Nowier: ‘Separation of strontium and cadmium ions from nitrate medium by ion-exchange membrane in an electrodialysis system’, J. Chem. Technol. Biotechnol., 2004, 79, 97–102.10.1002/(ISSN)1097-4660
- S. Simex, U. Ulusoy and Ö. Ceyhan: ‘Adsorption of UO22+, Tl+, Pb2+, Ra2+ and Ac3+ onto polyacrylamide-bentonite composite’, J. Radio. Anal. Nucl. Chem., 2003, 256, (2), 315–321.
- A. K. Kaygun and S. Akyil: ‘Study of the behaviour of thorium adsorption on PAN/zeolite composite adsorbent’, J. Hazard. Mater., 2007, 147, 357–362.10.1016/j.jhazmat.2007.01.020
- D. Baybaş and U. Ulusoy: ‘The use of polyacrylamide-aluminosilicate composites for thorium adsorption’, Appl. Clay Sci., 2011, 51, 138–146.
- E. H. Borai, M. M. E. Breky, M. S. Sayed and M. M. Abo-Aly: ‘Synthesis, characterization and application of titanium oxide nanocomposites for removal of radioactive cesium, cobalt and europium ions’, J. Colloid Interface Sci, 2015, 450, 17–25.10.1016/j.jcis.2015.02.062
- A. P. Lim and A. Z. Aris: ‘A review on economically adsorbents on heavy metals removal in water and wastewater’, Rev. Environ. Sci. Biotechnol., 2014, 13, 163–181.10.1007/s11157-013-9330-2
- M. Chiban, M. Zerbet, G. Carja and F. Sinan: ‘Application of low-cost adsorbents for arsenic removal: A review’, J. Environ. Chem. Ecotoxicol., 2012, 4, (5), 91–102.
- S. P. Mishra, V. K. Singh and D. Tiwari: ‘Radio tracer technique in absorption study: Part XIV: Efficient removal of mercury from aqueous solution by hydrous zirconium oxide’, Appl. Radiat. Isot., 1996, 47, 15–21.10.1016/0969-8043(95)00260-X
- M. A. Hickner, A. M. Herring and E. Coughlin: ‘Anion exchange membranes: Current status and moving forward’, J. Poly. Sci Part B: Poly. Phy., 2013, 51, 1727–1735.10.1002/polb.23395
- S. Chowdhury and R. Balasubramanian: ‘Recent advances in the use of graphene-family nanoadsorbents for removal of toxic pollutants from wastewater’, Adv. Colloid Interface Sci., 2014, 204, 35–56.10.1016/j.cis.2013.12.005
- A. I. Zouboulis and I. A. Katsoyiannis: ‘Arsenic removal using iron oxide loaded alginate beads’, Ind. Eng. Chem. Res., 2002, 41, 6149–6155.10.1021/ie0203835
- X. Guo and F. Chen: ‘Removal of arsenic by bead cellulose loaded with iron oxyhydroxide from groundwater’, Environ. Sci. Technol., 2005, 39, 6808–6818.10.1021/es048080k
- I. A. Katsoyiannis and A. I. Zouboulis: ‘Removal of arsenic from contaminated water sources by sorption onto iron-oxide-coated polymeric materials’, Water Res., 2002, 36, 5141–5155.10.1016/S0043-1354(02)00236-1
- L. M. Blaney, S. Cinar and A. K. Sengupta: ‘Hybrid anion exchanger for trace phosphate removal from water and wastewater’, Water Res., 2007, 41, 1603–1613.10.1016/j.watres.2007.01.008
- B. C. Pan, Q. R. Zhang, W. M. Zhang, B. J. Pan, W. Du, L. Lv, Q. J. Zhang, Z. W. Xu and Q. X. Zhang: ‘Highly effective removal of heavy metals by polymer-based zirconium phosphate: A case study of lead ion’, J. Colloid Interface Sci., 2007, 310, 99–105.10.1016/j.jcis.2007.01.064
- H. Qiu, S. Zhang, B. Pan, W. Zhang and L. Lv: ‘Effect of sulfate on Cu(II) sorption to polymer-supported nano-iron oxides: Behavior and XPS study’, J. Colloid Interfaces Sci., 2012, 366, 37–43.10.1016/j.jcis.2011.09.070
- K. Vijayaraghavan, T. V. N. Padmesh, K. Palanivelu and M. Velan: ‘Biosorption of nickel(II) ions onto Sargassum wightii: Application of two-parameter and three-parameter isotherm models’, J. Hazard. Mater. B, 2006, 133, 304–330.10.1016/j.jhazmat.2005.10.016
- B. Samiey, C.-H. Cheng and J. Wu: ‘Organic-inorganic hybrid polymers as adsorbents for removal of heavy metal ions from solutions: A review’, Materials, 2014, 7, 673–726.10.3390/ma7020673
- R. P. Rastogi, S. K. Shukla and N. B. Singh: ‘Nano-size copper oxide encapsulated urea – formaldehyde resin film for arsenic (III) removal from aqueous solutions’, Ind. J. Eng. Mater. Sci., 2011, 18, 390–392.
- S. K. Shukla, A. K. Mishra, O. A. Arotiba and B. B. Mamba: ‘Chitosan-based nanomaterials: A state-of-the-art review’, Int. J. Bio. Macromol, 2013, 59, 46–58.10.1016/j.ijbiomac.2013.04.043
- J. Shi, H. Li, H. Lu and X. Zhao: ‘Use of carboxyl functional magnetite nanoparticles as potential sorbents for the removal of heavy metal ions from aqueous solution’, J. Chem. Eng. Data, 2015, 60, (7), 2035–2041.10.1021/je5011196
- Y. Yang, H. Zhang, P. Wang, Q. Zheng and J. Li: ‘The influence of nano-sized TiO2 fillers on the morphologies and properties of PSF UF membrane’, J. Memb. Sci., 2007, 288, 231–238.10.1016/j.memsci.2006.11.019
- B.-H. Jeong, E. M. V. Hoek, Y. Yan, A. Subramani, X. Huang, G. Hurwitz, A. K. Ghosh and A. Jawor: ‘Interfacial polymerization of thin film nanocomposites: A new concept for reverse osmosis membranes’, J. Memb. Sci., 2007, 294, (1–2), 1–7.10.1016/j.memsci.2007.02.025
- M. M. Lakouraj, F. Mojerlou and E. N. Zare: ‘Nanogel and superparamagnetic nanocomposite based on sodium alginate for sorption of heavy metal ions’, Carbohydr. Polym., 2014, 106, 34–41.10.1016/j.carbpol.2014.01.092
- T. P. Valsala, S. C. Roy, J. G. Shah, J. Gabriel, K. Raj and V. Venugopal: ‘Removal of radioactive caesium from low level radioactive waste (LLW) streams using cobalt ferrocyanide impregnated organic anion exchanger’, J. Hazard. Mater., 2009, 166, 1148–1153.10.1016/j.jhazmat.2008.12.019
- A. Nilchi, H. Atashi, A. H. Javid and R. Saberi: ‘Preparations of PAN-based adsorbers for separation of cesium and cobalt from radioactive wastes’, Appl. Radiat. Isot., 2007, 65, 482–487.10.1016/j.apradiso.2006.12.003
- C.-Y. Chang, L.-K. Chau, W.-P. Hu, C.-Y. Wang and J.-H. Liao: ‘Nickel hexacyanoferrate multilayers on functionalized mesoporous silica supports for selective sorption and sensing of cesium’, Micro. Meso. Mater., 2008, 109, 505–512.10.1016/j.micromeso.2007.05.057
- R. Cortés-Martínez, M. T. Olguín and M. Solache-Ríos: ‘Cesium sorption by clinoptilolite-rich tuffs in batch and fixed-bed systems’, Desalination, 2010, 258, 164–170.10.1016/j.desal.2010.03.019
- R. R. Sheha and E. A. El-Shazly: ‘Kinetics and equilibrium modeling of Se(IV) removal from aqueous solutions using metal oxides’, Chem. Eng. J., 2010, 160, 63–71.
- M. O. Abd El-Magied, A. A. Tolba, H. S. El-Gendy, S. A. Zaki and A. A. Atia: ‘Studies on the recovery of Th(IV) ions from nitric acid solutions using amino-magnetic glycidyl methacrylate resins and application to granite leach liquors’, Hydrometallurgy, 2017, 169, 89–98.10.1016/j.hydromet.2016.12.011
- A. Z. Zach-Maor, R. Semiat and H. Shemer: ‘Synthesis, performance, and modeling of immobilized nano-sized magnetite layer for phosphate removal’, J. Colloid Interface Sci., 2011, 357, 440–446.10.1016/j.jcis.2011.01.021
- R. R. Sheha: ‘Synthesis and characterization of magnetic hexacyanoferrate (II) polymeric nanocomposite for separation of cesium from radioactive waste solutions’, J. Colloid. Interface Sci, 2012, 388, 21–30.10.1016/j.jcis.2012.08.042
- Y. Park, Y.-C. Lee, W. S. Shin and S.-J. Choi: ‘Removal of cobalt, strontium and cesium from radioactive laundry wastewater by ammonium molybdophosphate–polyacrylonitrile (AMP–PAN)’, Chem. Eng. J., 2010, 162, 685–695.10.1016/j.cej.2010.06.026
- H. Ma, B. S. Hsiao and B. Chu: ‘Ultrafine cellulose nanofibers as efficient adsorbents for removal of UO22+ in water’, ACS Macro Lett., 2012, 1, (1), 213–216.10.1021/mz200047q
- A. Ahmad, S. H. Mohd-Setapar, C. S. Chuong, A. Khatoon, W. A. Wani, R. Kumar and M. Rafatullah: ‘Recent advances in new generation dye removal technologies: novel search for approaches to reprocess wastewater’, RSC Adv., 2015, 5, 30801–30818.10.1039/C4RA16959J
- G. Moussavi and M. Mahmoudi: ‘Removal of azo and anthraquinone reactive dyes from industrial wastewaters using MgO nanoparticles’, J. Hazard. Mater., 2009, 168, 806–812.10.1016/j.jhazmat.2009.02.097
- M. T. Yagub, T. K. Sen, S. Afroze and H. M. Ang: ‘Dye and its removal from aqueous solution by adsorption: A review’, Adv. Colloid Interface Sci., 2014, 209, 172–184.10.1016/j.cis.2014.04.002
- R. Helmer and I. Hespanhol: ‘Water pollution control – a guide to the use of water quality management principles’, 1997, London, E & FN Spon.
- J. H. Lehr, T. E. Gass and W. Pettyjohn: ‘Domestic water treatment’, 1980, New York, McGraw-Hill Book Company.
- H.-Y. Zhu, R. Jiang, L. Xiao and W. Li: ‘A novel magnetically separable γ-Fe2O3/crosslinked chitosan adsorbent: Preparation, characterization and adsorption application for removal of hazardous azo dye’, J. Hazard. Mater., 2010, 179, 251–257.10.1016/j.jhazmat.2010.02.087
- A. Debrassi, T. Baccarin, C. Demarchi, N. Nedelko, A. Ślawska-Waniewska, P. Dłużewski, M. Bilska and C. A. Rodrigues: ‘Adsorption of Remazol Red 198 onto magnetic N-lauryl chitosan particles: equilibrium, kinetics, reuse and factorial design’, Environ. Sci. Pollut. Res, 2012, 19, 1594–1604.10.1007/s11356-011-0662-6
- H. Y. Zhu, R. Jiang, R. Y. Q. Fu, J. H. Jiang, L. Xiao and G. M. Zeng: ‘Preparation, characterization and dye adsorption properties of γ-Fe2O3/SiO2/chitosan composite’, Appl. Surf. Sci., 2011, 258, 1337–1344.10.1016/j.apsusc.2011.09.045
- J. P. Wang, H. C. Yang and C. T. Hsieh: ‘Adsorption of phenol and basic dye on carbon nanotubes/carbon fabric composites from aqueous solution’, Sep. Sci. Technol., 2010, 46, 340–348.10.1080/01496395.2010.508066
- H. Y. Zhu, R. Jiang, L. Xiao and G. M. Zeng: ‘Preparation, characterization, adsorption kinetics and thermodynamics of novel magnetic chitosan enwrapping nanosized γ-Fe2O3 and multi-walled carbon nanotubes with enhanced adsorption properties for methyl orange’, Bioresour. Technol., 2010, 101, 5063–5069.10.1016/j.biortech.2010.01.107
- G. Annadurai, R. S. Juang and D. J. Lee: ‘Use of cellulose-based wastes for adsorption of dyes from aqueous solutions’, J. Hazard. Mater., 2002, 92, 263–274.10.1016/S0304-3894(02)00017-1
- G. McKay, M. El-Geundi and M. M. Nassar: ‘Equilibrium studies for the adsorption of dyes on Bagasse Pith’, Adsorpt. Sci. Technol., 1997, 15, 251–270.10.1177/026361749701500401
- S. Ghorai, A. Sarkar, M. Raoufi, A. B. Panda, H. Schönherr and S. Pal: ‘Enhanced removal of methylene blue and methyl violet dyes from aqueous solution using a nanocomposite of hydrolyzed polyacrylamide grafted xanthan gum and incorporated nanosilica’, ACS Appl. Mater. Interfaces, 2014, 6, 4766–4777.10.1021/am4055657
- T. Yaoji, D. Ma and L. Zhu: ‘Sorption behavior of methyl violet onto poly(acrylic acid-co-acrylamide)/kaolin hydrogel composite’, Poly-Plast. Technol. Eng., 2014, 53, 851–857.
- A. Lopes and S. Martins: ‘Degradation of a textile dye C. I. Direct red 80 by electrochemical processes’, Portugaliae Electrochim. Acta, 2004, 22, 279–294.10.4152/pea.200403279
- Y. Gao, C. Hu, W. J. Zheng, S. Yang, F. Li, S. D. Sun, M. Zrínyi, Y. Osada, Z. M. Yang and Y. M. Chen: ‘Fe3O4 anisotropic nanostructures in hydrogels: efficient catalysts for the rapid removal of organic dyes from wastewater’, Chem. Phys. Chem., 2016, 17, (13), 1999–2007.10.1002/cphc.v17.13
- S. C. R. Santos, V. J. P. Vilar and R. A. R. Boaventura: ‘Waste metal hydroxide sludge as adsorbent for a reactive dye’, J. Hazard. Mater., 2008, 153, 999–1008.10.1016/j.jhazmat.2007.09.050
- F. P. Van der Zee and S. C. llaverde: ‘Combined anaerobic–aerobic treatment of azo dyes – A short review of bioreactor studies’, Water Res., 2005, 39, 1425–1440.10.1016/j.watres.2005.03.007
- V. C. F. da Silva and F. J. Contesini: ‘Enantioselective behavior of lipases from Aspergillus niger immobilized in different support’, J. Ind. Microbiol. Biotechnol., 2009, 36, (7), 949–954.10.1007/s10295-009-0573-4
- P. Tripathi, A. Kumari, P. Rath and A. M. Kayastha: ‘Immobilization of _-amylase from mung beans (Vigna radiata) on Amberlite MB 150 and chitosan beads: A comparative study’, J. Mol. Catal. B: Enzym., 2007, 49, (1–4), 69–74.10.1016/j.molcatb.2007.08.011
- R. C. Bansal and M. Goyal: ‘Activated carbon adsorption’, 2005, CRC Press. Taylor & Francis Group. ISBN 9780824753443.10.1201/9781420028812
- N. P. Cheremisinoff: ‘Handbook of water and wastewater treatment technologies’, 2002, Boston, MA, Butterworth-Heinemann.
- C. L. Mantell: ‘Adsorption’, 2nd edn, 1951, New York, McGraw-Hill Book Company.
- Water Purification, (ed. N. Gertsen and L. Sønderby), 2009, Nova Science Publishers. New York.
- N. P. Raval, P. U. Shah and N. K. Shah: ‘Adsorptive amputation of hazardous azo dye Congo red from wastewater: a critical review’, Environ. Sci. Pollut. Res., 2016, 23, (15), 14810–14853.10.1007/s11356-016-6970-0
- K. L. Li and X. H. Wang: ‘Adsorptive removal of Pb(II) by activated carbon prepared from Spartina alterniflora: Equilibrium, kinetics and thermodynamics’, Bioresour. Technol., 2009, 100, 2810–2815.10.1016/j.biortech.2008.12.032
- H. Mahmoodian, O. Moradi, B. Shariatzadeha, T. A. Salehf, I. Tyagi, A. Maity, M. Asif and V. K. Gupta: ‘Enhanced removal of methyl orange from aqueous solutions by poly HEMA–chitosan-MWCNT nano-composite’, J. Mol. Liq., 2012, 202, 189–198.
- B. G. Linsen: ‘Physical and chemical aspects of adsorbents and catalysts’, 1970, London, Academic Press.
- V. Dulman, S.-M. Cucu-Man, I. Bunia and M. Dumitras: ‘Batch fixed bed column studies on removal of Orange G acid dye by a weak base functionalized polymer’, Desalination and Water Treatment, 2016, 57, 14708–14727.10.1080/19443994.2015.1065767
- Y. Yu, Y.-Y. Zhuang and Z.-H. Wang: ‘Adsorption of water-soluble dye onto functionalized resin’, J. Colloid Interface Sci., 2001, 242, 288–293.10.1006/jcis.2001.7780
- X. Zhang, A. Li, Z. Jiang and Q. Jiang: ‘Adsorption of dyes and phenol from water on resin adsorbents: Effect of adsorbate size and pore size distribution’, J. Hazard. Mater., 2006, 137, 1115–1122.10.1016/j.jhazmat.2006.03.061
- M. Vakili, M. Rafatullah, B. Salamatinia, A. Z. Abdullah, M. H. Ibrahim, K. B. Tan, Z. Gholami and P. Amouzgar: ‘Application of chitosan and its derivatives as adsorbents for dye removal from water and wastewater: A review’, Carbohyd. Polym., 2014, 113, 115–130.10.1016/j.carbpol.2014.07.007
- W. Qing, L. Meiying, X. Yili, T. Jianwen, H. Qiang, D. Fengjie, M. Liucheng, Z. Qingsong, Z. Xiaoyong and W. Yen: ‘Facile and highly efficient fabrication of graphene oxide-based polymer nanocomposites through mussel-inspired chemistry and their environmental pollutant removal application’, J. Mater. Sci., 2017, 52, (1), 504–518.
- A. Azhar and F. Farshi: ‘Eco-friendly biopolymer/clay/conducting polymer nanocomposite: Characterization and its application in reactive dye removal’, Fibers Polym., 2014, 15, (6), 1321–1329.
- M. E. Verbyla and J. R. Mihelcic: ‘A review of virus removal in wastewater treatment pond systems’, Water Research, 2015, 71, 107–124.10.1016/j.watres.2014.12.031
- X. Zhang, J. Qian and B. Pan: ‘Fabrication of novel magnetic nanoparticles of multifunctionality for water decontamination’, Environ. Sci. Technol., 2016, 50, 881–889.10.1021/acs.est.5b04539
- U. Klueh, V. Wagner, S. Kelly and A. Johnson: ‘Efficacy of silver-coated fabric to prevent bacterial colonization and subsequent device-based biofilm formation’, J. Biomed. Mater. Res, 2000, 53, 621–631.10.1002/(ISSN)1097-4636
- P. Jain and T. Pradeep: ‘Potential of silver nanoparticle-coated polyurethane foam as an antibacterial water filter’, Biotechnol. Bioeng., 2005, 90, 59–63.10.1002/(ISSN)1097-0290
- K. A. Rieger, H. J. Cho, H. F. Yeung, W. Fan and J. D. Schiffman: ‘Antimicrobial activity of silver ions released from zeolites immobilized on cellulose nanofiber mats’, ACS Appl. Mater. Interfaces, 2016, 8, (5), 3032–3040.10.1021/acsami.5b10130
- G. McKay, J. F. Porter and G. R. Prasad: ‘The removal of dye colours from aqueous solutions by adsorption on low-cost materials’, Water Air Soil Pollut., 1999, 114, 423–438.10.1023/A:1005197308228
- A. Tiwari and S. K. Shukla: ‘Advanced carbon materials and technology’, 2013, Scrivener Publishing LLC. ISBN 978-1-118-68623-2. Scrivener Publishing.
- S. Y. Liu, X. Zheng, Y. Yang, J. Liu and J. Li: ‘The removal and inhibitory effect of CNTs on model viruses’, Mater. Sci. Forum, 2013, 743–744, 402–408.10.4028/www.scientific.net/MSF.743-744
- S. M. Al-Hakami, A. B. Khalil, T. Laoui and M. A. Atieh: ‘Fast disinfection of Escherichia coli bacteria using carbon nanotubes interaction with microwave radiation’, Bioinorg. Chem. Appl., 2012, 2013, 458943.
- M. Lilly, X. Dong, E. McCoy and L. Yang: ‘Inactivation of Bacillus anthracis spores by single-walled carbon nanotubes coupled with oxidizing antimicrobial chemicals’, Environ. Sci. Technol., 2012, 46, 13417–13424.10.1021/es303955k
- N. R. Srinivasan, P. A. Shankar and R. Bandyopadhyaya: ‘Plasma treated activated carbon impregnated with silver nanoparticles for improved antibacterial effect in water disinfection’, Carbon, 2013, 57, 1–10.10.1016/j.carbon.2013.01.008
- P. Gunawan, C. Guan, X. Song, Q. Zhang, S. S. J. Leong, C. Tang, Y. Chen and M. B. Chan-Park: ‘Hollow fiber membrane decorated with Ag/MWNTs: Toward effective water disinfection and biofouling control’, ACS Nano., 2011, 5, 10033–10040.10.1021/nn2038725
- T. K. Stevik, K. Aa, G. Ausland and J. F. Hanssen: ‘Retention and removal of pathogenic bacteria in wastewater percolating through porous media: a review’, Water Res., 2004, 38, 1355–1367.10.1016/j.watres.2003.12.024
- M. F. Ottaviani, P. Favuzza, M. Bigazzi, N. J. Turro, S. Jockusch and D. A. Tomalia: ‘A TEM and EPR investigation of the competitive binding of uranyl ions to starburst dendrimers and liposomes: Potential use of dendrimers as uranyl ion sponges’, Langmuir, 2000, 16, (19), 7368–7372.10.1021/la000355w
- E. R. Birnbaum, K. C. Rau and N. N. Sauer: ‘Selective anion binding from water using soluble polymers’, Sep. Sci. Technol., 2003, 38, (2), 389–404.
- C. Warwick, A. Guerreiro and A. Soares: ‘Sensing and analysis of soluble phosphates in environmental samples: A review’, Biosens. Bioelectro., 2013, 41, 1–11.10.1016/j.bios.2012.07.012
- X. Lin, X. Wu, X. Zenghong and K.-Y. Wong: ‘PVC matrix membrane sensor for fluorescent determination of phosphate’, Talanta, 2006, 70, 32–36.10.1016/j.talanta.2006.01.026
- S. Glazier and M. Arnold: ‘Phosphate-selective polymer membrane electrode’, Anal. Chem., 1988, 60, 2540–2542.10.1021/ac00173a024
- C. Kaittanis, S. A. Naser and J. M. Perez: ‘One-step, nanoparticle-mediated bacterial detection with magnetic relaxation’, Nano Lett., 2007, 7, 380–383.10.1021/nl062553z
- S. Ameen, M. S. Akhtar and H. S. Shin: ‘Hydrazine chemical sensing by modified electrode based on in situ electrochemically synthesized polyaniline/graphene composite thin film’, Sens. Actuators B, 2012, 173, 177–183.10.1016/j.snb.2012.06.065
- B. Kumar, J.-F. Feller, M. Castro and J. Lu: ‘Conductive bio-polymer nano-composites (CPC): Chitosan-carbon nanotube transducers assembled via spray layer-by-layer for volatile organic compound sensing’, Talanta, 2010, 81, 908–915.10.1016/j.talanta.2010.01.036
- M. Cerruti, J. Jaworski, D. Raorane, C. Zueger, J. Varadarajan, C. Carraro, S.-W. Lee, R. Maboudian and A. Majumdar: ‘Polymer-oligopeptide composite coating for selective detection of explosives in water’, Anal. Chem., 2009, 81, 4192–4199.10.1021/ac8019174
- A. Shirzadmehr, A. Afkhami and T. Madrakian: ‘A new nano-composite potentiometric sensor containing an Hg2+-ion imprinted polymer for the trace determination of mercury ions in different matrices’, J. Mol. Liq., 2015, 204, 227–235.10.1016/j.molliq.2015.01.014
- R. Verma and B. D. Gupta: ‘Detection of heavy metal ions in contaminated water by surface plasmon resonance based optical fibre sensor using conducting polymer and chitosan’, Food Chem., 2015, 166, 568–575.10.1016/j.foodchem.2014.06.045
- J. E. Lee, H. W. Shim, O. S. Kwon, Y.-I. Huh and H. Yoon: ‘Real-time detection of metal ions using conjugated polymer composite papers’, Analyst, 2014, 139, 4466.10.1039/C4AN00804A
- X. H. Zhao, R. M. Kong, X. B. Zhang, H. M. Meng, W. N. Liu, W. H. Tan, G. L. Shen and R. Q. Yu: ‘Graphene–DNAzyme based biosensor for amplified fluorescence “turn-on” detection of Pb2+ with a high selectivity’, Anal. Chem., 2011, 83, (13), 5062–5066.10.1021/ac200843x
- C. Cugnet, O. Zaouak, A. René, C. Pécheyran, M. Potin-Gautier and L. Authier: ‘A novel microelectrode array combining screen-printing and femtosecond laser ablation technologies: Development, characterization and application to cadmium detection’, Sens. Actuators B, 2009, 143, 158–163, DOI: 10.1016/j.snb.2009.07.059.
- M. A. R. Tehrania, H. Ghadimi and S. Ab Ghani: ‘Electrochemical studies of two diphenols isomers at graphene nanosheet–poly(4-vinyl pyridine) composite modified electrode’, Sens. Actuators B, 2013, 177, 612–619.10.1016/j.snb.2012.11.047
- A. C. Roy, V. S. Nisha, C. Dhand, M. A. Ali and B. D. Malhotra: ‘Molecularly imprinted polyaniline-polyvinyl sulphonic acid composite based sensor for para-nitrophenol detection’, Anal. Chim. Acta, 2013, 777, 63–71.10.1016/j.aca.2013.03.014
- X. Wang, X. Li, C. Luo, M. Sun, L. Li and H. Duan: ‘Ultrasensitive molecularly imprinted electrochemical sensor based on magnetism graphene oxide/β-cyclodextrin/Au nanoparticles composites for chrysoidine analysis’, Electrochim. Acta, 2014, 130, 519–525.10.1016/j.electacta.2014.03.039
- M. Mathew, S. Sureshkumar and N. Sandhyarani: ‘Synthesis and characterization of gold–chitosan nanocomposite and application of resultant nanocomposite in sensors’, Colloids Surf. B, 2012, 93, 143–147.10.1016/j.colsurfb.2011.12.028
- M. A. Chamjangali, H. Kouhestani, F. Masdarolomoor and H. Daneshinejad: ‘A voltammetric sensor based on the glassy carbon electrode modified with multi-walled carbon nanotube/poly(pyrocatechol violet)/bismuth film for determination of cadmium and lead as environmental pollutants’, Sens. Actuators B, 2015, 216, 384–393.10.1016/j.snb.2015.04.058
- S. Palanisamy, K. Thangavelu, S.-M. Chen, V. Velusamy, M.-H. Chang, T.-W. Chen, F. M. A. Al-Hemaid, M. A. Ali and S. K. Ramaraj: ‘Synthesis and characterization of polypyrrole decorated graphene/β-cyclodextrin composite for low level electrochemical detection of mercury (II) in water’, Sens. Actuators B, 2017, 243, 888–894.10.1016/j.snb.2016.12.068
- Y. Liang, L. Yu, R. Yang, X. Li, L. Qu and J. Li: ‘High sensitive and selective graphene oxide/molecularly imprinted polymer electrochemical sensor for 2,4-dichlorophenol in water’, Sens. Actuators B, 2017, 240, 1330–1335.10.1016/j.snb.2016.08.137
- M. H. M. Facure, L. A. Mercante, L. H. C. Mattoso and D. S. Correa: ‘Detection of trace levels of organophosphate pesticides using an electronic tongue based on graphene hybrid nanocomposites’, Talanta, 2017, 167, 59–66.10.1016/j.talanta.2017.02.005
- D. Sahu, N. I. Sarkar, G. Sahoo, P. Mohapatra and S. K. Swain: ‘Nano silver imprinted polyvinyl alcohol nanocomposite thin films for Hg2+ sensor’, Sensors Actuators B, 2017, 246, 96–107.10.1016/j.snb.2017.01.038
- Z. Zhou, T. Li, W. Xu, W. Huang, N. Wang and W. Yang: ‘Synthesis and characterization of fluorescence molecularly imprinted polymers as sensor for highly sensitive detection of dibutyl phthalate from tap water samples’, Sens. Actuators B, 2017, 240, 1114–1122.10.1016/j.snb.2016.09.092
- Y. Ma, S. Xu, S. Wang and L. Wang: ‘Luminescent molecularly-imprinted polymer nanocomposites for sensitive detection’, TrAC Trends Anal. Chem., 2015, 67, 209–216.10.1016/j.trac.2015.01.012
- S. J. Patil, N. Duragkar and V. R. Rao: ‘An ultra-sensitive piezoresistive polymer nano-composite microcantilever sensor electronic nose platform for explosive vapor detection’, Sens. Actuators B, 2014, 192, 444–451.10.1016/j.snb.2013.10.111
- I. Langmuir: ‘The constitution and fundamental properties of solids and liquids’, J. Am. Chem. Soc., 1916, 38, 2221–2295.10.1021/ja02268a002
- H. Freundlich: ‘Über die adsorption in läosungen’, Z. Phys. Chem., 1906, 57, 385–470.
- M. M. Dubinin and L. V. Radushkevich: ‘Equation of the characteristic curve of activated charcoal’, Proc. Acad. Sci. USSR, 1947, 55, 331–333.
- M. I. Temkin and V. Pyzhev: ‘Kinetics of ammonia synthesis on promoted iron catalyst’, Acta Physicochim, 1940, 12, 327–356.
- S. Brunauer, P. H. Emmett and E. Teller: ‘Adsorption of gases in multimolecular layers’, J. Am. Chem. Soc., 1938, 60, 309–319.10.1021/ja01269a023
- G. Z. Kyzas and K. A. Matis: ‘Nanoadsorbents for pollutants removal: A review’, J. Mol. Liq., 2015, 203, 159–168.10.1016/j.molliq.2015.01.004
- A. K. Bhattacharya and C. Venkobachar: ‘Removal of cadmium (II) by low cost adsorbents’, J. Environ. Eng. ASCE, 1984, 110, 110–122.10.1061/(ASCE)0733-9372(1984)110:1(110)
- K. G. Varshney, A. A. Khan, U. Gupta and S. M. Maheshwari: ‘Kinetics of adsorption of phosphamidon on antimony (V) phosphate cation exchanger: evaluation of the order of reaction and some physical parameters’, Colloids Surf. A, 1996, 113, 19–23.10.1016/0927-7757(96)03546-7
- M. Kumari, C. U. Pittman Jr and D. Mohan: ‘Heavy metals [chromium (VI) and lead (II)] removal from water using mesoporous magnetite (Fe3O4) nanospheres’, J. Colloid. Interface Sci., 2015, 442, 120–132.10.1016/j.jcis.2014.09.012
- S. Lagergren: ‘About the theory of so-called adsorption of soluble substances’, Handlingar, 1898, 24, 1–39.
- G. Blanchard, M. Maunaye and G. Martin: ‘Removal of heavy metals from waters by means of natural zeolites’, Water Res., 1984, 18, 1501–1507.10.1016/0043-1354(84)90124-6
- C. Ho and S. J. Slater: ‘Conformation of the C1 phorbol-ester-binding domain participates in the activating conformational change of protein kinase C’, Biochem. J., 1999, 344, 451–460.10.1042/bj3440451
- S. H. Chien and W. R. Clayton: ‘Application of Elovich equation to the kinetics of phosphate release and sorption in soils’, Soil Sci. Soc. Am. J., 1980, 44, 265–268.10.2136/sssaj1980.03615995004400020013x
- D. L. Sparks: ‘Kinetics of reaction in pure and mixed systems’ in ‘Soil Physical Chemistry’, (ed. D. L. Sparks), 63–145; 1986, Boca Raton, FL, CRC Press.
- G. McKay and V. J. P. Poots: ‘Kinetics and diffusion processes in colour removal from effluent using wood as an adsorbent’, J. Chem. Biotechnol., 1980, 30, 279–292.
- W. J. Weber and J. C. Morris: ‘Kinetics of adsorption on carbon from solution’, J. Sanit. Eng. Div. Am. Soc. Civ. Eng., 1963, 89, 31–59.
- Y. S. Ho and G. McKay: ‘A comparison of chemisorption kinetic models applied to pollutant removal on various sorbents’, Trans Icheme, 1998, 76, 332–340.10.1205/095758298529696
- L. Ai, Y. Zhou and J. Jiang: ‘Removal of methylene blue from aqueous solution by montmorillonite/CoFe2O4 composite with magnetic separation performance’, Desalination, 2011, 266, 72–77.10.1016/j.desal.2010.08.004
- B. H. Hameed and A. A. Ahmad: ‘Batch adsorption of methylene blue from aqueous solution by garlic peel, an agricultural waste biomass’, J. Hazard. Mater., 2009, 164, 870–875.10.1016/j.jhazmat.2008.08.084
- S. Arivoli, B. R. Venkatraman, T. Rajachandrasekar and M. Hema: ‘Adsorption of ferrous ion from aqueous solution by low cost activated carbon obtained from natural plant material’, Res. J. Chem. Environ., 2007, 17, 70–78.