ABSTRACT
Context: Ozone concentrations near the land surface are rising in Asia while they are declining or stagnating in Europe and North America. Ozone is the most widespread air pollutant negatively affecting vegetation, and its increased concentrations pose a major threat to food quality and production and other ecosystem services in Asia.Method: In this review, we provide an overview of scientific challenges in the impacts of ozone pollution on Asian vegetation, and synthesize the challenges toward mitigation of the impacts.Result: We argue that new policy initiatives need to seek both reduction of ozone levels and enhancement of plant tolerance to ozone to maintain food quality and ensure food supplies.Conclusion: The scientific advancements must be transferred to actions by two types of institutions: a) environmental agencies for reducing ozone levels and b) agricultural research institutions for enhancing plant tolerance to ozone. In connecting the scientific advancements with the institutional actions, scientists in Asian countries should play the key role taking advantages of interdisciplinary and international collaborations.
Introduction
Surface ozone (O3) level is rising over East (Ma et al. Citation2016), South (Lal et al. Citation2012), and Southeast (Assareh et al. Citation2016) Asia, following the rapid economic growth and urbanization. Ozone is a secondary pollutant formed in the atmosphere by sunlight-driven chemical reactions between the precursor gases including nitrogen oxides (NOx) and non-methane volatile organic compounds (NMVOCs) (The Royal Society Citation2008). As of 2014, Asia is the world’s largest emitter of the two precursors: China emits 30% of NOx and 19% of NMVOCs, followed by India’s emissions of 13% (NOx) and 11% (NMVOCs) of the global emissions (Hoesly et al. Citation2018) (). Across 30 years from 1980, major contributors to global tropospheric O3 have shifted from the developed countries in mid- and high-latitudes of the northern hemisphere to the developing countries in lower latitudes of South, Southeast, and East Asia (Zhang et al. Citation2016).
Figure 1. Regional contributions to anthropogenic emissions of nitrogen oxides (NOx) and non-methane volatile organic compounds (NMVOCs) as of 1990 and 2014. Data were extracted from Hoesly et al. (Citation2018). NOx emissions are expressed on NO2 basis. NMVOCs do not include biogenic VOCs (BVOCs)
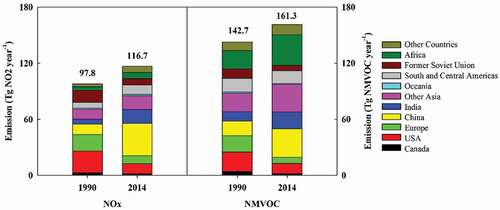
Ozone is the most widespread air pollutant threatening agricultural production, biodiversity, and ecosystem services more than any other air pollutant at large spatial scales from national and regional to the entire globe (Tang et al. Citation2013; Anav et al. Citation2016; Mills et al. Citation2018b; Agathokleous et al. Citation2020; Sicard et al. Citation2020; Xu Citation2021). It is also the second most important air pollutant negatively affecting human health after particulate matter (Brauer et al. Citation2016; Zhang, Wei, and Fang Citation2019; Orru et al. Citation2019). The extent of the negative impacts on major crops at current O3 levels is estimated to be comparable to other major stressors including pests and diseases in many major agricultural areas, including East and South Asia (Mills et al. Citation2018b). In addition to O3, Asian vegetation is subjected to atmospheric aerosols (Yue et al. Citation2017) and acid rain/nitrogen deposition (Liu et al. Citation2013; Sase Citation2017). The increasing impacts of O3 along with the other air pollutants could threaten food security and thereby human nutrition for the still increasing population in Asia, where 60% of the global population resides and major fractions of world’s main crop yield are produced, e.g., 90.3% of rice, 43.8% of wheat, and 31.5% of maize (FAOSTAT: http://www.fao.org/faostat/en/#data (last access 21 May 2020); based on the period 2014–2018). Considering that ecosystem can be defined as “a dynamic complex of plant, animal, and microorganism communities and the nonliving environment interacting as a functional unit” with humans being an integral part of it (Millennium Ecosystem Assessment Citation2003), the preceding discussion suggests that O3 pollution can impact ecosystem health.
Against the challenges of increasing air pollution impacts on vegetation in Asia, concerned scientists have gathered at the Asian Air Pollution Workshop (www.aapw.net.cn) annually from 2015 to 2019. Participants from diverse disciplines including air chemistry, ecology, plant physiology, and soil science shared findings, exchanged opinions, and discussed future collaborations for mitigating the negative impacts of air pollution. Throughout the discussions, the participants have identified current status and future challenges of scientific research in air pollution impacts on vegetation in Asia. Hence, a need has emerged to synthesize the result of this scientific activity with the aim to present the O3 impacts within the socio-environmental context of Asia, and to set out the roles of scientists in reducing O3 damages to Asian vegetation.
In this article, we give an overview of progresses and challenges in the science of O3 pollution impacts in Asia from three aspects: 1. Monitoring, modeling and assessment, 2. Effects of O3 on plant metabolism and how this threatens food quality and quantity for human and animal consumptions, and 3. Plant contribution to O3 pollution. We then synthesize the challenges toward mitigation of the O3 impacts in Asia. While the article concerns Asia, the discussion is based on available scientific studies, and not all Asian countries have any or sufficient studies on O3 pollution impacts. Therefore, the article is focused on countries for which there is sufficient available scientific information, e.g., China, India, and Japan. Furthermore, the article is not directed to discuss technical information covered in existing publications (Yamaguchi et al. Citation2011; Koike et al. Citation2013; Oksanen et al. Citation2013; Izuta Citation2017; Mukherjee et al. Citation2021; Xu Citation2021). It is directed to provide to policymakers, scientists working in different research areas, and other stakeholders an integrated synthesis of the issue in Asia, so to translate scientific knowledge into practical actions aiming at protecting the health of both natural ecosystems and managed agroecosystems.
Monitoring, modeling and assessment of ozone impacts in Asia
Monitoring of ozone pollution
International monitoring networks of air pollution in Asia are young and less developed compared to those in the U.S. and Europe. In East Asia, the Acid Deposition Monitoring Network (EANET: http://www.eanet.asia) started regular measurements of air pollution and ecological impacts in 2001. As of 2018, O3 was measured at 25 sites in seven countries. In South Asia, the “Malé Declaration on Control and Prevention of Air Pollution and its likely Transboundary Effects for South Asia” was initiated in 1998, and the monitoring network was established in 2003 (http://www.rrcap.ait.asia/male). A total of 15 sites from 8 countries are operated for monitoring O3 and other pollutants.
National networks have also been built and operated in some countries. For example, the TOAR (Tropospheric O3 Assessment Report) database accommodates O3 monitoring data at 1260 sites in Japan, 312 sites in South Korea, but only 26 in China (Schultz et al. Citation2017). For South Asia, 8 datasets were aggregated in the TOAR database. With these existing data, however, spatial coverage is clearly insufficient in Asia, as shown by the wide gaps of monthly mean O3 concentrations over Asia on the 5° × 5° grids (Fig. 6 of Schultz et al. (Citation2017)).
However, the situation for China has been drastically improved since 2013, when the Ministry of Environmental Protection of China started publicizing hourly data of major air pollutants including O3 at 1497 monitoring stations (Li et al. Citation2018a). In India, a project-based monitoring network for O3 was started with a few sites in 2008, and the site number grew to 14 in 2015 (Lal et al. Citation2017). However, a nation-wide monitoring network of air pollution (https://app.cpcbccr.com/ccr/#/caaqm-dashboard-all/caaqm-landing) has been developed in India since 2017.
Although these networks serve as foundations for regional air pollution assessment, the lack of uniform criteria and the limitation in spatio-temporal coverage reduce the effectiveness of using their data. Most monitoring stations are located in urban areas, while the stations covering rural and remote forested areas are limited. In addition, most of these networks are not integrated, and associated variables (e.g., meteorology, chemistry, and vegetation) are not often observed simultaneously.
Modeling of ozone pollution and its impacts
Models are useful tools to interpret monitoring data and perform large-scale assessment. However, because of the simplifications and assumptions in the process of modeling, model outputs are confronted with uncertainties from parameterizations, physical processes, and boundary conditions. In air quality modeling, large uncertainties exist due to the discrepancies between the models in model parameters, physical processes, and emission inventories.
Models of O3 impacts depend on empirical approaches, which characterize the O3 level as various dose metrics (Agathokleous, Kitao, and Kinose Citation2018). Some metrics are weighed means or sums of concentrations across a specified period, while others measure the amount of O3 absorbed by plants (Lefohn et al. Citation2018). The dose is then used as an indicator of the risks to vegetation (Tang et al. Citation2014; Mills et al. Citation2018a; Li et al. Citation2018a) or converted to damages (e.g., loss of crop yield or lower biomass production) using dose-response relationships (Feng et al. Citation2012, Citation2019a). The dose–response relationships have been derived from experiments conducted under manipulated O3 concentrations in facilities mimicking the real-world environment (Feng, Tang, and Kobayashi Citation2017). It was found, however, that the dose–response relationships differ between different types of facilities (Feng et al. Citation2018c; Agathokleous et al. Citation2019a). Uncertainties may thus arise from the dose metrics and dose–response relationships (Agathokleous et al. Citation2019b).
Recently, semi-mechanistic schemes and process-based analyses are emerging for impact modeling (Tian et al. Citation2016; Yue et al. Citation2017). Crop yield losses are modeled with explicit description of growth processes in response to O3 and other environmental changes (Tao et al. Citation2017; Emberson et al. Citation2018). Despite these progresses, uncertainties abound in the modeling of pollution impacts due to the diversity of model structures and processes included.
Assessment of risks and impacts due to ozone pollution
Studies conducted in Asia have shown significant crop losses due to O3 at the present levels (Ghude et al. Citation2014; Lal et al. Citation2017; Lin et al. Citation2018; Feng et al. Citation2019a). In India, national harvest losses were estimated at 5% for wheat and 2% for rice in one study (Ghude et al. Citation2014), whereas, in another study, they were in the ranges from 4.2% to 15% for wheat and from 0.3% to 6.3% for rice (Lal et al. Citation2017). Tang et al. (Citation2013) also estimated the national harvest loss of wheat due to O3 in the range from 8% to 22% for India, while from 6% to 15% for China. A study on national harvest losses in China estimated the ranges from 21% to 39% for wheat and from 7% to 15% for rice (Lin et al. Citation2018). More recently, a meta-analytic assessment suggested that Indian major crops rank wheat > mustard > rice > maize in terms of yield sensitivity to ambient O3, whereas yield sensitivity to elevated O3 varied with factors such as intrinsic defense response, exposure/dose, and stomatal flux (Mukherjee et al. Citation2021). Uncertainties thus abound in the estimated O3 impacts. The harvest losses showed wide ranges even within the individual studies (Tang et al. Citation2013; Lal et al. Citation2017; Lin et al. Citation2018) due to the difference between the dose–response relationships. Some of the relationships were derived from experiments conducted in the same region, whereas others were introduced from Europe or the US. In addition, differences between the air pollution models and emission inventories should have contributed to the large difference between the studies. Even with the same air pollution model, an overestimation of the impact was found when the model outputs were used without the correction for the steep decline in O3 level near the plant canopy top (Tang et al. Citation2013).
The uncertainties as noted above have significant implications to the estimates of O3-induced economic losses in agriculture. Some studies converted the mass-based yield losses to economic losses with the fixed market price and harvested area (Feng et al. Citation2019a), whereas other studies (e.g., Yi et al. (Citation2018)) used the yield losses as an input to an economic model allowing other variables, e.g., market price, to vary. In either way of estimation, the uncertainties in the yield loss estimate will lead to uncertainties in the economic loss estimate.
In addition to the impacts on quantity of harvested crops, O3 changes their quality. Protein concentration, for example, is commonly increased by O3 across species (Wang and Frei Citation2011), whereas the amount of protein harvested in wheat is reduced because the crop harvest is reduced to a greater extent than the increase of protein concentration (Broberg et al. Citation2015). It would therefore be misleading to interpret the higher protein concentration in crops grown in elevated O3 level as an improvement in human nutrition. It is noteworthy that O3 reduces digestibility of forage crops and crop residues for ruminant animals (Wang and Frei Citation2011).
Recently, a new regional high-resolution chemical transport model was run over Asia to determine the potential O3 risk to forests in Asia (De Marco et al. Citation2020). The authors estimated different concentration-based and dose-based O3 metrics, which showed different spatial distribution and exceedance extent. A high potential of O3 impacts on deciduous forest growth in Asia was found, while potential O3 impacts on evergreen forest types were lower. Most of evergreen forests (86–97%) were potentially exposed to O3 concentrations exceeding the limits for forest protection, while the percentage of evergreen forests exposed to O3 doses above the critical levels was lower (12–46%). This study suggests that Asian studies should focus on dose-based O3 metrics to provide relevant bases for developing proper standards.
Challenges for monitoring, modeling and assessment
Monitoring data from the existing networks need to be synthesized, while monitoring must be expanded to under-investigated areas, e.g., South Asia. The ground-based monitoring networks could be combined with model simulations to enhance spatial and temporal coverage. The monitoring data shall be valuable in validating the air pollution models, which would be used to estimate surface O3 over Asia to provide high-resolution inputs for the impact assessments. Application of ensemble predictions with multiple models and emission inventories shall be useful in reducing uncertainties in the prediction of O3 pollution.
The impact models need to be developed for Asian genotypes, as demonstrated by the greater susceptibility to O3 in Asian crop varieties than North American (Emberson et al. Citation2009) and European (Feng et al. Citation2012) counterparts. The progresses toward the process-based approaches will facilitate the impact models to account for the effects of other interacting variables, e.g., CO2 concentration and soil moisture. The model uncertainties could be reduced by verifications with observations in the field, for which O3-FACE (free-air concentration elevation of O3) shall be valuable (Oue et al. Citation2011; Kitao et al. Citation2015).
Effects of ozone on plant metabolism
Most O3 impact studies with Asian plants have focused on growth responses, stomatal regulation, and photosynthesis (Izuta Citation2017), while there is a growing number of studies on biochemical, metabolomic, proteomic or transcriptomic responses (Tsukahara et al. Citation2015; Zhang et al. Citation2017). Plants respond to O3 by adjusting the production of primary metabolites (mainly sugars, organic acids, amino acids) directly involved in growth and developmental processes. In addition, secondary metabolites (a vast group of different organic compounds such as phenolics) are mediating plant acclimation to stressful conditions (). Several of these primary and secondary metabolites are essential components of the plant defense systems. If changes in the biosynthetic pathways of such metabolites are likely, omic studies would greatly help to reveal the cellular and molecular mechanisms for plant responses to environmental stresses.
Figure 2. Plant responses to ozone at various scales. ASA: reduced ascorbate; Asc/Glu: ascorbate/glutathione cycle; PS: photosynthesis; Metab. Ir and IIr: primary and secondary metabolites; ROS: reactive oxygen species, e.g., H2O2, OH.
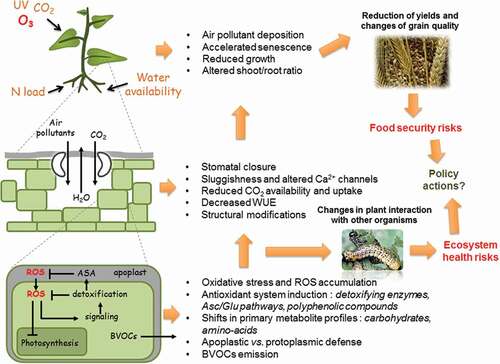
Variation in ozone susceptibility/tolerance between crop species and varieties
A wide variation in O3 susceptibility/tolerance has been recorded both among and within crop species in Asia. Most studies have been conducted with one or a few varieties, highlighting the requirement for a large-scale screening for genetic variability (Oksanen et al. Citation2013) as is the recent case of a screening of 40 Indian cultivars of Amaranthus hypochondriacus for O3 tolerance/susceptibility (Yadav, Mina, and Bhatia Citation2020). For example, large genetic variability in O3 susceptibility has been found among 18 cultivars of rice and 11 cultivars of wheat in India (Pandey et al. Citation2015, Citation2019), and among 15 cultivars of wheat and 19 cultivars of soybean in China (Jiang et al. Citation2018; Feng et al. Citation2018b). In all studies, the genetic variability in O3 susceptibility was associated with activities in antioxidant systems, especially those involving superoxide dismutase, catalase, glutathione and ascorbate. The antioxidant systems could therefore be a target of interest for engineering O3-tolerant crops, e.g., rice (Frei Citation2015).
Antioxidant systems have been well listed but are yet to be better understood regarding the high complexity in their spatial, temporal, and chemical processes (Wang et al. Citation2015). With this aim, the contributions of the defense systems in the cytosol and cell wall to O3 tolerance in Asian species have been of particular interest (Feng et al. Citation2010), but still remain unclear (Wang et al. Citation2015; Dai et al. Citation2020). Some research groups working on defense metabolism under O3 stress attempted to improve current knowledge about the genetic and molecular basis of ascorbate-glutathione pathways (Zhang et al. Citation2017), the different enzymatic isoforms involved and their respective compartments (Rahantaniaina et al. Citation2017), other antioxidants within secondary metabolism, such as phenolics (Li et al. Citation2021), or specific defense structures, such as glandular trichomes (Li et al. Citation2018b). However, these efforts are still at the initial stages and, thus, the understandings are yet to be developed.
Metabolism in tree species under O3-induced stress
Asian trees have gained less attention in molecular and metabolite researches compared with the crop species. In Japan, Japanese beech (Fagus crenata) has been most intensively studied regarding the metabolism under O3 pollution (Yamaguchi et al. Citation2011; Koike et al. Citation2013). A meta-analysis revealed that temperate woody species from China were more sensitive to O3 than those from North America and Europe in terms of gas exchange (Li et al. Citation2017). Evergreen species are generally more tolerant to O3 than deciduous species (Li et al. Citation2017; Feng et al. Citation2018a; Novriyanti et al. Citation2021), which can be attributed to the higher leaf mass per area (LMA) in evergreen species (Li et al. Citation2016; Feng et al. Citation2018a). Higher LMA is associated with higher tolerance to oxidative stress (Bussotti Citation2008).
Threats to food quality and quantity for humans and other animals arising from O3 effects on plant metabolism
As a result of the negative effects of chronic O3 pollution on plant metabolism, productivity and yields can be highly decreased, including in Asia (see Assessment of risks and impacts due to ozone pollution) . These suggest that food quantities in Asia may be threatened by O3 pollution. However, not only food quantity but also food quality may be threatened by O3 pollution. That O3 pollution can affect plant metabolism and biochemistry can be critical when it comes to the quality of food for human and other animal consumption. Imbalance in plant stoichiometry and potential reduction of the overall mineral concentration due to air pollution may affect the quality of human diet (Loladze Citation2002, Citation2014). Studies conducted in Japan, using insects as animal models, showed that the behavior and physiology of larvae and beetles of different insect species can be affected when fed with leaves affected by environmental stresses, such as O3 (Agathokleous et al. Citation2017, Citation2019c; Abu Elela, Agathokleous, and Koike Citation2018). These findings are also supported by studies conducted in other parts of the world showing effects of O3-stressed plant tissues to animal consumers (see Blande (Citation2021) and Masui et al. (Citation2021) for additional mechanisms driving plant-insect interactions). For example, decreased digestibility was found in rabbits (Oryctolagus cuniculus) fed with forage (a mixture of grassland species common in Southern Piedmont, USA) exposed to O3 concentrations twice the ambient (Gilliland et al. Citation2012). These suggest a potential threat to the quality of the diet of animals whose nutrition depends upon plants grown in O3-polluted areas.
Challenges for investigating plant metabolism under elevated O3
Novel technologies in genomics, proteomics and metabolomics must be fully used in Asia to reveal the molecular mechanisms of tolerance against O3. Study of defensive metabolites should be related to plant hormones and cell signaling, and extended to special structures, e.g., glandular trichomes, that are active in storing and secreting various secondary metabolites related to defense against adverse environment (Li et al. Citation2018b; Oksanen Citation2018; Karabourniotis et al. Citation2019). The detoxification capacity could also be related to the internal anatomical mechanism such as high LMA. The quantitative and qualitative understandings of the tolerance mechanisms must be integrated into the models of O3 pollution impacts.
Plant contribution to ozone pollution in Asia
Plants are not only affected by O3 but contribute to O3 pollution by emitting biogenic volatile organic compounds (BVOCs): isoprene, monoterpenes, and other VOCs.
Vegetation as a source of BVOCs
BVOCs play a key role in the formation of O3. This is because their reactivity is much higher than the anthropogenic NMVOCs (Atkinson and Arey Citation2003), and their estimated annual emission rate of 80 Tg y−1 (Fu et al. Citation2007) in Asia is as large as that of anthropogenic NMVOCs from Asia (78 Tg y−1 in ). Nevertheless, the estimate of BVOC emissions in Asia has large uncertainties. For example, a comparison between the estimates for BVOC emissions from the whole China in the studies after 2000 exhibits a sixfold difference between the lowest and highest estimates ().
Figure 3. Emissions of biogenic volatile organic compounds (BVOCs) in China at national level as estimated by various studies. ISO: isoprene, MONs: monoterpenes, OVOCs: other BVOCs. Anthropogenic emissions of non-methane VOCs (NMVOCs) are also shown by the range of estimates for years from 2004 onward. References are arranged in chronological order of publication from the left to the right. MONs and OVOCs are combined in the reference 10. See Table S1 for details
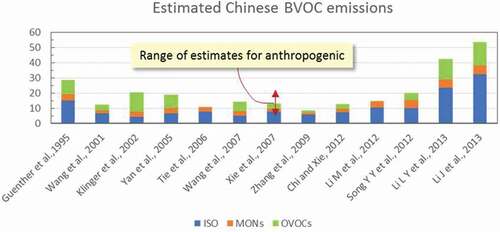
Among the many factors contributing to this uncertainty, a major contributor is the limited standardization of the emission rate in Asian native species. Many studies have been conducted on BVOCs emission rates in numerous species in China, Japan, and South Korea. For example, data collection from 411 species in China revealed that 88.9% of the studies species emit isoprene, 11.1% emit monoterpenes, and 6.6% emit both (Feng, unpublished data). Generally, broad-leaved species display high isoprene emission rates, especially Populus (most common poplar in China: P. euramericana cv. ‘74/76ʹ), Platanus occidentalis, Robinia pseudoacacia, and Salix badylonica, while coniferous species emit high rates of monoterpenes, such as Platycladus orientalis, Pinus griffithii, and Pinus armandii (Jing et al. Citation2020; Xu et al. Citation2020). However, these studies often lack uniform criteria and information needed for standardizing the emission rates, limiting a complete understanding of the BVOCs roles in local air quality (Wei et al. Citation2007) and modeling at continental and global scales (Sindelarova et al. Citation2014).
Contribution of BVOCs emissions to ozone levels
Contribution of BVOCs emissions to O3 levels is less studied in Asia than in Europe and North America (Duan et al. Citation2020). In China, the increase in O3 concentration by BVOCs is generally five ppb or less in Pearl River Delta (Wei et al. Citation2007) and the whole eastern China (Han, Ueda, and Matsuda Citation2005; Wang et al. Citation2008), whereas in Yangtze River Delta region, the contribution could be more than 10 ppb or even reach 18 ppb (Liu et al. Citation2018). High BVOCs-induced O3 increases were estimated also for the Beijing-Tianjin-Hebei and Sichuan Basin regions, with increases of up to 24 ppb due to the sensitivity of VOC-limited urban areas (Wu et al. Citation2020). In Japan, an increase of up to 6 ppb was found in monthly mean O3 concentrations due to BVOCs emissions indicating the strong influences of forest vegetation on O3 generation (Bao et al. Citation2010); however, the contributions of BVOCs remain uncertain in other Asian countries.
Responses of BVOCs emissions to rising ozone levels
Emissions of BVOCs could respond to environmental changes (biotic and abiotic), including O3 (Peñuelas and Staudt Citation2010; Heil Citation2014). In Asia, only a few studies have been conducted, and reported inconsistent results. Chronic exposure to higher O3 increased isoprene and monoterpene emissions in Ginkgo biloba trees (Li et al. Citation2009) and Pinus tabulaeformis (Xu et al. Citation2012), while it reduced isoprene emission from two Quercus species (Tani et al. Citation2017) and two hybrid poplars (Yuan et al. Citation2017). Monoterpenes emissions from hybrid larch were not affected by O3 (Mochizuki et al. Citation2017). A summary of 34 studies conducted in Europe and the US also showed conflicting responses of BVOCs emissions to higher O3 (Peñuelas and Staudt Citation2010). There were, nevertheless, more studies reporting significant decreases in isoprene emission than increases, and more studies reporting significant increases in monoterpenes emissions than decreases. Similarly, a recent meta-analysis indicated that isoprene is more sensitive to O3 and other environmental factors (e.g., elevated CO2 or drought) than monoterpenes (Feng et al. Citation2019b).
Challenges for investigating plant contribution to ozone pollution
Quantification of standard emission factors from native and introduced plant species in Asia must be expanded to reduce the uncertainties in the estimates of BVOCs emissions in Asia and to allow an optimal choice of low O3-forming-potential species for urban greening. The responses of BVOCs emissions to O3 and other environmental changes warrant further studies to unravel how BVOCs emissions will respond to the changing earth system. Field observations and model estimations should be combined to improve the BVOCs estimates and provide scientific guidance for O3 pollution control.
Toward mitigation of ozone impacts on plants and ecosystems in Asia
An ultimate goal of air pollution research is to reduce the negative impacts of air pollution. To this end, two approaches are available: (i) to lower the O3 levels and (ii) to lower the susceptibility of target vegetation to O3. We argue that, in Asia, both approaches must be pursued in a coordinated effort of policymakers and scientists.
Lowering ozone levels
Lowering O3 concentration by emission control can provide protection from the O3 pollution impacts to all the organisms in the target regions. A good example is the success of air quality management in Europe under the framework of the 1979 Convention of Long-range Transboundary Air Pollution (CLRTAP). Under CLRTAP, NOx emission in Europe was roughly halved since 1990, and sulfur emission was reduced by about 80% in the same period (Maas and Grennfelt Citation2016).
In Asia, however, the emission control shall be more challenging than that in Europe. The major source of the O3 precursor NOx is energy production, which will increase with the economic growth in Asia. China is the good example, and India is following suit (). Other populous countries, e.g., Indonesia, Pakistan, Bangladesh, Vietnam, are also on track of the economic growth. In 2010, China started to implement the clean air policies, and it is estimated that the emissions have been reduced by 35% for PM2.5 and 17% for NOx through to 2017 (Zheng et al. Citation2018). This cut in primary pollutant emission clearly is an encouraging development for human health, although there are still nationwide long-term challenges to attain air quality standards (Wang Citation2021). For protection of vegetation, it could also be good news but to a less extent, since emissions of the other precursor: NMVOC, have increased by 11% for the same period. Therefore, the trends in surface O3 level and its impacts on vegetation are yet to be investigated.
Against the increasing risk of O3 pollution on vegetation in Asia, we need to seek other approaches as well.
Lowering the susceptibility of vegetation to ozone
Ozone affects plants after being absorbed via stomata in the leaves (). It is therefore possible to lower the O3 impacts by reducing the gas uptake. Indeed, the faster leaf gas exchange and thereby O3 uptake has been assumed to be the main cause of higher susceptibility in modern soybean varieties to O3 than the older varieties (Osborne et al. Citation2016). It follows that lower gas exchange rate could be a desirable trait for crop varieties under high levels of O3, as argued for maize under water constraint, where lower-transpiration trait would benefit the crop yield (Messina et al. Citation2015).
Ozone uptake could also be reduced by partial stomatal closure induced by reduced irrigation, which could also save irrigation water. In rice-growing countries, alternate wetting and drying irrigation has become popular in an attempt to reduce water usage (Carrijo, Lundy, and Linquist Citation2017), but it would reduce the O3 pollution impacts as an unintended benefit (Mills et al. Citation2018b). Antitranspirants could also alleviate O3 impacts and water use via reduced gas exchange (Agathokleous et al. Citation2016). The high O3 peaks at a sensitive timing of the plant biological life cycle can be avoided by shifting cropping calendar. Such an avoidance measure may be effective in some combinations of location and crop species, if not at global scale (Teixeira et al. Citation2011).
Besides the reduced uptake of O3, the impact can also be alleviated by increasing plant tolerance. As mentioned in a preceding section, the genetic variability in O3 susceptibility relates to activities in antioxidant systems, which could be a good target for engineering O3-tolerant varieties. It has been estimated that, by choosing least O3-sensitive varieties in wheat, soybean and maize, production of the crops could be improved by 12% on global aggregate (Avnery, Mauzerall, and Fiore Citation2013). They also acknowledged that O3 impacts could be further reduced by extensive screening and targeted breeding for higher tolerance in the crop species.
The O3 susceptibility can also be lowered by chemicals, of which ethylenediurea (EDU) has been studied most extensively with a wide range of Asian species and genotypes (Oksanen et al. Citation2013; Agathokleous et al. Citation2015; Singh et al. Citation2015; Jiang et al. Citation2018; Pandey et al. Citation2019; Gupta et al. Citation2020). Understanding mechanisms of the chemical protectants could facilitate development of commercial products for protection of agricultural crops from O3. The chemical protectants will also facilitate the varietal screening for higher tolerance to O3 under agronomically realistic environment (Jiang et al. Citation2018; Pandey et al. Citation2019; Gupta et al. Citation2020).
Integrating the approaches for mitigation of ozone impacts
The emission control must not be compromised because of the prospects for higher plant tolerance to O3, since lowering the O3 level is the only measure to protect vegetation in unmanaged ecosystems (). To this end, monitoring is the critical first step, and the recent development of the nation-wide network of about 1500 monitoring sites has enabled the identification of significant O3 risks to temperate forests in China (Li et al. Citation2018a). China’s monitoring network will play a critical role in evaluating the efficacy of its efforts for emission control (Zheng et al. Citation2018).
South Asia is one of the world’s hotspots of O3 pollution impacts (Mills et al. Citation2018b), but continued monitoring of O3 concentrations has been limited. A monitoring network equivalent to that in China throughout South Asia would convincingly demonstrate the risks of O3 pollution, which will motivate the efforts toward the region-wide emission control.
To better support the efforts for emission control, we need progress with improved models to assess O3 impact on vegetation (). The air pollution models will benefit from better developed monitoring networks and understandings of the contributions of vegetation to O3 pollution. The impact models based on improved understandings of the mechanisms of damages would also have to account for the interaction with other environmental changes ().
Recognizing the escalating impacts of surface O3 and great challenges of emission control in Asia, we need to pursue the measures for increasing plant tolerance to O3 . They are limited to vegetation in managed ecosystems (), which, however, does not downplay their importance. As of 2015, the fractional employment in agriculture is more than 40% in countries of South Asia and 20% in China (ILOSTAT: http://www.ilo.org/ilostat/faces/oracle/webcenter/portalapp/pagehierarchy/Page3.jspx?MBI_ID=33 (last update on 9 July 2018)), whereas the fraction is only about 2% in Northern and Western Europe or even less in North America. Negative effects of O3 pollution on agriculture will directly hit a majority of society members across Asia. In addition, South Asia is among the two regions in the world, along with Sub-Saharan Africa, where food shortage will persist in 2050 (Alexandratos and Bruinsma Citation2012).
The societal significance of agriculture in Asia shall justify the efforts for developing cost-effective protectants and tolerant varieties. The benefits of this approach could be large. A recent analysis of experimental results from around the world indicated that wheat yield is increased by about 10% by reducing O3 from the current ambient level (about 40 ppb day-time mean concentrations) to that of pre-industrial times (about 15 ppb) (Pleijel et al. Citation2018). Such a large reduction of O3 levels is beyond the feasibility of emission controls, but the genetic or chemical measures could take advantage of the yield increase with the higher O3 tolerance. Besides the scientific challenges, however, these efforts would face an additional challenge: they have to be adopted by the huge number of farmers (). Convincing farmers of O3 impacts is far from an easy task (Frei Citation2015), but co-benefits from water-saving and tolerance against other stressors shall facilitate the adoption by farmers. The co-benefits are substantial due to the co-occurrence of O3 impacts with those by other stressors estimated for South and East Asia (Mills et al. Citation2018b).
Evidently, scientific findings in the two approaches to reduction of O3 impacts must be directed to very different audiences: governmental agencies in charge of environmental protection on the one hand, and agronomic institutions from national to local scales on the other hand. The huge diversity of plant production systems in Asia presents intimidating challenges against those who take the latter approach. They can, however, work with scientists in other disciplines, e.g., plant pathology and stress physiology, working for plant protection. In plant breeding also, stress tolerance is among the critical target traits. The real challenge for the latter approach would therefore be to develop interdisciplinary collaborations of scientists toward the common goal: higher food security and better agricultural performances.
Conclusions
We argue that we need both mitigation of and adaptation to the O3 pollution in Asia. To mitigate emission of O3 precursors, we need to develop better monitoring networks and improve the models of O3 pollution and its impacts. The impact assessment shall benefit from the improved understanding of the plant responses to O3 impacts and how plants interact with other organisms, such as pests and symbionts. Better understandings of the plant responses shall also help the efforts to increase tolerance in plants against O3 as adaptation to its risen levels.
To attain the goal of reducing O3 pollution impacts on vegetation and maintain food security, the scientific advancements need to be transferred to institutional actions: improved emission control by regulatory authorities and adoption of measures for higher tolerance in plants by farmers. To these ends, there must be a community of scientists concerned about the O3 impacts in each country, where these scientists would play the key role in connecting the scientific advancements with the institutional actions on the international and interdisciplinary collaborations.
Author contributions
ZF and KK designed the structure. XYue, HSas, XL and ADM authored a first draft of Monitoring, modeling and assessment of ozone impacts in Asia; EO, AG, YJ, SKS and HSaj authored a first draft of Effects of ozone on plant metabolism; EP, XYuan, YH authored a first draft of Plant contribution to ozone pollution in Asia; EA, MW and KK authored a first draft of Toward mitigation of ozone impacts on plants and ecosystems in Asia. XYuan and KK authored a first draft of Table S1. ZF, EA and KK edited the first draft of the manuscript. All authors revised the manuscript for important intellectual content and approved the final version for submission.
Acknowledgments
This study has been funded by National Natural Science Foundation of China (No. 41771034, 42061160479, 31950410547, 4190738, and M-0105), Key Research Program of Frontier Sciences, CAS (QYZDB-SSW-DQC019), CNR-CAS bilateral agreement 2017-2019 (Ozone impacts on plant ecosystems in China and Italy), and Chinese Academy of Sciences President’s International Fellowship Initiative (PIFI) for Senior Scientists (2018VCA0026). AG and YJ were supported by the French National Research Agency through the Laboratory of Excellence ARBRE (ANR-12- LABXARBRE-01).
Disclosure statement
There are no competing interests.
Additional information
Funding
References
- Abu Elela, S. A., E. Agathokleous, and T. Koike. 2018. “Growth and Nutrition of Agelastica coerulea (Coleoptera: Chrysomelidae) Larvae Changed When Fed with Leaves Obtained from an O3-enriched Atmosphere.” Environmental Science and Pollution Research 25 (13): 13186–14. doi:https://doi.org/10.1007/s11356-018-1683-1.
- Agathokleous, E., V. Araminiene, R. G. Belz, V. Calatayud, A. De Marco, M. Domingos, Z. Feng, et al. 2019a. “A Quantitative Assessment of Hormetic Responses of Plants to Ozone.” Environmental Research 176: 108527. doi:https://doi.org/10.1016/j.envres.2019.108527.
- Agathokleous, E., R. G. Belz, V. Calatayud, A. De Marco, Y. Hoshika, M. Kitao, C. J. C. J. Saitanis, P. Sicard, E. Paoletti, and E. J. Calabrese. 2019b. “Predicting the Effect of Ozone on Vegetation via Linear Non-threshold (LNT), Threshold and Hormetic Dose-response Models.” Science of the- Total Environment 649: 61–74.
- Agathokleous, E., Z. Feng, E. Oksanen, P. Sicard, Q. Wang, C. J. Saitanis, V. Araminiene, et al. 2020. “Ozone Affects Plant, Insect, and Soil Microbial Communities: A Threat to Terrestrial Ecosystems and Biodiversity.” Science Advances 6 (33): eabc1176. doi:https://doi.org/10.1126/sciadv.abc1176.
- Agathokleous, E., M. Kitao, and Y. Kinose. 2018. “A Review Study on Ozone Phytotoxicity Metrics for Setting Critical Levels in Asia.” Asian Journal of Atmospheric Environment 12 (1): 1–16. doi:https://doi.org/10.5572/ajae.2018.12.1.001.
- Agathokleous, E., T. Koike, M. Watanabe, Y. Hoshika, and C. J. Saitanis. 2015. “Ethylene-di-urea (EDU), an Effective Phytoproctectant against O3 Deleterious Effects and a Valuable Research Tool.” Journal of Agricultural Meteorology 71 (3): 185–195. doi:https://doi.org/10.2480/agrmet.D-14-00017.
- Agathokleous, E., C. J. Saitanis, D. Stamatelopoulos, A.-C. Mouzaki-Paxinou, E. Paoletti, and W. J. Manning. 2016. “Olive Oil for Dressing Plant Leaves so as to Avoid O3 Injury.” Water, Air, and Soil Pollution 227 (8): 282. doi:https://doi.org/10.1007/s11270-016-2986-9.
- Agathokleous, E., T. Sakikawa, S. A. Abu Elela, T. Mochizuki, M. Nakamura, M. Watanabe, K. Kawamura, and T. Koike. 2017. “Ozone Alters the Feeding Behavior of the Leaf Beetle Agelastica coerulea (Coleoptera: Chrysomelidae) into Leaves of Japanese White Birch (Betula platyphylla Var. japonica).” Environmental Science and Pollution Research 24 (21): 17577–17583. doi:https://doi.org/10.1007/s11356-017-9369-7.
- Agathokleous, E., Y. WaiLi, G. Ntatsi, K. Konno, C. J. Saitanis, M. Kitao, and T. Koike. 2019c. “Effects of Ozone and Ammonium Sulfate on Cauliflower: Emphasis on the Interaction between Plants and Insect Herbivores.” Science of the Total Environment 659: 995–100. doi:https://doi.org/10.1016/j.scitotenv.2018.12.388.
- Alexandratos, N., and J. Bruinsma. 2012. “World Agriculture Towards” 2030/2050: The 2012 Revision. ESA working paper No. 12-03. Rome.
- Anav, A., A. De Marco, C. Proietti, A. Alessandri, A. Dell’Aquila, I. Cionni, P. Friedlingstein, et al. 2016. “Comparing Concentration-based (AOT40) and Stomatal Uptake (PODY) Metrics for Ozone Risk Assessment to European Forests.” Global Change Biology 22 (4): 1608–1627. doi:https://doi.org/10.1111/gcb.13138.
- Assareh, N., T. Prabamroong, K. Manomaiphiboon, P. Theramongkol, S. Leungsakul, N. Mitrjit, and J. Rachiwong. 2016. “Analysis of Observed Surface Ozone in the Dry Season over Eastern Thailand during 1997-2012.” Atmospheric Research 178-179: 17–30. doi:https://doi.org/10.1016/j.atmosres.2016.03.009.
- Assessment, M. E. 2003. Ecosystems and Human Well-Being: A Framework for Assessment. Washington DC: Island Press. 212. ISBN: 1-55963-403-0.
- Atkinson, R., and J. Arey. 2003. “Atmospheric Degradation of Volatile Organic Compounds.” Chemical Reviews 103 (12): 4605–4638. doi:https://doi.org/10.1021/cr0206420.
- Avnery, S., D. L. Mauzerall, and A. M. Fiore. 2013. “Increasing Global Agricultural Production by Reducing Ozone Damages via Methane Emission Controls and Ozone-resistant Cultivar Selection.” Global Change Biology 19 (4): 1285–1299. doi:https://doi.org/10.1111/gcb.12118.
- Bao, H., K. L. Shrestha, A. Kondo, A. Kaga, and Y. Inoue. 2010. “Modeling the Influence of Biogenic Volatile Organic Compound Emissions on Ozone Concentration during Summer Season in the Kinki Region of Japan.” Atmospheric Environment 44 (3): 421–431. doi:https://doi.org/10.1016/j.atmosenv.2009.10.021.
- Blande, J. 2021. “Effects of Air Pollution on Plant–insect Interactions Mediated by Olfactory and Visual Cues.” Current Opinion in Environmental Science & Health 19: 100228. doi:https://doi.org/10.1016/j.coesh.2020.100228.
- Brauer, M., G. Freedman, J. Frostad, A. Van Donkelaar, R. V. Martin, F. Dentener, R. Van Dingenen, et al. 2016. “Ambient Air Pollution Exposure Estimation for the Global Burden of Diseases 2013.” Environmental Science & Technology 50 (1): 79–88. doi:https://doi.org/10.1021/acs.est.5b03709.
- Broberg, M. C., Z. Feng, Y. Xin, and H. Pleijel. 2015. “Ozone Effects on Wheat Grain Quality- A Summary.” Environmental Pollution 197: 203–213. doi:https://doi.org/10.1016/j.envpol.2014.12.009.
- Bussotti, F. 2008. “Functional Leaf Traits, Plant Communities and Acclimation Processes in Relation to Oxidative Stress in Trees: A Critical Overview.” Global Change Biology 14 (11): 2727–2739. doi:https://doi.org/10.1111/j.1365-2486.2008.01677.x.
- Carrijo, D. R., M. E. Lundy, and B. A. Linquist. 2017. “Rice Yields and Water Use under Alternate Wetting and Drying Irrigation: A Meta-analysis.” Field Crops Research 203: 173–180. doi:https://doi.org/10.1016/j.fcr.2016.12.002.
- Dai, L., K. Kobayashi, I. Nouchi, Y. Masutomi, and Z. Feng. 2020. “Quantifying Determinants Of Ozone Detoxification By Apoplastic Ascorbate In Peach (Prunus persica) Leaves Using a Model Of Ozone Transport and Reaction.” Global Change Biology 26 (5): 3147–3162. doi:https://doi.org/10.1111/gcb.15049.
- De Marco, A., A. Anav, P. Sicard, Z. Feng, and E. Paoletti. 2020. “High Spatial Resolution Ozone Risk-assessment for Asian Forests.” Environmental Research Letters 15 (10): 104095. doi:https://doi.org/10.1088/1748-9326/abb501.
- Duan, C., S. Zuo, Z. Wu, Y. Qiu, J. Wang, Y. Lei, H. Liao, and Y. Ren. 2020. “A Review of Research Hotspots and Trends in Biogenic Volatile Organic Compounds (Bvocs) Emissions Combining Bibliometrics with Evolution Tree Methods.” Environmental Research Letters 16 (1): 013003. doi:https://doi.org/10.1088/1748-9326/abcee9.
- Emberson, L. D., P. Büker, M. R. Ashmore, G. Mills, L. S. Jackson, M. Agrawal, M. D. Atikuzzaman, et al. 2009. “A Comparison of North American and Asian Exposure–response Data for Ozone Effects on Crop Yields.” Atmospheric Environment 43 (12): 1945–1953. doi:https://doi.org/10.1016/j.atmosenv.2009.01.005.
- Emberson, L. D., H. Pleijel, E. A. Ainsworth, M. Van Den Berg, W. Ren, S. Osborne, G. Mills, et al. 2018. “Ozone Effects on Crops and Consideration in Crop Models.” European Journal of Agronomy 100: 19–34. doi:https://doi.org/10.1016/j.eja.2018.06.002.
- Feng, Z., P. Büker, H. Pleijel, L. Emberson, P. E. Karlsson, and J. Uddling. 2018a. “A Unifying Explanation for Variation in Ozone Sensitivity among Woody Plants.” Global Change Biology 24 (1): 78–84. doi:https://doi.org/10.1111/gcb.13824.
- Feng, Z., A. De Marco, A. Anav, M. Gualtieri, P. Sicard, H. Tian, F. Fornasier, F. Tao, A. Guo, and E. Paoletti. 2019a. “Economic Losses Due to Ozone Impacts on Human Health, Forest Productivity and Crop Yield across China.” Environment International 131: 104966. doi:https://doi.org/10.1016/j.envint.2019.104966.
- Feng, Z., L. Jiang, V. Calatayud, L. Dai, and E. Paoletti. 2018b. “Intraspecific Variation in Sensitivity of Winter Wheat (Triticum aestivum L.) To Ambient Ozone in Northern China as Assessed by Ethylenediurea (EDU).” Environmental Science and Pollution Research 25 (29): 29208–29218. doi:https://doi.org/10.1007/s11356-018-2782-8.
- Feng, Z., J. Pang, I. Nouchi, K. Kobayashi, T. Yamakawa, and J. Zhu. 2010. “Apoplastic Ascorbate Contributes to the Differential Ozone Sensitivity in Two Varieties of Winter Wheat under Fully Open-air Field Conditions.” Environmental Pollution 158 (12): 3539–3545. doi:https://doi.org/10.1016/j.envpol.2010.08.019.
- Feng, Z., H. Tang, and K. Kobayashi. 2017. “Effects of Ozone on Crops in China. Pages 175–194.” In Air Pollution Impacts on Plants in East Asia, edited by T. Izuta, 322. Tokyo: Springer Japan.
- Feng, Z., H. Tang, J. Uddling, H. H. Pleijel, K. Kobayashi, J. Zhu, H. Oue, and W. Guo. 2012. “A Stomatal Ozone Flux-response Relationship to Assess Ozone-induced Yield Loss of Winter Wheat in Subtropical China.” Environmental Pollution 164: 16–23. doi:https://doi.org/10.1016/j.envpol.2012.01.014.
- Feng, Z., J. Uddling, H. Tang, J. Zhu, and K. Kobayashi. 2018c. “Comparison of Crop Yield Sensitivity to Ozone between Open-top Chamber and Free-air Experiments.” Global Change Biology 24 (6): 2231–2238. doi:https://doi.org/10.1111/gcb.14077.
- Feng, Z., X. Yuan, S. Fares, F. Loreto, P. Li, Y. Hoshika, and E. Paoletti. 2019b. “Isoprene Is More Affected by Climate Drivers than Monoterpenes: A Meta‐analytic Review on Plant Isoprenoid Emissions.” Plant, Cell & Environment 42 (6): 1939–1949. doi:https://doi.org/10.1111/pce.13535.
- Frei, M. 2015. “Breeding of Ozone Resistant Rice: Relevance, Approaches and Challenges.” Environmental Pollution 197: 144–155. doi:https://doi.org/10.1016/j.envpol.2014.12.011.
- Fu, T. M., D. J. Jacob, P. I. Palmer, K. Chance, Y. X. Wang, B. Barletta, D. R. Blake, J. C. Stanton, and M. J. Pilling. 2007. “Space-based Formaldehyde Measurements as Constraints on Volatile Organic Compound Emissions in East and South Asia and Implications for Ozone.” Journal of Geophysical Research Atmospheres 112 (D6): D6. doi:https://doi.org/10.1029/2006JD007853.
- Ghude, S. D., C. Jena, D. M. Chate, G. Beig, G. G. Pfister, R. Kumar, and V. Ramanathan. 2014. “Reductions in India’s Crop Yield Due to Ozone.” Geophysical Research Letters 41 (15): 5685–5691. doi:https://doi.org/10.1002/2014GL060930.
- Gilliland, N. J., A. H. Chappelka, R. B. Muntifering, F. L. Booker, and S. S. Ditchkoff. 2012. “Digestive Utilization of Ozone-exposed Forage by Rabbits (Oryctolagus cuniculus).” Environmental Pollution 163: 281–286. doi:https://doi.org/10.1016/j.envpol.2012.01.003.
- Gupta, S. K., M. Sharma, B. Majumder, V. K. Maurya, F. Deeba, J.-L. Zhang, and V. Pandey. 2020. “Effects of Ethylenediurea (EDU) on Regulatory Proteins in Two Maize (Zea mays L.) Varieties under High Tropospheric Ozone Phytotoxicity.” Plant Physiology and Biochemistry 154: 675–688. doi:https://doi.org/10.1016/j.plaphy.2020.05.037.
- Han, Z., H. Ueda, and K. Matsuda. 2005. “Model Study of the Impact of Biogenic Emission on Regional Ozone and the Effectiveness of Emission Reduction Scenarios over Eastern China.” Tellus B: Chemical and Physical Meteorology 57 (1): 12–27. doi:https://doi.org/10.3402/tellusb.v57i1.16775.
- Heil, M. 2014. “Herbivore‐induced Plant Volatiles: Targets, Perception and Unanswered Questions.” New Phytologist 204 (2): 297–306. doi:https://doi.org/10.1111/nph.12977.
- Hoesly, R. M., S. J. Smith, L. Feng, Z. Klimont, G. Janssens-Maenhout, T. Pitkanen, J. J. Seibert, et al. 2018. “Historical (1750–2014) Anthropogenic Emissions of Reactive Gases and Aerosols from the Community Emissions Data System (CEDS).” Geoscientific Model Development 11 (1): 369–408. doi:https://doi.org/10.5194/gmd-11-369-2018.
- Izuta, T. 2017. “Air Pollution Impacts on Plants in East Asia.“ edited by T. Izuta, Page, 322. Tokyo: Springer Japan.
- Jiang, L., Z. Feng, L. Dai, B. Shang, and E. Paoletti. 2018. “Large Variability in Ambient Ozone Sensitivity across 19 Ethylenediurea-treated Chinese Cultivars of Soybean Is Driven by Total Ascorbate.” Journal of Environmental Sciences 64: 10–22. doi:https://doi.org/10.1016/j.jes.2017.07.002.
- Jing, X., X. Lun, C. Fan, and W. Ma. 2020. “Emission Patterns of Biogenic Volatile Organic Compounds from Dominant Forest Species in Beijing, China.” Journal of Environmental Sciences (China) 95: 73–81. doi:https://doi.org/10.1016/j.jes.2020.03.049.
- Karabourniotis, G., G. Liakopoulos, D. Nikolopoulos, and P. Bresta. 2019. “Protective and Defensive Roles of Non-glandular Trichomes against Multiple Stresses: Structure–function Coordination.” Journal of Forestry Research 31 (1): 1–12. doi:https://doi.org/10.1007/s11676-019-01034-4.
- Kitao, M., M. Komatsu, K. Yazaki, S. Kitaoka, and H. Tobita. 2015. “Growth Overcompensation against O3 Exposure in Two Japanese Oak Species, Quercus mongolica var. crispula and Quercus serrata, Grown under Elevated CO2.” Environmental Pollution 206: 133–141. doi:https://doi.org/10.1016/j.envpol.2015.06.034.
- Koike, T., M. Watanabe, Y. Hoshika, M. Kitao, H. Matsumura, R. Funada, and T. Izuta. 2013. “Effects of Ozone on Forest Ecosystems in East and Southeast Asia.”, 371–390. In Climate Change, Air Pollution and Global Challenges: Understanding and Perspectives from Forest Research, edited by R. Matyssek, N. Clarke, P. Cudlin, T.N. Mikkelsen, J.-P. Tuovinen, G. Wieser, and E. Paoletti, Vol. 13, 648. Book Series: Developments in Environmental Science, Elsevier.
- Lal, D. M. M., S. D. Ghude, S. D. D. Patil, S. H. Kulkarni, C. Jena, S. Tiwari, and M. K. Srivastava. 2012. “Tropospheric Ozone and Aerosol Long-term Trends over the Indo-Gangetic Plain (IGP), India.” Atmospheric Research 116: 82–92. doi:https://doi.org/10.1016/j.atmosres.2012.02.014.
- Lal, S., S. Venkataramani, M. Naja, J. C. Kuniyal, T. K. Mandal, P. K. Bhuyan, K. M. Kumari, et al. 2017. “Loss of Crop Yields in India Due to Surface Ozone: An Estimation Based on a Network of Observations.” Environmental Science and Pollution Research 24 (26): 20972–20981. doi:https://doi.org/10.1007/s11356-017-9729-3.
- Lefohn, A. S., C. S. Malley, L. Smith, B. Wells, M. Hazucha, H. Simon, V. Naik, et al. 2018. “Tropospheric Ozone Assessment Report: Global Ozone Metrics for Climate Change, Human Health, and Crop/ecosystem Research.” Elem Sci Anth 6: 28. doi:https://doi.org/10.1525/elementa.279.
- Li, D., Y. Chen, Y. Shi, X. He, and X. Chen. 2009. “Impact of Elevated CO2 and O3 Concentrations on Biogenic Volatile Organic Compounds Emissions from Ginkgo biloba.” Bulletin of Environmental Contamination and Toxicology 82 (4): 473–477. doi:https://doi.org/10.1007/s00128-008-9590-7.
- Li, P., V. Calatayud, F. Gao, J. Uddling, and Z. Feng. 2016. “Differences in Ozone Sensitivity among Woody Species are Related to Leaf Morphology and Antioxidant Levels.” Tree Physiology 36 (9): 1105–1116. doi:https://doi.org/10.1093/treephys/tpw042.
- Li, P., A. De Marco, Z. Feng, A. Anav, D. Zhou, and E. Paoletti. 2018a. “Nationwide Ground-level Ozone Measurements in China Suggest Serious Risks to Forests.” Environmental Pollution 237: 803–813. doi:https://doi.org/10.1016/j.envpol.2017.11.002.
- Li, P., Z. Feng, V. Catalayud, X. Yuan, Y. Xu, and E. Paoletti. 2017. “A Meta-analysis on Growth, Physiological, and Biochemical Responses of Woody Species to Ground-level Ozone Highlights the Role of Plant Functional Types.” Plant, Cell & Environment 40 (10): 2369–2380. doi:https://doi.org/10.1111/pce.13043.
- Li, S., T. Tosens, P. C. Harley, Y. Jiang, A. Kanagendran, M. Grosberg, K. Jaamets, and Ü. Niinemets. 2018b. “Glandular Trichomes as a Barrier against Atmospheric Oxidative Stress: Relationships with Ozone Uptake, Leaf Damage, and Emission of LOX Products across a Diverse Set of Species.” Plant, Cell & Environment 41 (6): 1263–1277. doi:https://doi.org/10.1111/pce.13128.
- Li, Z., J. Yang, B. Shang, E. Agathokleous, K. Rubert-Nason, Y. Xu, and Z. Feng. 2021. “Nonlinear Responses of Foliar Phenylpropanoids to Increasing O3 Exposure: Ecological Implications in a Populus Model System.” Science of the Total Environment 767: 144358. doi:https://doi.org/10.1016/j.scitotenv.2020.144358.
- Lin, Y., F. Jiang, J. Zhao, G. Zhu, X. He, X. Ma, S. Li, C. E. Sabel, and H. Wang. 2018. “Impacts of O3 on Premature Mortality and Crop Yield Loss across China.” Atmospheric Environment 194: 41–47. doi:https://doi.org/10.1016/j.atmosenv.2018.09.024.
- Liu, X., Y. Zhang, W. Han, A. Tang, J. Shen, Z. Cui, P. Vitousek, et al. 2013. “Enhanced Nitrogen Deposition over China.” Nature 494 (7438): 459–462. doi:https://doi.org/10.1038/nature11917.
- Liu, Y., L. Li, J. An, L. Huang, R. Yan, C. Huang, H. Wang, Q. Wang, M. Wang, and W. Zhang. 2018. “Estimation of Biogenic VOC Emissions and Its Impact on Ozone Formation over the Yangtze River Delta Region, China.” Atmospheric Environment 186: 113–128. doi:https://doi.org/10.1016/j.atmosenv.2018.05.027.
- Loladze, I. 2002. “Rising Atmospheric CO2 and Human Nutrition: Toward Globally Imbalanced Plant Stoichiometry?” Trends in Ecology & Evolution 17 (10): 457–461. doi:https://doi.org/10.1016/S0169-5347(02)02587-9.
- Loladze, I. 2014. “Hidden Shift of the Ionome of Plants Exposed to Elevated CO2 Depletes Minerals at the Base of Human Nutrition.” eLife 3: e02245. doi:https://doi.org/10.7554/eLife.02245.
- Ma, Z., J. Xu, W. Quan, Z. Zhang, W. Lin, and X. Xu. 2016. “Significant Increase of Surface Ozone at a Rural Site, North of Eastern China.” Atmospheric Chemistry and Physics 16 (6): 3969–3977. doi:https://doi.org/10.5194/acp-16-3969-2016.
- Maas, R., and P. Grennfelt. 2016. “Towards Cleaner Air. Scientific Assessment Report”. 2016. Page R. Maas and P. Grennfelt, edited by. EMEP Steering Body and Working Group on Effects of the Convention on Long-Range Transboundary Air Pollution, xx+50pp. Oslo: United Nations Economic Commission.
- Masui, N., E. Agathokleous, T. Mochizuki, A. Tani, H. Matsuura, and T. Koike. 2021. “Ozone Disrupts the Communication between Plants and Insects in Urban and Suburban Areas: An Updated Insight Focusing on Plant Volatiles.” Journal of Forestry Research. In Press. doi:https://doi.org/10.1007/s11676-020-01287-4.
- Messina, C. D., T. R. Sinclair, G. L. Hammer, D. Curan, J. Thompson, Z. Oler, C. Gho, and M. Cooper. 2015. “Limited-transpiration Trait May Increase Maize Drought Tolerance in the US Corn Belt.” Agronomy Journal 107 (6): 1978–1986. doi:https://doi.org/10.2134/agronj15.0016.
- Mills, G., H. Pleijel, C. S. Malley, B. Sinha, O. R. Cooper, M. G. Schultz, H. S. Neufeld, et al. 2018a. “Tropospheric Ozone Assessment Report: Present-day Tropospheric Ozone Distribution and Trends Relevant to Vegetation.” Elementa 6: 47.
- Mills, G., K. Sharps, D. Simpson, H. Pleijel, M. Frei, K. Burkey, L. Emberson, et al. 2018b. “Closing the Global Ozone Yield Gap: Quantification and Cobenefits for Multistress Tolerance.” Global Change Biology 24 (10): 4869–4893. doi:https://doi.org/10.1111/gcb.14381.
- Mochizuki, T., M. Watanabe, T. Koike, and A. Tani. 2017. “Monoterpene Emissions from Needles of Hybrid Larch F1 (Larix gmelinii var. japonica × Larix kaempferi) Grown under Elevated Carbon Dioxide and Ozone.” Atmospheric Environment 148: 197–202. doi:https://doi.org/10.1016/j.atmosenv.2016.10.041.
- Mukherjee, A., D. S. Yadav, S. B. Agrawal, and M. Agrawal. 2021. “Ozone a Persistent Challenge to Food Security in India: Current Status and Policy Implications.” Current Opinion in Environmental Science & Health 19: 100220. doi:https://doi.org/10.1016/j.coesh.2020.10.008.
- Novriyanti, E., Q. Mao, E. Agathokleous, M. Watanabe, Y. Hashidoko, and T. Koike. 2021. “Elevated CO2 Offsets the Alteration of Foliar Chemicals (n-icosane, Geranyl Acetate, and Elixene) Induced by Elevated O3 in Three Taxa of O3-tolerant Eucalypts.” Journal of Forestry Research 32 (2): 789–803. doi:https://doi.org/10.1007/s11676-020-01133-7.
- Oksanen, E. 2018. “Trichomes Form an Important First Line of Defence against Adverse environment-New Evidence for Ozone Stress Mitigation.” Plant, Cell & Environment 41 (7): 1497–1499. doi:https://doi.org/10.1111/pce.13187.
- Oksanen, E., V. Pandey, A. K. Pandey, S. Keski-Saari, S. Kontunen-Soppela, and C. Sharma. 2013. “Impacts of Increasing Ozone on Indian Plants.” Environmental Pollution 177: 189–200. doi:https://doi.org/10.1016/j.envpol.2013.02.010.
- Orru, H., C. Åström, C. Andersson, T. Tamm, K. L. Ebi, and B. Forsberg. 2019. “Ozone and Heat-related Mortality in Europe in 2050 Significantly Affected by Changes in Climate, Population and Greenhouse Gas Emission.” Environmental Research Letters 14 (7): 074013. doi:https://doi.org/10.1088/1748-9326/ab1cd9.
- Osborne, S. A., G. Mills, F. Hayes, E. A. Ainsworth, P. Büker, and L. Emberson. 2016. “Has the Sensitivity of Soybean Cultivars to Ozone Pollution Increased with Time? an Analysis of Published Dose-response Data”. Global Change Biology 22:3097–3111.
- Oue, H., K. Kobayashi, J. Zhu, W. Guo, and X. Zhu. 2011. “Improvements of the Ozone Dose Response Functions for Predicting the Yield Loss of Wheat Due to Elevated Ozone.” Journal of Agricultural Meteorology 67 (1): 21–32. doi:https://doi.org/10.2480/agrmet.67.1.2.
- Pandey, A. K., B. Majumder, S. Keski-Saari, S. Kontunen-Soppela, A. Mishra, N. Sahu, V. Pandey, and E. Oksanen. 2015. “Searching for Common Responsive Parameters for Ozone Tolerance in 18 Rice Cultivars in India: Results from Ethylenediurea Studies.” Science of the Total Environment 532: 230–238. doi:https://doi.org/10.1016/j.scitotenv.2015.05.040.
- Pandey, A. K. A. K., B. Majumder, S. Keski-Saari, S. Kontunen-Soppela, V. Pandey, and E. Oksanen. 2019. “High Variation in Resource Allocation Strategies among 11 Indian Wheat (Triticum aestivum) Cultivars Growing in High Ozone Environment.” Climate 7 (2): 23. doi:https://doi.org/10.3390/cli7020023.
- Peñuelas, J., and M. Staudt. 2010. “BVOCs and Global Change.” Trends in Plant Science 15 (3): 133–144. doi:https://doi.org/10.1016/j.tplants.2009.12.005.
- Pleijel, H., M. C. Broberg, J. Uddling, and G. Mills. 2018. “Current Surface Ozone Concentrations Significantly Decrease Wheat Growth, Yield and Quality.” Science of the Total Environment 613-614: 687–692. doi:https://doi.org/10.1016/j.scitotenv.2017.09.111.
- Rahantaniaina, M. S., S. Li, G. Chatel-Innocenti, A. Tuzet, E. Issakidis-Bourguet, A. Mhamdi, and G. Noctor. 2017. “Cytosolic and Chloroplastic DHARs Cooperate in Oxidative Stress-driven Activation of the Salicylic Acid Pathway.” Plant Physiology 174 (2): 956–971. doi:https://doi.org/10.1104/pp.17.00317.
- Sase, H. 2017. “Acid Deposition.” In Air Pollution Impacts on Plants in East Asia, edited by T. Izuta. Pages, 43–53. Tokyo: Springer Japan.
- Schultz, M. G., S. Schröder, O. Lyapina, O. Cooper, I. Galbally, I. Petropavlovskikh, E. Von Schneidemesser, et al. 2017. “Tropospheric Ozone Assessment Report: Database and Metrics Data of Global Surface Ozone Observations.” Elementa 5: 58.
- Sicard, P., A. De Marco, E. Carrari, L. Dalstein-Richier, Y. Hoshika, O. Badea, D. Pitar, et al. 2020. “Epidemiological Derivation of Flux-based Critical Levels for Visible Ozone Injury in European Forests.” Journal of Forestry Research 31 (5): 509–1519. doi:https://doi.org/10.1007/s11676-020-01191-x.
- Sindelarova, K., C. Granier, I. Bouarar, A. Guenther, S. Tilmes, T. Stavrakou, J.-F. Müller, U. Kuhn, P. Stefani, and W. Knorr. 2014. “Global Data Set of Biogenic VOC Emissions Calculated by the MEGAN Model over the Last 30 Years.” Atmospheric Chemistry and Physics 14 (17): 9317–9341. doi:https://doi.org/10.5194/acp-14-9317-2014.
- Singh, A. A., S. Singh, M. Agrawal, and S. B. Agrawal. 2015. “Assessment of Ethylene Diurea-induced Protection in Plants against Ozone Phytotoxicity.” Reviews of Environmental Contamination and Toxicology 233: 129–184. doi:https://doi.org/10.1007/978-3-319-10479-9_4.
- Society, T. R. 2008. “Ground-level Ozone in the 21st Century: Future Trends, Impacts and Policy Implications”. Page Science Policy Report 15/08. Royal Society, London.
- Tang, H., J. Pang, G. Zhang, M. Takigawa, G. Liu, J. Zhu, and K. Kobayashi. 2014. “Mapping Ozone Risks for Rice in China for Years 2000 and 2020 with Flux-based and Exposure-based Doses.” Atmospheric Environment 86: 74–83. doi:https://doi.org/10.1016/j.atmosenv.2013.11.078.
- Tang, H., M. Takigawa, G. Liu, J. Zhu, and K. Kobayashi. 2013. “A Projection of Ozone-induced Wheat Production Loss in China and India for the Years 2000 and 2020 with Exposure-based and Flux-based Approaches.” Global Change Biology 19 (9): 2739–2752. doi:https://doi.org/10.1111/gcb.12252.
- Tani, A., T. Ohno, T. Saito, S. Ito, T. Yonekura, and M. Miwa. 2017. “Effects of Ozone on Isoprene Emission from Two Major Quercus Species Native to East Asia.” Journal of Agricultural Meteorology 73 (4): 195–202. doi:https://doi.org/10.2480/agrmet.D-17-00022.
- Tao, F., Z. Feng, H. Tang, Y. Chen, and K. Kobayashi. 2017. “Effects of Climate Change, CO2 and O3 on Wheat Productivity in Eastern China, Singly and in Combination.” Atmospheric Environment 153: 182–193. doi:https://doi.org/10.1016/j.atmosenv.2017.01.032.
- Teixeira, E., G. Fischer, H. Van Velthuizen, R. Van Dingenen, F. Dentener, G. Mills, C. Walter, and F. Ewert. 2011. “Limited Potential of Crop Management for Mitigating Surface Ozone Impacts on Global Food Supply.” Atmospheric Environment 45 (15): 2569–2576. doi:https://doi.org/10.1016/j.atmosenv.2011.02.002.
- Tian, H., W. Ren, B. Tao, G. Sun, A. Chappelka, X. Wang, S. Pan, et al. 2016. “Climate Extremes and Ozone Pollution: A Growing Threat to China’s Food Security.” Ecosystem Health and Sustainability 2 (1): e01203. doi:https://doi.org/10.1002/ehs2.1203.
- Tsukahara, K., H. Sawada, Y. Kohno, T. Matsuura, I. C. Mori, T. Terao, M. Ioki, and M. Tamaoki. 2015. “Ozone-induced Rice Grain Yield Loss Is Triggered via a Change in PANICLE Morphology that Is Controlled by ABERRANT PANICLE ORGNIZATION 1 Gene.” Plos One 10 (4): e0123308. doi:https://doi.org/10.1371/journal.pone.0123308.
- Wang, L., J. Pang, Z. Feng, J. Zhu, and K. Kobayashi. 2015. “Diurnal Variation of Apoplastic Ascorbate in Winter Wheat Leaves in Relation to Ozone Detoxification.” Environmental Pollution 207: 413–419. doi:https://doi.org/10.1016/j.envpol.2015.09.040.
- Wang, P. 2021. “China’s Air Pollution Policies: Progress and Challenges.” Current Opinion in Environmental Science & Health 19: 100227. doi:https://doi.org/10.1016/j.coesh.2020.100227.
- Wang, Q., Z. Han, T. Wang, and R. Zhang. 2008. “Impacts of Biogenic Emissions of VOC and NOx on Tropospheric Ozone during Summertime in Eastern China.” The Science of the Total Environment 395 (1): 41–49. doi:https://doi.org/10.1016/j.scitotenv.2008.01.059.
- Wang, Y., and M. Frei. 2011. “Stressed Food - the Impact of Abiotic Environmental Stresses on Crop Quality.” Agriculture, Ecosystems & Environment 141: 271–286.
- Wei, X. L., Y. S. Li, K. S. Lam, A. Y. Wang, and T. J. Wang. 2007. “Impact of Biogenic VOC Emissions on a Tropical Cyclone-related Ozone Episode in the Pearl River Delta Region, China.” Atmospheric Environment 41 (36): 7851–7864. doi:https://doi.org/10.1016/j.atmosenv.2007.06.012.
- Wu, K., X. Yang, D. Chen, S. Gu, Y. Lu, Q. Jiang, K. Wang, et al. 2020. “Estimation of Biogenic VOC Emissions and Their Corresponding Impact on Ozone and Secondary Organic Aerosol Formation in China.” Atmospheric Research 231: 104656. doi:https://doi.org/10.1016/j.atmosres.2019.104656.
- Xu, S., W. Chen, Y. Huang, and X. He. 2012. “Responses of Growth, Photosynthesis and VOC Emissions of Pinus tabulaeformis Carr. Exposure to Elevated CO2 And/or Elevated O3 in an Urban Area.” Bulletin of Environmental Contamination and Toxicology 88 (3): 443–448. doi:https://doi.org/10.1007/s00128-011-0462-1.
- Xu, X. 2021. “Recent Advances in Studies of Ozone Pollution and Impacts in China: A Short Review.” Current Opinion in Environmental Science & Health 19: 100225. doi:https://doi.org/10.1016/j.coesh.2020.100225.
- Xu, Y., J. S. Li., X. Y. Yuan, and Z. Z. Feng. 2020. “Emission Characteristics of Biogenic Volatile Compounds (Bvocs) from Common Greening Tree Species in Northern China and Their Correlations with Photosynthetic Parameters.” Environmental Science 41: 70–78. in Chinese.
- Yadav, P., U. Mina, and A. Bhatia. 2020. “Screening of Forty Indian Amaranthus hypochondriacus Cultivars for Tolerance and Susceptibility to Tropospheric Ozone Stress.” Nucleus 11 (1): 1–11. doi:https://doi.org/10.1080/19491034.2019.1710321.
- Yamaguchi, M., M. Watanabe, H. Matsumura, Y. Kohno, and T. Izuta. 2011. “Experimental Studies on the Effects of Ozone on Growth and Photosynthetic Activity of Japanese Forest Tree Species.” Asian Journal of Atmospheric Environment 5 (2): 65–78. doi:https://doi.org/10.5572/ajae.2011.5.2.065.
- Yi, F., B. A. McCarl, X. Zhou, and F. Jiang. 2018. “Damages of Surface Ozone: Evidence from Agricultural Sector in China.” Environmental Research Letters 13 (3): 034019. doi:https://doi.org/10.1088/1748-9326/aaa6d9.
- Yuan, X., B. Shang, Y. Xu, Y. Xin, Y. Tian, Z. Feng, and E. Paoletti. 2017. “No Significant Interactions between Nitrogen Stimulation and Ozone Inhibition of Isoprene Emission in Cathay Poplar.” Science of the Total Environment 601-602: 222–229. doi:https://doi.org/10.1016/j.scitotenv.2017.05.138.
- Yue, X., N. Unger, K. Harper, X. Xia, H. Liao, T. Zhu, J. Xiao, Z. Feng, and J. Li. 2017. “Ozone and Haze Pollution Weakens Net Primary Productivity in China.” Atmospheric Chemistry and Physics 17 (9): 6073–6089. doi:https://doi.org/10.5194/acp-17-6073-2017.
- Zhang, J. J., Y. Wei, and Z. Fang. 2019. “Ozone Pollution: A Major Health Hazard Worldwide.” Frontiers in Immunology 10: 2518. doi:https://doi.org/10.3389/fimmu.2019.02518.
- Zhang, L., B. Xu, T. Wu, M. X. Wen, L. X. Fan, Z. Z. Feng, and E. Paoletti. 2017. “Transcriptomic Analysis of Pak Choi under Acute Ozone Exposure Revealed Regulatory Mechanism against Ozone Stress.” BMC Plant Biology 17 (1): 236. doi:https://doi.org/10.1186/s12870-017-1202-4.
- Zhang, Y., O. R. Cooper, A. Gaudel, A. M. Thompson, P. Nédélec, S. Y. Ogino, and J. J. West. 2016. “Tropospheric Ozone Change from 1980 to 2010 Dominated by Equatorward Redistribution of Emissions.” Nature Geoscience 9 (12): 875–879. doi:https://doi.org/10.1038/ngeo2827.
- Zheng, B., D. Tong, M. Li, F. Liu, C. Hong, G. Geng, H. Li, et al. 2018. “Trends in China’s Anthropogenic Emissions since 2010 as the Consequence of Clean Air Actions.” Atmospheric Chemistry and Physics 18 (19): 14095–14111. doi:https://doi.org/10.5194/acp-18-14095-2018.