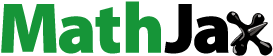
ABSTRACT
Potted rice seedlings independently treated with N, P, and NP were continuously13CO2 labeled to investigated the influence of N and P application on the contribution of photosynthesized C to the rhizosphere versus bulk soil and particulate organic matter (POM) versus mineral fraction (MIN). N and NP enhanced net assimilated 13C on day 14 (D14), with maximum C assimilation occurring on day 22 (D22) under NP. Aboveground biomass retained more 13C than belowground biomass for all treatments. 13C incorporation into the rhizosphere exceeded that in bulk soil, with the maximum (6–10%) found under N addition. Newly assimilated 13C incorporated into POM increased in the rhizosphere under N and NP conditions, whereas MIN remained largely unaffected. 13C-MBC proportion in the total microbial biomass C (MBC) pool revealed that N and NP stimulated microbial activity to a greater degree than P. The main portion of 13C in the rhizosphere and bulk soil was found in POM on D14, which decreased over time due to microbial utilization. Contrastingly, root-derived 13C in the MIN remained unchanged between sampling days, which indicates that the stabilization of rhizodeposits in this fraction might be the potential mechanism underlying SOM sequestration in paddy soils.
Introduction
About 75% of the world’s rice is produced under paddy conditions (Maclean et al. Citation2013; Oo et al. Citation2018), and paddy soil covers a total area of about 161 million ha worldwide . Besides challenges in food supply (Peng, Tang, and Zou Citation2009a), paddy soils play a crucial role in climate change mitigation, because they can sequester more carbon (C) than upland soils (Tian et al. Citation2013; Creamer et al. Citation2016).
Plant rhizodeposits, such as root exudates, mucilage, secretions, sloughed-off root cells/tissues, and senescence-derived compounds (Jones, Nguyen, and Finlay Citation2009), are among the main pools that link plant derived-C with soil organic matter (SOM), and can contribute up to 11% (about 30% of the C allocated to roots) of the net fixed C in the soil (Jones, Nguyen, and Finlay Citation2009; Liu et al. Citation2019a). As rhizodeposits are a highly labile part of plant derived-C (especially exudates), most of them are directly mineralized, whereas only about 5% can accumulate in the SOM (Hütsch, Augustin, and Merbach Citation2002; Kogel-Knabner Citation2002). The main location of this accumulated C in upland soils is the pool of particulate organic matter (POM) (up to 54%) or the mineral fraction (up to 46%) (Bradford, Fierer, and Reynolds Citation2008). The incorporation of root-derived C into these pools in paddy soils can be even greater (Wei et al. Citation2021) than that in upland soils, because the processes involved in mineralization of organic matter are inhibited under conditions of limited oxygen availability (Devêvre and Horwáth Citation2000; Olk, Brunetti, and Senesi Citation2000; Xu et al. Citation2018), which promotes C sequestration (Mandal et al. Citation2008). It is also possible that stabilization of rhizodeposited-C occurs in rhizosphere-free soil due to the specific water regime used in paddy soils, which results in migration of C molecules within the soil.
POM is expected to have faster turnover times and a lower capacity for long-term C storage than the mineral-associated pool, which suggests that mineral-associated C is processed more than POM by microorganisms and predominantly contains microbially derived material as a part of its composition (Schlesinger and Lichter Citation2001; Grandy and Robertson Citation2007; von Lützow et al. Citation2007). Therefore, more C associated with the mineral fraction is important for long-term SOC sequestration. Atere et al. (Citation2018) indicated that newly assimilated C or plant residues pass through two stages; i) first, incorporation into macroaggregates and ii) later, redistribution into smaller aggregates belonging to silt- and clay-size classes. However, the possible percentage of root-derived C that can accumulate in POM and mineral fractions of the SOM in paddy soils has not yet been estimated.
Among the factors affecting the amount of rhizodeposits, atmospheric CO2 concentration (van Ginkel, Gorissen, and van Veen Citation1997), light and temperature (Hew, Krotkov, and Canvin Citation1969), fertilizer application (An et al. Citation2015; Ge et al. Citation2015; Xiao et al. Citation2019), soil texture and irrigation management (Atere et al. Citation2018), tillage (Mo et al. Citation2019), plant growth stage (Sun et al. Citation2018), plant species (Derrien, Marol, and Balesdent Citation2004; Mwafulirwa et al. Citation2016), photosynthetic capacity (Kuzyakov and Cheng Citation2001), and assimilated 13C allocated into plant shoots and roots directly drive rhizodeposition (Ge, Luo, and He Citation2019). Nitrogen (N) and phosphorus (P) are essential plant nutrients, that affect the quality (indirect effects through buildup of more recalcitrant and less biodegradable) and quantity of photosynthesized C at various growth stages by stimulating plant growth (Saggar, Hedley, and Mackay Citation1997), and they also alter the distribution and fates of photosynthesized C into the shoots, roots, and soil-system (Liu et al. Citation2019b). Nitrogen fertilizers can nearly double the biomass of rice (Chun et al. Citation2013) because of the increase in chlorophyll content and the activity of rubisco enzymes, which consequently increases CO2 assimilation (Xiao et al. Citation2019) and rhizodeposition by 2–4 times (Ge et al. Citation2015; Xiao et al. Citation2019). However, N fertilization can also stimulate mineralization of both rhizodeposits and native SOM, resulting in only 2–33% of the net assimilated C remaining in the SOM composition (Liu et al. Citation2018). Phosphorus also improves the growth of plant roots and increases N uptake (Bowatte et al. Citation2006), thereby increasing the proportion of root-derived C allocated to the POM fraction by 1–1.5 times during rice growth (Atere et al. Citation2018). However, what remains unclear is the effect of N and P, and their combined application on the proportion of rhizodeposit-derived C that can be stabilized and fixed in SOC fractions, and the degree to which rhizosphere-free soil may be affected. Thus, the aim of this study was to investigate the allocation and fixation of newly photosynthesized C in response to N and P fertilization in a rice paddy system using the 13CO2 continuous labeling approach. We hypothesized that i) N and P addition will stimulate the allocation and fixation of newly assimilated C into the soil during the early plant growth stages with N having a greater effect than P, as it promotes plant biomass and root exudate production, and ii) N and P will promote the allocation and fixation of newly assimilated C into both the POM and mineral fraction in the rhizosphere and bulk soil.
Materials and methods
Study site description and soil properties
Soil was collected from the plowing layer (0–20 cm) of soil from a subtropical paddy rice field located at Changsha Research Station, Hunan, China (113°19′E, 28°33′N, 80 m a.s.l). The mean annual temperature and precipitation of the area are 17.5°C and 1,300 mm, respectively. For physicochemical analyses, soil was sieved through a < 4 mm sieve to remove the coarse root residues, and then air-dried at 35 ± 1°C and ground with a mortar and pestle prior to analysis. The soil was classified as a typical Stagnic Anthrosol and had the following texture: sand 28%, silt 66%, clay 6%. The soil contained total organic C, total N, and total P concentrations of 14.26, 1.45, 0.75 g kg,−1 respectively, and 12.75 mg Olsen-P kg−1 with a pH of 5.43 and cation exchange capacity of 7.71 mmol kg1.
Experimental design
Air-dried prepared soil (1.26 kg/pot) was used to fill 24 pots with an inner diameter of 11 cm and height of 20 cm. A rhizosphere bag (rhizo-bag with 0.34 kg soil; mesh 30 μm; 3.5 × 15 cm), which limited the passage of the root but permitted the movement of nutrients and water, was placed inside each pot to divide the soil into the rhizosphere and non-rhizosphere sections inside and outside the bag, respectively. Three 25-day-old rice seedlings of Zhongzao 39 (Oryza sativa L.,) were transplanted into the rhizo-bag. Further, basal fertilizers were added to each pot (KCl (80 mg K kg−1 soil), and dicyandiamide (nitrification inhibitor: 26 mg N kg−1). Soils in all pots were regularly saturated with distilled water (with a 2–3 cm water layer above the soil surface throughout the rice-growing season)
The experimental design was a factorial design with four treatments and three replicates (Control with zero N and P, N with 250 mg N kg−1 added in the form of urea; P with 80 mg P kg−1 added in the form of NaH2PO4; and NP with 250 mg N kg−1 plus 80 mg P kg−1 added in the form of urea and NaH2PO4, respectively.). Thus, the fertilization experiment comprised 12 pots, used for 13C labeling, and an additional 12 pots without 13C labeling (controls). The unlabeled controls served as references for the determination of natural 13C abundance and calculation of 13C atom percent excess. Therefore, the unlabeled pots were placed outside 10 m away from the labeled treatments in the field and were given the same amount of water and fertilizers.
13CO2 continuous labeling
Immediately after transplanted, rice seedlings were continuously labeled with 13CO2 over 22 days in air-tight growth chamber systems (80 × 250 × 120 cm) as reported by Ge et al. (Citation2012, Citation2015), which were exposed to natural sunlight in the rice field. A beaker with 1 M NaH13CO3 (50 atoms % 13C) was kept inside the glass chamber to release 13CO2 upon the addition of H2SO4 (10 mL, 0.5 M). 13CO2 concentration was constantly maintained between 360 and 380 μL CO2 L−1 inside the chamber via further reactions or diversion of gas flow through CO2 traps (1 M NaOH) for absorbing excess CO2 higher than the desired concentration. The growth labeling chamber was fitted with two temperature/humidity sensors (SNT-96S; Qingdao, China), and two fans for continuous air circulation to keep the temperature inside within 1°C of the ambient temperature via an air-conditioning system.
Sampling and harvesting
Plants and soil were harvested on days 14 and 22 (D14 and D22) after 13C labeling began. Shoots were cut off at the stem base, and the rhizosphere bags were collected from the pots. Roots were separated carefully from rhizosphere bags, then washed for a minute in 0.01 M CaCl2 (pH 6.2), and under fresh water to remove adhering soil. Samples were dried at 60°C for 48 h and then the shoots, roots, and soil sub-samples were ball milled and used to estimate the total C (TC), stable C isotope ratio (δ13C), total N (TN), and Olsen-P (OP). Meanwhile, fresh subsamples soil were used to measure both types of mineral N (NH4+ and NO3−), microbial biomass C (MBC), and dissolved organic C (DOC) content (Tian et al. Citation2013). MBC was determined using the chloroform fumigation-extraction method (Wu et al. Citation1990). The rhizosphere and bulk soils were used for aggregate fractionation and measurement of TC, δ13C, TN, mineral N, and OP. and TC of soil (aggregate) samples, which were analyzed using an isotope ratio mass spectrometer (IRMS, MAT253; Thermo-Fisher Scientific, Waltham, MA, USA), coupled with an elemental analyzer (Thermo Scientific FLASH 2000, USA; Thermo-Fisher Scientific, Waltham, MA, USA).
Soil physical fractionation
Soil organic C (SOC) was fractionated into POM (>53-µm) and MIN (<53-µm) using a method adapted from Cambardella and Elliott (Citation1993). Briefly, 10 g of subsample (of 2-mm sieved and air-dried soil) was shaken for 15 h with 0.5% sodium hexametaphosphate (1:3 ratio), and then, immediately after shaking, the dispersed soil suspension was passed through a 53-µm sieve. The dispersed particles retained on the sieve (>53-µm) were carefully collected and considered as POM fraction; the leftover suspension was centrifuged, and then, the settled soil particles were combined with the sediment and assigned to the MIN fraction. Separated POM and MIN fractions were oven-dried (60°C), homogenized, ground with a mortar and pestle, and then analyzed for TC and δ13C.
Calculations and statistical analysis
The amount of photosynthesized 13C accumulated in the plant organs (whole plant, roots, and shoots) and soil fractions (bulk, rhizosphere, POM, MIN, DOC, and MBC) (13Ca; mg 13C kg−1 or mg C m−2) were calculated using EquationEquation 1(1)
(1) :
where “l” and “nl” are labeled and non-labeled samples, respectively; “a” refers to a plant organ (whole plant, root, shoot) or a soil fraction (bulk, rhizosphere, POM, MIN, DOC, MBC); and “TCa” is the total carbon content (mg kg−1) of a.
Net assimilated 13C was calculated using EquationEquation 2(2)
(2) :
The photosynthesized 13C distribution in plant and soil was calculated as follows:
where 13Cproportion(a) is the proportion of total photosynthesized 13C amount in the plant-soil system represented by a (%);
To calculate 13C amount incorporated into plant organs (whole plant, root, shoot) or soil fractions (bulk, rhizosphere, POM, MIN) in each pot (as mg 13C kg−1 soil), the following equation was used:
where 13Camount(a) is 13C amount of a in each pot (mg 13C kg−1); and Ma is the mass (kg kg−1) of a in each pot.
13C incorporated into microbial biomass (13C-MBC) was calculated as follows:
where (atom 13C%) indicates atom 13C% in the fumigated (f) and unfumigated (uf) soil extract, and C is the TC content of the soil extract. “L,” and “NL” are labeled, and non-labeled samples, respectively. Thus, the amount of 13C allocated into MBC was calculated from 13C in fumigated soil minus that in unfumigated soil divided by a conversion factor of 0.45 (Vance, Brookes, and Jenkinson Citation1987).
The rhizosphere effect was calculated as the amount of 13C in rhizosphere soil divided by the amount of 13C in bulk soil.
The effect of fertilization (four factor levels: control, N, P, and NP) on the contribution of 13C to soil and plant pools was tested using one-way ANOVAs, and Tukey’s post-hoc tests were performed after the residuals were checked for normality and homogeneity.
Results
Effect of fertilization on plant growth
At D14, maximum shoot biomass was found with N and NP fertilization treatments (p < 0.001). While, at D22, highest shoot biomass was found under P and NP treatments. In contrast, root biomass was largely independent of fertilization on D14 (), and significantly affected by P application on D22 (p < 0. 05). Shoot C followed the same trends as the shoot biomass, and highest values were found on D14 for NP compared to control (1.8-fold that of the control). On D22, the respective increase in shoot C under NP was 2.21-fold that of the control. Nitrogen content increased in the shoots and roots under all treatments compared to the non-fertilized treatment, with the maximum amount found in response to the combined treatment (NP) on D22. Thus, the NP treatment had the greatest effect on shoot growth and C content of the shoot biomass.
Table 1. Biomass, total C (TC), total N (TN), and C/N ratio of rice plant roots and shoots on day 14 and 22 of 13C continuous labeling, and results of the ANOVA used to analyze the effects of N, P, and NP fertilization on these parameters. Values represent the means (±SE), n = 3
Distribution of Photosynthesized 13C in rice-soil system
Maximum content of net photosynthesized rice 13C (shoot and root + rhizo and bulk soil) ranged from 2247 to 16,635 mg 13C m−2 soil during the labeling period, with maximum values observed in response to NP (Figure S1; P < 0.001). The main fraction of the photosynthesized 13C was retained in the shoots (55.12–77.40%), whereas only 25–44% C was transferred by the rice plant belowground through rhizodeposition (). Maximum 13C was found in the shoots in response to NP on D14 (P < 0.001), whereas on D22, the proportion of 13C increased in response to all other treatments compared to that in the control. In contrast, the roots incorporated the lowest proportion of 13C under NP on D14. For all treatments (except NP), the proportion of 13C in the roots decreased between D14 and D22. Two to three times more 13C was found in the rhizosphere than that in bulk soil, with the maximum proportion (6–10%) observed under rhizosphere soil in response to N treatment on both sampling days (), while no effect was observed for the other fertilizer treatments. In bulk soil, a significant proportion of 13C was found in P (5.88% on D14 and 3.50% on D22) and control (4.1% on D14 and 3.6% on D22) treatments compared to that in N and NP treatments (P < 0.05). Thus, N and NP application promoted the proportion of 13C assimilated by the shoots, and N fertilization was responsible for the most input of C into the rhizosphere.
Figure 1. The photosynthesized 13C distribution in rice plant shoots, roots, rhizosphere, and bulk soil following fertilization on day 14 and 22 of 13C continuous labeling. Values represent the means ± SE, n = 3. Lowercase letters indicate significant differences between treatments within the same C pool i.e., shoot, root, rhizosphere (rhizo), and bulk soil (bulk) (p < 0.05)
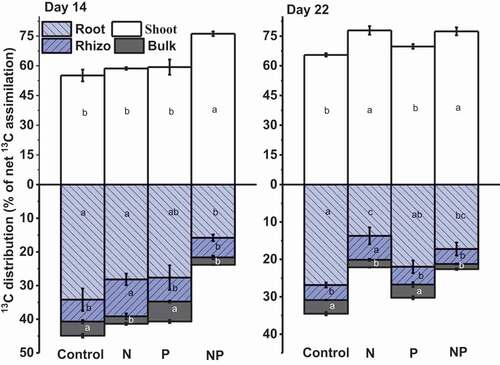
Effect of fertilization on 13C partitioning between organic matter pools
The proportion of assimilated 13C allocated into the POM fraction of the rhizosphere soil was 1.5–2 times higher than that assimilated into the MIN on both sampling days, with the maximum proportion observed in response to N and NP application (; P < 0.001). However, C allocated to the POM was not affected by the application of P alone on D22. The proportion of 13C in the MIN fraction was independent of fertilizer treatment in the rhizosphere soil on both sampling days. For bulk soil, both the POM and MIN fractions contained similar proportions of 13C, with a maximum amount observed in response to P treatment. Thus, the newly fixed C was preferentially incorporated into the POM fraction in response to N treatment, whereas the MIN fraction was largely unaffected by the addition of fertilizers.
Figure 2. Proportion of 13C-rhizodeposits in particulate (POM) and mineral (MIN) fractions of rhizosphere and bulk soils on day 14 and 22 of continuous 13C labeling. POM: particulate organic matter; MIN: mineral associated organic C. Values represent the means ± SE, n = 3. Lowercase letters indicate significant differences between treatments within the same C pool i.e., rhizosphere (rhizo) and bulk soil (bulk) (p < 0.05)
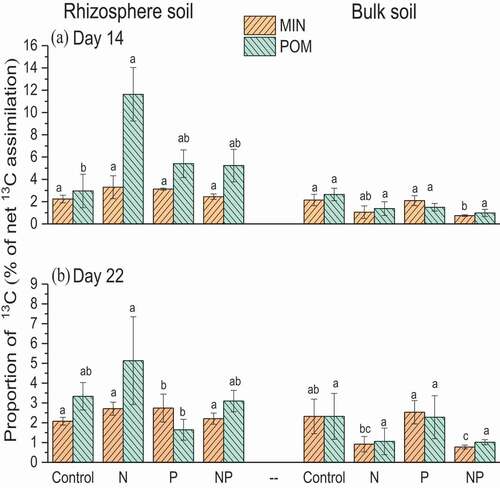
In rhizosphere soil, the amount of 13C incorporated into microbial biomass (MBC) was two times higher in case of N and NP than that in case of control and P treatment on D14 (Figure S2; P < 0.001) and tended to increase 3.5 times on D22 (Figure S2; P < 0.001). The DOC pool contained 2–5 times less 13C compared to the MBC for both rhizosphere and bulk soil, with significant amounts found for the rhizosphere soil (except the control) (). The proportion of 13C-MBC in the total MBC ranged from 0.005% to 0.32%, and was significantly higher under N and NP than that in the other treatments (Figure S2; P < 0.005), which suggests that the activity of microorganisms is higher under these fertilization regimes. The proportion of 13C in the DOC under N was two times higher than under the other treatments on D14 in rhizosphere soil, and two times higher under NP in bulk soil (; P < 0.05). The N treatment induced a higher 13C-rhizo/13C-bulk ratio compared to that observed in the other treatments on both sampling days (; P < 0.001). Assimilated 13C allocated into plant shoots and roots was significantly correlated with that incorporated into the MBC, DOC, SOC, MIN, and POM fractions (Table S3; P < 0. 01).
Table 2. The rhizosphere effect (rhizo/bulk ratio) of 13C allocation in soil organic carbon (SOC), dissolved organic carbon (DOC), microbial biomass carbon (MBC), mineral (MIN), and particulate organic matter (POM) fractions following fertilization treatments, and results of the ANOVA used to analyze the effects of N, P, and NP fertilization on these parameters. Values represent the means (±SE), n = 3
Figure 3. Proportion of 13C incorporated into dissolved organic C (DOC) and microbial biomass C (MBC) as % from total assimilated 13C. Values represent the means ± SE, n = 3. The lower-case letters indicate significant differences between treatments within the same C pool i.e., shoot, root, rhizosphere (rhizo) and bulk soil (bulk) (p < 0.05)
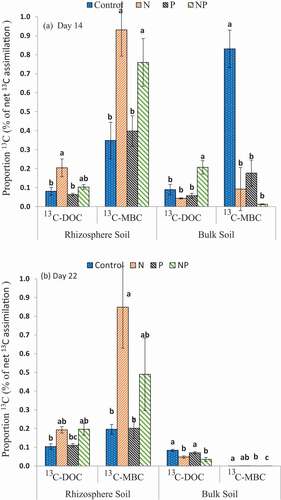
Discussion
Effect of fertilization on plant biomass and 13C allocation into SOM pools
The main primary sources of organic C in soils are plant residues and root exudates released into the rhizosphere (Ge et al. Citation2013), and the amount of these inputs depends on the life cycle of the plant and soil fertility, which are both strongly affected by the application of N and P fertilizers (Gu et al. Citation2009; Peng, Tang, and Zou Citation2009b; Chun et al. Citation2013; Chen et al. Citation2016; Guo et al. Citation2019). The application of N, P, and NP increased shoot and root biomass and reduced the C/N ratio of the roots particularly on D22 (). These effects can be attributed to an increase in chlorophyll content, especially under N application, and thus, rubisco enzyme activity, which promotes CO2 assimilation and consequently increases plant biomass (Xiao et al. Citation2019). Besides slowing C turnover in the above ground parts, fertilization can reduce the loss of assimilated 13C into the soil under N application (Xiao et al. Citation2019). The main fraction of the assimilated 13C was retained in the shoot mass under N and NP (; Leake et al. Citation2006), and less C was transferred to the roots compared to that observed in the case of the unfertilized treatment (control). This follows the resource optimization hypothesis, which indicates that when nutrient availability is increased, plant invest less effort in resource acquisition (Jones, Nguyen, and Finlay Citation2009).
The levels of newly assimilated C derived and fixed in the soil were also enhanced by the N application, particularly during the early growth stage (D14) and decreased with time (D22), which indicates that this fertilizer treatment, as well as C inputs in the form of rhizodeposits, can be used as the main treatment to promote high growth rates in rice. This finding supports our hypothesis that with fertilization, more photosynthetically fixed carbon would be exuded into the soil during the early growth stage, with N having a stronger effect than P. This may be because N fertilization stimulates the release of root exudates into the soil, whereas under P fertilization, the newly assimilated C could be retained for new root production or rapidly mineralized by microorganisms.
The amount of rhizo-derived 13C decreased from the root surface (rhizosphere soil) to the bulk soil (), because of the following reasons: i) the limitations of root exudate diffusion (Vale et al. Citation2005) and ii) the newly assimilated C is taken up and utilized rapidly by microorganisms (Gunina and Kuzyakov Citation2015; Zhu et al. Citation2016; Zhao et al. Citation2019). The relative proportion of 13C left in the soil also decreased with time ( and Figure S1), as it was decomposed by microorganisms. The fractionation method partitions the SOM pool into two fractions, MIN (<53 µm), composed of particles with large surface area (silt- and clay-sized particles); and POM (53–200 µm), which is related to macro and microaggregates. As newly photosynthetically fixed carbon is firstly allocated into large particles (macroaggregates) and then later redistributed into the microaggregate fraction (Atere et al. Citation2018a), it is clear why the POM pool was the dominant fraction that 13C entered into (), and why it decreased with time. In contrast, 13C associated with the MIN was stable during the experimental period, which shows that C associated with this fraction was more stable with respect to microbial utilization. More 13C was incorporated into the POM than the MIN fraction under fertilization treatments. This agrees with the results of Yan et al. (Citation2013), who found that the accumulation of SOC and its change in response to fertilization was significantly correlated with the carbon associated with the POM fraction, while that associated with the silt or clay fractions was independent of fertilization. Luo et al. (Citation2018) also found that N application increases the incorporation of newly fixed C into macroaggregates, but not into microaggregates, that is silt, or clay fractions (Luo et al. Citation2018), as the roots are usually located within large macroaggregates. Besides, roots also stimulate the formation of macroaggregates from microaggregates, silt, or clay fractions, leading to a decrease in newly photosynthesized C incorporated in small fractions. Thus, these results partly support our second hypothesis and show that N and P promote the allocation of newly fixed C into the POM fraction only, while the mineral fraction remains largely unaffected by fertilization.
Effect of fertilization on 13C allocation into the microbial biomass pool
The proportion of photosynthesized 13C incorporated into the MBC was 0.35–0.93% on D14 and 0.20–0.85% on D22 in rhizosphere soil, and was very low in the bulk soil except for the control treatment (). These data are in the range reported for pulse labeling of rice, i.e.,, 0.15–0.94% (Lu et al. Citation2002b). The incorporation of photosynthate-derived C into the MBC of rhizosphere soil was 2–3 times higher under N and NP than that in the control and P treatment on both sampling days (Figure S2; P < 0.001). Such results are associated with the decrease in the soil C/N ratio under fertilization (Table S2; P < 0.005), which can stimulate microbial activity, and can also be attributed to changes in the C/N ratio of root exudates, which make them more available to microorganisms (Wei et al. Citation2017). The proportion of 13C-MBC in the total MBC pool showed that the activity of microorganisms was significantly higher under N and NP fertilization on both sampling days (Figure S3; P < 0.005). This shows that rice-photosynthesized C inputs stimulated soil microorganisms, particularly in the rhizosphere (Lu, Watanabe, and Kimura Citation2004).
Conclusions
Overall, N and NP fertilization enhanced the net photosynthesized rice 13C in the rice-soil system compared to that of the unfertilized treatment (or control). The main fraction of the photosynthesized rice 13C (55–77%) was retained in the shoots; however, 25–44% was transferred into the roots (). Rhizosphere soil contained 2–3 times more 13C than bulk soil, which showed that the main accumulation of C in paddy soils occurred in the root zone. The greatest incorporation of 13C into the MBC pool was found in response to N and NP fertilization (on both D14 and D22), which showed that microorganisms are more active under these conditions. The main proportion of 13C in the rhizosphere and bulk parts was found in the POM on D14, whereas it decreased over time (on D22) owing to microbial utilization. In contrast, root-derived 13C in the MIN remained unchanged between sampling dates, which showed that stable rhizodeposits in this fraction can be the potential mechanism underlying SOM sequestration in paddy soils (). The main effects of fertilization on the stabilization of newly formed rhizodeposits were observed under NP treatments, indicating that this is a more promising fertilizer treatment for sustaining SOM levels under rice production.
Supplemental Material
Download MS Word (268.8 KB)Acknowledgments
We would like to thank the Public Service Technology Center, Institute of Subtropical Agriculture for providing access and facilities to conduct the experiment, and Chinese Academy of Sciences for their technical assistance.
Disclosure statement
No potential conflict of interest was reported by the author(s).
Supplementary materials
Supplemental data for this article can be accessed here.
Additional information
Funding
References
- An, T., S. Schaeffer, S. Li, S. Fu, J. Pei, H. Li, J. Zhuang, M. Radosevich, and J. Wang. 2015. “Carbon Fluxes from Plants to Soil and Dynamics of Microbial Immobilization under Plastic Film Mulching and Fertilizer Application Using 13C Pulse-labeling.” Soil Biology and Biochemistry 80: 53–11. doi:https://doi.org/10.1016/j.soilbio.2014.09.024.
- Atere, C. T., T. Ge, Z. Zhu, S. Liu, X. Huang, O. Shibsitova, G. Guggenberger, and J. Wu. 2019. „Assimilate allocation by rice and carbon stabilisation in soil: effect of water management and phosphorus fertilisation.„ Plant and Soil 445 (1):153-167. doi:https://doi.org/10.1007/s11104-018-03905-x.
- Bowatte, S., R. Tillman, A. Carran, and A. Gillingham. 2006. „Can phosphorus fertilisers alone increase levels of soil nitrogen in New Zealand hill country pastures?„ Nutrient Cycling in Agroecosystems 75 (1):57-66. doi:https://doi.org/10.1007/s10705-006-9011-4.
- Bradford, M. A., N. Fierer, and J. F. Reynolds. 2008. “Soil Carbon Stocks in Experimental Mesocosms are Dependent on the Rate of Labile Carbon, Nitrogen and Phosphorus Inputs to Soils.” Functional Ecology 22 (6): 964–974. doi:https://doi.org/10.1111/j.1365-2435.2008.01404.x.
- Cambardella, C. A., and E. T. Elliott. 1993. „Carbon and nitrogen distribution in aggregates from cultivated and native grassland soils.„ Soil Science Society of America Journal 57 (4):1071-1076. doi: https://doi.org/https://doi.org/10.2136/sssaj1993.03615995005700040032x.
- Chen, A., X. Xie, M. Dorodnikov, W. Wang, T. Ge, O. Shibistova, W. Wei, and G. Guggenberger. 2016. “Response of Paddy Soil Organic Carbon Accumulation to Changes in Long-term Yield-driven Carbon Inputs in Subtropical China.” Agriculture, Ecosystems & Environment 232: 302–311. doi:https://doi.org/10.1016/j.agee.2016.08.018.
- Chun, L. Y., H. Y. Gao, R. C. Zhong, G. L. Chun, W. C. Long, Y. Jiang, W. X. Jiao, H. Phendukani, and Z. Z. Hai. 2013. “Effects of Nitrogen Application on Chlorophyll Fluorescence Parameters and Leaf Gas Exchange in Naked Oat.” Journal of Integrative Agriculture 12 (12): 2164–2171. doi:https://doi.org/10.1016/S2095-3119(13)60346-9.
- Creamer, C. A., D. L. Jones, J. A. Baldock, Y. Rui, D. V. Murphy, F. C. Hoyle, and M. Farrell. 2016. “Is the Fate of Glucose-derived Carbon More Strongly Driven by Nutrient Availability, Soil Texture, or Microbial Biomass Size?” Soil Biology and Biochemistry 103: 201–212. doi:https://doi.org/10.1016/j.soilbio.2016.08.025.
- Derrien, D., C. Marol, and J. Balesdent. 2004. “The Dynamics of Neutral Sugars in the Rhizosphere of Wheat: An Approach by 13C Pulse-labelling and GC/C/IRMS.” Plant and Soil 267 (1): 243–253. doi:https://doi.org/10.1007/s11104-005-5348-8.
- Devêvre, O. C., and W. R. Horwáth. 2000. “Decomposition of Rice Straw and Microbial Carbon Use Efficiency under Different Soil Temperatures and Moistures.” Soil Biology and Biochemistry 32 (11–12): 1773–1785. doi:https://doi.org/10.1016/S0038-0717(00)00096-1.
- FAO Rice Market Monitor. April 2011. XIV. 2. P2. http://www.fao.org/docrep/014/am491e/am491e00.pdf
- Ge, T., C. Liu, H. Yuan, and Z. Zhao. 2015. “Tracking the Photosynthesized Carbon Input into Soil Organic Carbon Pools in a Rice Soil Fertilized with Nitrogen.” Plant and Soil 392 (1):17–25. doi:https://doi.org/10.1007/s11104-014-2265-8.
- Ge, T., H. Yuan, H. Zhu, X. Wu, S. Nie, C. Liu, C. Tong, J. Wu, and P. Brookes. 2012. „Biological carbon assimilation and dynamics in a flooded rice–soil system.„ Soil Biology and Biochemistry 48:39-46. doi:https://doi.org/10.1016/j.soilbio.2012.01.009.
- Ge, T., X. Wu, X. Chen, H. Yuan, Z. Zou, B. Li, P. Zhou, et al. 2013. “Microbial Phototrophic Fixation of Atmospheric CO2 in China Subtropical Upland and Paddy Soils.” Geochimica et Cosmochimica Acta 113: 70–78. doi:https://doi.org/10.1016/j.gca.2013.03.020.
- Ge, T., Y. Luo, and X. He. 2019. “Quantitative and Mechanistic Insights into the Key Process in the Rhizodeposited Carbon Stabilization, Transformation and Utilization of Carbon, Nitrogen and Phosphorus in Paddy Soil.” Plant and Soil 445 (1):1–5. doi:https://doi.org/10.1007/s11104-019-04347-9.
- Grandy, A. S., and G. P. Robertson. 2007. “Land-use Intensity Effects on Soil Organic Carbon Accumulation Rates and Mechanisms.” Ecosystems 10 (1): 58–73. doi:https://doi.org/10.1007/s10021-006-9010-y.
- Gu, Y., X. Zhang, S. Tu, and K. Lindstro. 2009. “Soil Microbial Biomass, Crop Yields, and Bacterial Community Structure as Affected by Long-term Fertilizer Treatments under Wheat-rice Cropping.” European Journal of Soil Biology 45 (3): 239–246. doi:https://doi.org/10.1016/j.ejsobi.2009.02.005.
- Gunina, A., and Y. Kuzyakov. 2015. “Sugars in Soil and Sweets for Microorganisms: Review of Origin, Content, Composition and Fate.” Soil Biology and Biochemistry 90: 87–100. doi:https://doi.org/10.1016/j.soilbio.2015.07.021.
- Guo, J., S. Yang, Y. Sun, Y. Kong, N. Ling, Q. Shen, and S. Guo. 2019. “Nitrogen Nutrient Index and Leaf Function Affect Rice Yield and Nitrogen Efficiency.” Plant and Soil 445 (1):7-21. doi:https://doi.org/10.1007/s11104-019-04076-z.
- Hew, C.-S., G. Krotkov, and D. T. Canvin. 1969. “Effects of Temperature on Photosynthesis and CO2 Evolution in Light and Darkness by Green Leaves.” Plant Physiology 44 (5): 671–677. doi:https://doi.org/10.1104/pp.44.5.671.
- Hütsch, B. W., J. Augustin, and W. Merbach. 2002. “Plant Rhizodeposition - an Important Source for Carbon Turnover in Soils.” Journal of Plant Nutrition and Soil Science 165 (4): 397–407. doi:https://doi.org/10.1002/1522-2624(200208)165:4<397::AID-JPLN397>3.0.CO;2-C.
- Jones, D. L., C. Nguyen, and R. D. Finlay. 2009. “Carbon Flow in the Rhizosphere: Carbon Trading at the Soil-root Interface.” Plant and Soil 321 (1): 5–33. doi:https://doi.org/10.1007/s11104-009-9925-0.
- Kogel-Knabner, I. 2002. “The Macromolecular Organic Composition of Plant and Microbial Residues as Inputs to Soil Organic Matter.” Soil Biology and Biochemistry 34 (2): 139–162. doi:https://doi.org/10.1016/S0038-0717(01)00158-4.
- Kuzyakov, Y., and W. Cheng. 2001. “Photosynthesis Controls of Rhizosphere Respiration and Organic Matter Decomposition.” Soil Biology and Biochemistry 33 (14): 1915–1925. doi:https://doi.org/10.1016/S0038-0717(01)00117-1.
- Leake, J. R., N. J. Ostle, J. I. Rangel-Castro, and D. Johnson. 2006. “Carbon Fluxes from Plants through Soil Organisms Determined by Field 13CO2 Pulse-labelling in an Upland Grassland.” Applied Soil Ecology 33 (2): 152–175. doi:https://doi.org/10.1016/j.apsoil.2006.03.001.
- Liu, Y., H. Zang, T. Ge, J. Bai, S. Lu, P. Zhou, P. Peng, et al.. 2018. “Intensive Fertilization (N, P, K, Ca, and S) Decreases Organic Matter Decomposition in Paddy Soil.” Applied Soil Ecology 127: 51–57. doi:https://doi.org/10.1016/j.apsoil.2018.02.012.
- Liu, Y., T. Ge, J. Ye, S. Liu, O. Shibistova, P. Wang, J. Wang, et al.. 2019a. “Initial Utilization of Rhizodeposits with Rice Growth in Paddy Soils: Rhizosphere and N Fertilization Effects.” Geoderma 338: 30–39. doi:https://doi.org/10.1016/j.geoderma.2018.11.040.
- Liu, Y., T. Ge, Z. Zhu, Y. Li, Y. Li, P. Wang, O. Gavrichkova, et al.. 2019b. “Carbon Input and Allocation by Rice into Paddy Soils : A Review.” Soil Biology and Biochemistry 133: 97–107. doi:https://doi.org/10.1016/j.soilbio.2019.02.019.
- Lu, Y., A. Watanabe, and M. Kimura. 2002. “Input and distribution of photosynthesized carbon in a flooded rice soil.” Global Biogeochemical Cycles 16 (4):32-1–8. doi:https://doi.org/10.1029/2002GB001864.
- Lu, Y., A. Watanabe, and M. Kimura. 2004. “Contribution of Plant Photosynthates to Dissolved Organic Carbon in a Flooded Rice Soil.” Biogeochemistry 71 (1):1–15. doi:https://doi.org/10.1007/s10533-004-3258-0.
- Luo, Y., Z. Zhu, S. Liu, P. Peng, J. Xu, P. Brookes, T. Ge, and J. Wu. 2018. “Nitrogen Fertilization Increases Rice Rhizodeposition and Its Stabilization in Soil Aggregates and the Humus Fraction.” Plant and Soil 445 (1):125-35. https://doi.org/https://doi.org/10.1007/s11104-018-3833-0
- Maclean, J. L., D. C. Dawe, B. Hardy, and G. P. Hettel, eds. 2002. Rice Almanac. 3rd Ed. CABI Publishing, Wallingford, United Kingdom.
- Mandal, B., B. Majumder, T. K. Adhya, P. K. Bandyopadhyay, A. Gangopadhyay, D. Sarkar, M. C. Kundu, et al.. 2008. “Potential of Double-cropped Rice Ecology to Conserve Organic Carbon under Subtropical Climate.” Global Change Biology 14 (9): 2139–2151. doi:https://doi.org/10.1111/j.1365-2486.2008.01627.x.
- Mo, F., Y. Y. Zhang, T. Li, Z. T. Wang, K. L. Yu, X. X. Wen, Y. C. Xiong, J. Z K, and Y. C. Liao. 2019. “Fate of Photosynthesized Carbon as Regulated by Long–term Tillage Management in a Dryland Wheat Cropping System.” Soil Biology and Biochemistry 138: 107581. doi:https://doi.org/10.1016/j.soilbio.2019.107581.
- Mwafulirwa, L., E. M. Baggs, J. Russell, T. George, N. Morley, A. Sim, C. de la Fuente Cantó, and E. Paterson. 2016. “Barley Genotype Influences Stabilization of Rhizodeposition-derived C and Soil Organic Matter Mineralization.” Soil Biology and Biochemistry 95: 60–69. doi:https://doi.org/10.1016/j.soilbio.2015.12.011.
- Olk, D. C., G. Brunetti, and N. Senesi. 2000. “Decrease in Humification of Organic Matter with Intensified Lowland Rice Cropping A Wet Chemical and Spectroscopic Investigation.” Soil Science Society of America Journal 64 (4): 1337–1347. doi:https://doi.org/10.2136/sssaj2000.6441337x.
- Oo, A. Z., S. Sudo, K. Inubushi, U. Chellappan, A. Yamamoto, K. Ono, M. Mano, et al.. 2018. “Mitigation Potential and Yield-scaled Global Warming Potential of Early-season Drainage from a Rice Paddy in Tamil Nadu, India.” Agronomy 8 (10):202. doi:https://doi.org/10.3390/agronomy8100202.
- Peng, S., Q. Tang, and Y. Zou. 2009a. “Current Status and Challenges of Rice Production in China.” Plant Production Science 12 (1): 3–8. doi:https://doi.org/10.1626/pps.12.3.
- Peng, S., Q. Tang, and Y. Zou. 2009b. “Current Status and Challenges of Rice Production in China.” Plant Production Science 12 (1): 3–8. doi:https://doi.org/10.1626/pps.12.3.
- Saggar, S., C. Hedley, and A. D. Mackay. 1997. “Partitioning and Translocation of Photosynthetically Fixed 14C in Grazed Hill Pastures.” Biology and Fertility of Soils 25: 152–158. doi:https://doi.org/10.1007/s003740050296.
- Schlesinger, W. H., and J. Lichter. 2001. “Limited Carbon Storage in Soil and Litter of Experimental Forest Plots under Increased Atmospheric CO2.” Nature 411 (6836): 466–469. doi:https://doi.org/10.1038/35078060.
- Sun, Z., Q. Chen, X. Han, R. Bol, B. Qu, and F. Meng. 2018. “Allocation of Photosynthesized Carbon in an Intensively Farmed Winter Wheat-soil System as Revealed by 14CO2 Pulse Labelling.” Scientific Reports 8: 6–15. doi:https://doi.org/10.1038/s41598-017-18627-w.
- Tian, J., J. Pausch, M. Fan, X. Li, Q. Tang, and Y. Kuzyakov. 2013. “Allocation and Dynamics of Assimilated Carbon in Rice-soil System Depending on Water Management.” Plant and Soil 363 (1–2): 273–285. doi:https://doi.org/10.1007/s11104-012-1327-z.
- Vale, M., C. Nguyen, E. Dambrine, and J. L. Dupouey. 2005. “Microbial Activity in the Rhizosphere Soil of Six Herbaceous Species Cultivated in a Greenhouse Is Correlated with Shoot Biomass and Root C Concentrations.” Soil Biology and Biochemistry 37 (12): 2329–2333. doi:https://doi.org/10.1016/j.soilbio.2005.04.014.
- Vance, E., P. Brookes, and D. Jenkinson. 1987. “An Extraction Method for Measuring Soil Microbial Biomass C.” Soil Biology & Biochemistry 19 (6): 703–707. doi:https://doi.org/10.1016/0038-0717(87)90052-6.
- von Lützow, M., I. Kögel-Knabner, K. Ekschmitt, H. Flessa, G. Guggenberger, E. Matzner, and B. Marschner. 2007. “SOM Fractionation Methods: Relevance to Functional Pools and to Stabilization Mechanisms.” Soil Biology and Biochemistry 39 (9): 2183–2207. doi:https://doi.org/10.1016/j.soilbio.2007.03.007.
- Van Ginkel, J. H., A. Gorissen, and J. A. van Veen. 1997. “Carbon and Nitrogen Allocation in Lolium Perenne in Response to Elevated Atmospheric CO2 with Emphasis on Soil Carbon Dynamics.” Plant and Soil. 188 (2): 299–308. https://doi.org/https://doi.org/10.1023/A:1004233920896
- Wei, L., T. Ge, Z. Zhu, Y. Luo, Y. Yang, M. Xiao, Z. Yan, Y. Li, J. Wu, and Y. Kuzyakov, 2021. „Comparing carbon and nitrogen stocks in paddy and upland soils: Accumulation, stabilization mechanisms, and environmental drivers„ Geoderma 398:115121.
- Wei, X., Y. Hu, P. Peng, Z. Zhu, C. T. Atere, O. A G, J. Wu, and T. Ge. 2017. “Effect of P Stoichiometry on the Abundance of Nitrogen-cycle Genes in Phosphorus-limited Paddy Soil.” Biology and Fertility of Soils 53 (7): 767–776. doi:https://doi.org/10.1007/s00374-017-1221-1.
- Wu, J., R. G. Joergensen, B. Pommerening, R. Chaussod, and P. C. Brookes. 1990. „Measurement of soil microbial biomass C by fumigation-extraction—an automated procedure. „ Soil Biology and Biochemistry 22 (8):1167–1169. https://doi.org/https://doi.org/10.1016/0038-0717(90)90046-3
- Xiao, M., H. Zang, S. Liu, R. Ye, Z. Zhu, Y. Su, J. Wu, and T. Ge. 2019. “Nitrogen Fertilization Alters the Distribution and Fates of Photosynthesized Carbon in Rice – Soil Systems : A 13 C-CO 2 Pulse Labeling Study.” Plant and Soil 445 (1–2): 101–112. doi:https://doi.org/10.1007/s11104-019-04030-z.
- Xu, Y., H. Tang, X. Xiao, W. Li, C. Li, and G. Sun. 2018. “Effects of Long-Term Fertilization Management Practices on Soil Microbial Carbon and Microbial Biomass in Paddy Soil at Various Stages of Rice Growth.” Revista Brasileira de Ciência do Solo 42:1–12. https://doi.org/https://doi.org/10.1590/18069657rbcs20170111
- Yan, X., H. Zhou, Q. H. Zhu, X. F. Wang, Y. Z. Zhang, X. C. Yu, and X. Peng. 2013. “Carbon Sequestration Efficiency in Paddy Soil and Upland Soil under Long-term Fertilization in Southern China.” Soil and Tillage Research 130: 42–51. doi:https://doi.org/10.1016/j.still.2013.01.013.
- Zhao, Z., T. Ge, A. Gunina, and Y. Li. 2019. “Carbon and nitrogen availability in paddy soil affects rice photosynthate allocation, microbial community composition, and priming : combining continuous C labeling with PLFA analysis.” Plant and Soil 445 (1):137–152. https://doi.org/https://doi.org/10.1007/s11104-018-3873-5.
- Zhu, Z., G. Zeng, T. Ge, Y. Hu, C. Tong, O. Shibistova, X. He, J. Wang, G. Guggenberger, and J. Wu. 2016. “Fate of Rice Shoot and Root Residues, Rhizodeposits, and Microbe-assimilated Carbon in Paddy Soil - Part 1: Decomposition and Priming Effect.” Biogeosciences 13 (15): 4481–4489. doi:https://doi.org/10.5194/bg-13-4481-2016.