Abstract
Endophytic fungi are an important component, are ubiquitous and occur within all know plants, including a broad range of hosts in various ecosystems, and therefore play an important role in the natural environment. More than 1 million species of endophytic fungi are estimated to exist based on a ratio of vascular plants to fungal species of 1:4 or 1:5. Nevertheless, our recognition of endophyte diversity is limited at present. In surveys of endophyte diversity, traditional techniques, such as cultivation-dependent methods, have been routinely used in previous studies. The discovery of endophytic fungi in natural environments, however, has been limited by traditional methodology due to some non-sporulating and non-culturable fungi. Molecular techniques, such as DNA fingerprinting and sequencing methods, have been successfully employed in the detection and identification of endophytic fungi, and different endophyte diversity and community composition have been documented by cultivation-dependent and molecular techniques. This review paper summarises recent progress in the study of endophytic fungal diversity and some key questions are highlighted for future research in endophyte biology.
1. Endophytic fungi
The term “endophyte”, originally introduced by de Bary (Citation1866), refers to any organisms occurring within plant tissues, distinct from the epiphytes that live on plant surfaces. Carroll (Citation1986) defined endophytes as mutualists that colonise aerial parts of living plant tissues and do not cause symptoms of disease, from which pathogenic and mycorrhizal fungi are excluded. Petrini (Citation1991) proposed an expansion of Carroll's definition to include all organisms inhabiting plant organs that, at some time in their life, can colonise internal plant tissues without causing apparent harm to the host. Therefore, latent pathogens known to live symptomlessly inside host tissues that have an epiphytic phase in their life cycle are also endophytes. Bills (Citation1996) pointed out that endophytes and certain types of mycorrhizae, e.g. ectendomycorrhizae, ericoid mycorrhizae and pseudomycorrhizae, are indistinct. Therefore, certain mutualistic root-inhabited or mycorrhizal fungi associated with plants in the Ericaceae and Orchidaceae have been referred to as endophytes (Stoyke and Currah Citation1991; Bayman et al. Citation1997). Endophytic microorganisms may or may not grow in culture media, i.e. may or may not be cultivated, and can inhabit the interior of plant tissues and organs without causing damage to its host and without producing structures emerging from the external plant (Azevedo and Araújo Citation2007). The concept of endophyte has been defined in many ways and there have been many reviews and publications on the subject (reviewed by Hyde and Soytong Citation2008). However, while there are many alternatives, the definition of Petrini (Citation1991) has been most commonly used in endophyte studies.
Endophytic fungi have been associated with plants for over 400 million years (Krings et al. Citation2007), and have been widely studied in various geographical and climatic zones. They are ubiquitous and occur within all known plants, including a broad range of host orders, families, genera and species, in ecosystems as diverse as mosses (Davey and Currah Citation2006), ferns (Swatzell et al. Citation1996), grasses (Müller and Krauss Citation2005; Su et al. Citation2010), shrubs (Petrini et al. Citation1982), deciduous and coniferous trees (Guo et al. Citation2008; Albrectsen et al. Citation2010; Mohamed et al. Citation2010; Sun et al. Citation2011), and lichens (Suryanarayanan et al. Citation2005; Li et al. Citation2007a, b). Endophytic fungi mainly consist of members of the Ascomycota or their mitosporic fungi, as well as some taxa of the Basidiomycota, Zygomycota and Oomycota (Zheng and Jiang Citation1995; Sinclair and Cerkauskas Citation1996; Guo Citation2001), which can produce various bioactive chemicals (Liu et al. Citation2008, Citation2009, Citation2010, Citation2011; Aly et al. Citation2010; Xu et al. Citation2010; Tejesvi et al. Citation2011), promote host growth and resistance to environmental stress (Cheplick et al. Citation1989; Ting et al. Citation2008; Saikkonen et al. Citation2010), and decompose litter (Purahong and Hyde Citation2011; Sun et al. Citation2011). Therefore, endophytic fungi, as an important component of natural ecosystems, can play a key role in material and energy recycle.
Plant tissues are multilayered, spatially and temporally diverse microbial habitats, and thus support a rich and varied endophytic mycobiota that form specialised associations with various plant species. The accepted estimate of 1.5 million fungal species on Earth is primarily based on a ratio of vascular plants to fungal species of 1:6, but endophytic fungi have not been seriously considered in the estimation (Hawksworth Citation1991). Furthermore, Petrini (Citation1991) suggests that there could be more than 1 million species of endophytic fungi remaining to be discovered and described based on ratios of vascular plants to fungal species of 1:4 or 1:5.
2. Traditional techniques used in endophyte studies
Traditionally, endophytic fungi inside plant tissues can be recognised by two basic techniques, i.e. direct observation and cultivation-dependent methods. In the direct observation method, endophytic fungal structures within living plant tissues are directly examined under a light and electron microscope, which can show all endophytic mycobiota within the plant tissue, particularly biotrophic fungi that cannot be cultured on standard growth media (Deckert et al. Citation2001; Lucero et al. Citation2011). However, most endophytic fungi within plant tissue have only a hyphal structure, and therefore cannot be identified to any taxonomic category according to morphology due to lack of spore-producing structures and sexual or asexual spores. In addition, endophytic isolates cannot be obtained as microbial resources for further use with the direct observation method. Therefore, this is not commonly used in endophyte diversity studies (Deckert et al. Citation2001).
In contrast to direct observation methods, cultivation-dependent techniques have been routinely employed in endophyte diversity studies (e.g. Petrini et al. Citation1982; Rodrigues and Samuels Citation1990; Guo et al. Citation2000; Sun et al. Citation2011; Vieira et al. Citation2011). It is important to isolate endophytic fungi for further detailed studies into their characterisation, population dynamics, species diversity, or as inocula to improve plant growth and health, or screening for novel biologically active secondary metabolites (Ding et al. Citation2009; Wang et al. Citation2010; Li et al. Citation2011; Tejesvi et al. Citation2011). With cultivation-dependent techniques, the isolation procedure is a critical and important step in working with endophytic fungi. The living plant tissues are subjected to a serial process of surface sterilisation to remove all organisms from the surface of the plant. Only internal fungi are isolated by means of incubation of the plant samples onto nutrient plates. Cultivation-dependent techniques generally include (1) thorough washing of the plant tissue under tap water to remove adhering soil particles, debris and major epiphytes, (2) surface sterilisation of plant tissue to kill any microorganisms on the host surface, applying different protocols to different tissue types (Hallmann et al. Citation2006), (3) isolation of endophytic fungi growing out from samples placed on nutrient agar, (4) purification and sporulation of endophytic isolates under various incubation conditions, and (5) identification of the endophytic fungi based on morphological characteristics in cultures (; Wang et al. Citation2007; Li et al. Citation2007b; Guo et al. Citation2008; Su et al. Citation2010; Sun et al. Citation2011).
The isolation technique is a method-dependent process, although it is effective for rapid recovery of a large number of endophytic fungal species from plant tissues. Endophytic fungus communities obtained from plants, however, are directly affected by surface sterilisation techniques, incubation conditions, and whether isolates sporulate. To be effective, the isolation procedure must be adapted to the respective plant species, tissues and fungi. In practice, the isolated strains can be assumed to be endophytic fungi when total surface sterilisation is confirmed, i.e. no fungal growth from imprinting the surface-sterilised plant tissues onto nutrient media or culturing aliquot of water from the last rinsing onto nutrient media. To increase fungal diversity, the sterilised plant tissue is cut into small segments (ca. 5 mm diam.), macerated or ground, then transferred onto nutrient agar for incubation, which may uncover fast- and slow-growing fungi. A number of different media should be used in the isolation procedure, such as standard PDA (potato dextrose agar) and MEA (malt extract agar), as well as minimal media with plant tissue or extract. In addition, the tissue size and numbers of plant samples can affect the observed fungal diversity and community composition. For examples, Gamboa et al. (Citation2002) demonstrated that, in several tropic plants, the number of endophytic fungi obtained increases with the decreasing size of tissue fragments incubated. However, more endophytic taxa were recovered from the incubation of whole leaf (35 taxa) than leaf disks (5 mm diam., 9 taxa) of Acer truncatum (Sun et al. Citation2011). Petrini et al. (Citation1992) pointed out that 40 individuals of a given plant species and 30–40 sampling units from each individual will yield at least 80% of endophytic taxa assumed to be present at one site. Our study has shown that 88% of estimated fungal taxa were obtained from 40 tissue fragments (5 mm diam.) of 20 individual specimens of A. truncatum in a mixed temperate forest in northern China (; Sun et al. Citation2011).
Figure 2. Species accumulation curves for endophytic fungi isolated from tissue segments of Acer truncatum. Line, observed species accumulation curve; dots, 95% confidence interval of the species accumulation curve; dashed, bootstrap mean of species richness.
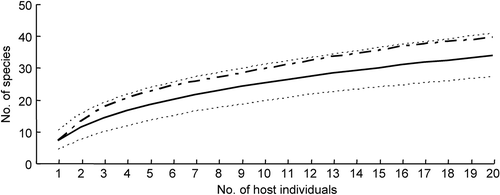
The large number of sterile isolates poses a special problem, because they cannot be identified to any taxonomic category according to morphological characteristics. Therefore, various methods have to be employed to promote isolate sporulation, such as the use of different media and inclusion of sterile host tissue in cultures. For example, Guo et al. (Citation1998) increased the initial 48% of sporulating isolates obtained from Livistona chinensis fronds to 59.5% by the addition of sterile palm leaf tissue onto the agar surface, and further increased the number of identifiable (sporulating) isolates to 83.5% by inoculating the remaining unidentified isolates onto sterile petiole pieces in conical flasks at room temperature for 3 months. In addition, some fungi may be missed due to failure to grow or grow slowly, and are easily outcompeted by fast-growing species under artificial conditions. The potential technical biases in traditional endophyte studies can be resolve by molecular techniques.
3. Molecular techniques used in endophyte studies
The development of molecular biology brings a new perspective to endophyte diversity studies. Application of molecular techniques, such as DNA fingerprinting and sequencing methods (), has the potential to overcome the obstacles in traditional cultivation-dependent methods.
3.1. Molecular identification of sterile mycelia
In traditional cultivation-dependent processes, fungal isolates can be identified from morphological characteristics if they sporulate on the media. Despite the development of various methods to promote sporulation (Guo et al. Citation1998, 2000; Taylor et al. Citation1999), high numbers of isolates (up to 54% of the total) do not sporulate in cultures (e.g. Petrini et al. Citation1982; Fisher et al. Citation1993; Guo et al. Citation2000, 2008; Photita et al. Citation2001; Cannon and Simmons Citation2002; Kumaresan and Suryanarayanan Citation2002; Wang and Guo Citation2007; Sun et al. Citation2008, Citation2011). Since conventional classification of fungi relies heavily on reproductive structures, these non-sporulating strains cannot be provided with taxonomic names. To appreciate the considerable diversity of these Mycelia sterilia, they are generally categorised as ‘morphotype’ based on similar cultural characteristics, such as colony colour, texture and growth rates (Guo et al. Citation2000, 2003; Wang et al. Citation2005; Sun et al. Citation2011). Arrangement of taxa into different morphotypes, however, does not reflect species phylogeny, because morphotypes are not real taxonomic entities.
Molecular methods are, therefore, required for the identification and understanding of the diversity of endophytic mycelia sterilia. In a survey of endophytic fungi from fronds of L. chinensis in Hong Kong, a large number of isolates (16.5% of total) did not sporulate, remaining as Mycelia sterilia (Guo et al. Citation2000). These non-sporulating isolates were grouped into 19 morphotypes based on their cultural morphology and identified to different genera (Diaporthe, Mycosphaerella and Xylaria), families (Pleosporaceae and Clypeosphaeriaceae), and order (Xylariales) based on ITS sequence analyses. Sun et al. (Citation2011) grouped 221 non-sporulating endophyte strains into 56 morphotypes, and placed these morphotypes into 37 taxa based on ITS sequence similarity and phylogenetic analyses (). Similarly, in a study of endophytic fungi of Pinus tabulaeformis in China, a large number of isolates (11% of total) remained as mycelia sterilia (Wang and Guo Citation2007). These non-sporulating isolates were grouped into 74 morphotypes according to their cultural morphology, and were further divided into 64 taxa based on ITS sequence analyses (Wang et al. Citation2005). Furthermore, a total of 18 sterile strains grouped in the white morphotype were identified into Rosellinia, Entoleuca and Nemania of Xylariaceae and members of Rhytismataceae by means of ITS sequence analyses, which demonstrated that some sterile isolates with similar cultural characteristics are distantly related taxa (Guo et al. Citation2003). In a survey of endophytic mycota associated with Vitis vinifera in central Spain, the non-sporulating strains (n = 78) were identified to the generic and species levels by comparing their ITS sequences with those in GenBank (González and Tello Citation2011). Therefore, molecular techniques can improve our recognition of fungal diversity in natural ecosystems, particular for non-sporulating isolates that cannot be identified into any taxonomic position based on morphology and are lost in the survey of diversity using traditional techniques.
Figure 3. Neighbour–Joining tree based on ITS (ITS1, 5.8S, ITS2) region sequences of endophyte morphotypes and references of Diaporthales. The tree is rooted with Xylaria hypoxylon of Xylariales. The numbers at each branch point represent percentage bootstrap support (more than 50%) calculated from 1000 replicate.
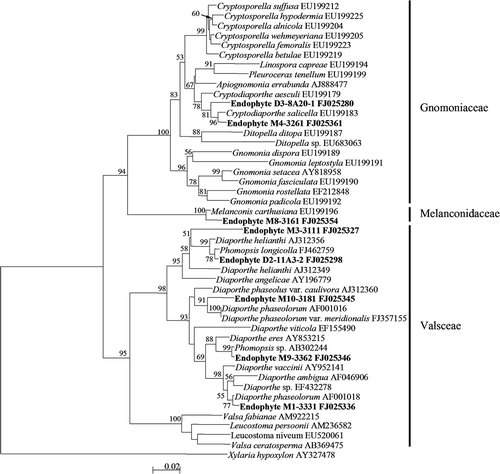
3.2. Molecular identification of isolate community
Endophytic fungus communities consist of a broad range of fungal origins, such as Ascomycota, Basidiomycota and Zygomycota (Zheng and Jiang Citation1995; Sinclair and Cerkauskas Citation1996). Therefore, it is a tough task for mycologists to identify various endophytic fungi into genera or species based on morphological characteristics. Furthermore, it is very time-consuming to make a complete identification. Therefore, DNA sequencing analyses coupled with morphology have been widely used in the investigation of endophyte diversity, particularly for ecology studies. For example, a total of 27 fungal genera belonging to Ascomycota, Zygomycota and Basidiomycota were isolated and identified from roots of Pseudotsuga menziesii and Pinus ponderosa using a combination of morphology and ITS sequence data (Hoff et al. Citation2004). Similarly, a total of 59 distinct ITS genotypes were isolated from asymptomatic foliage of Pinus taeda, which represented 24 and 37 unique groups based on 90% and 95% sequence similarity (Arnold et al. Citation2007). Ghimire et al. (Citation2011) identified the endophytic strains isolated from Panicum virgatum growing in native tallgrass prairies based on ITS sequence analyses, and found that a wide range of fungal species from at least 18 different taxonomic orders existed as endophytes in switchgrass plants and that the fungal communities from shoot tissues had significantly higher species diversity than those from root tissues. However, species diversity of endophytic fungi was much higher in roots than in leaves of Stipa grandis from the Inner Mongolian steppes of China (Su et al. Citation2010).
In endophyte studies, 18S and 28S genes have been employed in the identification of endophytic fungi at high taxonomic levels. For example, a total of 71 (of 257 strains) representative strains isolated from bamboos Phyllostachy and Sasa species were placed into Sordariomycetes and Dothideomycetes based on 18S gene sequence analyses. These strains were further identified into lower taxonomic levels according to ITS sequence data, and some strains may represent novel taxa (Morakotkarn et al. Citation2007). Similarly, a total of 47 distinct genotype groups based on 90% ITS sequence similarity were obtained from 280 representative strains isolated from Huperzia selago, Picea mariana and Dryas integrifolia in northern and southern boreal forest and Arctic tundra, and further phylogenetic analyses of combined data from 18S and 28S genes showed that these different genotypic endophytes represent Dothideomycetes, Sordariomycetes, Chaetothyriomycetidae, Leotiomycetes and Pezizomycetes of Ascomycota (Higgins et al. Citation2007). Fifty-nine of morphologically unidentifiable strains isolated from healthy stems and pods of Theobroma cacao trees were identified based on the sequence analyses of the 28S gene (Crozier et al. Citation2006). The majority of the isolates belonged to Basidiomycota, particularly to corticoid and polyporoid taxa. Of these isolates, some were rarely isolated genera, such as Byssomerulius, while the most commonly isolated basidiomycetous endophytes were members of the cosmopolitan genus Coprinellus (Agaricales). Botella and Diez (Citation2011) analysed the phylogenic diversity of fungal endophytes of Pinus halepensis based on the analyses of ITS and 28S gene sequences, and found that Dothideomycetes was the dominant class and further confirmed the endophytic stage of several pathogens previously associated with P. halepensis decline in Spain. Molecular sequencing techniques, therefore, can speed our recognition of fungal species diversity in natural ecosystems compared to morphology, regardless of isolate sporulation in cultures.
3.3. Molecular detection of endophytes within plant tissues
Due to the limitations of traditional isolation techniques, it is highly probable that some or even numerous endophytic fungi are never isolated. This is because some endophytic fungi cannot grow or grow slowly and are outcompeted by fast-growing species on the artificial media. To overcome the potential technical bias, molecular techniques have been employed in the detection of endophytic fungi directly within the host tissues. The procedure of molecular study generally involves (1) extraction of total genomic DNA (including fungi and plants) from surface-sterilised plant tissues, (2) amplification of DNA fragments (e.g. ITS, 28S and 18S genes) from total DNA with fungal-specific primers, (3) separation of PCR products (bands) by denaturing gradient gel electrophoresis (DGGE) and excision of different DGGE bands representing different taxa, (4) cloning of PCR products directly into plasmids (e.g. pGEM-T vector) and transferring into E. coli DH5α, (5) screening of positive clones for different taxa using DNA fingerprinting techniques (e.g. PCR-RFLP), (6) sequencing representative clones with different fingerprinting patterns and DGGE bands, and (7) theoretically identifying the sequences into various taxonomic levels based on phylogenetic analysis and sequence similarity comparison ().
In a previous Picea phylogeny study, a contaminated ITS sequence amplified from plant tissues was identified as Hormonema dematioides, a ubiquitous foliar endophyte of conifers (Camacho et al. Citation1997). A broad spectrum of fungal ITS sequences was directly amplified from genomic DNA extracted from Heterosmilax japonica tissues (Gao et al. Citation2005). some were identified as commonly isolated fungi – Aureobasidium, Botryosphaeria, Cladosporium, Glomerella, Mycosphaerella, Phomopsis and Guignardia – while others (e.g. YJ4-61, YJ4-9, YJ4-70) were similar to some uncultured environmental samples, which may represent novel fungal taxa (Gao et al. Citation2005). In our study, five fungal ITS regions were amplified directly from total DNA extracted from fronds of L. chinensis. Of these clone sequences, four were identified as the commonly isolated fungi Glomerella (anamorph Colletotrichum), Mycosphaerella (anamorph Cladosporium) and Herpotrichiellaceae of Ascomycetes; the other single sequence belonging to Basidiomycota was not found using traditional cultivation-dependent method (Guo et al. Citation2001). Endophytic fungal rDNA fragments (28S and ITS), amplified from surface-sterilised needles of 12 Pinus taeda trees (Arnold et al. Citation2007), showed that cloned endophytic fungi were distributed across multiple lineages of Ascomycota and Basidiomycota. Of these fungi, Dothideomycetes and Leotiomycetes of Ascomycota were dominant, which are commonly isolated from P. taeda using traditional cultural methods, but no Sordariomycetes were recovered from cloned endophytic sequences, despite the prevalence of this lineage among cultural endophytes. Similarly, a difference in diversity and community composition of endophytic fungi from the leaves of Rhododendron tomentosum was discovered using cultivation-dependent and molecular techniques (Tejesvi et al. Citation2011).
DGGE techniques, which are capable of separating closely related sequences by their differential mobilities in a gradient of denaturants, have recently been successfully applied to document endophytic fungal communities by excising and sequencing bands, thereby obtaining taxonomic information for members of the community via database searches and phylogenetic analysis (Götz et al. Citation2006). For example, Duong et al. (Citation2006) recovered 14 OTUs from Magnolia liliifera using DGGE coupled with sequencing, which were totally different from the morphospecies recovered by the traditional cultivation-dependent method (Promputtha et al. Citation2005). Despite the advantages of DGGE, there are also disadvantages. In general, shorter fragments (<500 bp) of DNA result in better resolution between bands in a profile, thereby limiting the taxonomic information to properly identify fungi at the generic or species levels (May et al. Citation2001; Duong et al. Citation2006). Moreover, even the most sensitive staining methods are often not sensitive enough to detect all the diversity present within a sample, particularly for rarer members of the fungal community (Anderson and Cairney Citation2004). Further studies should consider primers that are more universal for fungi and give better phylogenetic resolution at generic or species level.
The simple sequence repeat (SSR) marker technique is comparatively cheap, fast and easy to perform. It is similar to random amplified polymorphic DNA (RAPD) analysis but longer primers (ca. 18 nucleotides) are used and the conditions (e.g. annealing temperature) during amplification are more stringent. Furthermore, genomic regions containing microsatellites are evolving and mutating more rapidly than other areas of the genome (Levinson and Gutman Citation1987; Burgess et al. Citation2001). Therefore, the use of higher annealing temperatures and longer nucleotide primers results in highly reproducible SSR markers that are much more robust than the RAPD markers used (Peever et al. Citation2002; Liang et al. Citation2005). In endophyte studies, Groppe and Boller (Citation1997) developed specific primer pairs flanking a microsatellite-containing locus and successfully detected a rDNA fragment of endophytic Epichloë species from infected tissues of B. erectus, but no fragments were generated from total DNA isolated from uninfected plant material or unrelated fungi isolated from the same grass. The results of previous studies have shown that the diversity and community composition of endophytic fungi detected with molecular methods differ from that found using traditional cultivation-dependent methods.
4. High-throughput sequencing used in endophyte studies
The recently developed, high-throughput sequencing (pyrosequencing) enables metagenomic and metagenetic analyses and provides a powerful alternative to molecular studies of fungal community in natural environments. Pyrosequencing has the potential advantages of rapidity, being relatively inexpensive, with a free-cloning step and high product, which achieves an approximately 100-fold increase in throughput over Sanger sequencing technology (Margulies et al. Citation2005). This technique has been successfully employed in the study of fungal diversity in natural environments, such as clinical fungi (Gharizadeh et al. Citation2004), phyllosphere fungi (Jumpponen and Jones Citation2009), wood-inhabiting fungi (Ovaskainen et al. Citation2010), soil fungi (Jumpponen et al. Citation2010), freshwater fungi (Monchy et al. Citation2011), and mycorrhizal fungi (Lumini et al. Citation2010; Tedersoo et al. Citation2010; Dumbrell et al. Citation2011).
Pyrosequencing has potential advantages compared to traditional Sanger sequencing techniques in terms of cost, time, throughput of samples, recovery of more species, and providing a less biased qualitative picture of microbial community composition in natural ecosystems (Sogin et al. Citation2006; Öpik et al. Citation2009; Jumpponen et al. Citation2010). However, there are some technical biases which can affect fungal diversity in the pyrosequencing dataset (Bellemain et al. Citation2010; Tedersoo et al. Citation2010). For examples, DNA extraction methods have a substantial effect on the number of sequences and species recovered, which could be related to the differential ability to degrade cell walls and remove inhibitors. In addition to DNA extract, the primers employed strongly affect the number of fungal sequences recovered (Bellemain et al. Citation2010; Lumini et al. Citation2010; Tedersoo et al. Citation2010; Lucero et al. Citation2011). To overcome the PCR biases in environmental studies, DNA extraction protocols are optimised. Primers should cover all target taxa and multiple PCR replicates are used, and the number of PCR cycles is reduced to 20–25 (Taylor and McCormick Citation2008; Medinger et al. Citation2010; Tedersoo et al. Citation2010). In addition, the size of the recovered DNA fragments is not long enough in pyrosequencing (most have read-lengths from 350 to 500 bp), which may reduce the taxonomic resolution. Recently, Pacific Biosciences (Pacific Biosciences of California, Inc., Menlo Park, CA, USA) declares that an average read-length of 2700 bases had been achieved by single molecule real-time technology, which will be applied in future PacBio RS systems (http://www.pacificbiosciences.com). In an original pyrosequencing dataset, singletons account for the greatest source, and most singletons are artifactual and contain a strongly elevated proportion of insertions compared with natural intra- and inter-specific variation (Tedersoo et al. Citation2010). Therefore, automated species identification with adequate assessment of identification error will be necessary in future studies (Ovaskainen et al. Citation2010).
Pyrosequencing techniques significantly enhance the characterisation of fungal diversity compared to traditional Sanger sequencing methods. For example, Gillevet et al. (Citation2009) compared the number of different fungal ITS contigs from Spartina alterniflora in a salt-marsh by either clone sequencing or pyrosequencing methods, and demonstrated that pyrosequencing affords greater depth of coverage not only in terms of the number of different contigs detected but also in revealing entire clades missed by traditional sequencing. Pyrosequencing was first used in the investigation of endophytic fungi of Atriplex canescens and A. torreyi var. griffithsii (Lucero et al. Citation2011).
High-throughput sequencing represents an amazing technological feat that promises to reshape mycology, but a unified infrastructure for processing and interpretation of the results in a taxonomic context needs to be agreed upon and implemented. Significantly, Nilsson et al. (Citation2011) proposes a standardisation of the description and publication of next-generation sequencing datasets of fungal communities, which has benefits for future fungal diversity and ecology studies.
5. DNA barcode for endophytic fungi
DNA barcoding systems employ a short, effective and standardised gene region to identify species (Hebert et al. Citation2003). The inter-specific distance of the DNA barcode region should exceed the intra-specific distance, and identification is straightforward when a sequence is unique to a single species and constant within each species (Hebert et al. Citation2003; Letourneau et al. Citation2010). Ideally, the DNA barcode region used should be a single locus for all groups of organisms across all kingdoms. To date, DNA barcoding has been successfully used in the animal kingdom with a 648-bp region of cytochrome c oxidase 1 (CO1) gene (e.g. Hebert et al. Citation2003; Hajibabaei et al. Citation2006).
Mycologists have evaluated different DNA gene fragments for fungal DNA barcoding (Seifert Citation2009; Begerow et al. Citation2010; Letourneau et al. Citation2010; Stockinger et al. Citation2010; Zhao et al. Citation2011). For example, the CO1 gene was first evaluated but the results indicated that there are more introns (1–7) with various lengths (134 bp–3.1 kb) in the fungi, and is unsuitable as a fungal barcode (Seifert et al. Citation2007; Vialle et al. Citation2009). Druzhinina et al. (Citation2005) analysed 979 ITS sequences of 88 species of Hypocrea (anamorph Trichoderma) and established a DNA barcode system for species identification (The TrichOKEY v 1.0), and successfully identified 51 strains isolated from soil into known species using this system. ITS, as a candidate DNA barcode, has been widely used in the species identification of fungi, such as in Cortinarius (Frøslev et al. Citation2007), Amanita (Zhang et al. Citation2010), Chrysomyxa and Melampsora (Vialle et al. Citation2009), Mucorales (Schwarz et al. Citation2006), Tricholoma scalpturatum complex (Jargeat et al. Citation2010), lichenised fungi (Kelly et al. Citation2011), aquatic hyphomycete species (Seena et al. Citation2010), and ectomycorrhizal fungi in natural forest (Kõljalg et al. Citation2005; Tedersoo et al. Citation2008; Wang and Guo Citation2010; Hui et al. Citation2011; Wang et al. Citation2011a, Citationb).
The use of ITS as a DNA barcode has many indisputable advantages, such as high successful amplification among all lineages of fungi using universal primers, suitable fragment length, and a large number of available databases (Vilgalys Citation2003; Nilsson et al. Citation2009; Seifert Citation2009). However, the ITS barcode has several disadvantages. There are various inter- and intra-specific distances among the different fungal groups (Carbone and Kohn Citation1993; Morales et al. Citation1993; Zhao et al. Citation2011) and it is difficult to unify the threshold of ITS divergence for fungal species distinction at present (Guo et al. Citation2000, 2001; Sun et al. Citation2011). However, Arnold et al. (Citation2007) showed that ITS genotype groups based on 90% sequence similarity were concordant with 28S rDNA-delimited species of 72 Ascomycota and Basidiomycota, 145 cultured endophytic fungi, and 33 environmental PCR samples. A number of previous studies have demonstrated that ITS is insufficient for some species delimitation, especially in rapidly evolving or highly diverse genera or species complexes (Lacap et al. Citation2003; Gazis et al. Citation2011). There are limited numbers of reference sequences, i.e. less than 1% of the estimated 1.5 million fungal species presented in NBCI GenBank, EMBL and BOLD database, although there is a daily increase in fungal DNA sequences in public databases (Vilgalys Citation2003). In addition, misidentifications of named published sequences, where ∼20% of the named sequences may be attributed to incorrectly named organisms, may represent another problem restricting the feasibility of sequence-based identification of fungi (Vilgalys Citation2003; Hawksworth Citation2004; Jumpponen et al. Citation2010).
Recently, a workshop on the best gene for fungi DNA barcoding was held on 19–20 April 2011 in Amsterdam (involving 55 mycologists from 18 countries, most members of the Fungal Working Group of the Consortium for the Barcode of Life, CBOL). Based on preliminary analyses of the six preselected gene sequence data representing all major lineages in the Eumycota (http://www.fungalbarcoding.org), ITS, with a 72% identification success rate for all fungi, was demonstrated to be the best candidate as a DNA barcode marker for fungi. The meeting s concluded with a structured publication plan to CBOL for the formal proposal of ITS as the primary DNA barcode for fungi. Recently, ITS has been formally recommended as the primary DNA barcode of fungi at the Fourth International Barcode of Life conference (Adelaide, Australia, 28 Nov–3 Dec, 2011).
In endophyte studies, ITS is the most widely used DNA barcode in molecular identification and shows potential in diversity and ecology studies, despite some limitations in species distinction (Guo et al. Citation2003; Murali et al. Citation2007; Promputtha et al. Citation2007; U'ren et al. Citation2009; Sun et al. Citation2011).
6. Conclusions and future studies
Endophytic fungi comprise a diverse group of species existing in various ecosystems. Here, we summarise the study of endophyte diversity and community composition using different techniques but the vast majority of endophytes have yet to be adequately characterised. The revealed diversity and community composition of endophytic fungi are conspicuously different using cultivation-dependent and molecular techniques. Hyde and Soytong (Citation2008) suggested that researchers should realise that the techniques can severely influence observed endophyte diversity and should always highlight the methodological problems in endophyte studies. To obtain a completely qualitative picture of endophyte community composition, both traditional cultivation and molecular techniques should be employed. High-throughput sequencing, in particular, which has potential advantages compared to traditional Sanger sequencing techniques in terms of cost, time, throughput of samples, recovery of more species and providing a less biased qualitative picture of fungal community composition in natural environments, should be more widely employed in future endophyte studies.
DNA barcoding will doubtlessly be a central and most valuable element in fungal species identification in the future, although contemporary major sequence repositories are not optimally suited for such an operation due to the taxonomic reliability and insufficient annotations in public DNA databases. Therefore, taxonomic experts in all groups of fungi should make a greater effort to sequence well-identified and well-annotated (type) specimens from herbaria worldwide to form a sequence database with correct names. Significantly, ITS was formally accepted as the primary DNA barcode of fungi at the Fourth International Barcode of Life conference, which will greatly promote future applications of DNA barcoding in endophytic fungi.
In previous studies, conventional PCR-based molecular techniques have been employed in the detection and identification of endophytic fungi. Conventional PCR can identify endophytic fungi specifically, but it cannot be used to quantify endophyte biomass within plant tissues. However, real-time PCR can detect small quantities of DNA in environmental samples and has been successfully used to determine the population density of some fungal species, such as entomopathogenic fungi in insect (Bell et al. Citation2009), Armillaria biomass in planta (Baumgartner et al. Citation2010), H. minnesotensis in soil (Xiang et al. Citation2010), and anaerobic fungal biomass in the rumen (Khin et al. Citation2011). Maciá-Vicente (Citation2009) investigated the endophytic development of the fungal species Fusarium equiseti and Pochonia chlamydosporia in barley (Hordeum vulgare) roots with real-time PCR. Thus, real-time PCR techniques should be used to quantitatively detect the biomass and population size of endophytic fungi within plant tissues.
Endophytic fungi commonly exist within plant tissues and may play a key role in promoting host growth and resistance to environmental stress. However, the mechanism of endophytism is still not well understood. The key biological processes between plants and microorganisms have been investigated by high-throughput functional metagenomics or metatranscriptome strategies (Charles Citation2010; Reinhold-Hurek and Hurek Citation2011). Therefore, high-throughput sequencing could be a tool to explore the interaction between plants and endophytes through cultivation-independent study of the microbial community, thus providing further opportunities to reveal unknown functions and features of endophytes.
As potentially important microbial resources, endophytic fungi have been screened for new compounds with antimicrobial, antioxidant and antitumor activities (Liu et al. Citation2008, Citation2009, Citation2010, Citation2011; Aly et al. Citation2010; Li et al. Citation2011; Tejesvi et al. Citation2011). However, most current studies rely on high-throughput screening of endophyte cultures. A metagenomic library has been successfully constructed for natural product screening in soil microbes (Rondon et al. Citation2000; Clardy et al. Citation2006). These techniques, therefore, might be instructive in screening for novel bioactive compounds from endophytes within plant tissues, thus circumventing the cultivation obstacles in endophyte study.
Endophytic fungi are ubiquitous and occur within all known plants in various ecosystems, but the geographic differences in endophyte diversity, community composition and host/tissue preference have not been well documented. To understand the ecology of fungal endophytes, data regarding fundamental parameters of endophyte symbiosis are require from regional to continental scales and encompassing entire ecosystems (Peay et al. Citation2010). It is hoped that powerful, high-throughput sequencing technology will make the global assessment of endophyte diversity a reality and open up the ‘black box’ of fungal ecology.
Acknowledgments
This study is supported by the National Natural Science Foundation of China Grants (No. 30930005, 31070434 and 30870087) and the Chinese Academy of Sciences Grant (No. KSCX2-YW-Z-0935 and KSCX2-EW-Z-6).
References
- Albrectsen , BR , Bjorken , L , Varad , A , Hagner , A , Wedin , M , Karlsson , J and Jansson , S. 2010 . Endophytic fungi in European aspen (Populus tremula) leaves: diversity, detection, and a suggested correlation with herbivory resistance . Fungal Divers. , 41 : 17 – 28 .
- Aly , AH , Debbab , A , Kjer , J and Proksch , P. 2010 . Fungal endophytes from higher plants: a prolific source of phytochemicals and other bioactive natural products . Fungal Divers. , 41 : 1 – 16 .
- Anderson , IC and Cairney , JWG. 2004 . Diversity and ecology of soil fungal communities: increased understanding through the application of molecular techniques . Environ Microbiol. , 6 : 769 – 779 .
- Arnold , AE , Henk , DA , Eells , RL , Lutzoni , F and Vilgalys , R. 2007 . Diversity and phylogenetic affinities of foliar fungal endophytes in loblolly pine inferred by culturing and environmental PCR . Mycologia , 99 : 185 – 206 .
- Azevedo , JL and Araújo , WL. 2007 . “ Diversity and applications of endophytic fungi isolated from tropical plants ” . In Fungi: multifaceted microbes , Edited by: Ganguli , BN and Deshmukh , SK . 189 – 207 . Boca Raton , FL : CRC Press .
- Baumgartner , K , Bhat , R and Fujiyoshi , P. 2010 . A rapid infection assay for Armillaria and real-time PCR quantitation of the fungal biomass in planta . Fungal Biol. , 114 : 107 – 119 .
- Bayman , P , Lebrón , LL , Tremblay , RL and Lodge , DJ. 1997 . Variation in endophytic fungi from roots and leaves of Lepanthes (Orchidaceae) . New Phytol. , 135 : 143 – 149 .
- Begerow , D , Nilsson , H , Unterseher , M and Maier , W. 2010 . Current state and perspectives of fungal DNA barcoding and rapid identification procedures . Appl Microbiol Biotechnol. , 87 : 99 – 108 .
- Bell , AS , Blanford , S , Jenkins , N , Thomas , MB and Read , AF. 2009 . Real-time quantitative PCR for analysis of candidate fungal biopesticides against malaria: Technique validation and first applications . J Invertebr Pathol. , 100 : 160 – 168 .
- Bellemain , E , Carlsen , T , Brochmann , C , Coissac , E , Taberlet , P and Kauserud , H. 2010 . ITS as an environmental DNA barcode for fungi: an in silico approach reveals potential PCR biases . BMC Microbiol. , 10 : 189 – 197 .
- Bills , GF. 1996 . “ Isolation and analysis of endophytic fungal communities from wood plants ” . In Endophytic fungi in grasses and woody plants: systematics, ecology, and evolution , Edited by: Redlin , SC and Carris , LM . 31 – 65 . St. Paul , MN : APS Press .
- Botella , L and Diez , JJ. 2011 . Phylogenic diversity of fungal endophytes in Spanish stands of Pinus halepensis . Fungal Divers. , 47 : 9 – 18 .
- Burgess , T , Wingfield , MJ and Wingfield , BW. 2001 . Simple sequence repeat markers distinguish among morphotypes of Sphaeropsis sapinea . Appl Environ Microbiol. , 67 : 354 – 362 .
- Camacho , FJ , Gernandt , DS , Liston , A , Stone , JK and Klein , AS. 1997 . Endophytic fungal DNA, the source of contamination in spruce needle DNA . Mol Ecol. , 6 : 983 – 987 .
- Cannon , PF and Simmons , CM. 2002 . Diversity and host preference of leaf endophytic fungi in the Iwokrama Forest Reserve, Guyana . Mycologia , 94 : 210 – 220 .
- Carbone , I and Kohn , LM. 1993 . Ribosomal DNA sequence divergence within internal transcribed spacer 1 of the Sclerotiniaceae . Mycologia , 85 : 415 – 427 .
- Carroll , GC. 1986 . “ The biology of endophytism in plants with particular reference to woody plants ” . In Microbiology of the phyllosphere , Edited by: Fokkema , NJ and van den Heuvel , J . 205 – 222 . Cambridge , UK : Cambridge University Press .
- Charles , TC. 2010 . “ The potential for investigation of plant-microbe interactions using metagenomics methods ” . In Metagenomics: Theory, methods and applications , Edited by: Marco , D . 107 – 118 . Norfolk , UK : Marco D Caister/Academic Press .
- Cheplick , GP , Clay , K and Marks , S. 1989 . Interactions between infection by endophytic fungi and nutrient limitation in the grasses Lolium perenne and Festuca arundinacea . New Phytol. , 111 : 89 – 97 .
- Clardy , J , Fischbach , MA and Walsh , CT. 2006 . New antibiotics from bacterial natural products . Nat Biotechnol. , 24 : 1541 – 1550 .
- Crozier , J , Thomas , SE , Aime , MC , Evans , HC and Holmes , KA. 2006 . Molecular characterization of fungal endophytic morphospecies isolated from stems and pods of Theobroma cacao . Plant Pathol. , 55 : 783 – 791 .
- Davey , ML and Currah , RS. 2006 . Interactions between mosses (Bryophyta) and fungi . Can J Bot. , 84 : 1509 – 1519 .
- de Bary , A. 1866 . Morphologie und Physiologie der Pilze, Flechten, und Myxomyceten , Leipzig : W. Engelmann .
- Deckert , RJ , Melville , LH and Peterson , RL. 2001 . Structural features of a Lophodermium endophyte during the cryptic life-cycle phase in the foliage of Pinus strobus . Mycol Res. , 105 : 991 – 997 .
- Ding , G , Zheng , Z , Liu , S , Zhang , H , Guo , LD and Che , YS. 2009 . Photinides A-F, cytotoxic benzofuranone-derived γ-lactones from the plant endophytic fungus Pestalotiopsis photiniae . J Nat Prod. , 72 : 942 – 945 .
- Druzhinina , IS , Kopchinskiy , AG , Komon , M , Bissett , J , Szakacs , G and Kubicek , CP. 2005 . An oligonucleotide barcode for species identification in Trichoderma and Hypocrea . Fungal Genet Biol. , 42 : 813 – 828 .
- Dumbrell , AJ , Ashton , PD , Aziz , N , Feng , G , Nelson , M , Dytham , C , Fitter , AH and Helgason , T. 2011 . Distinct seasonal assemblages of arbuscular mycorrhizal fungi revealed by massively parallel pyrosequencing . New Phytol. , 190 : 794 – 804 .
- Duong , LM , Jeewon , R , Lumyong , S and Hyde , KD. 2006 . DGGE coupled with ribosomal DNA gene phylogenies reveal uncharacterized fungal phylotypes . Fungal Divers. , 23 : 121 – 138 .
- Fisher , PJ , Petrini , O and Sutton , BC. 1993 . A comparative study of fungal endophytes in leaves, xylem and bark of Eucalyptus nitens in Australia and England . Sydowia , 45 : 338 – 345 .
- Frøslev , TG , Jeppesen , TS , Laessoe , T and Kjoller , R. 2007 . Molecular phylogenetics and delimitation of species in Cortinarius section Calochroi (Basidiomycota, Agaricales) in Europe . Mol Phylogenet Evol. , 44 : 217 – 227 .
- Gamboa , MA , Laureano , S and Bayman , P. 2002 . Measuring diversity of endophytic fungi in leaf fragments: Does size matter? . Mycopathologia , 156 : 41 – 45 .
- Gao , XX , Zhou , H , Xu , DY , Yu , CH , Chen , YQ and Qu , LH. 2005 . High diversity of endophytic fungi from the pharmaceutical plant, Heterosmilax japonica Kunth revealed by cultivation-independent approach . FEMS Microbiol Lett. , 249 : 255 – 266 .
- Gazis , R , Rehner , S and Chaverri , P. 2011 . Species delimitation in fungal endophyte diversity studies and its implications in ecological and biogeographic inferences . Mol Ecol. , 20 : 3001 – 3013 .
- Gharizadeh , B , Norberg , E , Löffler , J , Jalal , S , Tollemar , J , Einsele , H , Klingspor , L and Nyrén , P. 2004 . Identification of medically important fungi by the Pyrosequencing (TM) technology . Mycoses , 47 : 29 – 33 .
- Ghimire , SR , Charlton , ND , Bell , JD , Krishnamurthy , YL and Craven , KD. 2011 . Biodiversity of fungal endophyte communities inhabiting switchgrass (Panicum virgatum L.) growing in the native tallgrass prairie of northern Oklahoma . Fungal Divers. , 47 : 19 – 27 .
- Gillevet , PM , Sikaroodi , M and Torzilli , AP. 2009 . Analyzing salt-marsh fungal diversity: comparing ARISA fingerprinting with clone sequencing and pyrosequencing . Fungal Ecol. , 2 : 160 – 167 .
- González , V and Tello , ML. 2011 . The endophytic mycota associated with Vitis vinifera in central Spain . Fungal Divers. , 47 : 29 – 42 .
- Götz , M , Nirenberg , H , Krause , S , Wolters , H , Draeger , S , Buchner , A , Lottmann , J , Berg , G and Smalla , K. 2006 . Fungal endophytes in potato roots studied by traditional isolation and cultivation-independent DNA-based methods . FEMS Microbiol Ecol. , 58 : 404 – 413 .
- Groppe , K and Boller , T. 1997 . PCR assay based on a microsatellite-containing locus for detection and quantification of Epichloë endophytes in grass tissue . Appl Environ Microbiol. , 63 : 1543 – 1550 .
- Guo , LD. 2001 . Advances of endophytic fungi . Mycosystema , 20 : 148 – 152 . in Chinese with English abstract
- Guo , LD , Hyde , KD and Liew , ECY. 1998 . A method to promote sporulation in palm endophytic fungi . Fungal Divers. , 1 : 109 – 113 .
- Guo , LD , Hyde , KD and Liew , ECY. 2000 . Identification of endophytic fungi from Livistona chinensis (Palmae) using morphological and molecular techniques . New Phytol. , 147 : 617 – 630 .
- Guo , LD , Hyde , KD and Liew , ECY. 2001 . Detection and identification of endophytic fungi within frond tissues of Livistona chinensis based on rDNA sequence . Mol Phylogenet Evol. , 20 : 1 – 13 .
- Guo , LD , Huang , GR , Wang , Y , He , WH , Zheng , WH and Hyde , KD. 2003 . Molecular identification of white morphotype strains of endophytic fungi from Pinus tabulaeformis . Mycol Res. , 107 : 680 – 688 .
- Guo , LD , Huang , GR and Wang , Y. 2008 . Seasonal and tissue age influences on endophytic fungi of Pinus tabulaeformis (Pinaceae) in Dongling Mountain, Beijing . J Integr Plant Biol. , 50 : 997 – 1003 .
- Hajibabaei , M , Janzen , DH , Burns , JM , Hallwachs , W and Hebert , PDN. 2006 . DNA barcodes distinguish species of tropical Lepidoptera . Proc Natl Acad Sci USA , 103 : 968 – 971 .
- Hallmann , J , Berg , G and Schulz , B. 2006 . “ Isolation procedures for endophytic microorganisms ” . In Microbial root endophytes , Edited by: Schulz , BJE , Boyle , CJC and Sieber , TN . 299 – 319 . Berlin : Springer .
- Hawksworth , DL. 1991 . The fungal dimension of biodiversity: magnitude, significance, and conservation . Mycol Res. , 95 : 641 – 655 .
- Hawksworth , DL. 2004 . ‘Misidentifications’ in fungal DNA sequence databanks . New Phytol. , 161 : 13 – 15 .
- Hebert , PDN , Cywinska , A , Ball , SL and de Waard , JR. 2003 . Biological identifications through DNA barcodes . Proc R Soc (Lond) B , 270 : 313 – 321 .
- Higgins , KL , Arnold , AE , Miadlikowska , J , Sarvate , SD and Lutzoni , F. 2007 . Phylogenetic relationships, host affinity, and geographic structure of boreal and arctic endophytes from three major plant lineages . Mol Phylogenet Evol. , 42 : 543 – 555 .
- Hoff , JA , Klopfenstein , NB , McDonald , GI , Tonn , JR , Kim , MS , Zambino , PJ , Hessburg , PF , Rogers , JD , Peever , TL and Carris , LM. 2004 . Fungal endophytes in woody roots of Douglas-fir (Pseudotsuga menziesii) and ponderosa pine (Pinus ponderosa) . For Pathol. , 34 : 255 – 271 .
- Hui , N , Jumpponen , A , Niskanen , T , Liimatainen , K , Jones , KL , Koivula , T , Romantschuk , M and Strömmer , R. 2011 . EcM fungal community structure, but not diversity, altered in a Pb contaminated shooting range in a boreal coniferous forest site in Southern Finland . FEMS Microbiol Ecol. , 76 : 121 – 132 .
- Hyde , KD and Soytong , K. 2008 . The fungal endophyte dilemma . Fungal Divers. , 33 : 163 – 173 .
- Jargeat , P , Martos , F , Carriconde , F , Gryta , H , Moreau , PA and Gardes , M. 2010 . Phylogenetic species delimitation in ectomycorrhizal fungi and implications for barcoding: the case of the Tricholoma scalpturatum complex (Basidiomycota) . Mol Ecol. , 19 : 5216 – 5230 .
- Jumpponen , A and Jones , KL. 2009 . Massively parallel 454 sequencing indicates hyperdiverse fungal communities in temperate Quercus macrocarpa phyllosphere . New Phytol. , 18 : 438 – 444 .
- Jumpponen , A , Jones , KL and Blair , J. 2010 . Vertical distribution of fungal communities in tallgrass prairie soil . Mycologia , 102 : 1027 – 1041 .
- Kelly , LJ , Hollingsworth , PM , Coppins , BJ , Ellis , CJ , Harrold , P , Tosh , J and Yahr , R. 2011 . DNA barcoding of lichenized fungi demonstrates high identification success in a floristic context . New Phytol. , 191 : 288 – 300 .
- Khin , L , Mika , H , Tomomi , B-T and Hiroki , M. 2011 . Real-time PCR assays for monitoring anaerobic fungal biomass and population size in the rumen . Curr Microbiol. , 62 : 1147 – 1151 .
- Kõljalg , U , Larsson , KH , Abarenkov , K , Nilsson , RH , Alexander , IJ , Eberhardt , U , Erland , S , Høiland , K , Kjøller , R Larsson , E . 2005 . UNITE: a database providing web-based methods for the molecular identification of ectomycorrhizal fungi . New Phytol. , 166 : 1063 – 1068 .
- Krings , M , Taylor , TN , Hass , H , Kerp , H , Dotzler , N and Hermsen , EJ. 2007 . Fungal endophytes in a 400-million-yr-old land plant: infection pathways, spatial distribution, and host responses . New Phytol. , 174 : 648 – 657 .
- Kumaresan , V and Suryanarayanan , TS. 2002 . Endophytic assemblages in young, mature and senescent leaves of Rhizophora apiculata: evidence for the role of endophytes in mangrove litter degradation . Fungal Divers. , 9 : 91 – 91 .
- Lacap , DC , Hyde , KD and Liew , ECY. 2003 . An evaluation of the fungal ‘morphotype’ concept based on ribosomal DNA sequences . Fungal Divers. , 12 : 53 – 66 .
- Letourneau , A , Seena , S , Marvanová , L and Bärlocher , F. 2010 . Potential use of barcoding to identify aquatic hyphomycetes . Fungal Divers. , 40 : 51 – 64 .
- Levinson , G and Gutman , GA. 1987 . Slipped-strand mispairing: a major mechanism for DNA sequence evolution . Mol Biol Evol. , 4 : 203 – 221 .
- Li , J , Li , L , Si , YK , Jiang , XJ , Guo , LD and Che , YS. 2011 . Virgatolides A–C, benzannulated spiroketals from the plant endophytic fungus Pestalotiopsis virgatula . Org Lett. , 13 : 2670 – 2673 .
- Li , WC , Guo , SY and Guo , LD. 2007a . Endophytic fungi associated with lichen Physcia stellaris using different surface sterilization methods . J Fungal Res. , 5 : 202 – 206 .
- Li , WC , Zhou , J , Guo , SY and Guo , LD. 2007b . Endophytic fungi associated with lichens in Baihua mountain of Beijing, China . Fungal Divers. , 25 : 69 – 80 .
- Liang , Y , Guo , LD and Ma , KP. 2005 . Population genetic structure of an ectomycorrhizal fungus Amanita manginiana in a subtropical forest over two years . Mycorrhiza , 15 : 137 – 142 .
- Liu , L , Liu , SC , Jiang , LH , Chen , XL , Guo , LD and Che , YS. 2008 . Chloropupukeananin, the first chlorinated pupukeanane derivative and its precursors from Pestalotiopsis fici . Org Lett. , 10 : 1397 – 1400 .
- Liu , L , Li , Y , Liu , SC , Zheng , ZH , Chen , XL , Zhang , H , Guo , LD and Che , YS. 2009 . Chloropestolide A, an antitumor metabolite with an unprecedented spiroketal skeleton from Pestalotiopsis fici . Org Lett. , 11 : 2836 – 2839 .
- Liu , L , Niu , SB , Lu , XH , Chen , XL , Zhang , H , Guo , LD and Che , YS. 2010 . Unique metabolites of Pestalotiopsis fici suggest a biosynthetic hypothesis involving a Diels-Alder reaction and then mechanistic diversification . Chem Commun. , 46 : 460 – 462 .
- Liu , L , Bruhn , T , Guo , LD , Götz , DCG , Brun , BR , Stich , A , Che , YS and Bringmann , G. 2011 . Chloropupukeanolides C–E, cytotoxic pupukeanane chlorides with a spiroketal skeleton from Pestalotiopsis fici . Chem Eur J. , 17 : 2604 – 2613 .
- Lucero , ME , Unc , A , Cooke , P , Dowd , S and Sun , SL. 2011 . Endophyte microbiome diversity in micropropagated Atriplex canescens and Atriplex torreyi var. griffithsii . PLoS ONE. , 6 : e17693
- Lumini , E , Orgiazzi , A , Borriello , R , Bonfante , P and Bianciotto , V. 2010 . Disclosing arbuscular mycorrhizal fungal biodiversity in soil through a land-use gradient using a pyrosequencing approach . Environ Microbiol. , 12 : 2165 – 2179 .
- Maciá-Vicente , JG , Jansson , H-B , Talbot , NJ and Lopez-Llorca , LV. 2009 . Real-time PCR quantification and live-cell imaging of endophytic colonization of barley. Hordeum vulgare. roots by Fusarium equiseti and Pochonia chlamydosporia . New Phytol. , 182 : 213 – 228 .
- Margulies , M , Egholm , M , Altman , WE , Attiya , S , Bader , JS , Bemben , LA , Berka , J , Braverman , MS , Chen , YJ Chen , ZT . 2005 . Genome sequencing in microfabricated high-density picolitre reactors . Nature , 437 : 376 – 380 .
- May , LA , Smiley , B and Schmidt , MG. 2001 . Comparative denaturing gradient gel electrophoresis analysis of fungal communities associated with whole plant corn silage . Can J Microbiol. , 47 : 829 – 841 .
- Medinger , R , Nolte , V , Pandey , RM , Jost , S , Ottenwälder , B , Schlötterer , C and Boenigk , J. 2010 . Diversity in a hidden world: potential and limitation of next-generation sequencing for surveys of molecular diversity of eukaryotic microorganisms . Mol Ecol. , 19 : 32 – 40 .
- Mohamed , R , Jong , PL and Zali , MS. 2010 . Fungal diversity in wounded stems of Aquilaria malaccensis . Fungal Divers. , 43 : 67 – 74 .
- Monchy , S , Sanciu , G , Jobard , M , Rasconi , S , Gerphagnon , M , Chabé , M , Cian , A , Meloni , D , Niquil , N Christaki , U . 2011 . Exploring and quantifying fungal diversity in freshwater lake ecosystems using rDNA cloning/sequencing and SSU tag pyrosequencing . Environ Microbiol. , 13 : 1433 – 1453 .
- Morakotkarn , D , Kawasaki , H and Seki , T. 2007 . Molecular diversity of bamboo-associated fungi isolated from Japan . FEMS Microbiol Lett. , 266 : 10 – 19 .
- Morales , VM , Pelcher , LE and Taylor , JL. 1993 . Comparison of the 5.8S rDNA and internal transcribed spacer sequences of isolates of Leptosphaeria maculans from different pathogenicity groups . Curr Genet. , 23 : 490 – 495 .
- Müller , CB and Krauss , J. 2005 . Symbiosis between grasses and asexual fungal endophytes . Curr Opin Plant Biol. , 8 : 450 – 456 .
- Murali , TS , Suryanarayanan , TS and Venkatesan , G. 2007 . Fungal endophyte communities in two tropical forests of southern India: diversity and host affiliation . Mycol Progress , 6 : 191 – 199 .
- Nilsson , RH , Ryberg , M , Abarenkov , K , Sjökvist , E and Kristiansson , E. 2009 . The ITS region as a target for characterization of fungal communities using emerging sequencing technologies . FEMS Microbiol Lett. , 296 : 97 – 101 .
- Nilsson , RH , Tedersoo , L , Lindah , B D , Kjøller , R , Carlsen , T , Quince , C , Abarenkov , K , Pennanen , T , Stenlid , J Bruns , T . 2011 . Towards standardization of the description and publication of next-generation sequencing datasets of fungal communities . New Phytol. , 191 : 314 – 318 .
- Öpik , M , Metsis , M , Daniell , TJ , Zobel , M and Moora , M. 2009 . Large-scale parallel 454 sequencing reveals host ecological group specificity of arbuscular mycorrhizal fungi in a boreonemoral forest . New Phytol. , 184 : 424 – 437 .
- Ovaskainen , O , Nokso-Koivista , J , Hottola , J , Rajala , T , Pennanen , T , Ali-Kovero , H , Miettinen , O , Oinonen , P , Auvinen , P Paulin , L . 2010 . Identifying wood-inhabiting fungi with 454 sequencing - what is the probability that BLAST gives the correct species? . Fungal Ecol. , 3 : 274 – 283 .
- Peay , KG , Martin , I. , Bidartondo , MI and Arnold , AE. 2010 . Not every fungus is everywhere: scaling to the biogeography of fungal-plant interactions across roots, shoots and ecosystems . New Phytol. , 185 : 878 – 882 .
- Peever , TL , Ibañez , A , Akimitsu , K and Timmer , LW. 2002 . Worldwide phylogeography of the citrus brown spot pathogen, Alternaria alternata . Phytopathology , 92 : 794 – 802 .
- Petrini , O. 1991 . “ Fungal endophytes of tree leaves ” . In Microbial ecology of leaves , Edited by: Andrews , JH and Hirano , SS . 179 – 197 . New York : Springer .
- Petrini , O , Stone , J and Carroll , FE. 1982 . Endophytic fungi in evergreen shrubs in western Oregon: A preliminary study . Can J Bot. , 60 : 789 – 796 .
- Petrini , O , Sieber , TN , Toti , L and Viret , O. 1992 . Ecology, metabolite production, and substrate utilization in endophytic fungi . Nat Toxins , 1 : 185 – 196 .
- Photita , W , Lumyong , S , Lumyong , P and Hyde , KD. 2001 . Endophytic fungi of wild banana (Musa acuminata) at doi Suthep Pui National Park, Thailand . Mycol Res. , 105 : 1508 – 1513 .
- Promputtha , I , Jeewon , R , Lumyong , S , McKenzie , EHC and Hyde , KD. 2005 . Ribosomal DNA fingerprinting in the identification of non sporulating endophytes from Magnolia liliifera (Magnoliaceae) . Fungal Divers. , 20 : 167 – 186 .
- Promputtha , I , Lumyong , S , Dhanasekaran , V , McKenzie , EHC , Hyde , KD and Jeewon , R. 2007 . A phylogenetic evaluation of whether endophytes become saprotrophs at host senescence . Microb Ecol. , 53 : 579 – 590 .
- Purahong , W and Hyde , KD. 2011 . Effects of fungal endophytes on grass and non-grass litter decomposition rates . Fungal Divers. , 47 : 1 – 7 .
- Reinhold-Hurek , B and Hurek , T. 2011 . Living inside plants: bacterial endophytes . Curr Opin Plant Biol. , doi: 10.1016/j.pbi.2011.04.004
- Rodrigues , KF and Samuels , GJ. 1990 . Preliminary study of endophytic fungi in a tropical palm . Mycol Res. , 94 : 827 – 830 .
- Rondon , MR , August , PR , Bettermann , AD , Brady , SF , Grossman , TH , Liles , MR , Loiacono , KA , Lynch , BA , MacNeil , IA Minor , C . 2000 . Cloning the soil metagenome: a strategy for accessing the genetic and functional diversity of uncultured microorganisms . Appl Environ Microbiol. , 66 : 2541 – 2547 .
- Saikkonen , K , Saari , S and Helander , M. 2010 . Defensive mutualism between plants and endophytic fungi? . Fungal Divers. , 41 : 101 – 113 .
- Schwarz , P , Bretagne , S , Gantier , JC , Garcia-Hermoso , D , Lortholary , O , Dromer , F and Dannaoui , E. 2006 . Molecular identification of Zygomycetes from culture and experimentally infected tissues . J Clin Microbiol. , 44 : 340 – 349 .
- Seena , S , Pascoal , C , Marvanová , L and Cássio , F. 2010 . DNA barcoding of fungi: a case study using ITS sequences for identifying aquatic hyphomycete species . Fungal Divers. , 44 : 77 – 87 .
- Seifert , KA. 2009 . Progress towards DNA barcoding of fungi . Mol Ecol Res , 9 ( Suppl.1 ) : 83 – 89 .
- Seifert , KA , Samson , RA , de Waard , JR , Houbraken , J , Lévesque , CA , Moncalvo , JM , Louis-Seize , G and Hebert , PDN. 2007 . Prospects for fungus identification using CO1 DNA barcodes, with Penicillium as a test case . Proc Natl Acad Sci USA , 104 : 3901 – 3906 .
- Sinclair , JB and Cerkauskas , RF. 1996 . “ Latent infection vs. endophytic colonization by fungi ” . In Endophytic fungi in grasses and woody plants: systematics, ecology, and evolution , Edited by: Redlin , SC and Carris , LM . 3 – 29 . St. Paul , MN : APS Press .
- Sogin , ML , Morrison , HG , Huber , JA , Welch , DM , Huse , SM , Neal , PR , Arrieta , JM and Herndl , GJ. 2006 . Microbial diversity in the deep sea and the underexplored “rare biosphere” . Proc Natl Acad Sci USA , 103 : 12115 – 12120 .
- Stockinger , H , Krüger , M and Schüßler , A. 2010 . DNA barcoding of arbuscular mycorrhizal fungi . New Phytol , 187 : 461 – 474 .
- Stoyke , G and Currah , RS. 1991 . Endophytic fungi from the mycorrhizae of alpine ericoid plants . Can J Bot , 69 : 347 – 352 .
- Su , YY , Guo , LD and Hyde , KD. 2010 . Response of endophytic fungi of Stipa grandis to experimental plant function group removal in Inner Mongolia steppe, China . Fungal Divers , 43 : 93 – 101 .
- Sun , JQ , Guo , LD , Zang , W , Ping , WX and Chi , DF. 2008 . Diversity and ecological distribution of endophytic fungi associated with medicinal plants . Sci China Ser C. , 51 : 751 – 759 .
- Sun , X , Guo , LD and Hyde , KD. 2011 . Community composition of endophytic fungi in Acer truncatum and their role in decomposition . Fungal Divers , 47 : 85 – 95 .
- Suryanarayanan , TS , Thirunavukkarasu , N , Hariharan , GN and Balaji , P. 2005 . Occurrence of non-obligate microfungi inside lichen thalli . Sydowia , 57 : 119 – 129 .
- Swatzell , LJ , Powell , MJ and Kiss , JZ. 1996 . The relationship of endophytic fungi to the gametophyte of the fern Schizaea pusilla . Int J Plant Sci , 157 : 53 – 62 .
- Taylor , DL and McCormick , MK. 2008 . Internal transcribed spacer primers and sequences for improved characterization of basidiomycetous orchid mycorrhizas . New Phytol , 177 : 1020 – 1033 .
- Taylor , JE , Hyde , KD and Jones , EBG. 1999 . Endophytic fungi associated with the temperate palm, Trachycarpus fortunei, within and outside its natural geographic range . New Phytol , 142 : 335 – 346 .
- Tedersoo , L , Jairus , T , Horton , BM , Abarenkov , K , Suvi , T , Saar , I and Kõljalg , U. 2008 . Strong host preference of ectomycorrhizal fungi in a Tasmanian wet sclerophyll forest as revealed by DNA barcoding and taxon-specific primers . New Phytol , 180 : 479 – 490 .
- Tedersoo , L , Nilsson , RH , Abarenkov , K , Jairus , T , Sadam , A , Saar , I , Bahram , M , Bechem , E , Chuyong , G and Kõljalg , U. 2010 . 454 Pyrosequencing and Sanger sequencing of tropical mycorrhizal fungi provide similar results but reveal substantial methodological biases . New Phytol , 188 : 291 – 301 .
- Tejesvi , MV , Kajula , M , Mattila , S and Pirttilä , AM. 2011 . Bioactivity and genetic diversity of endophytic fungi in Rhododendron tomentosum Harmaja . Fungal Divers , 47 : 97 – 107 .
- Ting , ASY , Meon , S , Kadir , J , Radu , S and Singh , G. 2008 . Endophytic microorganisms as potential growth promoters of banana . Biocontrol , 53 : 541 – 553 .
- U'Ren , JM , Dalling , JW , Gallery , RE , Maddison , DR , Davis , EC , Gibson , CM and Arnold , AE. 2009 . Diversity and evolutionary origins of fungi associated with seeds of a neotropical pioneer tree: a case study for analysing fungal environmental samples . Mycol Res , 113 : 432 – 449 .
- Vialle , A , Feau , N , Allaire , M , Didukh , M , Martin , F , Moncalvo , JM and Hamelin , RC. 2009 . Evaluation of mitochondrial genes as DNA barcode for Basidiomycota . Mol Ecol Resour , 9 : 99 – 113 .
- Vieira , PDS , de Souza Motta , CM , Lima , D , Torres , JB , Quecine , MC , Azevedo , JL and de Oliveira , NT. 2011 . Endophytic fungi associated with transgenic and non-transgenic cotton . Mycology , 2 : 91 – 97 .
- Vilgalys , R. 2003 . Taxonomic misidentification in public DNA database . New Phytol , 160 : 4 – 5 .
- Wang , Q and Guo , LD . 2010 . Ectomycorrhizal community composition of Pinus tabulaeformis assessed by ITS-RFLP and ITS sequences . Botany , 88 : 590 – 595 .
- Wang , Q , Gao , C and Guo , LD. 2011a . Ectomycorrhizae associated with Castanopsis fargesii (Fagaceae) in a subtropical forest, China . Mycol Progress , 10 : 323 – 332 .
- Wang , Q , He , XH and Guo , LD. 2011b . Ectomycorrhizal fungus communities of Quercus liaotugensis Koidz of different ages in a northern China temperate forest . Mycorrhiza , doi: 10.1007/s00572-011-0423-x
- Wang , Y and Guo , LD. 2007 . A comparative study of endophytic fungi in needles, bark, and xylem of Pinus tabulaeformis . Can J Bot , 85 : 911 – 917 .
- Wang , Y , Guo , LD and Hyde , KD. 2005 . Taxonomic placement of sterile morphotypes of endophytic fungi from Pinus tabulaeformis (Pinaceae) in northeast China based on rDNA sequences . Fungal Divers , 20 : 235 – 260 .
- Wang , YC , Zheng , ZH , Liu , SC , Zhang , H , Li , EW , Guo , LD and Che , YS. 2010 . Oxepinochromenones, furochromenone, and their putative precursors from the endolichenic fungus Coniochaeta sp . J Nat Prod , 73 : 920 – 924 .
- Xiang , MC , Xiang , PA , Jiang , XZ , Duan , WJ and Liu , XZ. 2010 . Detection and quantification of the nematophagous fungus Hirsutella minnesotensis in soil with real-time PCR . Appl Soil Ecol , 44 : 170 – 175 .
- Xu , J , Ebada , SS and Proksch , P. 2010 . Pestalotiopsis a highly creative genus: chemistry and bioactivity of secondary metabolites . Fungal Divers , 44 : 15 – 31 .
- Zhang , P , Chen , ZH , Xiao , B , Tolger , B , Bao , HY and Yang , ZL. 2010 . Lethal amanitas of East Asia characterized by morphological and molecular data . Fungal Divers , 42 : 119 – 133 .
- Zhao , P , Luo , J and Zhuang , WY. 2011 . Practice towards DNA barcoding of the nectriaceous fungi . Fungal Divers , 46 : 183 – 191 .
- Zheng , RY and Jiang , H. 1995 . Rhizomucor endophyticus sp. nov., an endophytic zygomycetes from higher plants . Mycotaxon , 56 : 455 – 466 .