ABSTRACT
Fungi, being natural decomposers, are the most potent, ubiquitous and versatile sources of industrial enzymes. About 60% of market share of industrial enzymes is sourced from filamentous fungi and yeasts. Mycozymes (myco-fungus; zymes-enzymes) are playing a pivotal role in several industrial applications and a number of potential applications are in the offing. The field of mycozyme production, while maintaining the old traditional methods, has also witnessed a sea change due to advents in recombinant DNA technology, optimisation protocols, fermentation technology and systems biology. Consolidated bioprocessing of abundant lignocellulosic biomass and complex polysaccharides is being explored at an unprecedented pace and a number of mycozymes of diverse fungal origins are being explored using suitable platforms. The present review attempts to revisit the current status of various mycozymes, screening and production strategies and applications thereof.
Introduction
Modern biologists classify fungi as lower eukaryotes as they show absorptive mode of nutrition. Fungi being obligate heterotrophs secrete an array of extracellular mycozymes to hydrolyse complex polymeric substrates around. Many times, these mycozymes are resilient enough to survive harsh conditions including acidic pH, low water activity level and high temperature. Solid state fermentation (SSF) of complex substrates, particularly agro-industrial wastes such as sugarcane bagasse, palm kernel cake, copra meal, wheat bran, etc. is naturally suitable for the fact that molds thrive well in xerophilic conditions.
The first use of the mycozymes goes back to the beginning of the 19th century. The first mycozyme, diastase, was known in the year 1833 (Payen and Persoz Citation1833; Asimov Citation1982) and the term enzyme was first used in 1877, which meant “in leaven” in Greek (Kuhne Citation1877). The first reference to the successful application of mycozymes, however, was taka-diastase from Aspergillus oryzae in the year 1894 to produce koji cultivated on rice by Jokichi Takamine. In 1897, yeast extract, free of yeast cells was used for sucrose hydrolysis. J.M. Nelson and E.G. Griffin, in 1916, demonstrated the immobilisation of invertase from yeast on charcoal and alumina and showed that enzymes can be reused (Bennett and Frieden Citation1969). Since then, a number of enzymes of fungal origin have been discovered and are exploited commercially in various industries. Most enzymes used in industries belong to the hydrolase class, i.e. enzymes that degrade various complex polymeric natural substances e.g. cellulose, starch, xylan and protein. The advancement in the fermentation processes lead to the large-scale manufacturing of mycozymes. Post 1980, mycozyme production was further improved by the application of genetic engineering, heterologous expression and efforts were made to alter characteristics of the fungal enzymes by protein engineering to acquire desirable properties suitable for industrial applications.
Selection of commercially important strain for mycozyme production is a very significant step in ascertaining economic feasibility of the enzyme production and application. This further helps in selecting the substrate, reaction conditions and recovery methods, which are economically favourable. The global enzyme market size was estimated at USD 9.9 billion in 2019 and is anticipated to grow at a compound annual growth rate (CAGR) of 7.1% from 2020 to 2027 and among all, more than 50% enzymes will be of fungal origin (https://www.grandviewresearch.com/industry-analysis/enzymes-industry). Increasing demand from user industries such as food, beverage, biofuel, animal feed, and home cleaning, is expected to drive the market growth over the forecast period. Increasing health awareness among consumers has resulted in the growing consumption of functional food products, which is expected to propel the product demand soon. Role of mycozymes in generation of health promoting prebiotic oligosaccharides and nutraceuticals has been the recent spurt of interest (Jana et al. Citation2018; Choukade and Kango Citation2019; Khangwal et al. Citation2020).
Apart from the conventional approaches, mycozymes are being explored using modern tools such as gene cloning and editing, directed evolution, protein engineering, molecular dynamic simulation etc. As a result, interesting chimeric enzymes, overexpression systems and robust enzymes are being produced and their functional patterns are being unravelled. Recently, Gilmore et al. (Citation2020) reported preservation of the protein domain order from Piromyces finnis wherein, the chimeric enzymes retained catalytic activity at temperatures over 80°C and were able to associate with cellulosomes purified from this anaerobic fungus.
In this review, we explore the current status of mycozymes, and the mechanism involved in their extracellular secretion, their UpToDate relevance in different industries and the advents made in their production and applications. The recent advancements in genetic engineering of these industrial enzymes and its impact on the enzyme due to gene cloning and expression in different hosts are also being explored in this review.
1. Regulatory aspects of mycozyme secretion
Filamentous fungi are a well-known source of industrial enzymes because of their remarkable capability for their extracellular production (Jun et al. Citation2011). Extracellular enzymes are synthesised inside the fungal cell and are then secreted in the extracellular environment, where their function is to catalyse transformation of complex substrates into smaller molecules which would be later taken up by fungus for its growth and development. Their potential to secrete hydrolases for digesting most complex macromolecules has been exploited for various applications at large-scale (). Baker (Citation2018) hypothesised three different biological processes that contribute to secretion of inducible mycozymes by filamentous fungi, (1) nutrient sensing, (2) transcriptional regulation and (3) translation and secretion which is illustrated in . Alazi and Ram (Citation2018) suggested that due to variation in the composition of plant biomass, the mycozyme cocktail may also vary, therefore, it becomes even more important in industrial interest to produce desired mycozyme blends constitutively, independent of the substrate or the inducer used. Fungus must elaborate the significant amount of mycozymes to digest complex substrates and generate building block nutrients for absorption; the expense of secreting an enzyme must be balanced with the nutritional repay of the biosynthetic expenditure and growth rate. Fungi are capable of recognising the ligands available for nutrition. This is attained through the G-protein-coupled receptors (GPCRs), which are located at the plasma membrane comprising seven transmembrane domains and are majorly involved in environmental sensing and cell-signalling. GPCRs are turned on, when nutritional ligands bind to GPCR and trigger the GDP-GTP exchange on the Gα protein coupled with dissociation of Gα and Gβγ followed initiation of downstream signal cascades by Gα (Gao et al. Citation2021), adenylate cyclase shoots up levels of cyclic AMP (cAMP), which in turn trigger protein kinase A (PKA) downstream signalling cascades resulting in carbon catabolite repression. The apparatus of transcription regulation that exquisitely regulates the carbon catabolite repression system and secretion of lignocellulosic deconstruction enzymes of fungi can differ; some of these regulatory genes encoding extracellular mycozymes are described by Aro et al. (Citation2005). Regulatory elements present in the promoter region have binding sites for the carbon catabolite repressor (CRE) gene, which encodes for plant cell wall degrading mycozymes. The inclusion of a deletion of the catabolite repressor gene, cre-1, in the triple β-glucosidase mutant of Neurospora crassa resulted in a strain that produced higher concentrations of extracellular active cellulases on cellobiose. Thus, the ability to induce cellulase gene expression using a common and soluble carbon source simplifies enzyme production and characterisation, which could be applied to other cellulolytic filamentous fungi (Znameroski et al. Citation2012). Following three measures have been suggested by Baker (Citation2018) for overproduction of mycozymes: (1) evading nutritional repressor cues, (2) stopping transcriptional repression and (3) enhancing protein translation and secretion.
Table 1. Mycozymes used in various industries and their applications
Figure 1. Regulatory aspects of mycozyme expression: Schematic illustration of G-protein signalling (1) Nutritional ligands bind to G protein-coupled receptor (GPCR) and (2) trigger the GDP-GTP exchange on the Gα protein coupled with dissociation of Gα and Gβγ (3) Gα triggers downstream signal cascades, which include the Cyclic AMP (cAMP) activated Protein Kinase A (PKA) pathway (4) Enzyme synthesis, post-translational folding and glycosylation and (5) extracellular secretion (Peberdy Citation1994; Baker Citation2018).
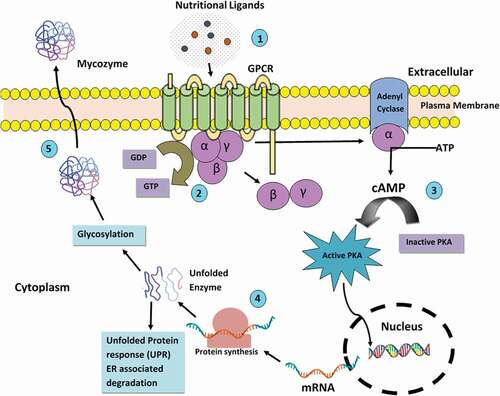
Fungal protein secretion has been delved at all stages, from protein targeting to endoplasmic reticulum (ER) to secretion and subsequent degradation by proteases with the goal of improving titre, rate and yield of target proteins. Generally, proteins secreted by fungi are glycosylated and many of them are associated with the cell envelope, the plasma membrane, and the cell wall. Deshpande et al. (Citation2008) studied the N-glycosylation pathway in the cytoplasm and ER and found that it was highly conserved evolutionarily across all the filamentous fungi considered. Finally, enzymes are secreted out from the surface of the plasma membrane into the periplasmic space, where they may be subsumed into the cell wall or, in many instances, may be discharged across the cell wall into the external medium. Before proteins are secreted, they undertake sundry of post-translational modifications. These start in the ER and perpetuate as the proteins travel through the Golgi apparatus. Presumably, three changes may happen to a protein molecule: (1) proteolytic cleavage of zymogenic form to remove the signal sequence from a propeptide sequence, if present; (2) a folding process involving the formation of disulphide bridges to develop the tertiary and quaternary structures of the protein; and (3) glycosylation (Peberdy Citation1994). After proper folding and glycosylation, enzymes are secreted extracellularly. On the other hand, the unfolded protein response (UPR) and ER-associated protein degradation (ERAD) are in-charge for managing the peptides with incorrect folding (Bernasconi and Molinari Citation2011; Wang et al. Citation2014). The UPR checks the presence of unfolded proteins in ER and initiates the biosynthesis of chaperones and folding enzymes, whereas the ERAD lyses the misfolded proteins. Lastly, the passage of the proper folded protein vesicles to the Golgi apparatus by fusion with target membrane and its secretion to the extracellular environment occurs (Wang et al. Citation2020b).
2. Screening strategies for mycozymes
The booming demand for environmentally benign industrial processes relies on the ability of finding a biocatalyst suitable to ideal process conditions. Detection of enzyme activity depends on a biochemical estimation that accounts for either the product formation or substrate depletion (Sheludko and Fessner Citation2020). Identification of native fungal isolates producing glycosyl hydrolases is extremely important, because of the increased demand for these mycozymes in many industries. Some of the classical methods of screening employ the polysaccharide (cellulose, xylan, mannan etc.) in solid medium and after the development of the fungal colony, Congo red or suitable polymer binding dye, is used to create a visible halo of the zone of hydrolysis. Prajapati et al. (Citation2018) screened cellulase production in Aspergillus tubingensis NKBP-55 by growing it on Czapek-Dox medium supplemented with (1%) carboxy-methyl cellulose as the sole C-source and observed clear zones of hydrolysis after staining with 1% (w/v) Congo red. Similarly, xylanase production in Aspergillus and Trichoderma and mannanase production in Malbranchea cinnamomea, Melanocarpus albomyces, Aspergillus terreus, Myceliophthora thermophila was detected using xylan and mannan supplemented medium followed by staining with Congo red and de-staining with 1% NaCl solution (Ramanjaneyulu et al. Citation2015; Ahirwar et al. Citation2017). Dye–polysaccharide inter-actions which provide a visual indication of polymer hydrolysis (clear zones or halos) have been used for decades. Screening of various mycozymes based on agar-plate assays has been listed in .
Table 2. Agar-plate assays for screening of various mycozymes
Addition of colour changing indicator dyes can enhance the visibility during screening process. Kruthi and Devarai (Citation2016) used modified Czapek–Dox (MCD) agar plates with L-asparagine (10 g/L) as a sole nitrogen source and used indicator dye, phenol red (0.009%) or bromothymol blue (0.007%), to observe colour change from yellow to pink or red and yellow to blue, respectively. Hydrolysis of synthetic substrates in the culture media, which is executed by the cleavage of the moiety attached to the substrate thus, producing a visible effect has also been used in detection of mycozymes. Panesar et al. (Citation2016) screened β-galactosidase from fungi like Aureobasidium pullulans NCIM 1050, Aspergillus oryzae NCIM 1212, A. niger NCIM 616, and A. flavus MTCC 9349 using 50 μL of X-gal (5-bromo-4-chloro-3-indole-β-D galactopyranoside) in the solid medium with 20 mg/mL DMSO as inducer and observed the presence of β-galactosidase in fungal colonies appearing blue in colour. Use of modified synthetic substrates is a specific, precise and rapid method to detect desired mycozyme from among the consortia secreted by the fungus. In this, the substrate is modified by addition of synthetic signalogenic moiety and on enzymatic reaction, the signalophore conjugate is released from the synthetic substrate delivering a measurable signal. Allison et al. (Citation2018) used fluorogenic soluble synthetic substrates like 7-amino-4-methylcoumarin (AMC) for detecting leucine aminopeptidase (LAP) and 4-methyumbelliferone (MUB) for ascertaining the presence of hydrolytic enzymes in the culture filtrate of Neurospora discrete. The fluorescence for hydrolytic enzymes was read at 365 nm/450 nm excitation/emission and for oxidative enzymes, absorbance was read at 410 nm. In recent years, there has been tremendous interest in the development of enzyme assays in connection with the high-throughput screening of enzymes for use in biocatalysis and drug discovery.
3. Production strategies of mycozymes
Fungi have the natural capability of colonising complex organics at low water activity level (aw). Occurrence of fungi in litter, wood logs, tree barks clearly suggests that these are adapted to grow on solid surfaces. In recent times, solid-state fermentation (SSF) has become an alternative industrial production system to produce enzymes and some of these important industrial mycozymes are listed in . SSF is a type of industrial fermentation with any microbial cultures’ growth on the surface of substrate or interior of the solid matrix in the absence of free-flowing water. Besides the koji-type systems, SSF cultures are now routinely used by researchers on other solid natural substrates, generally, agro-waste residues composed of lignocellulosic materials, like soybean hulls, wheat bran, rice straw, orange peels, red gram husk, soybean husk, rice stalk, sugarcane bagasse etc. (Dong-sheng et al. Citation2017; Lópeza et al. Citation2018; Mandari et al. Citation2019; Bruno et al. Citation2019; Melnichuk et al. Citation2020), but sometimes industrial waste of dairy, brewery, paper, pulp, and wood processing industries is also used as substrate for mycozyme production. For example, chicken feather meal for keratinase production by Trichoderma harzianum (Bagewadi et al. Citation2018) and maize distillery dried grain solubles (DDGS) for phytase production from Trichoderma atroviride (Pradoa et al. Citation2019). Thus, SSF is a technique to utilise organic wastes as raw materials to produce mycozymes, which could further be used for various industrial processes and at the same time contributing to solve the environmental issues caused by their inadequate disposal.
Table 3. Production of mycozymes in solid state fermentation (SSF)
Another type of SSF uses an inert support with absorbed liquid medium. The support can be of natural origin like sugarcane bagasse, or synthetics like polyurethane, amberlite or vermiculite etc. De la Cruz et al. (2015) carried out SSF using high-density polyurethane foam (PUF) impregnated with culture medium and spore solution to reach 70% moisture content. Fermentation was carried out at temperature 30°C with aeration rate 0.5 vKgm (air volume/kg material) for 36 h to produce 606.67 U/L of ellagitannase from A. niger. In this type of SSF, the product recovery from inert support is less cumbersome because of convenient extraction procedures and products are obtained with fewer impurities (Singhania et al. Citation2008), however, the costs of the inert support are higher than in the previous case.
Wang et al. (Citation2018) utilised textile wastes as substrate for cellulase production via submerged fungal fermentation and Trichoderma reesei ATCC 24449 was selected for highest cellulase activity (18.75 FPU/g). Abd El-Rahim et al. (2020) produced pectinase from fungi isolated from flax retting liquor using pectin mineral salt broth, at 30°C with shaking at 120 rpm for 120 h. Among various fungi, Aspergillus pulverulentus F23 produced 25.78 pectinase Unit/gram fungal biomass. Lately, issues with submerged fermentations (SmF), such as high-energy consumption, additional requirement of water and effluent generation are limiting its applications. Academic as well as industrial researchers are again taking notice of the advantages of SSF such as less water and energy consumption, low costs and high productivity. Ali et al. (2019) used 10 g of milled pistachio shell (particles size < 500 μM) and 2 mL liquid medium (yeast extract 0.2 g/L, glucose 2 g/L and CuSO4 0.625 g/L) to produce 172.0 U/mg of laccase from Lentinus tigrinus. Thus, mycozyme production using SSF is crucial in chemical, pharmaceutical and environmental industries, and also makes an important contribution in waste management and sustainable development (Chen Citation2013).
4. Some industrially relevant mycozymes
4.1 Proteases
Proteases (EC 3.4) catalyse hydrolytic reactions that degrade protein molecules down to peptides and eventually to free amino acids. They constitute another large and complex group of enzymes, which differ from each other in terms of substrate specificity, nature of active site and catalytic mechanism followed, as well as pH and temperature optima and heat stability. The specificity of proteases, in particular, is governed by the type of amino acid residue(s) in their catalytic site (Ramos and Malcata 2017). The physical and chemical parameters of protease from fungi have been widely studied and described. Due to its varied nature, proteases are used in food and feed, waste management, detergent and medical sectors.
Prominent fungal producers belong to the genera Aspergillus, Penicillium, Rhizopus, MucorandThermomyces. Peptidases of Kluyveromyces lactis, Saccharomyces cerevisiae, Debaryomyces hansenii and Pichia anomala accelerated cheese ripening (Klein et al. Citation2002). An aminopeptidase of Thermomyces lanuginosus expressed in A. niger strain HL-1 showed significant increase in the degree of hydrolysis of soy protein and removed more hydrophobic amino acids from the N-terminal region of the polypeptide to decrease the bitterness (Lin et al. Citation2019). Darío et al. (2018) reported production of 1000 U/mL aminopeptidase from A. niger using orange peels and soybean hulls as substrate for SSF. Boyce and Walsh (Citation2012) used protease of Schizophyllum commune for environment friendly clean-in-place of protein waste in dairy industry. Recently, Murthy et al. (Citation2020) reported cysteine proteases of A. oryzae for amelioration of cocoa organoleptics in biscuits, cookies and crackers. Kumara et al. (Citation2019) produced prolyl-endoprotease from A. niger and used it for cleaving proline and glutamine moieties present in gluten and making low immunogenic pasta for gluten sensitive population. Meat tenderisation, production of fish protein hydrolysate, viscosity reduction, skin removal and roe processing were carried out using aspartic proteases of Rhizomucor miehei (Sun et al. Citation2017). An acidic protease of Metschnikowia reukaufii SAP6 was expressed in E. coli and the purified enzyme had milk-clotting activity (Jing et al. Citation2010). Yang and Zhang (Citation2019) expressed transglutaminase in Pichia pastoris GS115 and used it to restructure pork and crosslinking of soy protein isolate with chicken myofibrillar protein which increased hardness and chewiness. Aspergillus flavipes produced 20.4 U/mL protease on wheat bran in SSF (Pradoa et al. Citation2019). A salt-tolerant glutaminase of A. oryzae expressed in E. coli was found suitable for brewing high quality soy sauce with a high L-glutamic acid concentration (Masuoa et al. Citation2004). Serine alkaline protease of Penicillium chrysogenum X5 was used for protein stain removal in textile industry (Omrane Benmrad et al. Citation2018). Alkaline protease of Neocosmospora sp. N1 was used for blood stain removal as it yields cleaner, whiter, smoother skin as compared to sulphide treatment (Matkawala et al. Citation2019). De Medeiros et al. (Citation2016) reported use of keratinase of Trichosporon loubieri RC-S6 in dehairing for leather production. The heterologous expression of SUB3 keratinase of Microsporum canis expressed in P. pastoris KM71 H provided a valuable tool for addressing investigations on the role of this keratinase in the specific cellular immune response and on its use in vaccination trials in cats (Descamps et al. Citation2003). L-asparaginase of A. terreus acted as an efficient anti-cancer drug against lung cancer cell lines A549 (Baskar et al. Citation2018). Saeed et al. (Citation2018) expressed L-asparaginase of A. terreus in E. coli for treatment of acute lymphoblastic leukaemia. Katrolia et al. (Citation2019) expressed protease of Cordyceps militaris CmFE in P. pastoris GS115 and used recombinant fibrinolytic enzyme as therapeutic agent for thrombolysis.
4.2 Cellulases
Cellulose is the most abundant renewable carbohydrate on the earth and the major constituent of plant cell wall. Cellulose is naturally embedded with lignin – hemicellulose matrix within the plant cell wall. It is being explored widely for the generation of fermentable sugar for bio-ethanol generation. Biofuel generation from cellulosic biomass utilises three steps viz. pre-treatment, enzymatic saccharification and ethanolic fermentation. After pre-treatment, generation of monosugars from the complex lignocelluloses is catalysed by cellulases and hemicellulases. Fermentation of released sugars is carried out by yeast for bio-ethanol production. Cellulose is a homopolymer composed of glucose units linked by β- 1,4 -glycosidic bonds. α-1,6-glycosidic bonding between glucose moieties and hydrogen bonding among cellulose fibrils gives rise to a compact crystalline structure which is difficult to digest by a single hydrolase. Cellulases represent a complex group of synergistically acting enzymes. They principally contain endo-1,4-glucanase (EC 3.2.1.4), which cleave randomly at internal amorphous cellulose sites causing rapid reduction in the cellulose DP while liberating cello-oligomers in the process; cellobiohydrolases (EC 3.2.1.91) act progressively on crystalline cellulose and primarily attack the reducing ends of polymer to produce cellobiose or short chain oligosaccharides and later, β-glucosidase (E.C. 3.2.1.21) hydrolyses cellobiose to glucose monomers (Wahlström and Suurnäkki Citation2015). Cellulases have remarkable applications in various industries including pulp and paper, textile, laundry, biofuels and food and feed and brewing industry (Prajapati et al. Citation2018).
Among fungi, major industrially used production hosts for cellulase production include Trichoderma, Penicillium and Aspergillus. Recently, Darwesh et al. (Citation2020) reported cellulase of A. niger MK 543209 for fibre modification (improving softness), deinking and bioethanol production. Wang et al. (Citation2018) used cellulase of T. reesei ATCC 24449 for valorisation of textile waste in textile and laundry industries. Cellulases of Trichoderma viride were used for pitch control during pulping process in paper and pulp industries (Kumar et al. Citation2018). Recently, Tao et al. (Citation2019) reported simultaneous production of xylooligosaccharides (XOS) and cello-oligosaccharides (COS) by recombinant endoglucanase I of T. reesei expressed in P. pastoris, showing a novel application of genetically modified mycozyme for maximum utilisation of natural biomass and fortification of food and feed with functional food ingredients.
4.3 Hemicellulases
Hemicelluloses, comprising the second most abundant part of the plant biomass, are diverse group of structural and storage polysaccharides. Xylan, mannan and other hemicelluloses make up to 30% of the dry weight of the wood. Their enzymatic hydrolysis using fungal hemicellulases has led to several industrial applications, viz., biobleaching; wastepaper deinking; fruit juice maceration; upgradation of feed, fodder, and fibres; and saccharification of biomass.
4.3.1 Xylanases
Xylan is a structural polysaccharide of plant cell wall, with a high potential for conversion into useful end products using xylanases. It is a heteroglycan composed of a linear chain of xylopyranose residues bound by β (1 → 4) linkages, with a variety of substituents linked to the main chain by glycosidic or ester linkages. Endo-1,4-β-xylanases (EC 3.2.1.8) are most important for xylan hydrolysis as they initiate the degradation of xylan into XOS and xylose (Ramanjaneyulu et al. Citation2015). Due to the structural heterogeneity of xylan, complete hydrolysis of xylan requires combined action of endo-1, 4-β-xylanase, β-1,4-D-xylan-xylanohydrolase, β-xylosidase and some accessory enzymes (Kango et al. Citation2005).
Many microbes such as bacteria, fungi and actinobacteria are known to produce xylanolytic enzymes, however, filamentous fungi are the preferred and most explored xylanase producers among all (Basu et al. Citation2018; Kumar et al. Citation2018; Aaa et al. Citation2018). Among these, Thermomyces, TrichodermaandAspergillus are the most exploited genera for xylanase production. Thermomyces lanuginosus (previously known as Humicola lanuginosa) has gained considerable interest due to its ability to produce high titres of thermostable endo-xylanase (Khangwal et al. Citation2020). Apart from being used in conjunction with cellulases for biofuel production, xylanases have numerous applications in various industries such as food and animal feed, paper and pulp processing, textiles etc. (Basu et al. Citation2018). Xylanase sourced from Trichoderma stromaticum improved the dough quality by reducing water content during pasta preparation (Almeida Carvalho et al. Citation2016). Similarly, Kumar et al. (Citation2018) reported xylanase of Fusarium equiseti MF-3 showing potential in pulp and paper industry for pitch control in pulping process. Xylanases of T. lanuginosus and T. reesei have been used for enzymatic hydrolysis of xylan rich agro-waste for production of 2 G-bioethanol (Juodeikiene et al. Citation2011). Xylanases of Talaromyces thermophilus showed enhanced pulp bleaching process efficiency and released chromophores and reduced sugars (Maalej-Achouri et al. Citation2012). Recently, Bhardwaj et al. (Citation2020) expressed xylanase gene, XynF1 of A. oryzae in E. coli. Cellulase-free xylanases are desirable for biobleaching where they replace chlorine based bleaching agents and thus, release of toxic organo-chloro compounds is avoided. Recently, Martínez-Pachecoa et al. (Citation2019) optimised the xylanase production with low cellulase titres in Fusarium solani by SSF. Nowadays, nutritional quality of food and feed is being enhanced by augmenting them with functional food ingredients like XOS. Hydrolysis of xylan rich agro-waste products, (e.g. corn cobs) using fungal xylanase generates XOS and also leads to value addition and proper disposal of agricultural waste. Recently, Zheng et al. (Citation2020a) provided a cost-effective method of producing XOS from corn cobs by expressing xylanase gene Taxy11 of Trichoderma asperellum ND-1 in P. pastoris.
4.3.2 Mannanases
Mannans, chiefly composed of mannose, are the plant storage and structural polysaccharides commonly known as gums. These being heteropolymeric require synergistic action of different enzymes for complete degradation including, β-mannanase (EC 3.2.1.78), β-mannosidase (EC 3.2.1.25), β-glucosidase (EC 3.2.1.21), α-galactosidase (EC 3.2.1.22) and acetyl esterase (EC 3.1.1.6). Several mannanolytic mycozymes are reported from the genera Aspergillus (Soni et al. Citation2016), Penicillium (Blibech et al. Citation2011) and Trichoderma (Elena et al. Citation2009). Aspergillus aculeatus endo-β-mannanase (Man1) and Talaromyces emersonii α-galactosidase (Agal) genes were co-expressed in S. cerevisiae Y294 and the maximum enzyme activity for Man1 (374 nkat/mL, pH 5.47) and Agal (135 nkat/mL, pH 2.37) were reported (Malherbe et al. Citation2014). β-Mannanases have extensive applications in industries such as food and feed processing. The N-glycosylation in the loop area intern improved thermal stability, pH stability and protease-resistance of the Armillariella tabescens β-mannanase (Hu et al. Citation2017). Rhizopus microsporus endo-β-mannanase was structurally characterised and it showed altered binding properties with different oligosaccharides (You et al. Citation2018). Mannanases from Malbranchea cinnamomea, A. oryzae and A. terreus that generate mannooligosaccharides (MOS) from locust bean gum, guar gum and konjac gum have been reported (Ahirwar et al. Citation2017; Li et al. Citation2017; Jana and Kango Citation2020).
4.3.3 Pectinases
Pectin is another structural polysaccharide that occurs in the primary cell wall and intracellular layer of fruits, such as apples, oranges, lemons etc. and is characterised by the presence of galacturonic acid residues (Mudgil Citation2017). Pectinases (EC 3.2.1.15) constitute a heterogeneous group of enzymes that break down complex polysaccharides of plant tissues into simpler molecules like galacturonic acids either by depolymerisation (hydrolases and lyases) or de-esterification (esterases) (Singh et al. Citation2019). Pectinases are produced predominantly by Aspergillus and Penicillium and are used to accelerate rates of clarification and filtration to remove pectin from fruit base prior to gel formation during jam manufacture. A. niger grown on fresh orange pomace in SSF produced exo-pectinases (55 U/g) and endo-pectinases (10 U/g) (Mahmoodi et al. Citation2019). Pectinase of A. tamarii was used for bioscouring and phytopigment processing in textile industry (Shanmugavel et al. Citation2018). Recently, Ahmed et al. (Citation2018) produced pectinase from Geotrichum candidum AA15 and used it for the degradation of pectin in fruits to decrease viscosity and clarify juice. Pectinase of Mucor sp. was used to breakdown pectin of brewer’s spent grain to accelerate pre-fermentation stage and enhance clarification during beer production (Hassan et al. Citation2019). The recombinant NfPG4 and NfPG5 genes of Neosartorya fischeri expressed in P. pastoris GS115 were shown to be exo- and endo-polygalacturonases, respectively. Both pectinases were tolerant towards a wide range of pH, temperature, metal ions, making them a suitable choice for industrial applications (Li et al. Citation2015).
4.3.4. Inulinase and Fructosyltransferase (FTase)
Inulins are made up of fructose units linked typically with a terminal glucose by glycosidic linkage. Inulin belongs to the fructan group of storage and transport polysaccharides and in many plant species it is synthesised from sucrose by adding a fructosyl unit (Choukade and Kango Citation2020). Commercially exploited prebiotic fructooligosaccarides, kestose (GF2), nystose (GF3), and β-fructofuranosylnystose (GF4) are produced from sucrose by fructosyltransferase (FTase) from plants, bacteria and fungi.
Inulinases (E.C. 3.2.1.7) are the key enzymes that take part in inulin metabolism in plants and microorganisms. They hydrolyse natural plant fructan inulin into fructose and inulooligosaccharides (IOS) upon acting on glycosidic linkages with terminal glucose (Kango and Jain Citation2011). Rawat et al. (Citation2015) reported inulinase and FTase activity in some Aspergilli and Penicillia. Fructosyltransferase (EC 2.4.1.9) is known to hydrolyse sucrose and transfer fructosyl group to an acceptor molecule to generate fructooligosaccharides (FOS) along with glucose and fructose (Ganaie et al. Citation2014). FTase possesses transfructosylating activity, cleaves the β-1,2 linkage of sucrose and transfers fructosyl group to an acceptor molecule leading to formation of FOS and release of glucose.
Industrial production of FOS involves the action of enzymes with transfructosylating activity isolated from microbial sources like fungi such as Aspergillus japonicus, A. niger, A. sydowii, A. foetidus, A. oryzae, A. pullulans, Penicillium citrinum, P. frequentans, and Fusarium oxysporum (Bali et al. Citation2015). Jiang et al. (Citation2016) isolated a novel yeast, Aureobasidium sp. P6, from a mangrove ecosystem producing 30.98 U/mL inulinase. The inulinase also had transfructosylating activity at higher concentration of sucrose (30%) leading to FOS production. Wang et al. (Citation2016a) used an industrial strain, A. niger ATCC 20611, to enhance the production of FOS, wherein they have used polyethylene glycol (PEG)-mediated protoplast transformation system for strain improvement. The transformed A. niger ATCC 20611, exhibited a 58% increase in specific β-fructofuranosidase activity (up to 507 U/g), compared to the parental strain (320 U/g). Previously, Tanriseven and Aslan (Citation2005) have also immobilised commercially available A. aculeatus FTase (Pectinex Ultra SP-L) in Eupergit C with efficacy of 96% and maintained the recycling up to 20 days to obtain GF4, GF3 and GF2 in FOS mixture. Immobilised enzyme also showed a higher temperature optimum at 65°C. Wang et al. (Citation2016d) cloned an endo-inulinase in S. cerevisiae and deleted its sucrase gene which resulted into high content FOS production (~90%) from inulin in a single step. Recently, Bao et al. (Citation2019) expressed Lipomyces starkeyi NRRL Y-11557 inulinase gene (INU3B) in E. coli for the production of nutraceutical FOS.
Production of an extracellular, thermostable inulinase was carried out by a newly isolated strain of A. tubingensis CR16 using wheat bran and corn steep liquor (CSL) in SSF. After parametric optimisation, the fungus produced 1358.6 U/g inulinase, showing 5-fold enhancement (Trivedi et al. Citation2012). Similarly, Singh et al. (Citation2018) demonstrated the applicability of apple pomace as a potent substrate in SSF for inulinase production by Mucor circinelloides.
4.4 Amylases
Starch is the most abundant storage polysaccharide on the earth and major component of many staple crops such as, potato, wheat, corn and rice. Apart from being staple food such as bread or rice, it also finds use as a thickener and a gelling agent in food industry. Starch consists of linear insoluble amylose and branched soluble amylopectin. In amylose, glucose is linked by 1,4-glycosidic bonds in a linear fashion, while in amylopectin some of the chains are linked by α-1,6-linkages giving it a branched structure.
Amylases, including α-amylase and glucoamylase, are perhaps the most important enzymes in present day biotechnology due to their wide range of applications in numerous industrial processes, including food, fermentation, textiles, and paper industries (Parashar and Satyanarayana Citation2017). As mentioned earlier, α-amylase bears historical relevance from the point of view of industrial application of mycozymes. After the production of Taka-diastase in 1894 from A. oryzae, α-amylase was also used as a textile desizing agent in Japan in 1905. Later in 1959, Rhizopus sp. was used to produce glucoamylase. Amylolytic enzymes account for about 30% of total industrial enzymes (Vaidya et al. Citation2015).
4.4.1 α-Amylases
α-Amylases (EC 3.2.1.1) are extracellular endo-acting enzymes that randomly hydrolyse α-1,4 glycosidic bonds in starch to produce maltose and dextrins. Most industrial applications use α-amylases for saccharification or liquefaction purposes. Fungal sources of industrial α-amylases are mostly confined to Aspergillus, Penicillium and Rhizopus sp. (Li et al. Citation2011). Aspergillus being one of the prominent and notably the most explored genera for α-amylases, particularly, A. oryzae (Taka-diastase) and A. niger α-amylases have been used extensively in the starch industry. Aggarwal et al. (Citation2019) reported amylase of Aspergillus sp. for removal of starch coating (desizing) from textile. Recently, Wang et al. (Citation2020a) reported amylases from R. miehei CAU432 which can be used for increasing bread volume and softness, enhance colour and flavour and even in preventing staling of bread. Xu-Cong et al. (Citation2015) produced α-amylase from Monascus purpureus, R. oryzae, Pichia guilliermondii, Saccharomycopsis fibuligera and S. cerevisiae, which were used for hydrolysing starch during traditional brewing of Wuyi Hong Qu glutinous rice for wine production. Similarly, ethanol biosynthesis by fast hydrolysis of cassava bagasse by amylase sourced from Rhizopus oligosporus has been demonstrated by (Escarambonia et al. Citation2018). However, being mesophilic, the enzymes are not thermostable and thus bacterial α-amylases replace them in the very first step of gelatinisation (or cooking) at high temperature. Wang et al. (Citation2019a) expressed a thermostable α-amylase active at 80°C from Thermomyces dupontii (TdAmyA) in P. pastoris and have demonstrated its applicability in maltose syrup production.
4.4.2 Glucoamylases
Glucoamylase (EC 3.2.1.3) is an exo-acting enzyme that cleaves α-1,4 linkages from the non-reducing ends but can also cleave α-1,6 linkages at the branching point of amylopectin, thus leading to successive and complete degradation of starch into glucose. Most commercial glucoamylases are sourced from Aspergillus spp. (Carrasco et al. Citation2017). Recently, Fabiane et al. (2020) produced ethanol from rice by-products using amylases secreted by Rhizopus microsporus var. oligosporus. Melikoglu et al. (Citation2015) used waste bread pieces for solid-state production of glucoamylase from Aspergillus awamori. Glucoamylase of A. niger was used for developing starch-based nanocrystals as natural carriers for nutraceutical delivery (Hao et al. Citation2010). GA2 gene of Aspergillus flavus glucoamylase was expressed in P. pastoris GS115 and the mycozyme generated larger, deeper, holes on the starch granules of raw sago starch, indicating rGA2 is an excellent candidate for industrial starch processing applications (Karima et al. Citation2019).
4.5 Laccases
After cellulose and hemicelluloses, lignin is the most abundant component of plant residues, and is relatively recalcitrant. Also, the degradation rates of lignocellulosic materials are negatively correlated to their lignin content or to their lignin-to-N ratio. Lignin is an aromatic biomolecule that is degraded at a much slower rate than cellulosic and non-cellulosic polysaccharides and proteins. Laccases (EC 1.10.3.2) are multicopper biocatalysts that catalyse the oxidation of mainly phenolic compounds by one electron transfer with the concurrent reduction of oxygen to water. Laccase is prominently used in wastewater management, textile, pulp and paper industries. Among fungi, laccases are particularly abundant in the white-rot fungi, which are the only organisms which have ability to decompose the whole wood components (i.e. cellulose, hemicellulose and lignin) so far (Daljit and Rakesh Citation2009). The most studied fungus for laccase production is the white-rot fungus, Trametes versicolor (Rodrıguez-Couto Citation2018). Recently, laccase of T. versicolor was used for increasing the oxidative stability of edible vegetable oil (Guerberoff and Camusso Citation2019). Ortiz-Monsalve et al. (Citation2017) utilised lignolytic mycozymes of Trametes villosa SCS-10 for the removal of unwanted fats and dyes during soaking and liming process and treatment of wastewater. Recently, Navada and Kulal (Citation2019) reported laccase of Phomopsis sp. for bleaching, deinking and biotransformation of aniline blue in textile industry. Digestion of lignin waste using laccase of Ganoderma lucidum MDU-7 for production of bioethanol is relevant for management of waste from pulp and paper industry (Saini et al. Citation2020). Improved activity, stability at alkaline pH and its role in the improved dye decolourisation suggested the application potential of the recombinant laccase of Coprinopsis cinerea expressed in P. pastoris GS115 for wastewater treatment (Xu et al. Citation2018).
4.6 Chitinases
Chitin, found as ordered crystalline microfibrils in the structural component of crustaceans and insects, is the most abundant biopolymer in nature after cellulose (Verma and Fortunati Citation2019). Chitinases (EC 3.2.1.14) hydrolyse chitin, a β-(1→4) linked N-acetyl glucosamine structural polysaccharide (Pérez and Tvaroška Citation2014). Chitinases are produced by plants as part of their defence mechanism against the invading fungal pathogens; whereas, microbial chitinases, produced by bacteria (Streptomyces and Bacillus spp.) are secreted to assist in the breakdown and assimilation of fungal cell walls, whereas fungal chitinases assist fungal cell-wall morphogenesis; only in species of mycoparasitic fungi, such as Trichoderma harzianum, Aphanocladium album and Gliocladium virens to attack and degrade hyphae of other molds (Ramos and Malcata 2017). Metarhizium anisopliae produced 12.07 U/g chitinase on sugarcane bagasse in SSF (Bruno et al. Citation2019). Chitinases have wide-ranging applications including the preparation of pharmaceutically important chito-oligosaccharides and N-acetyl,D-glucosamine, preparation of single-cell protein, isolation of protoplasts from fungi, control of pathogenic fungi, treatment of chitinous waste, mosquito control and morphogenesis etc. (Hamid et al. Citation2013). Recently, Liu et al. (Citation2020b) expressed chitosanase of Beauveria bassiana in P. pastoris GS115 and demonstrated its use in the production of chitosan oligosaccharides. Chitinase gene of T. harzianum, Chit46 expressed in P. pastoris GS115, proved to be a good candidate for the green recycling of chitinous waste and inhibiting the growth of the phytopathogenic fungus Botrytis cinerea (Jun-Jin et al. Citation2019). Seven chitinases from different bacteria and fungi were produced, characterised and their biocontrol abilities against graminaceous stem borers Eldana saccharina, Chilo partellus and Sesamia calamistis were assessed; out of which chitinase from the thermophilic T. lanuginosus SSBP (Chit1) was found to be more acid-stable than the bacterial counterparts and caused 70% mortality in star larvae of E. saccharina (Okongo et al. Citation2019). A potent chitin-hydrolysing enzyme from Myrothecium verrucaria (rMvEChi) has been shown to affect the growth and development of Helicoverpa armigera and control plant fungal pathogens (Ustilago maydis and Bipolaris sorokiniana) (Vidhate et al. Citation2019).
4.7 Lipases
Lipases (EC 3.1.1.3) catalyse the hydrolysis of long-chain triglycerides into glycerol and fatty acids. Lipases sourced from bacteria and fungi are relatively stable and are capable of catalysing a variety of reactions and thus, are potentially important for diverse industrial applications (Hou and Shimada Citation2009). A. niger when grown on Prosopis juliflorapods, red gram husk and cotton seed cake mixture in the ratio of (6.66:1.66:1.66) produced 269 U/gds lipase (Mandari et al. Citation2019). Multifarious industrial applications of fungal lipases in the detergent, bioremediation, food, flavour industries, biocatalytic resolution of pharmaceuticals, esters and amino acid synthesis, making of fine agrochemicals, biosensor, cosmetics and perfumery make them a very important enzyme (Hasan et al. Citation2006). Lipase from Candida rugosa was used for the conversion of non-polyunsaturated fatty acids to polyunsaturated fatty acids by removing glycerol backbone of triglycerol in kilka fish oil (Hosseini et al. Citation2018). Sahay and Chouhan (Citation2018) demonstrated lipid stain removal by facilitating cold-washing as a step towards mitigation of climate change using cold-active lipases of Penicillium canescens BPF4 and Pseudogymnoascus roseus BPF6. Lipase of Rhizopus chinensis expressed in P. pastoris GS115 showed esterification of short-chain fatty acids with ethanol (Yu et al. Citation2009). Recently, Spiropulos Gonçalves et al. (Citation2020) reported lipases of B. bassiana expressed in Aspergillus nidulans A773 and applied it for production of biodiesel by transesterification of triglycerides.
5. Cloning and expression of mycozymes
Although mycozymes have diverse potential applications and prominent presence, there are some limitations associated with them. First, it is difficult to obtain in-depth understanding of the biocatalytic action of the mycozyme in a mixture. Second, an accountable production economy can be challenging to obtain, as it may be difficult to optimise the production of a specific mycozyme without knowing the target gene. Third, the mixture may consist of proteases which can hamper the activity of the specific mycozyme in need. The recombinant mycozymes can be produced in high yields thus providing new tools for functional studies through careful selection of the expression system in the substantially higher purity. Wang et al. (Citation2019a) expressed α-amylase gene of Thermomyces dupontii, TdAmyA in P. pastoris GS115 with AOX1 promoter and it produced the highest maltose content of 51.8% after 8 h hydrolysis indicating application in maltose syrup production. The expression system allowed high level α-galactosidase production in medium with glucose as the sole carbon source and without a requirement for an inducer with a yield of 2.45 U/mL, which is nearly 3-fold higher than the yield obtained from A. fumigatus grown in locust bean gum containing medium (Gürköka et al. 2009). During the last few years expression cloning has been applied to several fungal enzymes and has proven very efficient in cloning of mycozyme genes. Recently, Bhardwaj et al. (Citation2020) expressed xylanase of A. oryzae in E. coli BL21 which exhibited a wide range of activity at different pH (3.0–10.0) range and temperature (30–70°C) with an optimum pH and temperature as 5.0 and at 30°C, respectively, making it useful for a variety of industrial applications. The superior properties of mannanase of Aspergillus kawachii expressed in P. pastoris X33, strongly facilitates MOS preparation and application in food and feed area (Liu et al. Citation2019). Some of the industrially relevant mycozymes which are cloned and expressed in a heterologous hosts are listed in . Fatimi et al. (Citation2018) expressed cellobiohydrolase of Trichoderma virens in A. niger strain PY11 with GlaPr as the promoter gene which enhanced the CbhI activity towards Avicel (0.011 U/mg), which could be useful in complex biomass degradation. Penicillium griseoroseum PG63 efficiently expressed phytase of P. chrysogenum with an increase of up to 5.1 times in the enzymatic activity and stability profiles. The ability to release inorganic phosphate from cereals which are commonly used for pig feed suggested the potential application of phytase produced by P. griseoroseum T73 in the animal nutrition industry (Ribeiro Corrêa et al. Citation2014). Furthermore, the new enzyme backbones may contribute significantly to a better understanding and determination of amino acid residues that may be of importance for the enzymatic characteristics.
Table 4. Heterologous expression of Mycozymes in various hosts
6. Conclusion and future prospects
Fungi produce myriad biocatalysts which find diverse applications in a range of industrial processes. On account of their ability to utilise low-value substrates, amenability to manipulation, and ability to generate enzymes in copious titres, they are preferred choice for production of industrial biocatalysts. Among industrial enzymes, 60% are sourced from about 25 fungal genera. Pertaining to rising number of applications, rapid growth in demand of mycozymes is indicative of their suitability in biofuel, food, detergent, pharmaceutical and nutraceutical industries. To overcome the bottle-neck of cost-effectiveness, a multi-pronged approach is required. Firstly, a more comprehensive understanding of dynamics of fungal growth and enzyme biosynthesis should be developed followed by development of state-of-the-art modules for submerged and solid-state cultures. Further, development of strains expressing robust and multifunctional (chimeric) enzymes using recombinant DNA technology, high-throughput screening of novel isolates, metagenomic screening, in silico enzyme engineering, site-directed mutagenesis, and directed evolution will pave a way to cater future demands. Recent advents such as CRISPR/Cas9 genome editing, number of available fungal genome sequences and knowledge of omics hold promises for development of robust fungal strains in near future.
Disclosure statement
No potential conflict of interest was reported by the author(s).
References
- Aaa EA, Saleh SA, Eid BM, Ibrahim NA, Mostafa FA. 2018. Thermodynamics characterization and potential textile applications of Trichoderma longibrachiatum KT693225 xylanase. Biocatal Agric Biotechnol. 14:129–137. doi:https://doi.org/10.1016/j.bcab.2018.02.011.
- Abrera AT, Chang H, Kracher D, Ludwig R, Haltrich D. 2020. Characterization of pyranose oxidase variants for bioelectrocatalytic applications. BBA - Proteins and Proteomics. 1868:140–335
- Aggarwal R, Dutta T, Sheikh J. 2019. Extraction of amylase from the microorganism isolated from textile mill effluent vis a vis desizing of cotton. Sustain Chem Pharm. 14:100–178.
- Ahirwar S, Soni H, Prajapati BP, Kango N. 2017. Isolation and screening of thermophilic and thermotolerant fungi for production of hemicellulases from heated environments. Mycol. 8(3):125–134. doi:https://doi.org/10.1080/21501203.2017.1337657.
- Ahmed A, Khan M, Ahmad A, Khan S, Sohail M. 2018. Optimization of pectinase production from Geotrichum candidum AA15 using response surface methodology. Pak J Bot. 51. doi:https://doi.org/10.30848/PJB2019-2(41).
- Alazi E, Ram AFJ. 2018. Modulating Transcriptional Regulation of Plant Biomass Degrading Enzyme Networks for Rational Design of Industrial Fungal Strains, Front. Bioeng Biotechnol. 6:133.
- Allison SD, Romero-Olivares AL, Lu Y, Taylor JW, Treseder KK. 2018. Temperature sensitivities of extracellular enzyme V max and K m across thermal environments. Change Biol. 24(7):2884–2897. doi:https://doi.org/10.1111/gcb.14045.
- Almeida Carvalho E, Mendes dos Santos Góes L, Apt U, Galvão Paranhos da Silva E, Brito RL, Priminho PC, Miura da Costa A. 2016. Thermoresistant xylanases from Trichoderma stromaticum: application in bread making and manufacturing xylo-oligosaccharides. Food Chem. 10:101–144.
- Aro N, Pakula T, Penttila M. 2005. Transcriptional regulation of plant cell wall degradation by filamentous fungi. FEMS Microbiol Rev. 29(4):719–739. doi:https://doi.org/10.1016/j.femsre.2004.11.006.
- Asimov I. 1982. Asimov’s Biographical Encyclopedia of Science and Technology. II ed. New York: Doubleday.
- Bagewadi ZK, Mulla SI, Ninnekar HZ. 2018. Response surface methodology based optimization of keratinase production from Trichoderma harzianum isolate HZN12 using chicken feather waste and its application in dehairing of hide. J Environ Chem Eng. 6(4):4828–4839. doi:https://doi.org/10.1016/j.jece.2018.07.007.
- Baker SE. 2018. Protein hyperproduction in fungi by design, Appl. Microbiol Biotechnol. 102(20):8621–8628. doi:https://doi.org/10.1007/s00253-018-9265-1.
- Bali V, Panesar PS, Bera MB, Panesar R. 2015. Fructo-oligosaccharides: production, purification and potential applications. Crit Rev Food Sci Nutr. 55(11):1475–1490. doi:https://doi.org/10.1080/10408398.2012.694084.
- Bao M, Niu C, Xua X, Zheng F, Liu C, Wang J, Li Q. 2019. Identification, soluble expression, and characterization of a novel endo-inulinase from Lipomyces starkeyi NRRL Y-11557. Int J Biol Macromol. 137:537–544. doi:https://doi.org/10.1016/j.ijbiomac.2019.06.096.
- Baskar G, Garrick BG, Lalitha K, Chamundeeswari M. 2018. Gold nanoparticle mediated delivery of fungal asparaginase against cancer cells. J Drug Deliv Sci Technol. 44. doi:https://doi.org/10.1016/j.jddst.2018.02.007.
- Basu M, Kumar V, Shukla P. 2018. Recombinant approaches for microbial xylanases: recent advances and perspectives. Curr Protein Pept Sci. 19:87–99.
- Batista JMS, Brandão - Costa, RMP, da Cunha HMNC, Rodrigues OS, Porto ALF. 2020. Purification and biochemical characterization of an extracellular fructosyltransferase - rich extract produced by Aspergillus tamarii Kita UCP1279. Biocatal Agric Biotechnol. 26:101–647. doi:https://doi.org/10.1016/j.bcab.2020.101647.
- Beatriz B, Cardoso Sara C, Silvério Abrunhosa L, José A, Teixeira Lígia R, Rodrigues. 2017. β-galactosidase from Aspergillus lacticoffeatus: a promising biocatalyst for the synthesis of novel prebiotics Int. J Food Microbiol. 257:67–74. doi:https://doi.org/10.1016/j.ijfoodmicro.2017.06.013.
- Bennett TP, Frieden E. 1969. Modern Topics in Biochemistry. London: Macmillan Ltd.
- Bernasconi R, Molinari M. 2011. ERAD and ERAD tuning: disposal of cargo and of ERAD regulators from the mammalian ER. Curr Opin Cell Biol. 23(2):176–183. doi:https://doi.org/10.1016/j.ceb.2010.10.002.
- Bhardwaj N, Verma VK, Chaturvedi V, Verma P. 2020. Cloning, expression and characterization of a thermo-alkali-stable xylanase from Aspergillus oryzae LC1 in Escherichia coli BL21(DE3). Protein Expr Purif. 168:105–551. doi:https://doi.org/10.1016/j.pep.2019.105551.
- Blibech M, Ellouz Ghorbel RE, Chaari F, Dammak I, Bhiri F, Neifar M, Ellouz Chaabouni SE. 2011. Improved mannanase production from Penicillium occitanis by fed-batch fermentation using acacia seeds. ISRN Microbiol. 1–5. doi:https://doi.org/10.5402/2011/938347.
- Boyce A, Walsh G. 2012. Identification of fungal proteases potentially suitable for environmentally friendly cleaning-in-place in the dairy industry. Chemosphere. 88(2):211–218. doi:https://doi.org/10.1016/j.chemosphere.2012.03.022.
- Bruno C, Aitaa Stéfani S, Spannemberga Schmaltza S, Giovani L, Zabotb Marcus V, Tresb Raquel C, Kuhna Marcio A, Mazutti. 2019. Production of cell-wall degrading enzymes by solid-state fermentation using agro-industrial residues as substrates. J Environ Chem Eng. 7:103–193.
- Carrasco M, Alcaíno J, Cifuentes V, Baeza M. 2017. Purification and characterization of a novel cold adapted fungal glucoamylase. Microb Cell Factories. 16(1):75. doi:https://doi.org/10.1186/s12934-017-0693-x.
- Chen H. 2013. Modern solid state fermentation. Theory and practice. The Netherlands: Springer. doi:https://doi.org/10.1007/978-94-007-6043-1.
- Choukade R, Kango N. 2019. Characterization of a mycelial fructosyltransferase from Aspergillus tamarii NKRC 1229 for efficient synthesis of fructooligosaccharides. Food Chem. 286:434–440. doi:https://doi.org/10.1016/j.foodchem.2019.02.025.
- Choukade R, Kango N. 2020. Applications of Fungal Inulinases. Reference Module in Life Sciences. doi:https://doi.org/10.1016/B978-0-12-819990-9.00016-0
- Da Cunhaa MC, Silvab LC, Satoa HH, De Castroa RJS. 2018. Using response surface methodology to improve the L-asparaginase production by Aspergillus niger under solid-state fermentation. Biocatal Agric Biotechnol. 16:31–36. doi:https://doi.org/10.1016/j.bcab.2018.07.018.
- Daljit SA, Rakesh KS. 2009. Ligninolytic Fungal Laccases and their Biotechnological Applications. Appl Biochem Biotechnol. 160:1760–1788.
- Dandan N, Xiaojing T, Peace MN, Chao J, Suren S, Xiaoguang L, Prior Bernard A, Fuping L. 2017. Biochemical characterization of three Aspergillus niger β-galactosidases. Electron J Biotechnol. 27:37–43. doi:https://doi.org/10.1016/j.ejbt.2017.03.001.
- Darwesh OM, El-Maraghyb SH, Abdel-Rahmanb HM, Zaghloul RA. 2020. Improvement of paper wastes conversion to bioethanol using novel cellulose degrading fungal isolate. Fuel. 262:116–518. doi:https://doi.org/10.1016/j.fuel.2019.116518.
- De La Cruz R, Ascacio-Valdés J, Buenrostro Figueroa J, Sepúlveda L, Rodriguez R, Prado LA, Contreras-Esquivel J, Aguilera A, Aguilar C. 2017. Optimization of Ellagitannase Production by Aspergillus Niger GH1 by Solid-State Fermentation. Prep Biochem Biotechnol. 45(7). doi:https://doi.org/10.1080/10826068.2014.940965.
- De Medeiros IP, Rozental S, Costa AS, Macrae A, Hagler AN, Jose RA, Vermelho AB. 2016. Biodegradation of keratin by Trichosporum loubieri RC-S6 isolated from tannery/leather waste. Int Biodeterior Biodegradation. 115:199–204. doi:https://doi.org/10.1016/j.ibiod.2016.08.006.
- Descamps F, Brouta F, Vermout S, Monod M, Losson B, Mignon B. 2003. Recombinant expression and antigenic properties of a 31.5-kDa keratinolytic subtilisin-like serine protease from Microsporum canis. FEMS Immunol. Med Microbiol. 38:29–34.
- Deshpande N, Wilkins MR, Packer N, Nevalainen H. 2008. Protein glycosylation pathways in filamentous fungi. Glycobiol. 18(8):626–637. doi:https://doi.org/10.1093/glycob/cwn044.
- Dolashki A, Abrashev R, Stevanovi S, Stefanova L, Abid Ali S, Velkova L, Hristova R, Angelova M, Voelter W, Devreese B, et al. 2008. Biochemical properties of Cu/Zn-superoxide dismutase from fungal strain Aspergillus niger 26. Spectrochim. Acta A Mol Biomol Spectrosc. 71(3):975–983. doi:https://doi.org/10.1016/j.saa.2008.02.023.
- Dong-sheng X, Yuan-long L, Dong-qiang L, Chun-jie G, Shan-Jing Y. 2017. Halostable catalytic properties of exoglucanase from a marine Aspergillus niger and secondary structure change caused by high salinities. Process Biochem, 58. https://doi.org/http://dx.doi.org/10.1016/j.procbio.2017.02.005
- Elena V, Eneyskaya Alexander M, Golubev Farid M, Ibatullin Gustav S. 2009. Transglycosylating and hydrolytic activities of the β-mannosidase from Trichoderma reesei. Biochimie. 91(5):632–638. doi:https://doi.org/10.1016/j.biochi.2009.03.009.
- Escarambonia B, Fernández Núñezb EG, Azevedo Carvalhoa AF, Oliva Neto P. 2018. Ethanol biosynthesis by fast hydrolysis of cassava bagasse using fungal amylases produced in optimized conditions. Ind Crops Prod. 112:368–377. doi:https://doi.org/10.1016/j.indcrop.2017.12.004.
- Falkoski DL, Guimarães VM, de Almeida MN, Alfenas AC, Colodette JL, de Rezende ST. 2013. Chrysoporthe cubensis: a new source of cellulases and hemicellulases to application in biomass saccharification processes. Bioresour Technol. 130:296–305. doi:https://doi.org/10.1016/j.biortech.2012.11.140.
- Fatimi A, Wahab AF, Abdul Karim NA, Ling JG, Hasan NS, Yee YH, Bharudin I, Kamaruddin S, Abu Bakar FD, Abdul Murad AM. 2018. Functional characterisation of cellobiohydrolase I (Cbh1) from Trichoderma virens UKM1 expressed in Aspergillus niger. Protein Expr Purif. 154:52–61.
- Gabriela AM, Tatiana FP. 2005. A rapid screening method for cutinase producing microorganisms. Braz J Microbiol. 36:388–394.
- Ganaie MA, Rawat HK, Wani OA, Gupta US, Kango N. 2014. Immobilization of fructosyltransferase by chitosan and alginate for efficient production of fructooligosaccharides. Process Biochem. 49(5):840–844. doi:https://doi.org/10.1016/j.procbio.2014.01.026.
- Gao J, Xu X, Huang K, Liang Z. 2021. Fungal G-Protein-Coupled Receptors: a Promising Mediator of the Impact of Extracellular Signals on Biosynthesis of Ochratoxin A. Front Microbiol. 12:193. doi:https://doi.org/10.3389/fmicb.2021.631392.
- Gao Y, Zhao J, Zu Y, Fu Y, Liang L, Luo M, Wang W, Efferth T. 2012. Antioxidant properties, superoxide dismutase and glutathione reductase activities in HepG2 cells with a fungal endophyte producing apigenin from pigeon pea [Cajanus cajan (L.) Millsp.]. Food Res Int. 49(1):147–152. doi:https://doi.org/10.1016/j.foodres.2012.08.001.
- Garcia NFL, Da Silva Santos FR, Bocchini DA, da Paza MF, Fonseca GG, Leite RSR. 2018. Catalytic properties of cellulases and hemicellulases produced by Lichtheimia ramosa: potential for sugarcane bagasse saccharification. Ind Crops Prod. 122:49–56. doi:https://doi.org/10.1016/j.indcrop.2018.05.049.
- Gilmore SP, Lillington SP, Haitjema CH, de Groot R, O’Malley MA. 2020. Designing chimeric enzymes inspired by fungal cellulosomes. Synth Syst Biotechnol. 5(1):23–32. doi:https://doi.org/10.1016/j.synbio.2020.01.003.
- Gopalan N, Nampoothiri KM, Szakacs G, Parameswaran B, Pandey A. 2016. Solid-state fermentation for the production of biomass valorizing feruloyl esterase. Biocatal Agric Biotechnol. 7:7–13.
- Griebeler N, Polloni AE, Remonatto D, Arbter F, Vardanega R, Cechet JL, Di Luccio M, De Oliveira M, Treichel H, Cansian RL, et al. 2011. Isolation and Screening of Lipase-Producing Fungi with Hydrolytic Activity. Food Bioproc Technol. 4(4):578–586. doi:https://doi.org/10.1007/s11947-008-0176-5.
- Guerberoff GK, Camusso CC. 2019. Effect of laccase from Trametes versicolor on the oxidative stability of edible vegetable oils. Food Sci Hum. 8(4):356–361.
- Gürköka S, Söylerb B, Bielyc P, Ögel ZB. 2009. Cloning and heterologous expression of the extracellular alpha-galactosidase from Aspergillus fumigatus in Aspergillus sojae under the control of gpdA promoter. J Mol Catal B Enzym. 64(3–4):146–149. doi:https://doi.org/10.1016/j.molcatb.2009.09.012.
- Hamid R, Khan MA, Ahmad M, Ahmad MM, Abdin MZ, Musarrat J, Javed S. 2013. Chitinases: an update. J Pharm Bioallied Sci. 5(1):21–29. doi:https://doi.org/10.4103/0975-7406.106559.
- Hao Y, Chen Y, Li Q, Gao Q. 2010. Preparation of starch nanocrystals through enzymatic pretreatment from waxy potato starch. Carbohydr Polym. 184:171–177. doi:https://doi.org/10.1016/j.carbpol.2017.12.042.
- Hasan F, Ali Shah A, Hameed A. 2006. Industrial applications of microbial lipases. Enzyme Microb Technol. 39(2):235–251. doi:https://doi.org/10.1016/j.enzmictec.2005.10.016.
- Hassan SS, Tiwari BK, Williams GA. 2019. Bioprocessing of brewer’s spent grain for production of xylanopectinolytic enzymes by Mucor sp. Bioresour Technol Rep. 9:100–371.
- Homthong M, Kubera A, Srihuttagum M, Hongtrakul V. 2016. Isolation and characterization of chitinase from soil fungi, Paecilomyces sp. Agric Nat Resour. 50(4):232–242.
- Hosseini H, Ghorbani M, Jafari SM, Mahoonak AS. 2018. Investigating the effect of lipase from Candida rugosa on the production of EPA and DHA concentrates from Kilka fish (Clupeonella cultiventris caspia). LWT - Food Sci Technol. 90:534–541. doi:https://doi.org/10.1016/j.lwt.2018.03.066.
- Hou CT, Shimada Y. 2009. Applied Microbiology: Industrial Lipases. In: Encyclopedia of Microbiology. Third ed. Amsterdam.: Elsevier Inc.: p. 385–392.
- Hu W, Liu X, Li Y, Liu D, Kuang Z, Qian C, Yao D. 2017. Rational design for the stability improvement of Armillariella tabescens β-mannanase MAN47 based on N-glycosylation modification. Enzyme Microb Technol. 97:82–89. doi:https://doi.org/10.1016/j.enzmictec.2016.11.005.
- Hui Shan GT, Bin Y, Philip C, Quan Liu S. 2011. Lipase-catalysed synthesis of natural aroma-active 2-phenylethyl esters in coconut cream. Food Chem. 124(1):80–84. doi:https://doi.org/10.1016/j.foodchem.2010.05.108.
- Huixing L, Zhang R, Tang L, Zhang J, Zhonggui M. 2014. Manganese Peroxidase Production from Cassava Residue by Phanerochaete chrysosporium in Solid State Fermentation and Its Decolorization of Indigo Carmine. Chin J Chem Eng. 23(1):227–233.
- Ismail SA, Hassan AA, Emran MA. 2019. Economic production of thermoactive endo β-mannanase for the removal of food stain and production of antioxidant mannooligosaccharides. Biocatal Agric Biotechnol. 22:101–387. doi:https://doi.org/10.1016/j.bcab.2019.101387.
- I-Son N, Chen-Wei L, Shuang-Pi C, Jiun-Ly C, Po Ting C, Chii-Gong T, Su-May Y, Tuan-Hua DH. 2010. High-level production of a thermoacidophilic β-glucosidase from Penicillium citrinum YS40-5 by solid-state fermentation with rice bran. Bioresour Technol. 101(4):1310–1317. doi:https://doi.org/10.1016/j.biortech.2009.08.049.
- Jana UK, Kango N. 2020. Characteristics and bioactive properties of mannooligosaccharides derived from agro-waste mannans. Int J Biol Macromol. 149:931–940. doi:https://doi.org/10.1016/j.ijbiomac.2020.01.304.
- Jana UK, Suryawanshi RK, Prajapati BP, Soni H, Kango N. 2018. Production optimization and characterization of mannooligosaccharide generating β-mannanase from Aspergillus oryzae. Bioresour Technol. 268:308–314. doi:https://doi.org/10.1016/j.biortech.2018.07.143.
- Jiang H, Ma Y, Chi Z, Liu GL, Chi ZM. 2016. Production, purification, and gene cloning of a β-fructofuranosidase with a high inulin-hydrolyzing activity produced by a novel yeast Aureobasidium sp. p6 isolated from a mangrove ecosystem. Mar Biotechnol. 18(4):500–510. doi:https://doi.org/10.1007/s10126-016-9712-x.
- Ge J, Jiang X, Liu W, Wang Y, Huang H, Bai Y, Su X, Yao B, Luo H. 2020. Characterization, stability improvement, and bread baking applications of a novelcold-adapted glucose oxidase from Cladosporium neopsychrotolerans SL16. Food Chem. 310:125-970.
- Jing L, Zhenming C, Xiang HW. 2010. Cloning of the SAP6 gene of Metschnikowia reukaufii and its heterologous expression and characterization in Escherichia coli. Microbiol Res. 165(3):173–182. doi:https://doi.org/10.1016/j.micres.2008.08.003.
- Jun H, Kieselbach T, Jönsson LJ. 2011. Enzyme production by filamentous fungi: analysis of the secretome of Trichoderma reesei grown on unconventional carbon source. Microb Cell Factories. 10(1):68. doi:https://doi.org/10.1186/1475-2859-10-68.
- Jun-Jin D, Dan S, He-hua M, Zhi-wei L, Shuang L, Ye K, Xiao-chun L. 2019. Heterologous expression and characterization of an antifungal chitinase (Chit46) from Trichoderma harzianum GIM 3.442 and its application in colloidal chitin conversion. Int J Biol Macromol. 134:113–121. doi:https://doi.org/10.1016/j.ijbiomac.2019.04.177.
- Juodeikiene G, Basinskiene L, Vidmantiene D, Makaravicius T, Bartkiene E, Schols H. 2011. The use of β-xylanase for increasing the efficiency of biocatalytic conversion of crop residues to bioethanol. Catalysis Today. 167(1):113–121. doi:https://doi.org/10.1016/j.cattod.2011.02.059.
- Jus S, Stachel I, Schloegl W, Pretzler M, Friess W, Meyer M, Birner-Gruenberger R, Guebitz GM. 2011. Cross-linking of collagen with laccases and tyrosinases. Mater Sci Eng C. 31(5):1068–1077. doi:https://doi.org/10.1016/j.msec.2011.03.007.
- Kango N, Agarwal SC, Jain PC. 2005. Production and properties of thermostable xylanase by Thermomyces lanuginosus NK-2 grown on lignocelluloses. Biotechnol. 5(2):148–152.
- Kango N, Jain PC. 2011. Production and Properties of Microbial Inulinases: recent Advances. Food Biotechnol. 25(3):165–212. doi:https://doi.org/10.1080/08905436.2011.590763.
- Karima KMR, Husaini A, Ngui Sing N, Tasnimc T, Sinang FS, Hussain H, Hossain MA, Roslan H. 2019. Characterization and expression in Pichia pastoris of a raw starch degrading glucoamylase (GA2) derived from Aspergillus flavus NSH9. Protein Expr Purif. 164:105–462.
- Katrolia P, Liu X, Zhao Y, Kopparapu NK, Zheng X. 2019. Gene cloning, expression and homology modeling of first fibrinolytic enzyme from mushroom (Cordyceps militaris). Int J Biol Macromol. 146:897–906. doi:https://doi.org/10.1016/j.ijbiomac.2019.09.212.
- Katrolia P, Yan Q, Jia H, Li Y, Jiang Z, Song C. 2011. Molecular cloning and high-level expression of a β-galactosidase gene from Paecilomyces aerugineus in Pichia pastoris. J Mol Catal B Enzym. 69(3–4):112–119. doi:https://doi.org/10.1016/j.molcatb.2011.01.004.
- Khangwal I, Nath S, Kango N, Shukla P. 2020. Endo-xylanase induced xylooligosaccharide production from corn cobs, its structural features, and concentration-dependent antioxidant activities. Biomass Conv Bioref. doi:https://doi.org/10.1007/s13399-020-00997-3
- Klein N, Zouraria A, Lortal S. 2002. Peptidase activity of four yeast species frequently encountered in dairy products—comparison with several dairy bacteria. Int Dairy J. 12(10):853–861. doi:https://doi.org/10.1016/S0958-6946(02)00081-X.
- Knob A, Izidoro SC, Lacerda LT, André R, De Lima VA. 2020. A novel lipolytic yeast Meyerozyma guilliermondii: efficient and low-cost production of acid and promising feed lipase using cheese whey. Biocatal Agric Biotechnol. 24:101–565. doi:https://doi.org/10.1016/j.bcab.2020.101565.
- Kruthi D, Devarai SK. 2016. Isolation and screening of L-asparaginase free of glutaminase and urease from fungal sp. 3 Biotech. 6:239.
- Kuhne W. 1877. Uber das Verhalten verchiedener organisirter und sog. Ungeformter Fermente. Verhandlungen des heidelb, Naturhist -Med Vereins Neue Folge. 1(3):190–193.
- Kumar NV, Rani ME, Gunaseeli R, Kannan ND. 2018. Enhanced biobleaching efficacy and heavy metal remediation through enzyme mediated lab-scale paper pulp deinking process. J Clean Prod. 203:926–932. doi:https://doi.org/10.1016/j.jclepro.2018.08.335.
- Kumara BV, Sarabhaia S, Prabhasankar P. 2019. Targeted degradation of gluten proteins in wheat flour by prolyl endo-protease and its utilization in low immunogenic pasta for gluten sensitivity population. J Cereal Sci. 87:59–67. doi:https://doi.org/10.1016/j.jcs.2019.03.001.
- Li K, Meng K, Pan X, Ma R, Yang P, Huang H, Yao B, Su X. 2015. Two thermophilic fungal pectinases from Neosartorya fischeri P1: gene cloning, expression and biochemical characterization. J Mol Catal B Enzym. 118:70–78. doi:https://doi.org/10.1016/j.molcatb.2015.05.005.
- Li S, Sing S, Wang Z. 2011. Improved expression of Rhizopus oryzae α-amylase in the methylotrophic yeast Pichia pastoris. Protein Expr Purif. 79(1):142–148. doi:https://doi.org/10.1016/j.pep.2011.05.007.
- Li Y, Yi P, Wang N, Liu J, Liu X, Yan Q, Jiang Z. 2017. High level expression of β-mannanase (RmMan5A) in Pichia pastoris for partially hydrolyzed guar gum production. Int J Biol Macromol. 105:1171–1179. doi:https://doi.org/10.1016/j.ijbiomac.2017.07.150.
- Lin MI, Nagata T, Katahira M. 2018. High yield production of fungal manganese peroxidases by E. coli through soluble expression, and examination of the activities. Protein Expr Purif. 145:45–52. doi:https://doi.org/10.1016/j.pep.2017.12.012.
- Lin X, Dong L, Yu D, Wang B, Pan L. 2019. High-level expression and characterization of the thermostable leucine aminopeptidase Thelap from the thermophilic fungus Thermomyces lanuginosus in Aspergillus niger and its application in soy protein hydrolysis. Protein Expr Purif. 167:105–544.
- Liu Y, Li Y, Tong S, Yuan M, Wang X, Wang J, Fan Y. 2020b. Expression of a Beauveria bassiana chitosanase (BbCSN-1) in Pichia pastoris and enzymatic analysis of the recombinant protein. Protein Expr Purif. 166:105–519. doi:https://doi.org/10.1016/j.pep.2019.105519.
- Liu Z, Ning C, Yuan M, Yang S, Wei X, Xiao M, Fu X, Zhu C, Mou H. 2019. High level expression of a thermophilic and acidophilic β-mannanase from Aspergillus kawachii IFO 4308 with significant potential in mannooligosaccharide preparation. Bioresour Technol. 295:122–257.
- Lópeza DN, Galantea M, Ruggieria G, Piaruchia J, Diba ME, Durana MN, Lombardia J, De Sanctisa M, Boerisa V, Rissoa PH, et al. 2018. Peptidase from Aspergillus niger NRRL 3: optimization of its production by solid-state fermentation, purification and characterization. LWT - Food Sci Technol. 98:485–491. doi:https://doi.org/10.1016/j.lwt.2018.09.013.
- Maalej-Achouri I, Guerfali M, Belhaj-Ben Romdhane I, Gargouri A, Belghith H. 2012. The effect of Talaromyces thermophilus cellulase-free xylanase and commercial laccase on lignocellulosic components during the bleaching of kraft pulp. Int Biodeterior Biodegradation. 75:43–48. doi:https://doi.org/10.1016/j.ibiod.2012.04.015.
- Mahmoodi M, Najafpour GD, Mohammadi M. 2019. Bioconversion of agroindustrial wastes to pectinases enzyme via solid state fermentation in trays and rotating drum bioreactors. Biocatal Agric Biotechnol. 21:101–280. doi:https://doi.org/10.1016/j.bcab.2019.101280.
- Malherbe AR, Rose SH, Viljoen-Bloom M, Van Zyl WH. 2014. Expression and evaluation of enzymes required for the hydrolysis of galactomannan. J Ind Microbiol Biotechnol. 41(8):1201–1209. doi:https://doi.org/10.1007/s10295-014-1459-7.
- Mandari V, Nema A, Devarai SK. 2019. Sequential optimization and large scale production of lipase using tri-substrate mixture from Aspergillus niger MTCC 872 by solid state fermentation. Process Bioch. 43:1.
- Mansora MS, Ramlia NY, Abdul Rashida N, Samatb MN, Lanid SA, Sharifudina S, Raseethac. 2019. Evaluation of selected agri-industrial residues as potential substrates for enhanced tannanase production via solid-state fermentation. Biocatal Agric Biotechnol. 20:101–216.
- Marraiki N, Viayaraghavan P, Elgorban AM, DeepaDhas DS, Al-Rashed S, Yassin V. 2020. Low cost feedstock for the production of Endoglucanase in solid state fermentation by Trichoderma hamatum NGL1 using response surface methodology and saccharification efficacy. J King Saud Univ Sci. 32(2):1718–1724. doi:https://doi.org/10.1016/j.jksus.2020.01.008.
- Martínez-Pachecoa MM, Flores-Garcíaa A, Zamudio-Jaramillob MA, Chávez-Pargab MC, Alvarez-Navarrete M. 2019. Optimization of production of xylanases with low cellulases in Fusarium solani by means of a solid-state fermentation using statistical experimental design. Rev Argent Microbiol. 52(4):328–338. doi:https://doi.org/10.1016/j.ram.2019.12.003.
- Masuoa N, Itob K, Yoshimunea K, Hoshinoa M, Matsushimab K, Koyamab Y, Moriguchi M. 2004. Molecular cloning, overexpression, and purification of Micrococcus luteus K-3-type glutaminase from Aspergillus oryzae RIB40. Protein Expr Purif. 38(2):272–278. doi:https://doi.org/10.1016/j.pep.2004.09.003.
- Matkawala F, Nighojkar S, Kumar A, Nighojkar A. 2019. Enhanced production of alkaline protease by Neocosmospora sp. N1 using custard apple seed powder as inducer and its application for stain removal and dehairing. Biocatal Agric Biotechnol. 21:101–310. doi:https://doi.org/10.1016/j.bcab.2019.101310.
- Melikoglu M, Lin CSK, Webb C. 2015. olid state fermentation of waste bread pieces by Aspergillus awamori: analysing the effects of airflow rate on enzyme production in packed bed bioreactors. Food Bioprod Process. 95:63–75. doi:https://doi.org/10.1016/j.fbp.2015.03.011.
- Melnichuk N, Braia MJ, Anselmi PA, Meini MR, Romanini D. 2020. Valorization of two agro-industrial wastes to produce alpha-amylase enzyme from Aspergillus oryzae by solid-state fermentation. Waste Management. 106:155–161. doi:https://doi.org/10.1016/j.wasman.2020.03.025.
- Mittal A, Singh G, Goyal V, Yadav A, Aneja KR, Gautam SK, Aggarwal NK. 2011. Isolation and biochemical characterization of acido-thermophilic extracellular phytase producing bacterial strain for potential application in poultry feed. Jundishapur J Microbiol. 4(4):273–282.
- Mudgil D. 2017. The interaction between insoluble and soluble fiber. In: rodney ASamaan, Editor. Dietary Fiber for the Prevention of Cardiovascular Disease. New York: Academic press; p. 35–59.
- Murthy PS, Palakshappa SH, Padela J, Kusumoto KI. 2020. Amelioration of cocoa organoleptics using A. oryzae cysteine proteases. LWT - Food Sci Technol. 120:108–919. doi:https://doi.org/10.1016/j.lwt.2019.108919.
- Naik B, Goyal SK, Tripathi AD, Kumar V. 2019. Screening of agro-industrial waste and physical factors for the optimum production of pullulanase in solid-state fermentation from endophytic Aspergillus sp. Biocatal Agric Biotechnol. 22:101–423. doi:https://doi.org/10.1016/j.bcab.2019.101423.
- Navada KK, Kulal A. 2019. Enhanced production of laccase from gamma irradiated endophytic fungus: a study on biotransformation kinetics of aniline blue and textile effluent decolourisation. J Environ Chem Eng. 8(2):103–550.
- Okongo RN, Puri AK, Wang Z, Singh S, Permaul K. 2019. Comparative biocontrol ability of chitinases from bacteria and recombinant chitinases from the thermophilic fungus Thermomyces lanuginosus. J Biosci Bioeng. 127(6):663–671. doi:https://doi.org/10.1016/j.jbiosc.2018.11.007.
- Omrane Benmrad M, Moujehed E, Ben Elhoul M, Mechri S, Bejar S, Zouari R, Baffoun A, Jaouadi B. 2018. Production, purification, and biochemical characterization of serine alkaline protease from Penicillium chrysogenium strain X5 used as excellent bio-additive for textile processing. Int J Biol Macromol. 119:1002–1016. doi:https://doi.org/10.1016/j.ijbiomac.2018.07.194.
- Ortiz-Monsalve S, Dornelles J, Poll E, Ramirez-Castrillon M, Valente P, Gutterres M. 2017. Biodecolourisation and biodegradation of leather dyes by a native isolate of Trametes villosa. Process Saf Environ. 109:437–451. doi:https://doi.org/10.1016/j.psep.2017.04.028.
- Oumer OJ, Abate D. 2018. Screening and molecular identification of pectinase producing microbes from coffee pulp. Biomed Res Int. Article ID 2961767. 7 pages. https://doi.org/10.1155/2018/2961767
- Panesar PS, Kaur R, Singh RS. 2016. Isolation and screening of fungal strains for β-Galactosidase production. Int J Biol Biomol Agri Food Biotechnol Eng. 10:7.
- Parashar D, Satyanarayana T. 2017. Engineering a chimeric acid-stable α-amylase-glucoamylase (Amy-Glu) for one step starch saccharification. Int J Biol Macromol. 99:274–281. doi:https://doi.org/10.1016/j.ijbiomac.2017.02.083.
- Payen A, Persoz JF. 1833. Memoir of diastase, the principal products of its reactions, and their applications to the industrial arts. Annales de Chimie et de Physique. 53:73–92.
- Peberdy JF. 1994. Protein secretion in filamentous fungi- trying to understand a highly productive black box. In: Trends Biotechnol. Elsevier ltd. Amsterdam. p. 12.
- Pérez S, Tvaroška I. 2014. Carbohydrate–Protein Interactions. Adv Carbohydr Chem Biochem. 71:9–136.
- Pires EBE, de Freitas AJ, Souza FF. 2019. Production of fungal phytases from agroindustrial byproducts for pig diets. Sci Rep. 9(1):9256. doi:https://doi.org/10.1038/s41598-019-45720-z.
- Pradoa DZ, Okino-Delgadoa CH, Zanutto-Elguia MR, Gomes da Silvab RB, Pereiraa MS, Jahnc L, Ludwig-Mullerc J, Ribeiro da Silvab M, Velinib EV, Fleuri LF. 2019. Screening of Aspergillus, Bacillus and Trichoderma strains and influence of substrates on auxin and phytases production through solid-state fermentation. Biocatal Agric Biotechnol. 19:101–165.
- Prajapati BP, Kumar Suryawanshi R, Agrawal S, Ghosh M, Kango N. 2018. Characterization of cellulase from Aspergillus tubingensis NKBP-55 for generation of fermentable sugars from agricultural residues. Bioresour Technol. 250:733–740. doi:https://doi.org/10.1016/j.biortech.2017.11.099.
- Ramanjaneyulu G, Praveen Kumar RG, Dileep Kumar K, Rajasekhar Reddy B. 2015. Isolation and Screening of Xylanase Producing Fungi from Forest Soils. Int J Curr Microbiol Appl Sci. 4(9):586–591.
- Ramos OL, Malcata FX. 2011. Food-Grade Enzymes. Comprehensive Biotechnol. 3:555–569.
- Rawat HK, Jain SC, Kango N. 2015. Production and properties of inulinase from Penicillium sp. NFCC 2768 grown on inulin-rich vegetal infusions. J Biocatal Biotransfor. 33(1):61–68. doi:https://doi.org/10.3109/10242422.2015.1018188.
- Regina Gern Sandra MM, Furlan Jorge A, Jonas NR. 2001. Screening for microorganisms that produce only endo-inulinase. Appl Microbiol Biotechnol. 55(5):632–635. doi:https://doi.org/10.1007/s002530000578.
- Ribeiro Corrêa TL, de Queiroz MV, de Araújo EF. 2014. Cloning, recombinant expression and characterization of a new phytase from Penicillium chrysogenum. Microbiol Res. 12:107–205.
- Rodrıguez-Couto S. 2018. Chapter 11 - Solid-State Fermentation for Laccases Production and Their Applications. In: Curr. Dev. Biotechnol. Bioeng. Elsevier B.V. Amsterdam. p. 211–234.
- Rungrattanakasina B, Premjet S, Thanonkeo S, Klanrita P, Thanonkeo P. 2018. Cloning and expression of an endoglucanase gene from the thermotolerant fungus Aspergillus fumigatus DBiNU-1 in Kluyveromyces lactis. Braz J Microbiol. 49(3):647–655. doi:https://doi.org/10.1016/j.bjm.2017.10.001.
- Sadeghian-Abadi S, Rezaei S, Yousefi-Mokri M, Faramarzi MA. 2019. Enhanced production, one-step affinity purification, and characterization of laccase from solid-state culture of Lentinus tigrinus and delignification of pistachio shell by free and immobilized enzyme. J Environ Manag. 244:235–246. doi:https://doi.org/10.1016/j.jenvman.2019.05.058.
- Saeed H, Ali H, Soudan H, Embaby A, El-Sharkawy A, Farag A, Husseina A, Atay F. 2018. Molecular cloning, structural modeling and production of recombinant Aspergillus terreus L-asparaginase in Escherichia coli. Int J Biol Macromol. 106:1041–1051. doi:https://doi.org/10.1016/j.ijbiomac.2017.08.110.
- Sahay S, Chouhan D. 2018. Study on the potential of cold-active lipases from psychrotrophic fungi for detergent formulation. J Genet Eng Biotechnol. 16(2):319–325. doi:https://doi.org/10.1016/j.jgeb.2018.04.006.
- Saini S, Chutani P, Kumar P, Sharma KK 2020. Development of an eco-friendly deinking process for the production of bioethanol using diverse hazardous paper wastes. Renew. Energy. 146:2362–2373.
- Schuster FPW, Maffessoni C, De Angelis DA, Giachini AJ, Cardoso DH, Moroni LS, Skoronski E, Kempka AP. 2019. Screening and evaluation of filamentous fungi potential for protease production in swine plasma and red blood cells-based media: qualitative and quantitative methods. Biocatal Agric Biotechnol. 21:101–313.
- Senthivelana T, Kanagaraja J, Pandab RC, Narayanib T. 2019. Screening and production of a potential extracellular fungal laccase from Penicillium chrysogenum: media optimization by response surface methodology (RSM) and central composite rotatable design (CCRD). Biotechnol Rep. 23:3–44.
- Shanmugavel M, Vasantharaj S, Yazhmozhi A, Bhavsar P, Aswin P, Felshia C, Mani U, Ranganathan B, Gnanamani A. 2018. A study on pectinases from Aspergillus tamarii: toward greener approach for cotton bioscouring and phytopigments processing. Biocatal Agric Biotechnol. 15:295–303. doi:https://doi.org/10.1016/j.bcab.2018.06.013.
- Sheludko YV, Fessner WD. 2020. Winning the numbers game in enzyme evolution – fast screening methods for improved biotechnology proteins. Curr Opin Struct Biol. 63:1–11. doi:https://doi.org/10.1016/j.sbi.2020.05.003.
- Singh RS, Chauhan K, Kaur K. 2018. Statistical optimization of solid-state fermentation for the production of fungal inulinase from apple pomace. Bioresour Technol Rep. 9:100–364.
- Singh RS, Singh T, Pandey A. 2019. Microbial Enzymes—An Overview. Adv Enzym Technol.Amsterdam: Elsevier B.V. 1–40.
- Singhania RR, Patel AK, Zoclo CR, Pandey A. 2008. Recent advances in solid-state fermentation. Biochem Eng J. 44(1):13–18. doi:https://doi.org/10.1016/j.bej.2008.10.019.
- Soni H, Rawat HK, Pletschke BI, Ahirwar N. 2016. Purification and characterization of β-mannanase from Aspergillus terreus and its applicability in depolymerization of mannans and saccharification of lignocellulosic biomass. 3 Biotech. 6(2):1–36. doi:https://doi.org/10.1007/s13205-016-0454-2.
- Spiropulos Gonçalves EC, Martí PM, Vici AC, Santos Salgado JC, de Souza Rocha M, Zaghetto de Almeida P, da Conceição Infante J, Sílvia de Almeida Scarcella A, Coutinho de Lucas R, Vieira AT, et al. 2020. Potential biodiesel production from Brazilian plant oils and spent coffee grounds by Beauveria bassiana lipase 1 expressed in Aspergillus nidulans A773 using different agroindustry inputs. J Clean Prod. 256:120–513.
- Sun Q, Chen F, Geng F, Luo Y, Gong S, Jiang Z. 2017. A novel aspartic protease from Rhizomucor miehei expressed in Pichia pastoris and its application on meat tenderization and preparation of turtle peptides. Food Chem. 245:570–577. doi:https://doi.org/10.1016/j.foodchem.2017.10.113.
- Sung HJ, Khan MF, Kim YH. 2019. Recombinant lignin peroxidase-catalyzed decolorization of melanin using in-situ generated H2O2 for application in whitening cosmetics. Int J Biol Macromol. 136:20–26. doi:https://doi.org/10.1016/j.ijbiomac.2019.06.026.
- Tanriseven A, Aslan Y. 2005. Immobilization of pectinex ultra SP-L to produce fructooligosaccharides. Enzym Microb Technol. 36(4):550–554. doi:https://doi.org/10.1016/j.enzmictec.2004.12.001.
- Tao Y, Yang L, Yin L, Lai C, Huang C, Li X, Yong Q. 2019. Novel approach to produce biomass-derived oligosaccharides simultaneously by recombinant endoglucanase from Trichoderma reesei. Enzym Microb Technol. 134:109–481.
- Trivedi S, Divecha J, Shah A. 2012. Optimization of inulinase production by a newly isolated Aspergillus tubingensis CR16 using low cost substrates. Carbohydr Polym. 90(1):483–490. doi:https://doi.org/10.1016/j.carbpol.2012.05.068.
- Vaidya S, Srivastava PK, Rathore P, Pandey AK. 2015. Amylases: a prospective enzyme in the field of biotechnology. J Appl Biosci. 41:1–18.
- Veerapagu M, Jeya KR, Sankaranarayanan A. 2016. Screening and production of fungal amylase from Aspergillus sp. by SSF. J Global Biosci. 5(8):4443–4450.
- Verma D, Fortunati E. 2019. Biopolymer processing and its composites. Biomass, Biopolymer-Based Materials, and Bioenergy. Woodhead Publishing. Cambridge. England. 3–23.
- Vidhate RP, Bhide AJ, Gaikwad SM, Giri AP. 2019. A potent chitin-hydrolyzing enzyme from Myrothecium verrucaria affects growth and development of Helicoverpa armigera and plant fungal pathogens. Int J Biol Macromol. 141:517–528. doi:https://doi.org/10.1016/j.ijbiomac.2019.09.031.
- Vidya CH, Gnanesh Kumar BS, Chinmayee CV. 2020. Purification, characterization and specificity of a new GH family 35 galactosidase from Aspergillus awamori. Int J Biol Macromol. 156:885–895. doi:https://doi.org/10.1016/j.ijbiomac.2020.04.013.
- Wahlström RM, Suurnäkki A. 2015. Enzymatic hydrolysis of lignocellulosic polysaccharides in the presence of ionic liquids. Green Chem. 17(2):694–714. doi:https://doi.org/10.1039/C4GC01649A.
- Wang D, Li FL, Wang SA. 2016d. A one-step bioprocess for production of high-content fructo-oligosaccharides from inulin by yeast. Carbohydr Polym. 151:1220–1226. doi:https://doi.org/10.1016/j.carbpol.2016.06.059.
- Wang G, Zhang D, Chen S. 2014. Effect of earlier unfolded protein response and efficient protein disposal system on cellulase production in Rut C30. World J Microbiol Biotechnol. 30(10):2587–2595. doi:https://doi.org/10.1007/s11274-014-1682-4.
- Wang H, Kaur G, Pensupa N, Uisan K, Du C, Yang X, Lin CSK. 2018. Textile waste valorization using submerged filamentous fungal fermentation. Process Saf Environ. 118:143–151. doi:https://doi.org/10.1016/j.psep.2018.06.038.
- Wang J, Kang L, Liu Z, Yuan S. 2016a. Gene cloning, heterologous expression and characterization of a Coprinopsis cinerea endo-β-1,3(4)-glucanase. Fungal Biol. 121(1):61–68. doi:https://doi.org/10.1016/j.funbio.2016.09.003.
- Wang Q, Zhong C, Xiao H. 2020a. Genetic Engineering of Filamentous Fungi for Efficient Protein Expression and Secretion. Front Bioeng Biotechnol. 8:293. doi:https://doi.org/10.3389/fbioe.2020.00293.
- Wang Y, Zhao N, Ma J, Liu J, Yan Q, Jiang Z. 2019a. High-level expression of a novel α-amylase from Thermomyces dupontii in Pichia pastoris and its application in maltose syrup production. Int J Biol Macromol. 127:683–692.
- Wang YC, Hu HF, Ma JW, Yan QJ, Liu HJ, Jiang ZQ. 2020b. A novel high maltose-forming α-amylase from Rhizomucor miehei and its application in the food industry. Food Chem. 305:125–447. doi:https://doi.org/10.1016/j.foodchem.2019.125447.
- Wang Z, Hea Z, Shen Q, Gua Y, Li S, Yuan Q. 2005. Purification and partial characterization of recombinant Cu, Zn containing superoxide dismutase of Cordyceps militaris in E. coli. J. Chromatogr B Biomed Appl. 826(1–2):114–121. doi:https://doi.org/10.1016/j.jchromb.2005.08.010.
- Li XJ. Zheng RC. Wu ZM. Ding X. Zheng YG. 2014. Thermophilic esterase from Thermomyces lanuginosus: molecular cloning, functional expression and biochemical characterization. Protein Expr Purif. 101:1–7. doi:https://doi.org/10.1016/j.pep.2014.05.006.
- Xu G, Wang J, Yin Q, Fang W, Xiao Y, Fang Z. 2018. Expression of a thermo and alkali-philic fungal laccase in Pichia pastoris and its application. Protein Expr Purif. 154:16–24. doi:https://doi.org/10.1016/j.pep.2018.09.015.
- Xu-Cong L, Qi-Qi C, Xin-Xin K, Chen F, Ping-Fan R, Li N. 2015. Characterization of fungal community and dynamics during the traditional brewing of Wuyi Hong Qu glutinous rice wine by means of multiple culture-independent methods. Food Control. 54:231–239. doi:https://doi.org/10.1016/j.foodcont.2015.01.046.
- Yang X, Zhang Y. 2019. Expression of recombinant transglutaminase gene in Pichia pastoris and its uses in restructured meat products. Food Chem. 291:245–252. doi:https://doi.org/10.1016/j.foodchem.2019.04.015.
- You X, Qin Z, Li Y, Yan Q, Li B, Jiang Z. 2018. Structural and biochemical insights into the substrate-binding mechanism of a novel glycoside hydrolase family 134 β-mannanase. Biochim Biophys Acta Gen Subj BBA-GEN SUBJECTS. 1862(6):1376–1388. doi:https://doi.org/10.1016/j.bbagen.2018.03.016.
- Yu XW, Wang LL, Xu Y. 2009. Rhizopus chinensis lipase: gene cloning, expression in Pichia pastoris and properties. J Mol Catal (B): Enzym. 57(1–4):304–311. doi:https://doi.org/10.1016/j.molcatb.2008.10.002.
- Zhao J, Yuan PST, Huang H, Li Z, Meng K, Yang P, Yao B. 2012. Purification, gene cloning and characterization of an acidic β-1,4-glucanase from Phialophora sp. G5 with potential applications in the brewing and feed industries. J Biosci Bioeng. 114(4):379–384. doi:https://doi.org/10.1016/j.jbiosc.2012.04.021.
- Zheng F, Songa L, Basita A, Liua J, Miaoa T, Wena J, Caob Y, Jiang W. 2020a. An endoxylanase rapidly hydrolyzes xylan into major product xylobiose via transglycosylation of xylose to xylotriose or xylotetraose. Carbohydr Polym. 237:116–121. doi:https://doi.org/10.1016/j.carbpol.2020.116121.
- Zheng L, Yu X, Wei C, Qiu L, Yu C, Xing Q, Fan Y, Deng Z. 2020b. Production and characterization of a novel alkaline protease from a newly isolated Neurospora crassa through solid-state fermentation. LWT - Food Sci Technol. 122:108–990. doi:https://doi.org/10.1016/j.lwt.2019.108990.
- Zhi NY, Wen Jia W, Zhen SC, Fan LH, Bo CW, Qi Ping Z, Gui ZL, Xuan X, Juan MJ, Kun Y, et al. 2019. Antioxidant and Anti-inflammatory capacity of ferulic acid released from wheat bran by solid-state fermentation of Aspergillus niger. Biomed Environ Sci. 32(1):11–21.
- Znameroski EA, Samuel Coradetti T, Christine Roche M, Jordan Tsaia C, Anthony Iavarone T, Jamie Catea HD, Louise Glass N. 2012. Induction of lignocellulose-degrading enzymes in Neurospora crassa by cellodextrins. PNAS. 109(16):6012–6017. doi:https://doi.org/10.1073/pnas.1118440109.