ABSTRACT
A novel species of Hericium was recently collected in the Afrotemperate forests (Knysna – Amatole region) of Southern Africa. The novel species shares many similar, dentate features common to other species in Hericium, and its basidiome first appears stark white and yellows with age. However, the substrate choice and gloeocystidia and basidiospore sizes of the specimens collected were distinct from other Hericium species. This was confirmed by sequencing the ITS and 28S genetic markers, respectively. The novel species is described as Hericium ophelieae sp. nov. and appears unique as it grows on hardwoods indigenous to Southern Africa. The species has larger basidiospores and wider gloeocystidia compared to its closest relative. H. ophelieae sp. nov. is the first endemic species of the medicinal mushroom genus Hericium to be described from Southern Africa, and the second to be described from Africa, after its closest relative, H. bembedjaense, which was isolated in Cameroon. Although this is the first Hericium to be described from the Southern African region, there are likely others to be discovered, and this study highlights the need for further research into the fungal diversity of Afrotemperate environments.
1. Introduction
The family Hericiaceae (Russulales, Basidiomycota) consists of the three genera Hericium (Scop.) Pers, Laxitextum (Pers.) Lentz, and Dentipellis Donk (Das et al. Citation2011). The genus Hericium is currently represented by 28 species (Jumbam et al. Citation2019) and was first described by Schrank in 1786 as Hericiaceae (Index Fungorum Citation2023). It was renamed by Persoon, who in 1794 named Hericium coralloides (Scop.) Pers as the type species for the genus (Persoon Citation1794). Despite the comparative rarity of the genus in nature, the number of known species and the known distribution of the genus has expanded significantly, with the most recent species described by Jumbam et al. (Citation2019) and Singh and Das (Citation2019). To date, two species of Hericium have been recorded in Africa, namely H. erinaceus (Bull.) Persoon (Ouali et al. Citation2020) and H. bembedjaense Jumbam (Jumbam et al. Citation2019), although H. erinaceus is found on other continents such as Asia and North America. H. bembedjaense was described from a specimen found in the broadleaf tropical forests of the Dja Biosphere reserve, Cameroon. H. bembedjaense is the only endemic African species of this genus described to date, although there are very likely other species that are yet to be discovered (Jumbam et al. Citation2019). However, progress has been hindered by the lack of studies on African Russulales (Jumbam et al., Citation2019) and fungi in general, while the cryptic morphology of Hericium species makes it difficult to distinguish between species (Hallenberg, Nilsson, and Robledo, Citation2013). Molecular analysis techniques are now more regularly used in the identification and description of new species.
Members of the genus Hericium are a relatively rare sight in nature (Singh and Das Citation2019). These coral-like, dentate basidiomycetes are white rot decomposers. Ecologically, Hericium species play an important role in the breakdown of hardwoods and conifers (Jumbam et al. Citation2019) and can be found growing on both standing or fallen dead trees (Boddy et al. Citation2011).
Hericium species are often cultivated for their medicinal and culinary values. Historically, H. erinaceus has long been part of traditional Chinese and Asian medicine (Ghosh et al. Citation2021). The metabolites produced by both the fruiting bodies and mycelia of some members of the genus Hericium are of particular interest due to their bioactive nature. Several of the compounds produced – such as hericenones, erinacines, and corallocins -, have the potential to treat a variety of ailments with negligible side effects (Ma et al. Citation2010; Wittstein et al. Citation2016; Jumbam et al. Citation2019). For example, H. erinaceus has been shown to treat neuropsychiatric disorders like depression (Chong et al. Citation2020), while H. novae-zealandiae has anticancer properties (Chen et al. Citation2019). H. coralloides was found to have anti-ageing and antioxidant properties (Zhang et al. Citation2019).
Recently, specimens believed to be H. coralloides were collected from hardwoods indigenous to the Knysna – Amatole Southern Afrotemperate region of South Africa (Mucina and Rutherford Citation2006). However, based on phylogenetic analysis and differences in morphology, it is believed that these specimens represent a previously undescribed species. In this paper, this novel species was characterised based on phylogenetic analysis and morphological characteristics and described as Hericium ophelieae sp. nov.
2. Materials and Methods
2.1. Morphological study
Fruiting bodies were sporadically collected for three years (2019–2021) between December and March from the Knysna – Amatole Southern Afrotemperate region. The specimens for this species were collected from Rapanea melanophloeos (L.) Mez, Ilex mitis (L.) Radlk., Olea capensis Lam., and Ocotea bullata (Burch.) E. Meyer host trees. The macro features of the fresh basidiomata were noted. Fresh specimens were photographed in the field, collected, and then preserved for further study by freezing at −20 °C or by drying using silica gel.
For the micromorphological studies, dried fruiting bodies were hydrated using 3% KOH. Tissue samples were stained using Congo Red (Pallua et al. Citation2012) and fixed to slides using Shears mounting fluid. The basidiospores, gloeocystidia, and basidia were observed, measured, and drawn under a differential interference contrast microscope (Nikon Eclipse E800, Japan) with a CFI plain Apochromat VC 100X lens. At least 20 examples for each feature were examined and measured at 400X and 1,000X magnification. Spore sizes were measured in length (L) and width (W) with the spores in side profile. Calculations were made as (La – Lb – Lc) and (Wa – Wb – Wc) where Xa = smallest measurement, Xb = average of the measurements and Xc = the largest measurement (Jumbam et al. Citation2019).
2.2. DNA extraction, PCR amplification, and sequencing
Genomic DNA from both dried Hericium basidiomata and cultured mycelia was extracted using ZR Quick-DNA Fungal/Bacterial Miniprep Kits (Zymo Research, USA). Approximately 100 mg of fungal tissue was used per extraction and extractions were carried out according to the manufacturer’s instructions. Successful DNA extractions were visualised on a 1% agarose gel with ethidium bromide. DNA was then kept at −20 °C for storage until Polymerase Chain Reaction (PCR) reactions were performed.
A PCR amplification was performed on the extracted DNA, using universal primers to target the Internal Transcribed Spacer (ITS) and 28S ribosomal RNA regions. For ITS, ITS1 (5’-TCCGTAGGTGAACCTGCG-3’) and ITS4 (5’-TCCTCCGCTTATTGATATGC-3’) primers were used, and LR0R (5’-ACCCGCTGAACTTAAGC-3’) and LR6 (5’-CGCCAGTTCTGCTTACC-3’) were used for the 28S region (Moncalvo, François M. Lutzo, Citation2000; Vilgalys and Hester Citation1990; White et al. Citation1990; Jumbam et al. Citation2019). Each 10 μL amplification reaction consisted of 4.1 μL MilliQ water, 5 μL KAPA Taq ReadyMix 2X (Sigma-Aldrich, USA), 0.2 μL forward and reverse primers (0.2 mmol/L), and 0.5 μL of 20 mg/μL extracted DNA.
The PCR runs were carried out on a 2720 Thermal Cycler (Thermo Fisher Scientific, USA) using the following parameters. For the ITS region, the initial denaturing at 94 °C for 5 min, followed by 30 cycles at 94 °C for 30 s, 56 °C for 30 s, and a final extension at 72 °C for 7 min. The samples were held at 4 °C. For 28S, the initial denaturing happens at 94 °C for 5 min, followed by 40 cycles at 94 °C for 45 s, 54 °C for 45 s, and a final extension at 72 °C for 6 min. The samples were also held at 4 °C.
Successful PCRs were visualised using a 1% agarose gel, with ethidium bromide. A sequence PCR reaction was set up with the successful PCRs. Each 10 μL sequence reaction consists of 1 μL amplified DNA, 1.25 μL Buffer, 1 μL BigDye, 1 μL forward primer (0.2 mmol/L) (ITS1 or LR0R, respectively), and 5.75 μL MilliQ water. The sequence reaction was performed on the 2720 Thermal Cycler, with initial denaturing at 96 °C for 1 min, followed by 25 cycles at 96 °C for 10 s. The annealing temperature was set at 50 °C for 10 s and extension step was 60 °C for 4 min. The sequence reaction products were subsequently analysed at the Central Analytical Facility (CAF) using an ABI3730xl sequencer (CAF, Stellenbosch University).
2.3. Phylogenetic analysis
The sequences obtained were trimmed using Chromas 2.6.6 (Technelysium, DNA Sequencing Software, Australia) for quality control, after which they were deposited at the National Center for Biotechnology Information’s (NCBI) database, GenBank. The accession numbers OP458323, OP458324, and OP458325 were assigned to the three sequences representing the ITS data, and OP458326, OP458327, and OP458328 were assigned to the 3 sequences representing the 28S data. Subsequently, the sequences were compared to similar species on the NCBI GenBank database using the nucleotide Nucleotide BLAST algorithm of Citation2022 (Altschul et al. Citation1997). ITS and 28S regions of similar Hericium species as well as Dentipellis leptodon, the designated outgroup, were downloaded. A multiple alignment was performed on the 21 ITS sequences () using Geneious Alignment (Geneious Prime 2021.2.2). The aligned sequences were then trimmed, and ambiguous regions were removed prior to tree construction. The software package PAUP v. 4.0a (Swofford Citation2002) was used to construct the phylogenetic trees. Phylogenetic analysis was performed using a maximum parsimony with a heuristic search. Characters were treated as unweighted with gaps treated as missing data. Maxtrees were set at 500 trees. Branch swapping was done through stepwise addition, using a TBR algorithm, swapping on best trees only. Tree length (TL), consistency index (CI), retention index (RI), rescaled consistency index (RC), and Homoplasy index (HI) were calculated. Confidence was calculated using bootstrap analysis of 1,000 replicates using a full heuristic search. The analysis was run with the model TPM2uf+G selected by AICc with jModeltest 0.1.1 (Guindon and Gascuel Citation2003; Darriba et al. Citation2012). All trees were saved in Treebase (30179). A Bayesian analysis was run using MrBayes v. 3.2.634 (Ronquist et al. Citation2012). The analysis was run with the model K80 + G selected by BIC with jModeltest 0.1.1 (Guindon and Gascuel Citation2003; Darriba et al. Citation2012). The analysis included four parallel runs of 5,000,000 generations, with a sampling frequency of 1,000 generations. The posterior probability values were calculated after the initial 25% of trees were discarded as the chains converged after 118,600 generations.
Table 1. List of ITS sequence accession numbers from GenBank used in the phylogenetic analysis, country of origin is specified as far as known.
3. Results
Blast results of the ITS and 28S sequences confirmed that the fruiting bodies collected in the Knysna – Amatole Afrotemperate forest matched those of the genus Hericium. The ITS sequences showed highest similarity to H. bembedjaense, with a percentage identity of 98.86% (Jumbam et al. Citation2019). The LSU sequences were not used for phylogenetic analysis as the availability of reference sequences for this gene in the genus Hericium is very limited, and this region did not resolve a number of the known species of Hericium and were not used for further analysis. It was, however, interesting to note that the species from Africa clustered separately from other species in Hericium. The dataset for the ITS analysis contained 668 characters of which 535 characters were constant, 59 variable characters were parsimony-uninformative and 74 characters parsimony-informative characters. A total of 52 trees were retained, with tree length = 168, CI = 0.893, RI = 0.938, RC = 0.838, and HI = 0.107. The tree generated from the analysis of the ITS dataset showed that the African strains formed a separate clade, and H. ophelieae sp.nov. and H. bembedjaense grouped in separate clades with high bootstrap and posterior probability values.
3.1. Taxonomy
Hericium ophelieae Van der Merwe and Jacobs sp. nov. ()
Figure 1. Phylogenetic tree inferred from the ITS dataset. Sequences are referred to by their species name and GenBank accession number. Bootstrap values are indicated as the first value on the branch followed by the probability value in italics. T indicates a type strain.
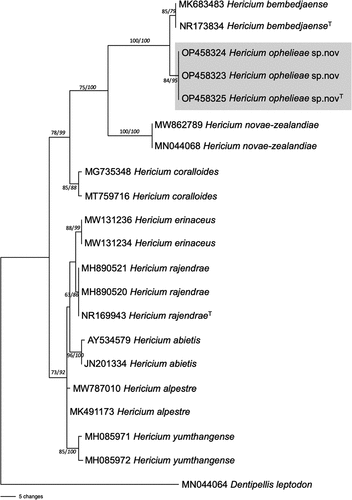
Figure 2. Plates a–e showing the macro features of Hericium ophelieae basidiomes in the field (10 cm to 30 cm in width), at varying stages of development (Photographs: P. Herrmann).
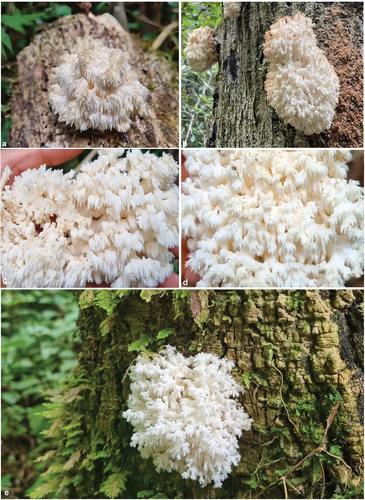
Figure 3. Plates a, b showing two strains of Hericium ophelieae growing on 90 mm malt extract agar Petri Dishes after 14 days of growth at 26 °C. Plate c basidiospores at 100X magnification, enhanced with Congo Red. Plate d basidia, at 100X magnification, enhanced with Congo Red. Scale bars = 10 μm. (Photographs: T. Conradie, B. Van der Merwe).
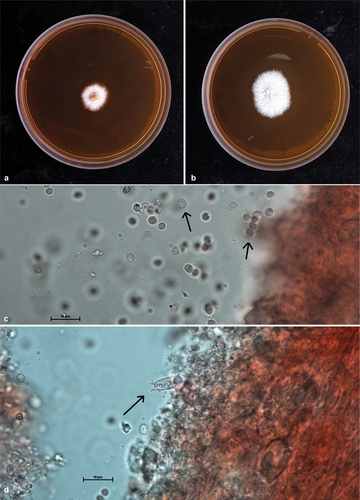
MycoBank: 847,627.
Type: South Africa, Knysna – Amatole Afrotemperate region. Collected from a fallen decomposing Rapanea melanophloeos log, 3 January 2021, B. Van der Merwe. (Holotype: Dried specimen) deposited for long-term storage at the PREM herbarium, PREM 63384. GenBank accession numbers OP458325 (ITS) and OP458328 (28S).
Etymology: Named after the poem Ophélie, by Arthur Rimbaud. Lines such as “long veils … a white phantom … beautiful as snow” seem to be an apt description for the cascading fruiting bodies.
Description: Basidiocarp large (300 mm x 60 mm). Singular – growing from the side of a decaying, standing log. Coral like, soft, and fleshy. Cascading clusters of spines stemming from elongated branches. Spines varying in length (1 mm – 10 mm). Young spines and fruiting body stark white with a pinkish blush, yellowing in age. Basidiospores Length: (2.5 μm – 3.0 μm – 3.5 μm) Width: (2.0 μm – 2.5 μm – 3.0 μm). Smooth, roughly ovaloid in shape. Basidia Length: (15.5 μm – 16.5 μm) Width: (5.0 μm – 7.0 μm). Clavate. Gloeocystidia width (7.5 μm – 9.5 μm). Elongated, irregularly textured.
4. Discussion
The species of Hericium found in the Knysna – Amatole forests was long thought to be H. coralloides (Inaturalist, Citation2022). However, observed differences in morphological and environmental characteristics warranted further study. Fruiting bodies were sequenced in early 2022, and phylogenetic and morphological analysis indicated that H. ophelieae sp. nov. is a previously undescribed species. This is unsurprising, as the genus Hericium contains cryptic species complexes, with species that are often only accurately identified through phylogenetic studies (Hallenberg, Nilsson and Robledo, Citation2013).
Locally, H. ophelieae sp. nov. has been recorded on hardwoods indigenous to the Knysna-Amatole Southern Afrotemperate region of South Africa. Host trees include, but are not necessarily limited to, Rapanea melanophloeos (Cape Beech), Ilex mitis (Cape Holly), Olea capensis (Ironwood), and Ocotea bullata (Stinkwood) trees. Olea capensis is endemic to the Southern Afrotemperate forest region, and the other three host trees (Rapanea melanophloeos, Ilex mitis, and Ocotea bullata) are indigenous to Southern Africa (Van Wyk and Van Wyk Citation1997). H. ophelieae sp. nov. forms fruiting bodies from December to March, coinciding with the rainy season of this region. The formation of fruiting bodies seems to be coupled to heavy rains, humidity, and warmer temperatures (Herrmann, Pers. Comm.).
H. ophelieae sp. nov. shares very similar macro-characteristics with its closest relative, H. bembedjaense and other members of the genus Hericium. However, apart from the differences in phylogeny, H. ophelieae sp. nov. has noticeably larger spores than H. bembedjaense (3.0 μm – 2.5 μm vs 2.8 μm – 2.0 μm), as well as wider gloeocystidia (7.5 μm/9.5 μm vs 3.4 μm /6.7 μm). It also appears that H. ophelieae sp. nov. differs from other Hericium species in substrate choice, as they are growing on hardwoods indigenous to Southern Africa. This is compared to H. bembedjaense that grows on Gilbertiodendron dewevrei and H. coralloides that prefers Fagaceae species (Jumbam et al. Citation2019). To date, no other Hericium species have been found in this region or in South Africa (Kinge et al. Citation2020).
H. ophelieae sp. nov. is the first Hericium to be described from Southern Africa, however it is likely not the only species to be found here. Many of the biomes in South Africa are known hotspots for plant diversity, and high plant diversity has been shown to increase fungal diversity (Gao et al. Citation2013; Shen et al. Citation2021; Wang et al. Citation2022). Many novel species could remain undescribed due to the slow development of mycology in South Africa, and Africa as a whole (Crous et al. Citation2006). As a result, amateur mycologists are often involved in the discovery of new species. Accumulated citizen science projects could provide value to mycology in Africa, like it has in other continents (e.g. Heilmann-Clausen et al. Citation2019).
This discovery will hopefully prompt more studies in the region and will further our knowledge on local biodiversity and microbial – environmental interactions. Reports like these can also have far reaching impacts as Hericium members are well known for their production of secondary metabolites that are at the centre of a fast-growing drug discovery field. Further studies into the medicinal properties of Hericium ophelieae sp. nov. will hopefully elucidate the potential applications of this novel, medically relevant fungus.
Acknowledgements
We are grateful to Ms Elize Le Roux for the specimens she collected for this study. The assistance and support of the Jacobs Lab is also noted, with mention to Mr Aiden Visagie for his contributions. This work would not be possible without Stellenbosch University and the Department of Microbiology at Stellenbosch University. We are also thankful for the comments of the two anonymous reviewers whose valuable comments assisted us to improve on the initial manuscript.
Disclosure statement
No potential conflict of interest was reported by the authors.
Correction Statement
This article has been corrected with minor changes. These changes do not impact the academic content of the article.
References
- Altschul SF, Madden TL, Schäffer AA, Zhang J, Zhang Z, Miller W, Lipman DJ. 1997. Gapped BLAST and PSI-BLAST: a new generation of protein database search programs. Nucleic Acids Res. 25((17):):3389–3402. doi:10.1093/nar/25.17.3389.
- Boddy L, Crockatt ME, Ainsworth AM. 2011. Ecology of Hericium cirrhatum, H. coralloides and H. erinaceus in the UK. Fungal Ecol. 4(2):163–173. doi:10.1016/j.funeco.2010.10.001.
- Chen ZG, Bishop KS, Tanambell H, Buchanan P, Smith C, Quek SY. 2019. Characterization of the bioactivities of an ethanol extract and some of its constituents from the New Zealand native mushroom: Hericium novae-zealandiae. Food Funct. 10(10):6633–6643.
- Chong PS, Fung ML, Wong KH, Lim LW. 2020. Therapeutic potential of Hericium erinaceus for depressive disorder. Int J Mol Sci. 21(1):163.
- Crous PW, Rong IH, Wood A, Lee S, Glen H, Botha W, Slippers B, de Beer WZ, Wingfield MJ, Hawksworth DL. 2006. How many species of fungi are there at the tip of Africa? Stud Mycol. 55:13–33.
- Darriba D, Taboada GL, Doallo R, Posada D. 2012. JModelTest 2: more models, new heuristics and parallel computing. Nat Methods. 9(8):772.
- Das K, Stalpers J, Eberhardt U. 2011. A new species of Hericium from Sikkim Himalaya (India). Cryptogam Mycol. 32(3):285–293.
- Gao C, Shi NN, Liu YX, Peay KG, Zheng Y, Ding Q, Mi XC, Ma KP, Wubet T, Buscot F, et al. 2013. Host plant genus-level diversity is the best predictor of ectomycorrhizal fungal diversity in a Chinese subtropical forest. Mol Ecol. 22(12):3403–3414.
- Ghosh S, Nandi S, Banerjee A, Sarkar S, Chakraborty N, Acharya K. 2021. Prospecting medicinal properties of Lion’s mane mushroom. J Food Biochem. 45(8):e13833.
- Guindon S, Gascuel O. 2003. A simple, fast, and accurate algorithm to estimate large phylogenies by Maximum Likelihood. Syst Biol. 52(5):696–704.
- Hallenberg N, Nilsson RH, Robledo G. 2013. Species complexes in Hericium (Russulales, Agaricomycota) and a new species - Hericium rajchenbergii - from Southern South America. Mycol Prog. 12(2):413–420.
- Heilmann-Clausen J, Bruun HH, Ejrnæs R, Frøslev TG, Læssøe T, Petersen JH. 2019. How citizen science boosted primary knowledge on fungal biodiversity in Denmark. Biol Conserv. 237:366–372.
- Inaturalist. 2008–2023. Accessed 2022 Nov 15. https://www.inaturalist.org/observations?place_id=6986&subview=map&taxon_id=49160
- Index Fungorum. 2023. Index Fungorum Partnership: UK; [Accessed 16 Feb]. https://www.indexfungorum.org/
- Jumbam B, Haelewaters D, Koch RA, Dentinger BTM, Henkel TW, Aime MC. 2019. A new and unusual species of Hericium (Basidiomycota: Russulales, Hericiaceae) from the Dja Biosphere Reserve, Cameroon. Mycol Prog. 18(10):1253–1262.
- Kinge TR, Goldman G, Jacobs A, Ndiritu GG, Gryzenhout M. 2020. A first checklist of macrofungi for South Africa. MycoKeys. 63:1–48.
- Ma BJ, Shen JW, Yu HY, Ruan Y, Wu TT, Zhao X. 2010. Hericenones and erinacines: stimulators of nerve growth factor (NGF) biosynthesis in Hericium erinaceus. Mycology. 1(2):92–98.
- Moncalvo JM, Lutzoni FM, Rehner SA, Johnson J, Vilgalys R. 2000. Phylogenetic relationships of agaric fungi based on nuclear large subunit ribosomal DNA sequences. Syst Biol. 49(2):278–305.
- Mucina L, Rutherford MC 2006. The vegetation of South Africa, Lesotho and Swaziland.South African National Biodiversity Institute: Pretoria, South Africa. Memoirs of the Botanical Survey of South Africa, Strelitzia 19.
- Ouali Z, Sbissi I, Boudagga S, Rhaiem A, Hamdi C, Venturella G, Saporita P, Jaouani A, Gargano ML. 2020. First report of the rare tooth fungus Hericium erinaceus in North African temperate forests. Plant Biosyst. 154(1):24–28.
- Pallua JD, Recheis W, Pöder R, Pfaller K, Pezzei C, Hahn H, Huck-Pezzei V, Bittner LK, Schaefer G, Steiner E, et al. 2012. Morphological and tissue characterization of the medicinal fungus Hericium coralloides by a structural and molecular imaging platform. Analyst. 137(7):1584–1595.
- Park HG, Ko HG, Kim SH, Park WM. 2004. Molecular identification of Asian isolates of medicinal mushroom Hericium erinaceum by phylogenetic analysis of nuclear ITS rDNA. J Microbiol Biotechnol. 14(4):816–821.
- Persoon CH. 1794. Neuer Versuch einer systematischen Einteilung der Schwämme. Römer Neues Mag Bot. 1:63–128.
- Ronquist F, Teslenko M, van der Mark P, Ayres DL, Darling A, Höhna S, Larget B, Liu L, Suchard MA, Huelsenbeck JP. 2012. Mrbayes 3.2: efficient Bayesian phylogenetic inference and model choice across a large model space. Syst Biol. 61(3):539–542.
- Shen C, Wang J, He JZ, Yu FH, Ge Y. 2021. Plant diversity enhances soil fungal diversity and microbial resistance to plant invasion. Appl Environ Microbiol. 87(11):e00251–21.
- Singh U, Das K. 2019. Hericium rajendrae sp. nov. (Hericiaceae, Russulales): an edible mushroom from Indian Himalaya. Nova Hedwigia. 108(3–4):505–515.
- Swofford DL 2002. PAUP*. Phylogenetic Analysis Using Parsimony (*and other methods). Version 4. Sinauer Associates, Sunderland (Massachusetts).
- Vilgalys R, Hester M. 1990. Rapid genetic identification and mapping of enzymatically amplified ribosomal DNA from several Cryptococcus species. J Bacteriol. 172(8):4238–4246.
- Wang C, Ma L, Zuo X, Ye X, Wang R, Huang Z, Liu G, Cornelissen JHC. 2022. Plant diversity has stronger linkage with soil fungal diversity than with bacterial diversity across grasslands of northern China. Global Ecol Biogeography. 31(5):886–900.
- White TJ, Bruns T, Lee S, Taylor J. 1990. Amplification and direct sequencing of fungal ribosomal RNA genes for phylogenetics. PCR Protocol. 18(1):315–322.
- Wittstein K, Rascher M, Rupcic Z, Löwen E, Winter B, Köster RW, Stadler M. 2016. Corallocins A-C, Nerve growth and brain-derived neurotrophic factor inducing metabolites from the mushroom Hericium coralloides. J Nat Prod. 79(9):315–322.
- Wyk BV. 1997. Field guide to trees of Southern Africa. South Africa: Struik Publishers (Pty) Ltd.
- Zhang J, Zhang J, L Zhao, X Shui, LA Wang, U Wu. 2019. Antioxidant and anti-aging activities of ethyl acetate extract of the coral tooth mushroom, Hericium coralloides (Agaricomycetes). Int J Med Mushrooms. 21(6):561–570.