ABSTRACT
Phyllosticta (Phyllostictaceae, Botryosphaeriales) species are widely distributed globally and constitute a diverse group of pathogenic and endophytic fungi associated with a broad range of plant hosts. In this study, four new species of Phyllosticta, i.e. P. endophytica, P. jiangxiensis, P. machili, and P. xinyuensis, were described using morphological characteristics and multi-locus phylogeny based on the internal transcribed spacer region (ITS) with intervening 5.8S rRNA gene, large subunit of rRNA gene (nrLSU), translation elongation factor 1-alpha gene (tef1), actin gene (act), and glyceraldehyde-3-phosphate dehydrogenase gene (gapdh). Phyllosticta machili is the first species of this genus reported to infect plants of the Machilus genus.
KEYWORDS:
1. Introduction
The genus Phyllosticta (Phyllostictaceae, Botryosphaeriales, and Ascomycota) was established by Persoon (Persoon Citation1818). Presently, a total of 3,215 names are documented for Phyllosticta in the Index Fungorum (accessed on 29 March 2023). However, many of these names have been synonymised. Currently, 1,499 species are recognised in this genus (Bánki et al. Citation2022). Most members of Phyllosticta are important plant pathogens and endophytes with a wide host range and geographical distribution (Van der Aa and Vanev Citation2002). Some Phyllosticta species cause significant impacts on economic crops, such as citrus black spot caused by P. citricarpa (McAlpine) Aa, which is regarded as a quarantine pathogen in Europe and the USA (Baayen et al. Citation2002; Wulandari et al. Citation2009; Glienke et al. Citation2011), black rot disease on grapevines by P. ampelicida (Engelm.) Aa species complex (Wicht et al. Citation2012), and banana freckle disease by P. musarum (Cooke) Aa species complex (Pu et al. Citation2008; Wong et al. Citation2012). In addition, some species of Phyllosticta have also been isolated from a wide range of hosts as endophytic fungi. For example, P. capitalensis Henn. is an endophytic fungus distributed in more than 20 different families of plants in eight countries (Baayen et al. Citation2002; Wulandari et al. Citation2010; Glienke et al. Citation2011). However, endophytes can sometimes be transformed into pathogens, such as P. capitalensis, which can cause black freckle disease on Rubus chingii HU and leaf spots on Ligustrum japonicum Thunb. as a pathogen, thus affecting the visual value of ornamental plants (Sabahi et al. Citation2022; Zhang et al. Citation2022). Some species of Phyllosticta can survive by degrading organic material from dead plants as saprobes, such as P. carpogena (Shear) Aa and P. ericae Allesch (Trigiano et al. Citation2004) occurring on Rubus sp. (Rosaceae) and Erica carnea (Ericaceae), respectively (Van der Aa and Vanev Citation2002).
In China, some species of Phyllosticta can infect economic plants and cause economic losses. For example, P. theicola Curzi and P. capitalensis can both cause leaf spots on Camellia sinensis (L.) O. Ktze. (Cheng et al. Citation2019; Tian et al. Citation2019). Phyllosticta capitalensis can cause leaf spots on oil palms (Nasehi et al. Citation2020), and P. citricarpa can cause black spot disease on citrus leading to black spots on fruit and premature fruit drop (Wang et al. Citation2012; Tran et al. Citation2020).
The aim of this study was to identify Phyllosticta strains isolated from different host plants in China by morphological characterisation and multi-gene phylogenetic analysis.
2. Material and methods
2.1. Isolation and culture
Diseased leaf tissues and healthy tissues from different hosts were collected from China’s Anhui, Hubei, Yunnan, and Jiangxi provinces. Fragments (5 mm × 5 mm) were taken from the margin of leaf lesions and healthy parts of leaves. All fragments were disinfected in 75% ethanol for 30 s, followed by 10% sodium hypochlorite for 3 min, washed in sterile water three times, then placed on potato dextrose agar (PDA), and cultured at room temperature (25 ± 3) °C. Cultures were dried after sporulation and sent as holotype to Chinese Academy of Forestry (CAF; http://museum.caf.ac.cn), and ex-type living cultures of new species were deposited in the China Forestry Culture Collection Center (CFCC; http://cfcc.caf.ac.cn/) and Capital Normal University Culture Collection Center (CNUCC).
2.2. Morphological analysis
Isolates were cultured for 12–15 days and examined periodically until sporulation. The pycnidia and conidia on cultures were examined with a dissecting microscope (Nikon SMZ-1000) and an upright microscope (Olympus BX51). Mature pycnidia were immersed and crushed in water, releasing both conidia and conidiogenous cells on glass slides. Twenty of each structure, including pycnidia, conidiogenous cells, and conidia, were measured to calculate the average size as described. Colony colours were described based on the ColorHexa code (https://www.colorhexa.com/).
2.3. Phylogenetic analysis
Isolates were cultured on PDA for seven days at room temperature. Mycelia were collected with a sterile inoculation shovel, and genomic DNA was extracted using the M5 Plant Genomic DNA Kit (Mei5 Biotechnology Co., Ltd., China) following the manufacturer’s instructions. Five loci, including ITS, act, nrLSU, gapdh, and tef1 (), were amplified and sequenced using primers pairs ITS-1F (Gardes and Bruns Citation1993) and ITS-4 (White et al. Citation1990), ACT-512-F and ACT-783-R (Carbone and Kohn Citation1999), LR0R (Moncalvo et al. Citation1995) and LR5 (Vilgalys and Hester Citation1990), Gpd1-LM and Gpd2-LM (Myllys et al. Citation2002), and EF1-728F and EF2 (Carbone and Kohn Citation1999), respectively (). The 25 μL reaction volume consisted of 12.5 μL Master Mix (Mei5 Biotechnology, Co., Ltd., Beijing, China), 1 μL of each forward and reverse primer, 1 μL template genomic DNA (about 10 ng/μL), and 8.5 μL distilled deionised water. PCR products were visually inspected in agarose electrophoresis gels and compared with band intensities of 200 bp DNA ladders.
Table 1. Genes, PCR primers, procedures, and references used in this study.
Purification and sequencing were performed by Zhongkexilin Biotechnology Company (Beijing, China). DNA sequences using forward and reverse primers were aligned using Editseq v5.0 to obtain consensus sequences. The new sequences in this study were submitted to the GenBank. Other sequences of the ITS, nrLSU, tef1, act, and gapdh genes were downloaded from GenBank (). The segmentation homogeneity test was performed to determine the consistency of gene segments (Farris et al. Citation1994; Huelsenbeck et al. Citation2003). Aligned using the online MAFFT tool (https://www.ebi.ac.uk/Tools/msa/mafft/) and edited using Gblocks (https://www.phylogeny.fr/one_task.cgi?task_type = gblocks) by selecting DNA and all options for less stringent criteria.
Table 2. Isolates and GenBank accession numbers used in the phylogenetic analyses of Phyllosticta.
The phylogenetic analyses were based on a combined ITS, nrLSU, act, tef1, and gapdh matrix, including the type and representative species of Phyllosticta (Norphanphoun et al. Citation2020). A maximum parsimony (MP) tree was constructed with PAUP v4.0b10 (Swofford Citation2003). Trees were inferred using the heuristic search option with TBR branch swapping and 1,000 random sequence additions. Branches of zero length were collapsed and all equally most parsimonious trees were saved. Tree length (TL), consistency index (CI), retention index (RI), and rescaled consistency index (RC) were calculated for the generated parsimony trees. For the Bayesian inference (BI) analysis, MrModeltest v2.3 with the Akaike information criterion was used as a substitution model for the concatenated dataset (Nylander Citation2004). The GTR+I+G model was proposed for ITS, nrLSU, and gapdh, HKY+I+G for act, and GTR+G for tef1. 1,000,000 generations were launched with a random starting tree, and Markov chains were sampled every 100 generations. The analysis was stopped when the average standard deviation of the separation frequency was 0.01. During the burn-in phase of the analysis, the first 25% of trees were discarded, and the remaining trees were used to calculate the posterior probabilities (PPs).
3. Results
3.1. Phylogenetic analysis
Single-gene phylogenetic trees were constructed before the multi-gene phylogenetic analysis to examine topology and clade support based on the ITS, nrLSU, act, tef1, and gapdh genes (Supplementary Figures 1, 2, 3, 4, and 5). The topology of the single-gene phylogenetic tree for ITS, act, and tef1 was similar to the multi-gene phylogenetic tree, but the support values of the deep nodes were lower. The topology of the single-gene phylogenetic tree of nrLSU and gapdh differed slightly from the multi-gene phylogenetic tree.
In the multi-locus phylogenetic analysis, 311 sequences of 81 isolates were used to construct a five-locus phylogenetic tree; Botryosphaeria obtusa (Schwein.) Shoemaker (CMW 8232) was used as the outgroup. The dataset of five loci comprised 2,470 characters, including the alignment gaps, of which 623 characters were parsimony-informative, 305 parsimony-uninformative, and 1,542 constants. The analytical analysis yielded 1,000 similar trees, one of which (TL = 3,034, CI = 0.467, RI = 0.727, RC = 0.340, and HI = 0.533) is shown in . The Bayesian tree confirmed the topology of the obtained tree using the maximum parsing method.
Figure 1. MP phylogenetic tree inferred from combined ITS, act, nrLSU, gapdh, and tef1 sequence data from 81 strains of the genus Phyllosticta and Botryosphaeria obtusa CMW 8232 as an outgroup. MP bootstrap values above 70% and Bayesian PPs above 0.90 are given at the nodes in this order. Strain names and sequences of new species in this study are indicated in bold. Isolates marked with “*” are ex-type or ex-epitype strains.
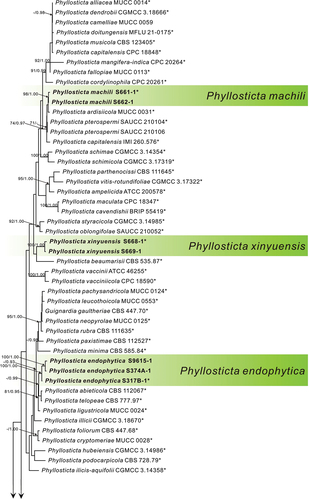
In phylogenetic analysis, three strains of P. endophytica formed a sister clade (MPB = 100, PP = 1.00) with P. abieticola Wikee & Crous, P. telopeae H.Y. Yip, and P. ligustricola Motohashi & C. Nakash. Three strains of P. jiangxiensis formed an independent clade with high support (MPB = 100, PP = 1.00). Two strains of P. machili clustered with Phyllosticta ardisiicola Motohashi, I. Araki & C. Nakash., P. pterospermi Z.X. Zhang, X.Y. Liu, Z. Meng & X.G. Zhang, and P. capitalensis with high support (MPB = 98, PP = 1.00). Two strains of P. xinyuensis clustered with P. beaumarisii with high support (MPB = 100, PP = 1.00).
3.2. Taxonomy
Based on the phylogenetic analysis and morphological comparisons, 10 strains were attributed to four new species of Phyllosticta.
Phyllosticta endophytica X.N. Sui & C.L. Hou, sp. nov.
Figure 2. Phyllosticta endophytica (holotype, CAF 8000201). a: Colonies (left-above, right-reverse) after 7 days on PDA; b: Conidiomata; c: Conidiogenous cells; d: Conidia; e: Spermatogenous cells producing spermatia; f: Spermatia. Scale bars: 200 μm (b); 10 μm (c–f).
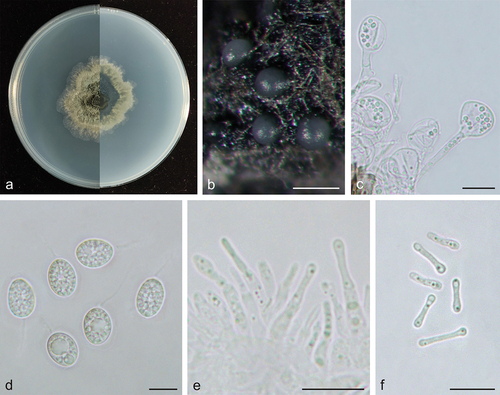
MycoBank: 848949.
Etymology: endophytica, referring to its lifestyle, endophytic.
Type: China, Yunnan Province: Daguan County Hekou Village, on healthy leaves of Cunninghamia lanceolata (Lamb.) Hook., 13 April 2019, Q.T. Wang & C.L. Hou (holotype, CAF 8000201, ex-holotype living culture CNUCC S317-1 = CFCC 59082).
Description: Conidiophores are cylindrical to ampulliform, often reduced to conidiogenous cells. Conidiogenous cells 14.5–28.0 × 2.0–5.0 μm, hyaline, cylindrical. Conidia 12.5–16.0 × 9.0–11.0 μm, mean ± SD = 14.1 ± 0.8 × 10.3 ± 0.6 μm, hyaline, aseptate, ovoid, ellipsoidal to subglobose, surrounded by a thin mucoid sheath, bearing a hyaline, apical mucoid appendages, 7.0–15.0 μm, unbranched, and flexible. Spermatiogenous cells are subcylindrical to ampulliform, 7.0–12.0 × 2.0–3.0 μm. Spermatia aseptate, dumbbell-shaped, 6.0–11.0 × 1.0–2.0 μm, mean ± SD = 8.5 ± 1.4 × 1.5 ± 0.2 μm.
Culture characteristics: Colonies up to 4.3 cm in diameter at 7 days on PDA. Irregular at the edge, dark desaturated green (#273517) to greyish-white (#e0e0e0) on the obverse, and dark lime green (#002700) to greyish-white (#e0e0e0) on the reverse side. Conidiomata are visible after 15 days with dark grey oozing (#a3a3a3) tendrils.
Additional specimens examined: China, Hubei Province: Xiangjia Mountain Villa Tea plantation, on healthy leaves of Cunninghamia lanceolata, 6 May 2019, Q.T. Wang & C.L. Hou, living culture S374A-1. China, Anhui Province: Shucheng Hepeng Camellia Nursery tea plantation, on healthy leaves of Cunninghamia lanceolata, 17 February 2017, Q.T. Wang & C.L. Hou, living culture S9615-1.
Notes: In the phylogenetic analysis, the three strains of P. endophytica clustered together with high support values (MPB = 100, PP = 1.00, ) and formed a sister clade with P. abieticola, P. ligustricola, and P. telopeae. However, P. endophytica (S317-1) differs from P. abieticola by 28 nucleotides (18/570 in ITS, 5/211 in act, and 5/861 in nrLSU), from P. ligustricola by 37 nucleotides (27/648 in ITS and 10/210 in act) and from P. telopeae by 43 nucleotides (10/570 in ITS, 8/211 in act, 22/210 in tef1, 2/623 in gapdh, and 1/882 in nrLSU). Morphologically, the conidia of P. endophytica (9.0–11.0 μm) are wider than those of P. abieticola (7.0–8.0 μm) (Wikee et al. Citation2013b), P. ligustricola (4.9–7.4 μm) (Motohashi et al. Citation2008b), and P. telopeae (8.0–9.5 μm) (Yip Citation1989). Furthermore, the apical appendages of P. endophytica (7.0–15.0 μm) are shorter than those of P. abieticola (20.0–25.0 μm) (Wikee et al. Citation2013b) and P. telopeae (20.0–26.0 μm) (Yip Citation1989), and longer than those of P. ligustricola (4.9–9.8 μm) (Motohashi et al. Citation2008b). Phyllosticta endophytica has the same host as P. cryptomeriae Kawam., Phyllosticta cunninghamii Allesch., and P. concentrica Sacc. P. cunninghamii does not have molecular data (Brittingham et al. Citation1978). The conidia of P. endophytica (12.5–16.0 × 9.0–11.0 μm) are longer than those of P. cryptomeriae (9 − 13.5 μm) (Petrini et al. Citation1991) and P. cunninghamii (2.0–3.0 μm) (Sydow Citation1897) and wider than those of P. cunninghamii (1.0 μm) (Sydow Citation1897) and P. concentrica (6.0–9.0 μm) (Wikee et al. Citation2013a). Therefore, Phyllosticta endophytica is proposed as a new species.
Phyllosticta jiangxiensis X.N. Sui & C.L. Hou, sp. nov.
Figure 3. Phyllosticta jiangxiensis (holotype, CAF 8000202). a: Leaf lesions on living leaf of Camellia oleifera; b: Colonies (left-above, right-reverse) after 7 days on PDA; c: Conidiomata; d, e: Conidiogenous cells with conidia; f: Conidia. Scale bars: 200 μm (c); 10 μm (d–f).
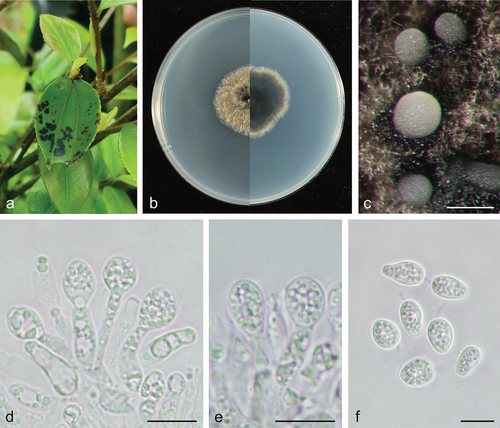
MycoBank: 848950.
Etymology: jiangxiensis, derived from the province name of the type locality of this species, Jiangxi Province.
Type: China, Jiangxi Province: Changbu Forest Plantation, on diseased leaves of Camellia oleifera, 22 April 2021, Q.T. Wang & C.L. Hou (holotype CAF 8000202, ex-holotype living culture CNUCC S686-1 = CFCC 59083).
Description: Leaf spots irregularly circular, black (#000000), dark red (#270000) on the outer edge. Conidiomata not observed on leaves. Conidiophores are cylindrical to ampulliform, often reduced to conidiogenous cells. Conidiogenous cells 7.5–15.0 × 2.0–5.5 μm, hyaline, and cylindrical. Conidia 8.0–12.0 × 6.0–8.0 μm, mean ± SD = 9.7 ± 0.9 × 7.1 ± 0.4 μm, hyaline, aseptate, ovoid, ellipsoidal to subglobose, and surrounded by a thick mucoidsheath. Some conidia have thin and long apical appendages 4.0–14.0 μm.
Culture characteristics: Colonies up to 4.3 cm in diameter at 7 days on PDA. Round with ciliate at the edge, dark lime green (#1f2820) to dark yellow (#8e7f00) on the obverse, greyish-white (#ececec) on the outer edge, and dark lime green (#171c1a) to light grey (#ececec) on the reverse side. Conidiomata are visible after 14 days with pale yellow (mostly white) (#ffffec) oozing on PDA.
Additional specimens examined: China, Jiangxi Province: Changbu Forest Plantation, on diseased leaves of Camellia oleifera, 22 April 2021, Q.T. Wang & C.L. Hou, living culture S687-1. China, Jiangxi Province: Changbu Forest Plantation, on diseased leaves of Camellia oleifera, 22 April 2021, Q.T. Wang & C.L. Hou, living culture S688-1.
Notes: In the phylogenetic analysis, the sequences of S686-1, S687-1, and S688-1 formed an independent clade with high support values in the multi-gene phylogenetic tree (MPB = 100, PP = 1.00, ). S686-1 was isolated from the leaves of Camellia oleifera, one of China’s four major woody oil plants. Phyllosticta camelliae Westend., P. camelliaecola Brunaud, P. capitalensis, P. erratica Ellis & Everh., P. theae Speschnew, and P. theacearum Aa have been reported on Camellia oleifera. Among these, only P. capitalensis and P. camelliae (Motohashi et al. Citation2009) have molecular data but are distantly related to P. jiangxiensis in the phylogenetic tree. Other species without molecular data can be distinguished by morphological characters. For example, P. jiangxiensis produces bigger conidia (8.0–12.0 × 6.0–8.0 μm) than P. camelliaecola (5.0–6.0 × 2.0–3.0 μm) (Brunaud Citation1888), P. theae (6.0–8.0 × 1.5–2.0 μm) (Speschnew Citation1904), and longer conidia than P. erratica (6.0–8.0 μm) (Ellis and Everhart Citation1900). Furthermore, P. jiangxiensis have longer conidiogenous cells (7.5–15.0 × 2.0–5.5 μm) than P. theacearum (4.0–6.0 × 2.0–2.5 µm) (Van der Aa Ha Citation1973), P. capitalensis (7.0–10.0 × 3.0–5.0 μm) (Glienke et al. Citation2011). Therefore, Phyllosticta jiangxiensis is proposed as a new species.
Phyllosticta machili X.N. Sui & C.L. Hou, sp. nov.
Figure 4. Phyllosticta machili (holotype, CAF 8000203). a: Colonies (left-above, right-reverse) after 7 days on PDA; b: Conidiomata; c, d: Conidiogenous cells with conidia; e: Conidia. Scale bars: 200 μm (b); 10 μm (c–e).
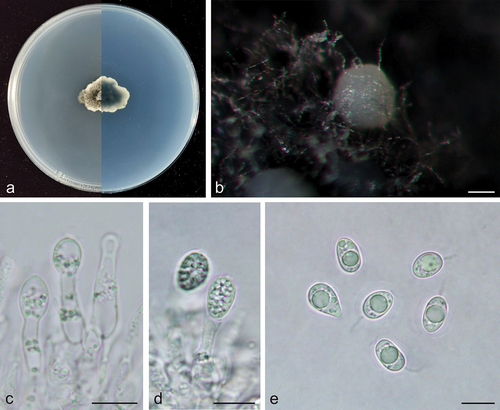
MycoBank: 848951.
Etymology: machili, from the genus name of the host plant, Machilus.
Type: China, Jiangxi Province: Xinyu Fir Plantation, on diseased leaves of Machilus pauhoi, 22 April 2021, Q.T. Wang & C.L. Hou (holotype CAF 8000203, ex-holotype living culture CNUCC S661-1 = CFCC 59084).
Description: Conidiogenous cells 12.0–22.0 × 2.5–4 μm, hyaline, and cylindrical. Conidia 9.0–13.5 × 6.5–9.0 μm, mean ± SD = 11.0 ± 1.1 × 8.0 ± 0.7 μm, hyaline, aseptate, ovoid, ellipsoidal to subglobose, containing a single large central guttule, and surrounded by a thick mucoid sheath. Some conidia have apical appendages 3.0–7.0 μm.
Culture characteristics: Colonies up to 2.2 cm in diameter at 7 days on PDA. The entire edge is irregular, dark greyish tallow colour (#4a4a36) with aerial hyphae to greyish-white (#e0e0e0) on the obverse, dark greyish lime green (#2a392a) on the outer edge, and dark greyish lime green (#2a392a) to greyish-white (#e0e0e0) on the reverse side. Conidiomata are light grey (#c9c9c9) and visible after 14 days.
Additional specimens examined: China, Jiangxi Province: Xinyu Fir Plantation, on diseased leaves of Machilus pauhoi, 22 April 2021, Q.T. Wang & C.L. Hou, living culture S662-1.
Notes: In the phylogenetic analysis, two strains of P. machili clustered in a single clade with high support values (MPB = 98, PP = 1.00, ), and P. machili was closely related to P. ardisiicola, P. capitalensis, and P. pterospermi (MPB = 74, PP = 0.97, ). However, P. machili (S661-1) differs from P. capitalensis by 29 nucleotides (13/565 in ITS, 13/232 in act, 3/214 tef1, and 9/639 in gapdh), from P. pterospermi by 38 nucleotides (14/617 in ITS, 12/250 in act, 3/380 in tef1, 3/835 in gapdh, and 3/882 in nrLSU), and from P. ardisiicola by 34 nucleotides (23/625 in ITS and 11/214 in act). Morphologically, P. machili can be distinguished by producing longer conidiogenous cells (12.0–22.0 × 2.5–4 μm) than P. pterospermi (7.5–11.0 × 2.5–4.5 μm) (Zhang et al. Citation2022), P. capitalensis (7.0–10.0 × 3.0–5.0 μm) (Glienke et al. Citation2011), and P. ardisiicola (5–12.5 × 1.2–2.5 μm) (Motohashi et al. Citation2008b). Phyllosticta plumbaginicola V.G. Rao (8–13 μm) (Nag Raj Citation1993) does not have molecular data but has similar morphological characters to P. machili; however, P. machili has shorter apical appendages (3–7 μm). Combined with phylogenetic analysis and morphological comparisons, this fungus was identified as a new species. Phyllosticta machili is the first Phyllosticta species reported from Machilus.
Phyllosticta xinyuensis X.N. Sui & C.L. Hou, sp. nov.
Figure 5. Phyllosticta xinyuensis (holotype, CAF 8000204). a: Leaf lesions on living leaf of Camellia oleifera; b: Colonies (left-above, right-reverse) after 7 days on PDA; c: Conidiomata; d, e: Conidiogenous cells with conidia; f: Conidia. Scale bars: 200 μm (c); 10 μm (d–f).
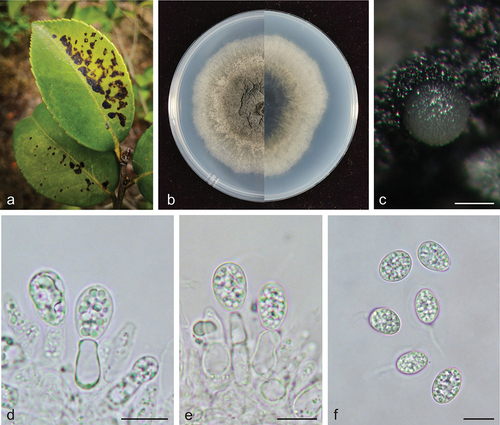
MycoBank: 848952.
Etymology: xinyuensis, derived from the type locality of this species, Xinyu City.
Type: China, Jiangxi Province: Changbu Forest Plantation, on diseased leaves of Camellia oleifera, 22 April 2021, Q.T. Wang & C.L. Hou (holotype, CAF 8000204, ex-holotype living culture CNUCC S668-1 = CFCC 59085).
Description: Leaf spots irregularly circular, black (#000000), dark red (#270000) on the outer edge. Conidiomata not observed on leaves. Conidiophores are cylindrical to ampulliform, often reduced to conidiogenous cells. Conidiogenous cells 9.0–16.0 × 3.0–5.0 μm, hyaline, and cylindrical. Conidia 10.0–14.0 × 7.0–10.0 μm, mean ± SD = 12.2 ± 0.9 × 8.0 ± 0.7 μm, hyaline, aseptate, ovoid, ellipsoidal to subglobose, and surrounded by a thick mucoid sheath. Some conidia have thin and long apical appendages 3.0–7.0 μm.
Culture characteristics: Colonies up to 6.9 cm in diameter at 7 days on PDA. Round with a scalloped margin, dark greyish-green centre (#5a6053) to greyish-green edges (#808976) on the obverse and reverse sides. Formation of olive green to green lamellae as black masses. Conidiomata are visible after 12 days with light grey (mostly white) (#f8f8f8) tendrils on PDA.
Additional specimens examined: China, Jiangxi Province: Changbu Forest Plantation, on diseased leaves of Camellia oleifera, 22 April 2021, Q.T. Wang & C.L. Hou, living culture S669-1.
Notes: In the phylogenetic analysis, the two strains of P. xinyuensis clustered together in a clade with high support values (MPB = 100, PP = 1.00, ) and grouped with P. beaumarisii A.P. Paul & M.D. Blackburn. Morphologically, P. xinyuensis produces wider conidia (10.0–14.0 × 7.0–10.0 μm) than P. beaumarisii (7.5–15 × 6.5–8.75 μm) (Paul and Blackburn Citation1986). Phyllsoticta camelliae, P. camelliaecola, P. capitalensis, P. erratica, P. theae, and P. theacearum have the same host as P. xinyuensis. Among these, only P. capitalensis and P. camelliae (Motohashi et al. Citation2009) have molecular data but are distantly related to P. xinyuensis in the phylogenetic tree. Other species without molecular data can be distinguished by morphological characters. For example, P. xinyuensis produces wider conidia (10.0–14.0 × 7.0–10.0 μm) than P. camelliaecola (5.0–6.0 × 2.0–3.0 μm) (Brunaud Citation1888), P. theae (6.0–8.0 × 1.5–2.0 μm) (Speschnew Citation1904), P. theacearum (8.0–12.5 × 5.5–7.0 μm) (Van der Aa Ha Citation1973), P. capitalensis (11.0–12.0 × 6.0–7.0 µm) (Glienke et al. Citation2011) and longer conidia than P. erratica (6.0–8.0 μm long) (Ellis and Everhart Citation1900). Therefore, Phyllosticta xinyuensis is introduced as a novel species.
4. Discussions
The initial identification of Phyllosticta species was mainly based on morphology, culture characteristics, and host associations. Van der Aa and Vanev (Citation2002) revised more than 2,000 species in Phyllosticta and suggested more accurate and reliable morphological characteristics for its classification. However, the Phyllosticta species share many morphological similarities, making it difficult to classify them accurately using morphology alone. Hyde et al. (Citation2010) proposed a connection between molecular data and the correct taxonomic unit using morphological characters in combination with molecular data to accurately identify fungi. Wikee et al. (Citation2012) placed Phyllosticta within Phyllostictaceae using molecular phylogenetic analysis based on the ITS, nrLSU, act, tef1, and gapdh genes, combined with morphological characters.
In this study, four new species were described based on morphology and phylogenetic analysis. Single-gene phylogenetic trees for each of the five genes were also constructed separately. However, the multi-locus phylogenetic tree provided better resolution for the Phyllosticta species. Including protein-coding genes in the analysis greatly facilitated species-level identification, also demonstrated by Wikee et al. (Citation2011). Multi-locus phylogeny and three signal locus (ITS, tef1, and act) supported the four new species. The difference between the topology of the single-gene phylogenetic trees of nrLSU and gapdh with that of the multi-locus phylogenetic tree may be due to fewer available sequences (only 32 linked data for gapdh in 71 recognised species, and 34 linked data for gapdh in 71 recognised species). Among the phylogenetic trees for a single locus, the gapdh gene tree showed the greatest difference in topological structure compared with the multi-gene phylogenetic tree. This difference may be due to the few available genes for gapdh (only 37 linked data for gapdh in 70 recognised species).
Phyllosticta species can cause lesions on many plant species with a wide host range. The 10 strains in this study were collected from three host species, including Camellia oleifera, Machilus pauhoi, and Cunninghamia lanceolata. Among these strains, P. machili is the first reported isolation from M. pauhoi; whether the species is host-specific needs to be determined by pathogenicity testing. Until now, six species of Phyllosticta isolated from C. oleifera have been reported, i.e. P. camelliae, P. camelliaecola, P. capitalensis, P. erratica, P. theae, and P. theacearum. Among these species, P. capitalensis has a wide host range, with nearly 357 species, whereas the other species have been isolated only from C. oleifera (Norphanphoun et al. Citation2020). Phyllosticta cryptomeriae, P. concentrica, and P. cunninghamii have previously been isolated from Cu. lanceolata. In addition, Phyllosticta cunninghamii has also been isolated from Rhododendron cinnamomeum var. cunninghamii Paxton (Norphanphoun et al. Citation2020). P. cryptomeriae can be parasitic on Cryptomeria japonica (L.f.) D. Don. and P. concentrica has a wide range of hosts, with about 40 host species. Therefore, species of Phyllosticta may not have host specificity for C. oleifera and Cu. lanceolata. In addition, host specialisation in Phyllosticta may be related to lifestyle, and the endophytic fungus is less host-specific than the pathogenic fungus (Wikee et al. Citation2011); thus, P. capitalensis has a wider host range, which may be related to its endophytic lifestyle. A more in-depth study of whether there is host specificity and how it relates to fungal lifestyle would require a comparative analysis of genomic data, such as comparing the number and type of carbohydrate-active enzymes.
According to Okane et al. (Citation2003), Phyllosticta species, including pathogens, latent pathogens, and endophytes, are widely distributed and have a variety of lifestyles. Phyllosticta endophytica was isolated as an endophytic fungus from Cu. lanceolata in this study. Endophytes can transform into pathogenic organisms under certain circumstances (Rodriguez and Redman Citation2008; Rodriguez et al. Citation2009). Therefore, whether these species have specific hosts, their habitats, lifestyles, and the conditions under which they may transform need further investigation. This has important implications for the potential biological control of plant diseases.
Acknowledgements
We are grateful to Dr. Qiu-Tong Wang at Capital Normal University for her improvements to the manuscript.
Disclosure statement
No potential conflict of interest was reported by the author(s).
Additional information
Funding
References
- Baayen RP, Bonants P, Verkley G, Carroll GC, van der Aa HA, de Weerdt M, van Brouwershaven IR, Schutte GC, Maccheroni JW, Glienke de Blanco C, et al. 2002. Nonpathogenic isolates of the citrus black spot fungus, Guignardia citricarpa, identified as a cosmopolitan endophyte of woody plants, G. mangiferae (Phyllosticta capitalensis). Phytopathology. 92(5):464–477. doi:10.1094/PHYTO.2002.92.5.464.
- Bánki O, Roskov Y, Döring M, Ower G, Vandepitte L, Hobern D, Remsen D, Schalk P, DeWalt RE, Keping M, et al. 2022. Catalogue of life checklist (Version 2022-03-21). Catalogue Life. doi:10.48580/dfpd.
- Brittingham RL, O’Brien MJ. 1978. Occurrence of Phyllosticta cryptomeriae on Cryptomeria japonica in the United States. Plant Dis Reporter. 62:152–153.
- Brunaud P. 1888. Miscellanées mycologiques. Actes de la Société Linnéenne de Bordeaux. 42(2):85–104.
- Carbone I, Kohn LM. 1999. A method for designing primer sets for speciation studies in filamentous ascomycetes. Mycologia. 91(3):553–556. doi:10.1080/00275514.1999.12061051.
- Cheng LL, Thangaraj K, Deng C, Deng WW, Zhang ZZ. 2019. Phyllosticta capitalensis causes leaf spot on tea plant (Camellia sinensis) in China. Plant Dis. 103(11):2964. doi:10.1094/PDIS-04-19-0768-PDN.
- Ellis JB, Everhart BM 1900. The North American Phyllostictas with descriptions of the species published up to August. 1–79.
- Farris JS, Källersjö M, Kluge AG, Bult C 1994. Testing significance of incongruence. Cladistics. 10:315–319.
- Gardes M, Bruns TD. 1993. ITS primers with enhanced specificity for basidiomycetes application to the identification of mycorrhizae and rusts. Mol Ecol. 2(2):113–118. doi:10.1111/j.1365-294x.1993.tb00005.x.
- Glienke C, Pereira OL, Stringari D, Fabris J, Kava-Cordeiro V, Galli-T Erasawa L, Cunnington J, Shivas RG, Groenewald JZ, Crous PW. 2011. Endophytic and pathogenic Phyllosticta species, with reference to those associated with citrus black spot. Persoonia. 26(1):47–56. doi:10.3767/003158511X569169.
- Hyde KD, Chomnunti P, Crous PW, Groenewald JZ, Damm U, KoKo TW, Shivas RG, Summerell BA, Tan YP. 2010. A case for re-inventory of Australia’s plant pathogens. Persoonia. 25(1):50–60. doi:10.3767/003158510X548668.
- Moncalvo JM, Wang HH, Hseu RS. 1995. Phylogenetic relationships in Ganoderma inferred from the internal transcribed spacers and 25S ribosomal DNA sequences. Mycologia. 87(2):223–238. doi:10.1080/00275514.1995.12026524.
- Motohashi K, Inaba S, Anzai K, Akamatsu ST, Nakashima C. 2009. Phylogenetic analyses of Japanese species of Phyllosticta sensu stricto. Mycoscience. 50(4):291–302. doi:10.1007/S10267-009-0487-Z.
- Motohashi K, Nakashima C, Nishikawa J, Akiba M. 2008b. Studies on the Japanese species belonging to the genus Phyllosticta. Mycoscience. 1(49):11–18. doi:10.1007/S10267-007-0386-0.
- Myllys L, Stenroos S, Thell A. 2002. New genes for phylogenetic studies of lichenized fungi: glyceraldehyde-3-phosphate dehydrogenase and beta-tubulin genes. The Lichenologist. 34(3):237–246. doi:10.1006/lich.2002.0390.
- Nag Raj TR. 1993. Coelomycetous anamorphs with appendage-bearing conidia. Waterloo: Mycologue Publications.
- Nasehi A, Sathyapriya H, Wong MY. 2020. First Report of leaf spot on oil palm caused by Phyllosticta capitalensis in Malaysia. Plant Dis. 104(1):288. doi:10.1094/PDIS-06-19-1232-PDN.
- Norphanphoun C, Hongsanan S, Gentekaki E, Chen YJ, Kuo CH, Hyde KD. 2020. Differentiation of species complexes in Phyllosticta enables better species resolution. Mycosphere. 11(1):2542–2628. doi:10.5943/mycosphere/11/1/16.
- Nylander JAA. 2004. MrModeltest v2. Program distributed by the author. Sweden: Evolutionary Biology Centre, Uppsala University.
- Okane I, Lumyong S, Ito T, Nakagiri A. 2003. Extensive host range of an endophytic fungus, Guignardia endophyllicola (anamorph, Phyllosticta capitalensis). Mycoscience. 44(5):353–363. doi:10.1007/S10267-003-0128-X.
- Paul AR, Blackburn MD. 1986. Phyllosticta beaumarisii sp. nov., a cause of leafspot on Muehlenbeckia adpressa. Australas Plant Pathol. 15(2):40–41. doi:10.1071/APP9860040.
- Persoon CH. 1818. Traité sur les champignons comestibles, contenant l’indication des espèces nuisibles; a l’histoire des champignons. Paris (France): Belin-Leprieur.
- Petrini LE, Petrini O, Leuchtmann A, Carroll GC. 1991. Conifer inhabiting species of Phyllosticta. Sydowia. 43:148–169.
- Pu J, Xie Y, Zhang X, Qi Y, Zhang C, Liu X. 2008. Preinfection behaviour of Phyllosticta musarum on banana leaves. Australas Plant Path. 37(1):60–64. doi:10.1071/AP07079.
- Rodriguez R, Redman R. 2008. More than 400 million years of evolution and some plants still can’t make it on their own: plant stress tolerance via fungal symbiosis. J Exp Bot. 59(5):1109–1114. doi:10.1093/jxb/erm342.
- Rodriguez R, White JJ, Arnold A, Redman R. 2009. Fungal endophytes: diversity and functional roles. New Phytol. 182(2):314–330. doi:10.1111/j.1469-8137.2009.02773.x.
- Ronquist F, Huelsenbeck JP. 2003. MrBayes 3: Bayesian phylogenetic inference under mixed models. Bioinformatics. 19(12):1572–1574. doi: 10.1093/bioinformatics/btg180.
- Sabahi F, Mafakheri H, Mirtalebi M, Dolatabadi S, Guarnaccia V, Kharrati-Koopaee H, Banihashemi Z. 2022. First report of Phyllosticta capitalensis causing leaf spot of Japanese privet (Ligustrum japonicum) in Iran. Plant Pathol. 88(3):217–223. doi:10.1007/s10327-022-01063-8.
- Speschnew. 1904. Phyllosticta theae. Sada: Trudy Tbilissk. Bot. Inst. p. 74.
- Swofford DL. 2003. PAUP*. Phylogenetic analysis using parsimony (*and other methods). Version 4.0b10. Sunderland (Massachusetts): Sinauer Associates.
- Sydow P. 1897. Beiträge zur Kenntnis der Pilzflora der Mark Brandenburg. I Beiblatt Zur Hedwigia. 36:157–164.
- Tian LY, Lian T, Ke SK, Qin CS, Xu JC, Zhao DY, Qiu HL, Yang H, Jin XF, Li NL. 2019. The fungal diseases of Chinese fir in northern Guangdong. J for Environ Sci. 35(4):90–96.
- Tran NT, Miles AK, Dietzgen RG, Shuey TA, Mudge SR, Papacek D, Chandra KA, Drenth A. 2020. Inoculum dynamics and infection of Citrus fruit by Phyllosticta citricarpa. Phytopathology. 110(10):1680–1692. doi: 10.1094/PHYTO-02-20-0047-R.
- Trigiano RN, Windham MT, Windham AS. 2004. Plant pathology: concepts and laboratory exercises. Boca Raton: CRC Press. p. 1–600. doi:10.1201/9781315380773.
- Van der Aa Ha. 1973. Studies in Phyllosticta. Stud Mycol. 5:1–110.
- Van der Aa HA, Vanev S. 2002. A revision of the species described in Phyllosticta. Netherlands (Utrecht): Centraalbureau voor Schimmelcultures (CBS). p. 1–510.
- Vilgalys R, Hester M. 1990. Rapid genetic identification and mapping of enzymatically amplified ribosomal DNA from several Cryptococcus species. J Bacteriol. 172(8):4238–4246. doi:10.1128/jb.172.8.4238-4246.1990.
- Wang X, Chen G, Huang F, Zhang J, Hyde KD, Li H. 2012. Phyllosticta species associated with citrus diseases in China. Fungal Divers. 52(1):209–224. doi:10.1007/s13225-011-0140-y.
- White T, Burns T, Lee S, Taylor J. 1990. Amplification and direct sequencing of ribosomal RNA genes for phylogenetics. New York: PCR protocols: a guide to methods and applications. San Diego, California: Academic Press; p. 315–322. doi:10.1016/B978-0-12-372180-8.50042-1.
- Wicht B, Petrini O, Jermini M, Gessler C, Broggini GAL. 2012. Molecular, proteomic and morphological characterization of the ascomycete Guignardia bidwellii, agent of grape black rot: a polyphasic approach to fungal identification. Mycologia. 104(5):1036–1045. doi:10.3852/11-242.
- Wikee S, Lombard L, Crous PW, Nakashima C, Motohashi K, Chukeatirote E, Alias SA, McKenzie EHC, Hyde KD. 2013a. Phyllosticta capitalensis, a widespread endophyte of plants. Fungal Divers. 60(1):91–105. doi:10.1007/s13225-013-0235-8.
- Wikee S, Lombard L, Nakashima C, Motohashi K, Chukeatirote E, Cheewangkoon R, McKenzie EHC, Hyde KD, Crous PW. 2013b. A phylogenetic re-evaluation of Phyllosticta (Botryosphaeriales). Stud Mycol. 76:1–29. doi:10.3114/sim0019.
- Wikee S, Udayanga D, Crous PW, Chukeatirote E, McKenzie EHC, Bahkali AH, Dai DQ, Hyde KD. 2011. Phyllosticta – an overview of current status of species recognition. Fungal Divers. 51(1):43–61. doi:10.1007/s13225-011-0146-5.
- Wikee S, Wulandari NF, McKenzie EHC, Hyde KD. 2012. Phyllosticta ophiopogonis sp. nov. from Ophiopogon japonicas (Liliaceae). Saudi J Biol SC. 19(1):13–16. doi:10.1016/j.sjbs.2011.10.003.
- Wong MH, Crous PW, Henderson J, Groenewald JZ, Drenth A. 2012. Phyllosticta species associated with freckle disease of banana. Fungal Divers. 56(1):173–187. doi:10.1007/s13225-012-0182-9.
- Wulandari NF, Hyde KD, Duong LM, De Gruyter J, Meffert JP, Groenewald JZ, Crous PW. 2009. Phyllosticta citriasiana sp. nov., the cause of citrus tan spot of Citrus maxima in Asia. Fungal Divers. 34:23–39.
- Wulandari NF, To-Anun C, Lei C, Abd-Elsalam KA, Hyde KD. 2010. Guignardia/ Phyllosticta species on banana. Cryptogamie Mycol. 31(4):403–418.
- Yip HY. 1989. Five new species of Phyllosticta on Australian native plants. Mycol Res. 93(4):489–496. doi:10.1016/S0953-7562(89)80041-3.
- Zhang WH, Su D, Sun R. 2022. First report of Phyllosticta capitalensis causing black freckle disease on Rubus chingii in China. Plant Dis. 106(5):1517. doi:10.1094/PDIS-05-21-1031-PDN.