Abstract
The present study describes the structure, composition and regeneration of tree species in undisturbed and disturbed tropical forests in Bhuban hills in southern Assam, India. The study area is part of one of the globally recognized biodiversity hot spots and in spite of the increasing human activities in these forests, so far no information is available on their impacts on plant assemblages in the area. In each forest, the vegetation was analysed through 50 quadrats (10 m × 10 m) in a belt transect of 500 m × 10 m. A total of 49, 47 and 42 tree species were recorded in disturbed forests and 62, 61 and 60 species in undisturbed forests. The average tree density and basal area were significantly higher in undisturbed forests (708.67 and 42.01 m2 ha−1, respectively) than disturbed forests (443.33 and 18.44 m2 ha−1, respectively). The population structure of tree species in disturbed forests showed a fluctuating curve, and in undisturbed forests, it was a reversed J-shaped curve. The present study reveals that the species richness, diversity, density and basal area have significantly declined (P < 0.01) due to anthropogenic disturbances. As reflected from the population structure, most of the tree species in disturbed forests showed poor regeneration status. If degradation of these forests continues at the present pace, several of these species which are providing various ecosystem services to the local human communities may disappear in the very near future.
Introduction
Although tropical forests harbour the world’s most species rich plant communities, they are also vulnerable to deforestation and degradation (World Conservation and Monitoring Centre Citation1992; LaFrankie et al. Citation2006; Lu et al. Citation2010). The degradation of tropical forests and habitat loss due to anthropogenic activities are the major causes of decline in biodiversity (Pickett & White Citation1985). To meet the increasing demands of rapidly increasing human population, natural forest resources are being utilized far beyond their regenerative capacity. This has resulted in decreasing size of natural forests at alarming rates in India and other parts of the world. The effect of human disturbances on species diversity is an issue that has engaged ecologist since long (Pickett & White Citation1985; Tilman Citation1988; Vetaas Citation1997; Sheil Citation1999; Lawes et al. Citation2007; Sapkota et al. Citation2009; Lu et al. Citation2010). For the management of both undisturbed and disturbed forest communities, it is very important to understand forest structure and composition (Congdon & Herbohn Citation1993; Ssegawa & Nkuutu Citation2006). Such an understanding helps the forest managers to assess the potential impacts, the amelioration of effects of disturbance, optimization of productivity and rehabilitation of degraded ecosystems (Congdon & Herbohn Citation1993). Assessment of floristic composition and structure of forest communities is also useful in identifying important elements of plant diversity, protecting threatened and economic species, and monitoring the forest communities, among others (Tilman Citation1988; Ssegawa & Nkuutu Citation2006).
Human disturbance in tropical forests is not simply a phenomenon of the colonial and modern eras, but dates back to early human occupation in tropical region (Devenan Citation1976; Fairhead & Leach Citation1998). Disturbances of both natural and human origin influence forest dynamics and tree diversity at local and regional scales (Sheil Citation1999; Sapkota et al. Citation2009). Disturbances not only influence diversity, but also post disturbance regeneration and dominance of tree species (Lawes et al. Citation2007). Several workers (Vetaas Citation1997; Sheil Citation1999; Venkateswaran & Parthasarathy Citation2003; Lawes et al. Citation2007) have explained the relationship between disturbance and species richness, but the studies that elucidate how disturbances influence stand structure, species composition and regeneration of tree species are very limited (Sapkota et al. Citation2009).
Tropical forests in southern part of Assam are one of the biodiversity rich regions of northeast India. The tropical forests in this region still retain significant proportion of its phytodiversity, possibly due to long years of isolation and difficult terrain. However, they are now under increasing anthropogenic pressure (such as timber extraction, firewood collection, cattle grazing, etc.) to unleash its resources for economic development (Borah & Garkoti Citation2011). In spite of the increasing human activities in the forests, no studies have been conducted to assess their impacts on plant assemblages in the area. Past studies indicate that due disturbance in the forests; conditions become unfavourable for existing species and fail to re-establish themselves (Kramer Citation1933; Singh & Singh Citation1992). Based on this understanding, we hypothesized that the disturbance would help light demanding opportunist species to get established in the gaps created due to forest biomass extraction and hence would result in changes in forest community structure and regeneration patterns. The present study determined the impact of anthropogenic activities on the diversity, composition and structure of the tropical forests in this region.
Materials methods
Study area
The southern part of Assam, popularly known as Barak Valley, consists of three districts (viz. Cachar, Hailakandi and Karimganj). The region experiences a warm and humid climate having mean annual rainfall of 2508 mm (Athokpam & Garkoti Citation2013). According to Champion and Seth (Citation1968), vegetation of the region is dominated by Cachar tropical evergreen forests (1/1/B/C3) and Cachar tropical semi-evergreen forests (2/2B/C3). The forests of this region are relatively unexplored harbouring rich plant diversity. The vegetation of this region has been free from anthropogenic disturbances over centuries. But due to rapid population growth and development activities, some parts of the forests are under huge anthropogenic pressure such as over exploitation of species for timber, fuelwood, fodder, bamboo harvesting and settlement.
Methodology
For the present study, a total of six forest sites (three undisturbed and three disturbed) were selected based on species density, canopy cover, intensity and frequency of anthropogenic activities, etc., in the Bhuban Hills of Assam. Study Sites I, II and III were at the foothill and closer to the human settlements (). Due to close proximity, people frequently visit these forests for collection of various provisioning services from the forests like timber, fuelwood, bamboo and other non-timber forest products (NTFPs). Study Sites IV, V and VI were at higher elevations and far from the human settlements. People rarely visit IV, V and VI forest sites to collect forest resources due to the distance, difficult terrains and also availability of resources in nearby forests. Consequently, they have in general remained free from anthropogenic pressures.
Table 1. Study sites with disturbance index and GPS coordinates, altitude, disturbance index, nearest village and distance of the forest from the village.
The vegetation was analysed through a belt transect of 500 m × 10 m in each forest following Ganesh et al. (Citation1996) and Borah and Garkoti (Citation2011). Each belt transect was again subdivided into 50 quadrats of 10 m × 10 m size along its length. Girths at breast height (gbh at 1.37 m from the ground) of all trees in each quadrat were measured. Arbitrarily an individual with ≥10 cm gbh was categorized as tree. The cut stumps and lopped trees in each quadrat were counted and the girth measured at ground height (i.e. 10 cm from the ground). The saplings and seedlings were sampled at each 50 m along the belt transect using a 5 m × 5 m quadrat. Specimens of each species were brought to the laboratory and prepared for the herbarium. All the plant species were identified with the help of ‘Flora of Assam’ (Kanjilal Citation1934–1940), ‘Assam Flora’ (Chowdhury et al. Citation2005) and the herbarium of the Botanical Survey of India, Shillong. The phytosociological data were quantitatively analysed for density, frequency, basal area (Curtis & McIntosh Citation1950), relative density, relative frequency and relative dominance (Phillips Citation1959; Garkoti & Singh Citation1994). To analyse and interpret species population dynamics, gbh structure of all tree species was stressed. Trees were categorized into nine gbh classes starting with 10 cm and ending with >240 cm class for the analysis of population structure. All the individuals of ≥3 to <10 cm girth were considered as tree saplings and <3 cm girth considered as tree seedlings. The total number of individuals belonging to these classes was calculated for undisturbed and undisturbed forest following Muller-Dombois & Ellenberg (Citation1974).
A disturbance index for each forest site was calculated following Kanzaki and Kyoji (Citation1986), Rao et al. (Citation1990) and Bhuyan et al. (Citation2003). The disturbance index (DI) was calculated as the basal area of cut trees measured at the ground level expressed as fraction of total basal area of all trees:
Structure of the present study forests were assessed quantitatively by calculating density, basal area and importance value index (IVI) following Magurran (Citation2004).
Diversity index was calculated following Shannon and Wiener (Citation1963) as follows:
The concentration of dominance was calculated following Simpson (Citation1949) as follows:
The species evenness was calculated following Pielou (Citation1975) as follows:
Statistical analyses were performed by using SPSS 16.0 (SPSS Inc., Chicago, IL, USA). To determine the effects of anthropogenic disturbances on structure and composition of forest sites, comparisons of different phytosociological attributes (such as species richness, density, basal area and diversity indices) between undisturbed and disturbed forests were done by paired t-test. Pearson bivariate correlation was performed to determine the correlations between different phytosociological attributes and disturbance index. The nearest distance from the human settlements to the study sites were estimated with the help of Google map and Google Earth software (Google Inc., Santa Clara County, CA, USA).
Results
Species richness and diversity
Both tree species richness and diversity have decreased from undisturbed forests to undisturbed forests of the present study. A total of 88 tree species were recorded in the present study, of which 75 and 56 tree species were recorded from undisturbed and disturbed forests, respectively. Several species were common to both sites. The highest number of tree species (62 species from 35 families) was recorded in the undisturbed forest Site V while the lowest number of species (42 species from 26 families) was recorded in the disturbed forest Site III (). In the undisturbed and disturbed forests, the average tree species number per 0.5 ha area were 61 species (from 32 families) and 46 species (26 families), respectively (). The t-test shows that species richness and family richness were significantly different (P < 0.05) in the undisturbed and disturbed forests (). The Shannon diversity index (H′) ranged from 1.44 to 1.98 (). The average value of Shannon diversity index was also significantly higher (P < 0.001) in the undisturbed forests (1.95) than the disturbed forests (1.49) (). The average species evenness (J′) and Hmax values () were higher in undisturbed forests (56.03 and –0.029, respectively) than that of disturbed forests (41.11 and 0.036, respectively).
Table 2. Different plant community attributes of the forest study sites.
Table 3. Comparison of different plant community attributes between undisturbed and disturbed forests.
Species composition
Although both undisturbed and disturbed forests have several common species but disturbance in the forests resulted arrival and establishment of many new species adapted to disturbance only. Therefore, disturbance has resulted in the change of species composition in the present study forests. Cynometra polyandra was the most dominant tree species in the undisturbed forests (with an average IVI value 25.59) followed by Mesua ferrea, Stereospermum personatum, Artocarpus chama, Palaquium polyanthum, Mesua floribunda, Dysoxylum binectariferum, Trewia nudiflora, Pterygota alata, etc. (). In the disturbed forests, Artocarpus chama was the most dominant tree species (IVI value 32.27) followed by Schima wallichii, Tetrameles nudiflora, Mitragyna rotundifolia, Toona ciliata, Castanopsis purpurella, Callicarpa arborea, etc. (). Some species such as Holarrhena pubescens, Grewia nervosa, Maesa indica, Streblus asper, Albizia procera, Bridelia monoica, Maesa Montana, Alangium chinensis, Cassia fistula, Symplocos cochinensis were unique to the disturbed forests. On the other hand, some important tree species such as Cinnamomum glaucescens, Crysophyllum roxburghii, Dillenia pentagyna, Dysoxylum binectariferum, Garcinia cowa, Hydnocarpus kurzii, Mangifera sylvatica, Mesua ferrea, Michelia champaca, Pterygota alata, Podocarpus nerifolia, Saraca asoca, Xerospermum glabratum, Zanthoxylum rhetsa, etc., found only in the undisturbed forests.
Table 4. Tree species recorded in the disturbed (D) and undisturbed (UD) forests with their average IVI.
Tree density and basal area
The density and basal area of the tree species were significantly lower in the disturbed forest sites than the undisturbed forest sites. The highest tree density (794 trees ha−1) was recorded in the undisturbed forest Site VI and the lowest (344 trees ha−1) in the disturbed forest Site III (). The average tree density was significantly higher (P < 0.05) in undisturbed forests (708.67 trees ha−1) compared to the disturbed forests (443.33 trees ha−1). The tree basal area in this study ranged from 17.21 to 45.07 m2 ha−1 (). The average tree basal area was also significantly higher (P < 0.001) in the undisturbed forests (42.01 m2 ha−1) than the disturbed forests (18.44 m2 ha−1) ().
Population structure
Distribution of tree densities in different girth classes in undisturbed forests revealed reversed J-shaped curve (). In undisturbed forests, population densities decreased with increased girth classes. But in disturbed forest, a fluctuating pattern of population was recorded. In disturbed forests, trees from the girth class 150–180 cm and >210 trees were absent.
Figure 1. Distribution of (a) population density and (b) tree basal area in different girth classes in undisturbed (UD) and disturbed forests (D).
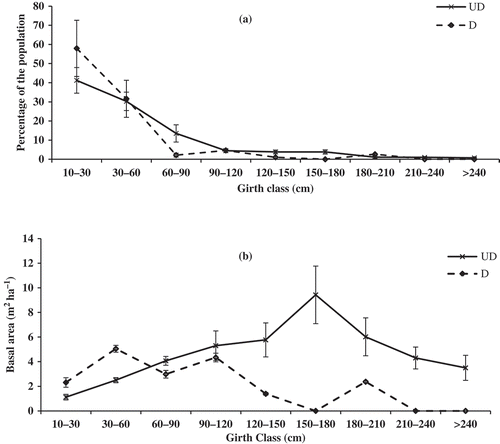
The distribution of basal area in different girth classes is shown in the . In undisturbed forests, the basal area increased with increase in girth class up to 150–180 cm girth class and then declined. But in disturbed forests, 30–60 cm contributed more basal area than the other girth classes and followed a fluctuating pattern of distribution curve.
Relationship between different community attributes and disturbance index
In the present study, plant community attributes such as species richness, Shannon diversity index, tree density and tree basal area were negatively correlated with disturbance index and positively correlated with the distance from the human settlements (). All the studied community characteristics, except for tree density, were positively correlated (P < 0.01) with altitude. Also disturbance index was positively correlated with the distance from human settlements (P < 0.001) and altitude (P < 0.01) (). The present study also examined the relationship between standing tree basal area and cut stump basal area in different girth classes. A positive and significant (P < 0.01) correlation was recorded between the standing tree basal area and cut stump basal area in different girth classes ().
Figure 2. Relationship between standing tree basal area and cut stump’s basal area of different girth classes.
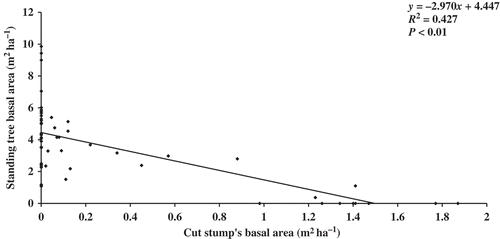
Table 5. Relationships of different community attributes with disturbance index, distance from the human settlements and altitude.
Seedlings and saplings
The species richness and densities of saplings and seedlings were also lower in the disturbed forest sites than those of the undisturbed forest sites. The maximum numbers of sapling species (37 species) was recorded in undisturbed forest Site V and minimum number of species (22 species) in disturbed forest Site II. The highest number of seedling species (26 species) was recorded in the undisturbed forest Site IV and lowest number of seedling species (13 species) occurred in disturbed forest Site I (). The sapling density ranged from 3160 to 6200 individuals ha−1 and seedling density ranged from 3080 to 4360 individuals ha−1 (). The highest sapling basal area (3.32 m2 ha−1) was recorded in the undisturbed forest Site V and the lowest sapling basal area (1.65 m2 ha−1) in the disturbed forest Site I (). The seedling basal area ranged from 0.047 to 0.089 m2 ha−1 (). The average number of sapling species, density and basal area were higher in undisturbed forests (34.33 species, 5786.67 individuals ha−1 and 3.06 m2 ha−1, respectively) than in the disturbed forests (24.67 species, 4066.67 individuals ha−1 and 2.28 m2 ha−1, respectively). Sapling species richness and densities were significantly (P < 0.05) different in undisturbed and disturbed forests ().
Table 6. Average species richness, density and basal area of seedlings and saplings in different forest sites of the present study.
The seedling species richness in undisturbed forests ranged from 19 to 26 species with an average of 22.3 species while in disturbed forests, it ranged from 13 to 17 species with average seedling species richness of 15.7 species ( and ). But the statistical analyses did not show any significant difference in seedling richness between disturbed and undisturbed forest (). The seedling density (3880 individuals ha−1) and basal area (0.07 m2 ha−1) were higher in disturbed forest than the values recorded in the undisturbed forests (3586.67 individuals ha−1 and 0.06 m2 ha−1, respectively) ().
Discussion
Species richness of a disturbed forest is a cumulative outcome of differential responses of species to disturbances (Sagar et al. Citation2003). In the present study, tree species richness were lower in disturbed forest sites (49, 47 and 42 species, respectively, in Sites I, II and III) than that of undisturbed sites (61, 62 and 60 species, respectively, in Sites IV, V and VI). This may be due to more frequent and heavy extraction of forest resources (such as timber, fuelwood and other minor forest products) by the local community near the disturbed sites. A large number of cut stumps recorded in the disturbed sites indicate tree extraction in those sites. Local people still depend heavily on different ecosystem services (such as fuelwood, timber, fodder and various NTFPs) provided by the forests for their subsistence. The absence of a number of species (recorded in the undisturbed sites) from the disturbed sites may be due to increased human demand and overexploitation of certain resources. Earlier studies (Noble & Dirzo Citation1997; Sheil Citation1999; Sagar et al. Citation2003) have also shown that environmental change due to the anthropogenic disturbances result in loss of species from different plant communities. The highest species richness and Shannon diversity were recorded in the Site V (undisturbed forest) which is located at comparatively higher and difficult terrain than disturbed sites. Due to the availability of resources in nearby forest ecosystems, people do not prefer to go long distances. The difficult terrain also deterred people from exploiting the forests at those sites for timber or other plant resources. Hence they have remained undisturbed and contributed to the maintenance of plant diversity in the area. Lack of human disturbance may create more conducive environmental conditions for growth and establishment of tree species, which in turn may result in higher species richness in undisturbed forests compared to the disturbed forests. Leigh (Citation1965) reported that ecosystems with higher species richness are more complex, with higher number of interactions among them, which gives the ecosystem more stability than ecosystems with lower species richness.
Shannon diversity index values for the present study forests fall within the range (0.70–3.57) reported for the tropical forests of the region (Bhuyan et al. Citation2003; Mishra et al. Citation2004; Deb et al. Citation2009; Tripathi et al. Citation2010; Borah & Garkoti Citation2011; Borah et al. Citation2012). The lower Shannon diversity index in disturbed forest sites may be due to the human disturbances which occurred in them. According to Noble and Dirzo (Citation1997), anthropogenic disturbances such as logging usually cause an immediate decline in species diversity. This explains why in the present study, a positive and significant correlation was recorded between diversity index and disturbance.
The present study revealed that human disturbances affected not only the species richness and diversity, but also the species composition of the forests. The most remarkable change in species composition due to disturbance was the change of dominance (based on IVI) in plant communities. Cynometra polyandra, Mesua ferrea, Stereospermum personatum, Artocarpus chama, Palaquium polyanthum, Mesua floribunda, Dysoxylum binectariferum, Trewia nudiflora, Pterygota alata, etc., were dominant tree species in the undisturbed forests; on the other hand A. chama, Schima wallichii, Tetrameles nudiflora, Mitragyna rotundifolia, Toona ciliata, Castanopsis purpurella, Callicarpa arborea, etc., have dominated in disturbed forest sites. Continuous removal of tree biomass from the forests resulted in formation of canopy gaps of variable sizes which enabled increased light intensity to reach on the forest floor. Disturbances in the forest ecosystems can alter the environmental condition in the forests by changing light availability and soil conditions which ultimately change the species composition of the forests (Singh et al. Citation1997; Fredericksen and Mostacedo Citation2000). Several light demanding successional species (such as Artocarpus chama, Schima wallichii, Mitragyna rotundifolia, Toona ciliata, Castanopsis purpurella and Callicarpa arborea) occupied the gaps and dominated plant community after the disturbance. The over extraction of important timber species from the disturbed forests may have led to the local disappearance of several species and establishment of light demanding tree species (Borah & Garkoti Citation2011). Some earlier workers (Thorington et al. Citation1982; Mishra et al. Citation2004) reported that the shift in tree species dominating plant communities seems to be linked with the level of anthropogenic disturbances.
The negative correlation of tree density and basal area with disturbance index in the present study indicates that increased disturbance lowered the tree density and basal area of the forest. The heavy and rapid extraction of tree species from disturbed forest sites led to lower tree density and basal area in these forest sites than that of undisturbed forest sites. The tree density recorded in the present study is comparable with findings of some earlier reports from the tropical forests of northeast India (245–1620 trees ha−1) by Bhuyan et al. (Citation2003), Mishra et al. (Citation2004, Citation2005), Deb et al. (Citation2009), Tripathi et al. (Citation2010), Borah and Garkoti (Citation2011) and Borah et al. (Citation2012). The tree basal area recorded in the present study is comparable to the values reported from other tropical forest ecosystems (Parthasarathy & Sethi Citation1997; Upadhaya et al. Citation2004; Nath et al. Citation2005; Kumar et al. Citation2006; Deb et al. Citation2009; Tripathi et al. Citation2010). In the present study, a strong and significant (P < 0.01) negative correlation was recorded between standing tree basal area and cut stump’s basal area in different girth classes. Distribution of basal area in different girth classes showed a fluctuating pattern of curve in disturbed forests. Lower basal area in higher girth classes indicates the removal of those trees from the forest in past. This trend supports the findings of Bhuyan et al. (Citation2003) and Borah et al. (Citation2012) for tropical wet evergreen forests of northeast region.
Tree size class distribution is an important indicator of changes in population structure and species composition of a forest ecosystem (Newbery & Gartlan Citation1996). Similar to earlier reports from different tropical forests of India (Kadavul & Parthasarathy Citation1999; Nath et al. Citation2005; Rajkumar & Parthasarathy Citation2008; Deb et al. Citation2009; Page et al. Citation2010; Borah & Garkoti Citation2011; Borah et al. Citation2012), population structure of tree species in the undisturbed forests showed a reverse J-shaped curve pattern. The reverse J-shaped distribution curves are characteristics of the forests with continuous regeneration (Khamyong et al. Citation2004). However, the disturbed forests showed a fluctuating pattern of distribution curve which is generally common in disturbed forests (Borah & Garkoti Citation2011). Fluctuating pattern of tree size class distribution may indicate the presence of unplanned and unsustainable exploitation of trees in the forest (Alelign et al. Citation2007). The number of large and old trees was low in the disturbed forest sites of the present study, indicating the heavy removal of mature trees from these sites. The low abundance of large and mature trees in the disturbed forests might have had adverse effects on tree natural regeneration due to a reduction in the number of seeds produced by the forests (Singh et al. Citation1997).
Lower sapling and seedling richness, sapling density and basal area in disturbed forests in the present study may be due to damage and trampling of seedlings and saplings during the biomass extraction. Furthermore, this trend may be due to decline in number of adult individuals of several species of reproductive age that could produce sufficient number of seeds for regeneration. Contrary to the findings of Pare et al. (Citation2009) and Borah et al. (Citation2012), which showed significant positive relationship between seedling and adult tree density, seedling density and basal area were higher in disturbed forests. It seems that increased light intensity, decreased inter-specific and intra-specific competition for available soil resources in the gaps created by disturbances may have favoured seedlings of light demanding successional species (such as Callicarpa arborea, Grewia nervosa, Holarrhena pubescens, Mallotus ferrugineus, Mitragyna rotundifolia, Schima wallichii) in the disturbed sites. However, due to excessive disturbance, even the seedlings of those light demanding species failed to reach the sapling stage (Chapman & Chapman Citation1997; Eilu & Obua Citation2005; Garkoti Citation2008).
Consistent with the findings of Singh et al. (Citation1997) and Shaheen et al. Citation2011, in the present study, disturbance index was negatively correlated with the distance from human settlements (P < 0.001) and altitude of study sites (P < 0.01) (). From these correlations, it is clear that the forests near the human habitation and at lower altitude are more disturbed than those located far and higher at altitude.
Conclusion
Anthropogenic pressures due to the unsustainable exploitation of the forests resources from the sites closer to the human settlements have resulted in decline in plant diversity and density, and have affected regeneration of tree species. Species population structure indicates that most of the tree species in the disturbed forests show poor regeneration. Several important species that were typical to undisturbed forests were absent in the disturbed forests which has affected community structure and species composition. It appears that if degradation of these forests continues at present pace several of these species which are providing various ecosystem services to the local human communities may disappear locally in very near future.
Acknowledgements
We thank anonymous reviewers for their comments and valuable suggestions. The work was funded by Department of Biotechnology, Government of India.
References
- Alelign A, Teketay D, Yemshaw Y, Edwards S. 2007. Diversity and status of regeneration of woody plants on the peninsula of Zegie, northwestern Ethiopia. Trop Ecol. 48:37–49.
- Athokpam FD, Garkoti SC. 2013. Variation in evergreen and deciduous species leaf phenology in Assam, India. Trees. 27:985–997.
- Bhuyan P, Khan ML, Tripathi RS. 2003. The tree diversity and population structure in undisturbed and human impacted stands of tropical wet evergreen forest in Arunachal Pradesh, Eastern Himalayas, India. Biodivers Conserv. 12:1753–1773.
- Borah N, Das AK, Garkoti SC. 2012. Community structure and diversity of tropical forests along with disturbance gradients in Barak Valley of Assam, India. Ass Uni J Sci Tech. 9:38–45.
- Borah N, Garkoti SC. 2011. Tree species composition, diversity and regeneration patterns in undisturbed and disturbed forests of Barak Valley, South Assam, India. Int J Ecol Environ Sci. 37:131–141.
- Champion HG, Seth SK. 1968. A revised survey of the forest type of India. New Delhi: Govt. of India publications.
- Chapman C, Chapman LJ. 1997. Forest regeneration in logged and unlogged forests of Kibale National Park, Uganda. Biotropica. 29:369–412.
- Chowdhury S, Nath AK, Bora A, Das PP, Phukan U. 2005. Assam’s flora. Assam (India): Science Technology and Environment Council, Guwahati, Assam.
- Congdon RA, Herbohn JL. 1993. Ecosystem dynamics of disturbed and undisturbed sites in north Queensland wet tropical rain forest. I Floristic composition, climate and soil chemistry. J Trop Ecol. 9:349–363.
- Curtis JT, McIntosh RP. 1950. The interrelations of certain analytic and synthetic phytosociological characters. Ecology. 31:434–455.
- Deb P, Sundriyal RC, Shankar U. 2009. Tree diversity and population structure in a low land tropical rain forest in the Eastern Himalayas India. Indian Forester. 135:1526–1544.
- Denevan WM. 1976. The native population of the Americans in 1492. Madison (WI): University of Wisconsin Press.
- Eilu G, Obua J. 2005. Tree condition and natural regeneration in disturbed sites of Bwindi Impenetrable Forest National Park, southwestern Uganda. Trop Ecol. 46:99–111.
- Fairhead J, Leach M. 1998. Reframing deforestation, global analysis and local realities: studies in west Africa. London: Routledge.
- Fredericksen TS, Mostacedo B. 2000. Regeneration of Timber species following selection logging in a Bolivian tropical dry forest. For Ecol Manage. 131:47–55.
- Ganesh T, Ganesan R, Soubadra Devy M, Davidar P, Bawa KS. 1996. Assessment of plant biodiversity at mid-elevation evergreen forest of Kalakand Mundanthuari Tiger Reserve, Western Ghats, India. Curr Sci. 71:379–392.
- Garkoti SC. 2008. Estimates of biomass and primary productivity in a high altitude maple forest of central Himalaya. Ecol Res. 23:41–49.
- Garkoti SC, Singh SP. 1994. Nutrient cycling in three central Himalayan forests ranging from close-canopied to open-canopied tree-line forests. Arc Alp Res. 26:339–348.
- Kadavul K, Parthasarathy N. 1999. Structure and composition of woody species in tropical semi-evergreen forest of Kalayan Hills, Eastern Ghats, India. Trop Ecol. 40:247–260.
- Kanjilal UN. 1934–1940. Flora of Assam. Vol. I–IV. Shillong: Government of Assam.
- Kanzaki M, Kyoji Y. 1986. Regeneration in subalpine coniferous forests: mortality and pattern of death of canopy trees. Bot Mag Tokyo. 99:37–52.
- Khamyong S, Lykke AM, Seramethakun D, Barford AS. 2004. Species composition and vegetation structure of an upper montane forest at summit of Mt. Doi Inthanon, Thailand. Nord J Bot. 23:83–97.
- Kramer F. 1933. De natuurlijke verjonging in het Goennoeng Greden complex. Tectona. 26:156–185.
- Kumar A, Marcot BG, Saxena A. 2006. Tree species diversity and distribution patterns in tropical forests of Garo Hills. Curr Sci. 91:1370–1381.
- LaFrankie JV, Ashton PS, Chunyong GB, Co L, Condit R, Davaries SJ, Fooster R, Hubbell SP, Kenfack D, Lagunzad D, et al. 2006. Contrasting structure and composition of the understory in species rich tropical rainforests. Ecology. 87:2298–2305.
- Lawes MJ, Joubert R, Griffiths ME, Boudreau S, Chapman CA. 2007. The effect of the spatial scale of recruitment on tree diversity in Afromontane forest fragments. Biol Conserve. 139:447–456.
- Leigh E. 1965. On a relation between the productivity biomass, stability and diversity of a community. Proc Natl Acad Sci USA. 53:777–783.
- Lu XT, Yin JX, Tang JW. 2010. Structure, tree species diversity and composition of tropical rainforests in Xishuangbanna, south west China. J Trop Forest Sci. 22:260–270.
- Magurran AE. 2004. Ecological diversity and its measurement. Cambridge (MA): University Press.
- Mishra BP, Tripathi OP, Laloo RC. 2005. Community characteristic of climax subtropical humid forest of Meghalaya and population structure of ten important tree species. Trop Ecol. 46:241–251.
- Mishra BP, Tripathi OP, Tripathi RS, Pandey HN. 2004. Effect of anthropogenic disturbance on plant diversity and community structure of sacred grove in Meghalaya, north-east India. Biodivers Conserv. 13:421–436.
- Mullar Dombios D, Ellenberg H. 1974. Aims and methods of vegetation analysis. New York (NY): Wiley.
- Nath PC, Arunachalam A, Khan ML, Arunachalam K, Barbhuiya AR. 2005. Vegetation analysis and tree population structure of tropical wet evergreen forest in and around Namdapha National Park, northeast India. Biodivers Conserv. 14:2109–2136.
- Newbery DMC, Gartlan JS. 1996. A structural analysis of rain forests at Korup and Douala-Edea, Cameroon. Proc Roy Soc Edin. 104B:107–124.
- Noble IR, Dirzo R. 1997. Forest as human-dominated ecosystems. Science. 277:522–525.
- Page NV, Qureshi Q, Rawat GS, Kushalappa CG. 2010. Plant diversity in sacred forest fragments of Western Ghats: a comparative study of four life forms. Plant Ecol. 206:237–250.
- Pare S, Savadogo P, Tigabu M, Oden PC, Ouadba JM. 2009. Regeneration and spatial distribution of seedling populations in Sudanian dry forests in relation to conservation status and human pressure. Trop Ecol. 50:339–353.
- Parthasarathy N, Sethi P. 1997. Tree and Lianas species and population structure in a tropical dry evergreen forest in South India. Trop Ecol. 38:19–30.
- Philips EA. 1959. Methods of vegetation study. New York (NY): Holt, Rinehart and Winston.
- Pickett STA, White PS. 1985. The ecology of natural disturbance and patch dynamics. Orlando (FL): Academic Press.
- Pielou EC. 1975. Ecological diversity. New York (NY): Wiley.
- Rajkumar M, Parthasarathy N. 2008. Tree diversity and structure of Andaman giant evergreen forests, India. Taiwania. 53:356–368.
- Rao PB, Barik SK, Pandey HN, Tripathi RS. 1990. Community composition and tree population structure of subtropical humid forest along a disturbance gradient. Vegetatio. 88:151–192.
- Sagar R, Raghubanshi AS, Singh JS. 2003. Tree species composition, dispersion and diversity along a disturbance gradient in a dry tropical forest region of India. Forest Ecol Manag. 186:61–71.
- Sapkota IP, Tigabu M, Oden PC. 2009. Spatial distribution, advanced regeneration and stand structure of Nepalese sal (Shorea robusta) forest subject to disturbances of different intensities. Forest Ecol Manag. 257:1966–1975.
- Shaheen H, Qureshi RA, Shinwari ZK. 2011. Structural diversity, vegetation dynamics and anthropogenic impact on lesser Himalayan subtropical forests of Bagh district, Kashmir. Pak J Bot. 43:1861–1866.
- Shannon CE, Weaver W. 1963. The Mathematical theory of communication. Urbana (IL): University of Illinois Press; p. 117.
- Sheil D. 1999. Tropical forest diversity, environmental change and species augmentation: after intermediate disturbance hypothesis. J Veg Sci. 10:851–860.
- Simpson EH. 1949. Measurement of diversity. Nature (London). 163:688.
- Singh SP, Rawat YS, Garkoti SC. 1997. Failure of brown oak (Quercus leucotrichophora) to regenerate in Central Himalaya – a case of environmental semisurprise. Curr Sci. 73:371–374.
- Singh JS, Singh SP. 1992. Forests of Himalaya: structure, functioning and impact of man. Nainital: Gyanodaya Prakashan.
- Ssegawa P, Nkuutu DN. 2006. Diversity of vascular plants on Ssese Islands in Lake Victoria, Central Uganda. Afr J Ecol. 44:22–29.
- Thorington RW Jr, Tannenbaum B, Tarak A, Rundran R. 1982. Distribution of tree in Barro Colorado, Island: a five hectare sample. In: Leigh EG Jr., Rand AS, Windser DM, editors. The ecology of tropical forest-seasonal rhythms and long-term changes. Washington (DC): Smithsonia Tropical Research Institute; p. 83–94.
- Tilman D. 1988. Plant strategies and the dynamics and structure of plant communities. Princeton (NJ): Princeton University Press.
- Tripathi OP, Upadhaya K, Tripathi RS, Pandey HN. 2010. Diversity, dominance and population structure of tree species along fragment- size gradient of subtropical humid forest of northeast India. Res J Envt Earth Sci. 2:97–105.
- Upadhaya K, Pandey HN, Law PS, Tripathi RS. 2004. Diversity and population characteristics of woody species in subtropical humid forest exposed to cultural disturbance in Meghalaya, Northeast, India. Trop Ecol. 45:303–314.
- Venkateswaran R, Parthasarathy N. 2003. Tropical dry evergreen forests on the Coromandel Coast of India: structure, composition and human disturbance. Ecotropica. 9:45–58.
- Vetaas OR. 1997. Spatial and temporal vegetation changes along a moisture gradient in northeastern Sudan. Biotropica. 25:164–175.
- World Conservation and Monitoring Centre. 1992. Global biodiversity strategy. In: Groombridge B, editor. Global biodiversity: status of the earth’s living resources. London(UK): Chapman and Hall.