Abstract
Although mangroves are recognized by high capacity of carbon storage and sequestration, few studies have been dedicated to determine the monetary value of this ecosystem service. Accordingly, the aim of this study is to assign monetary values to this service in a protected area (Southeastern Brazil). This economic valuation was performed considering preexisting estimates of carbon storage and sequestration in the aboveground biomass of these forests and average transaction values of carbon credits. The mean values of the service of carbon sequestration varied according to the physiographic type from 19.00 ± 10.00 US$ ha−1 yr−1 (basin forests, high intertidal) to 82.28 ± 32.10 US$ ha−1 yr−1 (fringe forests, low intertidal). Considering the area occupied by each physiographic type, the service of carbon sequestration may be worth up to 455,827 US$ yr−1. In regard to carbon storage, 3,477,041 US$ are stored in these forests, and values between 104,311 and 208,622 US$ ha−1 yr−1 can be considered as the annual maintenance cost of this service. The income generated by future projects for the maintenance of carbon-related functions may represent a major advance for the conservation of this ecosystem.
1. Introduction
Mangroves are coastal forest ecosystems that occur in the sheltered intertidal zones of the tropical and subtropical regions of the world. They are globally recognized to be of extreme ecological, economic, social, and cultural importance because of the variety of goods and services they provide, reaching an estimated annual economic value of more than USD 900,000 km−2 (UNEP-WCMC Citation2006). Some of the most important goods and services provided by mangroves include the protection of the coastline from the energy of the winds and waves and the conservation of fishing and biodiversity in the coastal and adjacent estuary waters (Ewel et al. Citation1998; Mazda et al. Citation2005; Nagelkerken et al. Citation2008).
The increased emissions of greenhouse gases, in the recent decades, has enhanced the society’s perception of the social and economic damage that may be caused by climate changes, leading to an increasing interest in minimizing the potential impacts of these changes (Parry et al. Citation2007). In this sense, the large contribution of forest and wetland conversion to the global anthropogenic emissions of greenhouse gases (17% – van der Werf et al. Citation2009) draws attention to the need for their conservation and understanding of their role in carbon sequestration. In the case of mangroves, although there is still considerable uncertainty in the estimates of the carbon balance in this ecosystem (Bouillon et al. Citation2008), recent studies have shown their potential for carbon storage (Donato et al. Citation2011; McLeod et al. Citation2011). Thus, the function of carbon storage and sequestration adds another reason in favor of the conservation of mangroves. This appeal is even greater since climate change is intensifying (Parry et al. Citation2007) and international agreements are being signed to reduce and offset the emissions of greenhouse gases.
After the Kyoto Protocol, it has become possible for Certified Emission Reduction from Clean Development Mechanism (CDM) projects to originate from actions involving activities of land use, land use change, and forestry. These projects are proposed by companies in partnership with governments and non-governmental organizations in developing countries. However, thus far, only reforestation and afforestation activities are considered eligible, and conservation or forest management activities in natural systems are not included. Therefore, in the case of mangroves growing in protected areas, carbon sequestration rates related to tree growth over time cannot be converted into official carbon credits in the context of the Climate Convention, until the Reducing Emissions from Deforestation and Forest Degradation (REDD) methodology is included in the post-Kyoto period.
REDD’s intention is that developing countries may be financially rewarded for keeping carbon stored in their natural forests, preventing the economic growth of these countries to happen at the expense of environmental services promoted by such forests. Although unofficial, the contribution of REDD to the volume of tons of CO2 traded in voluntary carbon markets has increased over time (Diaz et al. Citation2011). These voluntary transactions are being carried out in an attempt to secure a market reserve after the regulation of REDD in the ambit of the Climate Convention. Diaz et al. (Citation2011) showed that in 2007, the volume of CO2e (sum of all greenhouse gas emissions equivalent to the potential contribution of CO2 to the greenhouse effect) negotiated from REDD projects was 1.2 million tCO2e, while in 2010, this value increased to 19.5 million tCO2e. This increase was not observed in afforestation and reforestation projects, which went from 3.5 million tCO2e in 2007 to 5.8 million tCO2e in 2010.
The aim of this study is to assign monetary values to the service provided by mangrove ecosystems regarding carbon storage and sequestration in a protected area, considering approaches related to the CDM and REDD.
2. Methods
2.1. Study area
The mangrove of the Guaratiba State Biological Reserve (), located in the Sepetiba Bay, southeast coast of Brazil (23.00°S, 43.57°W), was used as a case study. Almeida et al. (Citation2011) estimated the total area of mangroves in this protected area to be 4290 ha, with 3356 ha of mangrove forests and 934 ha of salt flats. Three typical mangrove species are found in the study area: Avicennia schaueriana, Laguncularia racemosa, and Rhizophora mangle. The climate in Guaratiba is defined by an average annual temperature of 23.5°C and average annual rainfall of 1067 mm, with the months of the highest rainfall between January and March and the driest months between June and August (Estrada et al. Citation2008). The tidal regime is microtidal with a range of less than 2 m.
Figure 1. Map of the study area (Biological Reserve of Guaratiba, SE-Brazil), indicating the distribution of mangrove forests, salt flats, and water bodies.
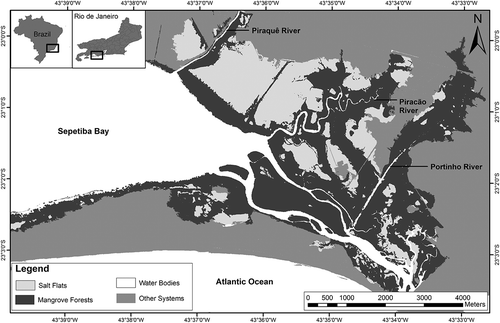
Because of the existence of an extensive coastal plain, the structure of the mangrove forests in Guaratiba varies according to the frequency of tidal flooding and to the relative position of the sources of continental drainage (river and groundwater). These factors enable the identification of three physiographic types: fringe (high frequency of tidal flooding); basin (intermediate-to-low frequency); and transition with salt flats, where mangroves develop a shrub growth form due to the low frequency of tidal flooding and the resulting high salinity (Estrada et al. Citation2013). According to these authors, such forests are characterized by a gradient of reduction of the structural development from the fringe to the transition forests (). In the same direction, interstitial water salinity increases () as a response to a gradually lower tidal flooding frequency. Among the species found in this region, R. mangle and A. schaueriana alternate as dominants in the fringe, basin, and transition forests, depending on the prevailing environmental conditions. The contribution of L. racemosa is considerably low, with the exception of some transition forests located upstream of the Piracão river.
Table 1. Mean (± standard deviation) structural parameters and mean interstitial water salinity of the mangrove forests of the Biological Reserve of Guaratiba (Rio de Janeiro, Brazil) per physiographic type. Data obtained from Estrada et al. (Citation2013) under permission.
2.2. Carbon storage and sequestration
To conduct this study, data on carbon storage and sequestration in the aboveground biomass (AGB) were obtained from Estrada (Citation2013). Since this reference is a PhD thesis in Portuguese, we present below a detailed summary of its methodology. Carbon storage was estimated from 94 plots (mean size of 250 m2), the same plots that were used by Estrada et al. (Citation2013) for structural characterization. Those plots were established along the gradients of tidal flooding frequency (see Estrada et al. Citation2013 for microtopography data) and distributed into 16 transects that extended from the margin of the estuary (low intertidal zone) to the salt flat (high intertidal zone). The plots were classified into physiographic types according to the height above mean sea level and the distance from the water body. At each plot, AGB was estimated from specific allometric models developed by Soares and Schaeffer-Novelli (Citation2005) and Estrada et al. (Citation2014). Those models were developed from regression analysis and estimate AGB of each tree from height, dbh (diameter at breast height, or 130 cm), and basal area. From the several types of allometric models presented by those authors for each species, the most precise and accurate ones were selected (lowest standard error of estimation – SEE; highest coefficient of determination R2) (). The AGB of the whole plot was obtained from the sum of the biomass of each tree. Carbon storage was then converted from AGB considering a carbon content of 45%, as suggested by Twilley et al. (Citation1992), and presented as tC ha−1. Means of carbon storage per physiographic type (Fringe = 31; Basin = 41; Transition = 22) were calculated considering the same classification of the plots presented by Estrada et al. (Citation2013).
Table 2. Allometric models used to estimate aboveground biomass (AGB, in grams) of live and dead trees and subsequent calculation of carbon storage and sequestration.
Carbon sequestration in the AGB was estimated in 30 of the 94 plots used for carbon storage (Fringe = 12; Basin = 12; Transition = 06). The permanent plots were monitored annually, between 2003 and 2012. Since height is more variable throughout the time and less precise than dbh, AGB estimation for carbon sequestration was restricted to allometric models that present dbh as the independent variable (). The rates of carbon sequestration were calculated from the increment of AGB resulting from growth and recruitment and presented as tC ha−1 year−1. Since two of the four authors we cite as a source for carbon sequestration monetary values use CO2 instead of C, we also present in the rates of carbon sequestration in tCO2 ha−1 year−1, which includes the atomic molecular mass of O2. The means of carbon storage and sequestration per physiographic type are presented in and .
Table 3. Monetary values of the service of carbon sequestration based on the average prices of carbon credits presented by Diaz et al. (Citation2011), Hamilton et al. (Citation2010), Medeiros et al. (Citation2011), and Kairo et al. (Citation2009).
Table 4. Monetary values of the service of carbon storage and annual cost of maintenance of the carbon storage (annual rental tax), based on the approach of Medeiros et al. (Citation2011).
2.3. Mangrove area estimation
In order to proceed with the valuation for the system as a whole, the values of carbon storage and sequestration were multiplied by the area (total and by physiographic type) of the study area. Area estimation had a global accuracy of 77% and was presented previously by Almeida et al. (Citation2011) and was based on the method of object-based classification, using eCognition software, IKONOS imagery (from 2002) and field data. The physiographic types were mapped considering both spectral differences (in response to structural characteristics) and distance from the estuary and salt flat. The areas determined by these authors were: total = 3356 ha; fringe = 305 ha; basin = 2470 ha; and transition = 582 ha.
2.4. Monetary valuation of carbon storage and sequestration
The monetary values for carbon storage and sequestration were calculated using two approaches: the first one took into account the carbon sequestration rates derived from the increment of AGB, following the reasoning applied to the CDM and proposed by Kairo et al. (Citation2009); and the second one took into account the conservation of carbon storage in the protected area, according to the reasoning applicable to REDD and proposed by Medeiros et al. (Citation2011).
The prices assigned for carbon or CO2 incorporated or maintained in the vegetation were obtained from Hamilton et al. (Citation2010) and Diaz et al. (Citation2011), who reviewed the global market for forest carbon, Kairo et al. (Citation2009), who studied mangrove forests plantations in Kenya, and Medeiros et al. (Citation2011), who analyzed the value of the carbon storage in the Brazilian conservation units.
Hamilton et al. (Citation2010), while performing an analysis on the global forest carbon market (taking as reference 226 forest projects in 40 countries) in voluntary and regular markets, observed that the prices for forest carbon credits ranged from US$ 0.65 tCO2e−1 to around US$ 50 tCO2e−1. In approximately 20 years, the weighted average of the prices of carbon, considering the volume of tons of CO2 in each project, was US$ 7.88 tCO2e−1. Diaz et al. (Citation2011) updated this analysis and found that the average price of offsets through the primary markets for forest carbon increased from US$ 3.80tCO2e−1 in 2008 to US$ 4.50tCO2e−1 in 2009 and US$ 5.50tCO2e−1 in 2010.
Because of the increasing importance of REDD, the market has established, as a fundamental requirement for a wide appreciation among buyers, that the projects should provide benefits for both biodiversity and local communities. Thus, the Climate, Community, and Biodiversity (CCB) certificate, conceived by the Climate, Community, and Biodiversity Alliance, a consortium of international non-governmental organizations, has been increasingly considered. The CCB certification standards do not generate tradable credits but assess social and environmental issues, and the generation of co-benefits for local communities.
Around 53% of all the projects that take into account co-benefits and were evaluated on the basis of the CCB standards also received the Verified Carbon Standard (VCS) certification (Diaz et al. Citation2011) that specifically assesses the carbon calculations and methodological-related issues. Hence, besides the average market value of US$ 5.50tCO2e−1 in 2010, for Guaratiba, the median price for the VCS standard (US$ 8.50tCO2e−1) was also used.
Kairo et al. (Citation2009) used the estimate published by Niles et al. (Citation2002) of US$ 10.00tC−1 for mangrove forest plantations in Kenya. This price was set by Niles et al. (Citation2002) as the default for the reforestation interventions of degraded areas in order to avoid deforestation and for sustainable agricultural practices.
Medeiros et al. (Citation2011) used the standard value of US$ 18.00tC−1, quoted as an average of the value of forest carbon transactions in the major global markets. In addition to the carbon storage value, annual rental taxes were also estimated for the current study. According to these authors, rental taxes should be considered for the compensation of the economic activities that could not be developed in the areas reserved for conservation due to legal restrictions; that is, it could be set from the opportunity cost of capital in real terms, discounted for inflation. The rental rates corresponded to 3 or 6% of the total carbon storage value and were also applied in the present study.
3. Results
The mean values of the service of carbon sequestration varied from 19.00 ± 10.00 US$ ha−1 yr−1 in the basin forests to 82.28 ± 32.10 US$ ha−1 yr−1 in the fringe forests (). Considering the total area occupied by each physiographic type in Guaratiba, we obtain values of up to 43,933 US$ yr−1 in the fringe forests; 315,666 US$ yr−1 in the basin forests; and 96,228 US$ yr−1 in the transition forests. Thus, the total value of the Guaratiba mangrove forests would be up to 455,827 US$ yr−1.
Regarding the value of carbon storage, we estimate that US$ 3.5 million are stored in the AGB of the Guaratiba mangrove forests and that between 104,000 and 209,000 US$ yr−1 would be the amount for the annual maintenance cost (or rental tax) of this storage ().
4. Discussion
The maximum values found for carbon sequestration rate in the three physiographic types in Guaratiba () were close to the value found by Kairo et al. (Citation2009) for a 12-year-old plantation of R. mucronata in Kenya (44.42 US$ ha−1 yr−1) if the monetary value per ton of sequestered carbon presented by the same authors was used. If the average values were considered, this proportion would decrease to 35–45% of the value of Kairo et al. (Citation2009). This difference could be explained, in part, by the lower age of the managed forests that were considered by these authors, since young forests tend to present higher productivity levels than mature forests (Kira & Shidei Citation1967; Whittaker & Woodwell Citation1967; Binkley et al. Citation2002). The fact that transition forests presented higher mean values of carbon sequestration (and thus higher monetary values for this service) than basin forests and higher maximum values than both fringe and basin forests despite being exposed to lower tidal flooding frequency and higher salinity (Estrada et al. Citation2013; ) is surprising and indicates that other factors not related to edaphic conditions may be driving this pattern. A possible explanation for this pattern is that transition forests are younger than basin and fringe forests and hence present higher productivity, as mentioned before. This hypothesis, which is partially supported by Estrada et al. (Citation2013), will be tested in future studies.
The economic value (US$ 3.5 million) of carbon storage in the 3356 ha of the Guaratiba mangrove forests would be higher if belowground biomass (roots) and soil were included. If the best estimates in the literature for the carbon storage in roots (54.95 tC ha−1: average of the values compiled by Komiyama et al. Citation2008) and soil (552.4 tC ha−1: global average for a depth of 1 m presented by Chmura et al. Citation2003) of mangroves are applied to the Guaratiba mangrove forests, the economic value for the maintenance of carbon storage would increase up to US$ 40,168,423.
However, it would still be possible to question whether the preservation of the Guaratiba mangroves would be economically viable in the face of other possibilities of use. In this regard, Siikamäki et al. (Citation2012) demonstrated that, up to a value of 10 US$ tCO2−1 (37 US$ tC−1), REDD projects in mangroves are economically viable in comparison with the reduction of emissions in the industrial and energy sectors, which determines the price of carbon credits of the European Union’s Emissions Trading System – EU ETS: between 10 and 20 US$ tCO2−1. Therefore, considering that the estimation of US$ 40 million for the Guaratiba mangroves was derived from an average carbon value of 18 US$ tC−1 (Medeiros et al. Citation2011), we could suggest that their conservation, only for the maintenance of the carbon storage, is economically viable. If we also consider that mangroves provide a wide variety of goods and services (Ewel et al. Citation1998; Mazda et al. Citation2005; Nagelkerken et al. Citation2008), the economic value of this ecosystem would be greater. Besides the values of direct or indirect use, the values of non-use, related to the very existence of the system and to the intention that it remains preserved as a legacy for future generations (Soares Citation2002), should also be acknowledged.
The protected areas of Brazil are managed by the municipal, state, or federal government and generally lack financial resources, which limits the ability to manage them. The Guaratiba State Biological Reserve is managed by the Rio de Janeiro State Government, through its Environmental Institute, but it has no resources of its own and a poor infrastructure, which translates into a low management capacity of the area. This limitation makes it highly vulnerable to urban expansion, since it is located in the metropolitan area of Rio de Janeiro, on the route of one of the main expansion areas of the city of Rio de Janeiro. Thus, the payment for the service of carbon storage and sequestration, directly and/or through the payment of annual rental taxes, could generate an annual revenue between 117,169 and 593,609 US$ yr−1 considering annual rental taxes and the monetary value of the service of carbon sequestration. Such resources could be applied on the improvement of the reserve management infrastructure, reducing the vulnerability to the impacts caused by urban expansion.
Even if raising financial resources for the conservation of the entire ecosystem seems beneficial, several authors (McAfee Citation1999; Igoe & Brockington Citation2007; Kosoy & Corbera Citation2010; Büscher et al. Citation2012; Corbera Citation2012) warn about the dangers of changing the logic of conservation by the commodification of certain ecosystem functions. The change consists of replacing the old ethical and inter-generational argument that nature needs to be managed and protected for the survival of ecosystems and species, with one that prioritizes some elements of nature that seem useful to humans. Assigning a monetary value to an ecosystem service heavily depends on scientific measurements and threatens to compromise other sources of valuation, such as those from local communities that depend directly on the forests. Thus, because of the power asymmetries, the valuation process may generate socio-environmental conflicts between those interested in carbon storage and the communities (Jindal Citation2004; De Paula & Morais Citation2012; Packer Citation2012), as described by Beymer-Farris and Bassett (Citation2012) for the mangrove forests in Tanzania. We thus position ourselves cautiously with respect to the market mechanisms and to the possibility of offsetting emissions. As suggested by Corbera (Citation2012), a global fund of ecological debt could be constituted where the developed countries finance the development with conservation in developing countries, as long as the latter develop their own environmental agendas and are not conditioned to the carbon market.
In the present study, monetary values were assigned to the service provided by the mangroves of Guaratiba regarding of carbon storage and sequestration, considering the reasoning of both CDM and REDD. The results showed that the economic values of this ecosystem service vary according to the physiographic type (fringe, basin, and transition forests) and to the area occupied by each physiographic type. Based on these results, it is highly recommended that future studies about the economic valuation of carbon storage in mangroves take into account the spatial variability of the system (e.g., different physiognomies, physiographic types, or successional stages). Since developing countries generally lack financial resources to sustain long-term environmental management actions, the income resulting from REDD or CDM projects could be an important way of improving the conservation status of mangroves.
Additional information
Funding
References
- Almeida PMM, Soares MLG, Estrada GCD, Fernandez V, Santos DMC, Machado MRO, Estevam MRM, Rodrigues DP. 2011. A aplicação de sistemas de informação geográfica no mapeamento de tipos fisiográficos de manguezais. Paper presented at: XIV Congresso Latino-Americano de Ciências do Mar; Balneário Camboriú-SC, Brazil.
- Beymer-Farris BA, Bassett TJ. 2012. The REDD menace: resurgent protectionism in Tanzania’s mangrove forests. Glob Environ Chang. 22:332–341.
- Binkley D, Stape JL, Ryan MG, Barnard HR, Fownes J. 2002. Age-related decline in forest ecosystem growth: an individual-tree, stand-structure hypothesis. Ecosystems. 5:58–67.
- Bouillon S, Borges AV, Castañeda-Moya E, Diele K, Dittmar T, Duke NC, Kristensen E, Lee SY, Marchand C, Middelburg JJ, et al. 2008. Mangrove production and carbon sinks: a revision of global budget estimates. Glob Biogeochem Cycles. 22:1–12.
- Büscher B, Sullivan S, Neves K, Igoe J, Brockington D. 2012. Towards a synthesized critique of neoliberal biodiversity conservation. Capital. Nat Social. 23:4–30.
- Chmura GL, Anisfeld SC, Cahoon DR, Lynch JC. 2003. Global carbon sequestration in tidal, saline wetland soils. Glob Biogeochem Cycles. 17:1–12.
- Corbera E. 2012. Problematizing REDD+ as an experiment in payments for ecosystem services. Curr Opin Environ Sustain. 4:612–619.
- De Paula EA, Morais MJ. 2012. O conflito está no ar: povos da Floresta e espoliação sob o capitalismo verde. Paper presented at: 36º Encontro Anual da ANPOCS. Resumos do 36º Encontro Anual da ANPOCS; Águas de Lindóia, Brazil.
- Diaz D, Hamilton K, Johnson E. 2011. State of the forest carbon markets 2011: from canopy to currency. Washington (DC): Ecosystem Marketplace/Forest Trends; p. 70.
- Donato DC, Kauffman JB, Murdiyarso D, Kurnianto S, Stidham M, Kanninen M. 2011. Mangroves among the most carbon-rich forests in the tropics. Nat Geosci. 4:293–297.
- Estrada GCD. 2013. Análise espaço-temporal do sequestro e do estoque de carbono na biomassa aérea de manguezais [Ph.D. thesis]. Rio de Janeiro (RJ): Programa de Pós-Graduação em Ecologia/Universidade Federal do Rio de Janeiro.
- Estrada GCD, Callado CH, Soares MLG, Lisi CS. 2008. Annual growth rings in the mangrove Laguncularia racemosa (Combretaceae). Trees. 22:663–670.
- Estrada GCD, Soares MLG, Chaves FO, Cavalcanti VF. 2013. Analysis of the structural variability of mangrove forests through the physiographic types approach. Aquat Bot. 111:135–143.
- Estrada GCD, Soares MLG, Santos DMC, Fernandez V, Almeida PMM, Estevam MRM, Machado MRO. 2014. Allometric models for aboveground biomass estimation of the mangrove Avicennia schaueriana. Hydrobiologia. 734:171–185.
- Ewel KC, Zheng S, Pinzón ZS, Bourgeois JA. 1998. Environmental effects of canopy gap formation in high – rainfall mangrove forests. Biotropica. 30:510–518.
- Hamilton K, Chokkalingam U, Bendana M. 2010. State of the Forest Carbon Markets 2009: taking Root & Branching Out. Washington (DC): Ecosystem Marketplace/Forest Trends; p. 70.
- Igoe J, Brockington D. 2007. Neoliberal conservation: a brief introduction. Conserv Soc. 5:534–561.
- Jindal R. 2004. Measuring the socio-economic impact of carbon sequestration on local communities: an assessment study with specific reference to the Nhambita pilot project in Mozambique [dissertation]. Edinburgh: Department of Resource Management, University of Edinburgh.
- Kairo JG, Wanjiru C, Ochiewo J. 2009. Net pay: economic analysis of a replanted mangrove plantation in Kenya. J Sustain For. 28:395–414.
- Kira T, Shidei T. 1967. Primary production and turnover of organic matter in different forest ecosystems of the Western Pacific. Jpn J Ecol. 17:70–87.
- Komiyama A, Ong JE, Poungparn S. 2008. Allometry, biomass, and productivity of mangrove forests: a review. Aquat Bot. 89:128–137.
- Kosoy N, Corbera E. 2010. Payments for ecosystem services as commodity fetishism. Ecol Econ. 69:1228–1236.
- Mazda Y, Kobashi D, Okada S. 2005. Tidal-scale hydrodynamics within mangrove swamps. Wetl Ecol Manag. 13:647–655.
- McAfee K. 1999. Selling nature to save it? Biodiversity and green developmentalism. Environ Plan Soc Space. 17:203–219.
- McLeod E, Chmura GL, Bouillon S, Salm R, Björk M, Duarte CM, Lovelock CE, Schlesinger WH, Silliman BR. 2011. A blueprint for blue carbon: toward an improved understanding of the role of vegetated coastal habitats in sequestering CO2. Front Ecol Environ. 9:552–560.
- Medeiros R, Young CEF, Pavese HB, Araújo FFS. 2011. Contribuição das unidades de conservação brasileiras para a economia nacional: sumário Executivo. Brasília DF, Brazil: United Nations Environment Program-World Conservation Monitoring Center; p. 44.
- Nagelkerken I, Blaber SJM, Bouillon S, Green P, Haywood M, Kirton LG, Meynecke JO, Pawlik J, Penrose HM, Sasekumar A, Somerfield PJ. 2008. The habitat function of mangroves for terrestrial and marine fauna: a review. Aquat Bot. 89:155–185.
- Niles JO, Brown S, Pretty J, Ball A, Fay J. 2002. Potential carbon mitigation and income in developing countries from changes in use and management of agricultural and forest lands. Phil Trans R Soc Lond A. 360:1621–1639.
- Packer L. 2012. Inside a champion. an analysis of the Brazilian development model. Publication series on democracy. Rio de Janeiro (RJ): Heinrich Böll Foundation. From Nature to Natural Capital. How New Legal and Financial Mechanisms Create a Market for the Green Economy; p. 114–128.
- Parry ML, Canziani OF, Palutikof JP, van der Linden PJ, Hanson CE. 2007. Climate change 2007: impacts, adaptation and vulnerability. Contribution of Working Group II to the fourth assessment report of the intergovernmental panel on climate change. Cambridge (UK): Cambridge University Press; p. 976.
- Siikamäki J, Sanchirico JN, Jardine SL. 2012. Global economic potential for reducing carbon dioxide emissions from mangrove loss. P Natl Acad Sci-Biol. 109:14369–14374.
- Soares MLG. 2002. Ética e Sustentabilidade. Rio de Janeiro (RJ): E-Papers Serviços Editoriais. Parte II, Ética e conservação da diversidade biológica; p. 99–132.
- Soares MLG, Schaeffer-Novelli Y. 2005. Above-ground biomass of mangrove species. I. Analysis of models. Estuar Coast Shelf Sci. 65:1–18.
- Twilley RR, Chen RH, Hargis T. 1992. Carbon sinks in mangroves and their implications to carbon budget of tropical coastal ecosystems. Water Air Soil Pollut. 64:265–288.
- [UNEP-WCMC] United Nations Environment Project-World Conservation Monitoring Centre. 2006. Shoreline protection and other ecosystem services from mangroves and coral reefs. Cambridge (UK): UNEP-WCMC; p. 33.
- van der Werf GR, Morton DC, De Fries RS, Olivier JGJ, Kasibhatla PS, Jackson RB, Collatz GJ, Randerson JT. 2009. CO2 emissions from forest loss. Nat Geosci. 2:737–738.
- Whittaker RH, Woodwell GM. 1967. Surface area relations of woody plants and forest communities. Am J Bot. 54:931–939.