ABSTRACT
The recent interest in multi-functional agricultural landscapes has not been matched with formal assessment of the roles that trees play across the spectrum of ecosystem services (ESs) provided in Sub-Saharan Africa (SSA). A structured literature review (1995–2014) assessed 350 journal articles about provision of one or more ESs by trees on farms and in agricultural landscapes in SSA. This revealed information on 15 ESs from studies in 23 countries covering arid (1% of studies), semi-arid (49%), sub-humid (26%) and humid (24%) agro-ecological zones. The majority of the studies reported provisioning (39%) and supporting (35%) followed by regulating (26%) ESs while studies on cultural services were scarce. Beneficial impacts of trees were dominating (58%), in particular in semi-arid zones where they were associated with enhancing water and nutrient cycling. A decline in some ESs was reported in 15% of the studies, while 28% found no effect of trees. Although the effects of trees were mainly positive, a decline in crop production was noted as a key trade-off against the provisions of ESs, such as modification of microclimate. This highlights the need to manage trade-offs among impacts of trees on ES provision to reduce competition and increase complementarity between trees and crops.
EDITED BY Christine Fürst
Introduction
Expansion and intensification of agricultural land is a major driver of the recent decline of biodiversity and ecosystem services (ESs) (Foley et al. Citation2005). This underscores the emerging value placed on multi-functional agricultural landscapes and their potential to supply multiple ESs (Tscharntke et al. Citation2011). For example, in addition to providing food and fibre, agricultural landscapes offer habitats for insects that deliver pollination and pest control services (Chaplin-Kramer & Kremen Citation2012; Morandin & Kremen Citation2013), and soil organisms that influence soil structure and nutrient cycling thus altering water and nutrient flows within the landscape (Barrios et al. Citation2012). A majority of the ESs in these landscapes are mediated by species diversity, including trees (Van Noordwijk et al. Citation2011).
ESs have particular relevance in Sub-Saharan Africa (SSA), where the majority of the human population dwell in rural areas and rely on ESs for their living through smallholder farming, pastoralism and fisheries (Egoh et al. Citation2012). Sub-Saharan Africa has high levels of poverty and diminishing ESs provided by natural and semi-natural habitats, e.g. due to deforestation. With increasing population, communities have been moving to marginal and fragile areas, and are especially vulnerable where regulating and supporting services get diminished, resulting in greater susceptibility to drought, pest and disease outbreaks or soil degradation (Boko et al. Citation2007). Enhanced ES provision is, therefore, critical for building resilience and improving food and nutrition security for smallholder farmers in SSA.
Trees are an integral part of many farming landscapes, where they provide a range of ESs that support livelihoods (Sinclair Citation1999). These trees are introduced into farms for particular reason(s), or are selectively retained after land use change. Farmers raise and manage a mix of exotic and indigenous trees in different ways to provide a variety of ESs (Nyaga et al. Citation2015). For example, leguminous trees grown for green manure or fodder can be managed in hedgerows to also stabilize soil against erosion and provide firewood (Angima et al. Citation2002; Mutegi et al. Citation2008). ESs provided by trees may be involved in both trade-offs (where some ESs increase while others decrease) and synergies (where multiple ESs are enhanced simultaneously) (Rodríguez et al. Citation2006; Raudsepp-Hearne et al. Citation2010). Trade-offs and synergies are caused by interactions among ESs (e.g. competition or complementarity) or simultaneous responses to the same driver(s) of ecosystem change (Bennett et al. Citation2009).
The recent surge of interest in ESs within agricultural landscapes requires formal assessment of the roles that trees play across the spectrum of ES provision now considered important in SSA. This review focuses on two related questions: (1) what is the scientific evidence base that integration of trees on farms and in agricultural landscapes contributes to enhanced ES provision for smallholder farmers in SSA, and which are the trade-offs?, (2) what quantitative studies are available, in which context and at which scale were they carried out, and what do they show? Whereas the importance of trees in provision of individual ES in SSA farms is widely studied; the question of which ESs increase or decrease when trees are incorporated in SSA agricultural landscapes has received less attention so far. Previous reviews and meta-analyses on the role of trees have focused on only one or a few selected ES at a time, for example impact of fertilizer trees on maize production (Sileshi et al. Citation2009; Akinnifesi et al. Citation2010) and the effect of agroforestry on pest control (Pumariño et al. Citation2015). This review aimed to (1) identify provisioning, supporting, regulating and cultural ESs of trees in agricultural landscapes in SSA; (2) determine the impacts of trees on ES provision and discuss how potential trade-offs can be managed; and (3) identify knowledge gaps that need to be further addressed by research.
A structured review with vote count was used to review, analyse and synthesize published data on the roles of trees in agricultural landscapes in order to quantify different ESs at field, farm and landscape scale in different agro-ecological (arid, semi-arid, sub-humid and humid) zones of SSA. This enabled us to capture the widest possible range of ESs.
Methods
Literature search and retrieval
The review process involved electronic search of material indexed in Web of Science and Scopus. A comprehensive list of ESs provided by trees was prepared from literature. The services were grouped into four categories following the millennium ecosystem assessment classification (MA Citation2005). Four search strings were set up retrieving documents indexed in Web of Science for ESs in each category (). This was supplemented by search on Google and Google scholar to identify other published articles and find web pages that might provide references. Published articles, theses, reports, conference proceedings and working papers were examined to identify potential peer reviewed publications that had arisen from the work, or presence of relevant citations.
Table 1. Search strategy used to retrieve publications from databases.
Identification of relevant studies
Studies retrieved were assessed for relevance using the title followed by the abstract and finally full text appraisal. Publications included in the study were those that satisfied the following criteria: (1) published peer reviewed journal article (for practical reasons limited to 20 years; 1995–2014), (2) study conducted on farms or in agricultural landscapes in SSA and (3) study reporting the ES provided or affected by trees (as compared to a non-tree treatment/situation).
Reviewer bias was reduced by having each of the 12 authors appraising a random sample of six publications. Consequently, about 20% of the studies were assessed by two authors to determine the repeatability of the selection criteria, the ES identified and the metadata obtained.
Summarizing the data
Each investigation that had been analysed separately was considered as an independent study. For example, if one paper presented results from the same experiment conducted at different locations and analysed separately, they were considered as two independent studies. A total of 361 peer-reviewed journal articles that reported 438 studies were reviewed (). Each study was categorized according to the FAO classification of agro-ecological zones (arid, semi-arid, sub-humid and humid).
Different types of information were extracted from each study reviewed: (1) where the study was conducted (region within SSA, country and name of the site and the geographic coordinates, agro-ecological zone); (2) how the study was conducted, including the design (experimental, observational or modelling), method of investigation (on-station or on-farm) and scale of investigation (field, farm, landscape); (3) number of ES reported, ES indicators and effect of trees on ES; (4) agroforestry practice, control treatment and the agroforestry components, such as tree species, crops and livestock. In addition, general information about the study (author, the year of publication and journal where the study was published), and biophysical characteristics of the site (climatic details, edaphic conditions, slope and altitude) were recorded.
A vote-count approach was used to determine the impact of trees on the ES, with results from each study being classified as either positive – meaning that the trees led to a significant increase in an ES; negative – that trees led to a significant decline in an ES; and neutral – where the influence of trees on an ES was not significant. The frequencies and proportions of studies with different effects of trees on a particular ES were calculated based on the overall number of studies reviewed.
Results
Distribution, context, design and spatial scale of studies
The review yielded 438 studies dispersed in 23 countries across SSA. The studies were carried out in arid (1%), semi-arid (49%), sub-humid (26%) and humid (24%) agro-ecological zones []. The majority of the studies have been conducted in East and West Africa followed by Southern Africa whereas very few studies were carried out in Central Africa. Over half of the studies were conducted in four countries: Kenya, Nigeria, Cameroon and Malawi []. A large proportion of the studies were from Kenya; evidence within the study that are specific to this country are identified so.
Figure 1. Distribution of studies in four agro-ecological zones: (a) the spatial representation of the studies on ecosystem services (ESs) across Sub-Saharan Africa, (b) the distribution of the studies on ESs in different countries. Studies in highlands are captured within the respective humid and sub-humid agro-ecological zones.
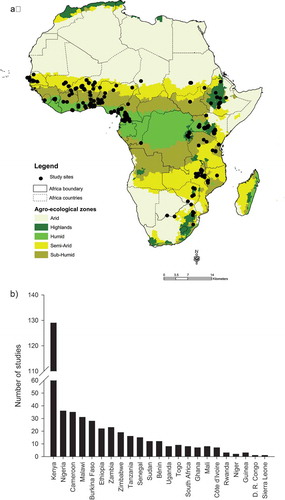
There was variation in the context, design and spatial scale at which research was conducted () with more than half of the studies conducted on-farm (57%) and the rest carried out on-station (43%). The majority of the reported studies were manipulative experiments (84%), followed by observational (15%) and modelling studies (1%). ESs in the studies reviewed were mainly documented at field scale (68%) and less at farm (23%) and landscape scale (9%).
ESs affected by trees
Fifteen ESs potentially affected by trees were reported in the reviewed studies. Provisioning ESs were the leading category reported (39%), followed by supporting (35%) and regulating ES (26%); studies on cultural ES provided by trees were scarce (). Over half of the studies reporting provisioning, regulating and supporting ESs were conducted in the semi-arid zone (). Fifty two percent of the studies focused on single ES; the rest (48%) reported data on multiple ESs – averaging three ES per study. The majority were examined as part of a broader investigation, indicating the possibility of assessing ESs using methods designed to address broader research questions.
Provisioning ESs were dominated by food provision (). Trees influence the production of food from crops and livestock; such effects are reported in the majority of the papers in this category. Some trees also produce fruits and nuts that can be used for human consumption, e.g. Adansonia digitata (baobab), Parkia biglobosa (African locust bean), Sclerocarya birrea (marula), Vitellaria paradoxa (shea tree) and Ziziphus jujuba (jujube red date). Five percent of the studies reviewed considered trees as providers of fodder (). A total of 49 fodder tree and shrub species were evaluated mainly for their nutritive value and effect on animal growth and milk production. Provisioning of green manure was reported in 4% of the studies; where tree foliage was carried from outside the cultivated fields and applied on crops to supply nutrients. Wood provision was reported in 3% of the studies and linked to fuel wood and timber use. Studies of trees that provided wood were mainly carried out in alleys (Aihou et al. Citation1998), improved fallows (Girma et al. Citation2006; Jama et al. Citation2008) and rotational woodlots (Kimaro et al. Citation2007, Nyadzi et al. 2003, Citation2008). Non-timber tree products, such as gum (gum arabic) and genetic resources were reported in few studies, 2% in total.
Regulating ES documented were directly linked to agricultural production and productivity. Water regulation was reported in 10% of the studies, highlighting the regulatory role of trees on water resources. The main indicators of water regulation documented were the effects of trees on soil moisture, infiltration rate and evapotranspiration (). Trees influenced pest control in 6% of the studies, with weed control being most commonly studied and evenly distributed across agro-ecological zones, with the exception of arid zones. The majority of studies in this category focused on Striga weeds (a parasitic plant genus mostly attacking cereals such as maize, millet and sorghum), e.g. Gacheru and Rao (Citation2005). Carbon storage was documented in 4% of the studies. Most of the studies highlighted carbon stored in the aboveground biomass; only few studies documented the sequestration potential of the trees belowground (e.g. in the roots and soil) or the carbon fluxes. Trees were reported to modify microclimate in 3% of the studies, through shading crops at field and farm scale, and as windbreaks or shelterbelts at landscape scale (Michels et al. Citation1998). Only nine studies (1%) reported the role of trees in regulation of soil erosion by slowing water flows and wind speed, holding the soil together with their roots and increasing infiltration.
Nutrient cycling (22%) and soil fertility improvement (14%) were the main supporting ESs documented, often evaluated alongside provisioning ESs. The trees mediated nutrient cycling by taking up inorganic nutrients (N, P, K, Mg, Ca, S and micronutrients) and cycling these to crops. Nutrient cycling was also mediated by application of un-decomposed fresh or wilted green material (green manure) from trees. Studies also explored the role of trees in rebuilding soil fertility by restoring soil organic matter (SOM), enhancing mineral nutrient concentrations to support crop productivity and restoring degraded lands (Barrios et al. Citation1996a, Citation1998).
ESs that increase and decrease with trees
Overall, beneficial effects of trees were evidenced in 58% of the studies reviewed while 15% reported a decline in ES and 28% found no effect of trees (). The results show that trees, while supplying one or more ES, in some cases negatively affected others, thus creating trade-offs (). In the following, we discuss the impacts of trees on six examples of ESs that were reported to increase or decrease because of trees and were represented in many studies: crop yield, water regulation, soil fertility, microclimate, nutrient cycling and regulation of pests. Whereas a higher proportion of positive effects of trees on crop yield, water regulation, nutrient cycling and soil fertility were reported in semi-arid zones, studies reporting positive influence of trees on pest regulation were mostly from humid zones.
Table 2. The effect of trees on provision of selected ESs in Sub-Saharan African agricultural landscapes.
Crop yield
Crop yields increased under tree-based systems compared to tree-less systems in 68% of the studies, decreased in 18% and were unaffected in 14% of the studies (). Crop yields increased due to improved microclimate, nutrient cycling and soil fertility under a variety of agroforestry technologies including: fallowing, tree–crop intercrop, alley cropping and biomass transfer (; see for a full list of agroforestry practices and other management options including trees that have been reported from the studies). High yields after improved fallows, for example, are mainly attributed to increased soil inorganic nitrogen (Barrios et al. Citation1997; Chirwa et al. Citation2004). Nitrogen-fixing shrubs and trees were the species that increased the yields of subsequent crops. Yield increase under inter-cropping systems largely depended on climatic conditions and management of trees (Boffa et al. Citation2000; Bayala et al. Citation2002, Citation2008). Yield increases after application of green manures and prunings of shrubs depended mainly on the mode and time of application (Egbe et al. Citation1998; George et al. Citation2001; Ganunga et al. Citation2005). A combination of green manure with inorganic fertilizer had the largest impact on maize yield (Akinnifesi et al. Citation2007, Citation2010).
Trees on farm can in some cases also lead to reduced yields by taking up land that would otherwise be used for crop production or by competing with crops for nutrients, water and light. The former was notable for green manure trees and fallowing, which is often associated with loss of a growing season and this will have especially large impact where there is only one short growing season (Bünemann et al. Citation2004). Yields can also be reduced if trees compete with the crops for nutrients, water and light; a phenomenon common when the density of trees is high (Everson et al. Citation2009), and in close proximity to crops (Jama et al. Citation1995, Citation2000; Boffa et al. Citation2000). Certain tree species are considered competitive and provide too much shading when grown together with crops, and this may reduce yields (Mathuva et al. Citation1998; Bayala et al. Citation2002; Gaafar et al. Citation2006). Such species, e.g. eucalyptus, have further been criticized because of their tendency to deplete water from surrounding crop lands (Kidanu et al. Citation2004; Gindaba et al. Citation2007). Even for trees that have been shown to be less competitive, crop yields could decrease during dry seasons or with older (15 years) trees (Fadl Citation2009; Fadl & Sheikh Citation2010) when competition for water resources increases (Chikowo et al. Citation2004b).
Microclimate
Microclimatic conditions (air and soil temperature, relative humidity, solar radiation, wind speed, etc.) were favourably altered in 61% and negatively altered in 39% of the studies; there was no report of this ES being unaffected (). Favourable alteration of microclimate resulted in increased growth and yield of crops due to reduced temperature and evapotranspiration, especially in semi-arid zones (Bayala et al. Citation2002). The majority of studies reported effects of trees on cereals, especially maize (a staple crop in several countries in SSA; Sileshi et al. Citation2009). Beneficial effects of moderate shading on growth and yields were also reported on a number of other crops, such as cocoa (Isaac et al. 2007), chilli pepper, millet (Kho et al. Citation2001) and coffee.
In studies where microclimatic conditions were reporting negative effects in tree–crop systems, it was mainly due to reduced photosynthetically active radiation in semi-arid zones (McIntyre et al. Citation1997; Ong et al. Citation2000; Bayala et al. Citation2002; Everson et al. Citation2009), higher relative humidity and lower air temperature compared to monoculture in sub-humid zones (Koech & Whitbread Citation2006; Schroth, Balle, et al. 1995). This resulted in reduced crop yields (McIntyre et al. Citation1997; Everson et al. Citation2009) and increased pest infestation (Koech & Whitbread Citation2006; Schroth, Balle, et al. 1995).
Water regulation
Trees regulate the amount of water available in the soil by controlling soil–plant–atmosphere water relations. Fifty one percent of the studies on water regulation reported a positive effect of trees, 35% found a negative effect and 14% showed no effect (). Soil water content was higher on farms with rather than without trees. This was attributed to increased infiltration rate (Chirwa et al. Citation2003, Citation2004; Makumba et al. Citation2006; Sanou et al. Citation2010; Nyamadzawo et al. Citation2012), reduced soil evaporation (Jackson and Wallace Citation1999) and reduced transpiration (Mungai et al. Citation2000) in semi-arid zones. Another positive effect of trees on water dynamics in semi-arid zones was hydraulic redistribution by some tree and shrub species (Burgess et al. Citation1998; Bayala et al. Citation2008; Kizito et al. Citation2012). However, in some cases trees in simultaneous agroforestry practices showed competition with crops for water resources, resulting in lower amounts of soil water close to trees than in continuous cropping or natural grass fallows, or at greater distances from trees (Hartemink et al. Citation1996; Odhiambo et al. Citation2001; Livesley et al. Citation2004). These studies attributed reduced soil water content to uptake of water by trees, increased transpiration from trees and possibly reduced input of rainfall through canopy interception. Continuous cropping can also lower infiltration rates due to soil compaction as a result of decreased SOM and degradation of soil structure. Depletion patterns in soil moisture content varied with depth in the soil and distance from the trees (Gathumbi et al. Citation2002; Livesley et al. Citation2004), and was influenced by factors, such as plant and soil characteristics and climatic conditions (Kidanu et al. Citation2004; Gaafar et al. Citation2006). Depletion of soil moisture increased with tree density but was reduced by pruning (Bayala et al. Citation2002; Gaafar et al. Citation2006). The impact of trees on soil water content was significant during the dry season when refill from rainfall did not compensate depletion (Nyadzi et al. 2003).
Regulation of pests
Trees were found to most often reduce weeds, insect pests and disease causing plant pathogens. Sixty-eight percent of the studies on pest control reported a positive influence of trees, 15% found a negative effect and 26% found no effect (). This result is in agreement with a global meta-analysis of the effect of agroforestry practices on pest control (Pumariño et al. Citation2015). The biomass of Striga and other arable weeds was reduced in tree-based systems compared with monoculture (Barrios et al. Citation1998; Sileshi & Mafongoya Citation2003; Gacheru & Rao Citation2005; Gworgwor Citation2007). The primary mechanisms by which trees reduced Striga infestation include a ‘trap crop effect’ that triggers suicidal germination of Striga, improvement of soil inorganic nitrogen and suppression of aboveground weed growth (Gacheru & Rao Citation2005). Trees also reduced the abundance, spread and damage caused by insect pests by modifying the microclimate, acting as barriers to movement of insects and harbouring natural enemies. For example, shade canopy reduced mirid (bug) density and the overall damage caused by insect pests in cocoa (Babin et al. Citation2010; Bisseleua et al. Citation2013). The role of trees in crop disease control has been less studied, but a number of studies in Kenya reported that disease control depended on microclimate and whether the tree species is a host plant or not (Desaeger & Rao Citation1999, Citation2000; Kandji et al. Citation2001, Citation2002; Schroth, Balle, et al. 1995).
Trees increased the infestations of insect pests and plant pathogens in some studies. For example improved fallows with tree species that are hosts of snout beetles increased snout beetle infestation of maize as compared to natural grass fallows or maize grown continuously in monoculture (Sileshi et al. Citation2000; Sileshi & Mafongoya Citation2003). In addition, higher levels of shade increased infestations by the white stem borer on coffee in eastern Uganda, probably due to a modified microclimate (Jonsson et al. Citation2014).
Nutrient cycling
Trees contribute to the flow of macro- and micro-nutrients in agricultural landscapes. Sixty percent of studies on nutrient cycling found a positive effect of trees, 8% found a negative effect while 33% found no effect (). Certain tree species increased nutrient cycling by retrieving sub-soil inorganic N and P from deep soils and cycling these to crops through decomposing biomass (Aweto & Iyanda Citation2003; Gindaba et al. Citation2005). Biological nitrogen fixation represented the most effective type of nutrient cycling commonly used to accumulate nitrogen in the soil. Crops grown in the presence of N-fixing tree legumes had significantly greater foliar N concentration than those grown in the absence of trees (Barrios et al. Citation1997; Chirwa et al. Citation2004; Akinnifesi et al. Citation2006; Chikowo et al. Citation2007). A total of 17 tree species evaluated in the studies are known to fix N from the atmosphere, and their litter and prunings contributed to N cycling either directly or via manure if used as fodder for livestock. The negative effects of trees on nutrient cycling in crops included sub-optimal K uptake (Isaac et al. Citation2007), low concentrations of leaf N (Hartemink et al. Citation1996, Citation2000; Sileshi et al. Citation2008) and low concentrations of foliar Zn and Mn (Lehmann et al. Citation1999) aggravated by competition from the trees.
Soil fertility
Fifty-three percent of the studies on soil fertility reported an increase in a range of soil fertility indicators, 9% reported a reduction while 38% showed no effects of trees on some aspects of soil fertility (). Trees improved soil fertility in cultivated land by increasing nutrient inputs from organic matter via litterfall and root decay (Barrios et al. Citation1997; Aihou et al. Citation1998; Ganunga et al. Citation2005). Other trees reduced loss of nutrients by reducing soil erosion in Kenya (Angima et al. Citation2000; Kinama et al. Citation2007) and improving soil physical properties in Zimbabwe (Mlambo et al. Citation2005). Specifically, legumes in alleys and fallows frequently increased SOM, SOC, inorganic-N, -K, plant-available P, exchangeable bases (Ca, K, Mg) and maintained higher soil pH than natural grass fallows or continuous maize cultivation (Barrios et al. Citation1996a, Citation1996b; Ikerra et al. Citation1999; Kang et al. Citation1999). Exceptions were reported in prolonged fallow periods with Gliricidia where the benefit was diminished (Kaya & Nair Citation2001). In parklands, the concentrations of SOC, SOM and nutrients, such as N, P and K were greater beneath tree canopies than on open ground between trees (Boffa et al. Citation2000; Kho et al. Citation2001). In these studies, the nutrient concentrations decreased with distance from tree stems and with soil depth, a pattern caused by nutrients accumulation resulting from greater organic inputs under tree canopies.
Trees sometimes reduced certain soil nutrients in relation to continuous cropping. Low concentrations of exchangeable bases (Ca, K and Mg) were reported particularly in alley cropping with leguminous trees (Kang et al. Citation1999; Schroth, Oliver, et al. 1995). Other parameters recorded in low quantities in agroforestry treatments as compared to cereal mono-cropping were total soil C and N, and plant available P (Olsen P) (Mathuva et al. Citation1998; Kang et al. Citation1999; Makumba et al. Citation2009). These cases of low concentration of nutrients in the soil were presumably because of nutrient uptake by trees (Isaac et al. Citation2007).
Biodiversity and ESs
The review shows that trees influence species diversity at field, farm and landscape scale, and that the effects of trees differ between taxa, arrangement of the trees in the landscape and land-use intensity. Biodiversity increased in 56% of the studies and decreased in 16%, while 28% found no effect (). The studies found that organisms were more abundant and species’ richness was greater in improved fallows and multi-strata agroforests than in monocultures (). In particular, the abundance, richness and overall diversity of soil organisms were positively influenced by presence of trees in the semi-arid zone (Adejuyigbe et al. Citation1999; Sileshi & Mafongoya Citation2003; Sileshi et al. Citation2008).
Table 3. Reported effects of trees on biodiversity in Sub-Saharan African agricultural landscapes.
Biodiversity was documented alongside an array of ESs provided by trees, such as food production, genetic resources, water regulation, microclimate, carbon storage, pest control, nutrient cycling and soil fertility. The general trend found was that increased belowground biodiversity positively co-varied with increased crop yield and improved soil fertility (Sileshi & Mafongoya Citation2006a; Sileshi et al. Citation2008). Increased aboveground biodiversity co-varied with increased fruit yield in multi-strata (cocoa) agroforests (Oke & Odebiyi Citation2007), increased infiltration rate (Sileshi & Mafongoya Citation2006b) and also increased pest control (Bisseleua et al. Citation2013). This indicates that biodiversity underpins supply of ES in agricultural landscapes through maintenance of ecological interactions (Barrios et al. Citation2012).
Discussion
Effects of trees on ESs in farms and agricultural landscapes
The review summarized 15 ESs provided by trees from studies conducted in farms and agricultural landscapes across SSA. Beneficial effects of trees were found in 58% of the studies reviewed while 15% reported a decline and 28% found no effect on ES. Thus, overall this review suggests that conserving and adding trees on farms and in agricultural landscapes of SSA is likely to increase the provision of multiple ESs. This is in line with other reviews that have shown that pest control is mostly improved under agroforestry practices (Pumariño et al. Citation2015), that legume tree species improve soil fertility (Jama et al. Citation2000; Akinnifesi et al. Citation2010) and increase maize yield (Jama et al. Citation2000; Sileshi et al. Citation2009), and that planting trees in agricultural fields increases infiltration capacity of the soils (Ilstedt et al. Citation2007). It is also in line with conclusions that trees can be a source of disservices depending on how they are managed; appropriate management practices are essential to enjoying the services and reducing the disservices from trees (Tscharntke et al. Citation2011).
This review identified a wide range of benefits of trees in agriculture. Most striking is the finding that crop yields increased under tree-based systems compared to tree-less systems in the majority of the reviewed studies (68%) and were unaffected in another 14%. Studies reporting the effect of trees on increased crop yields often also reported the role of trees on nutrient cycling and soil fertility (Makumba et al. Citation2006; Kimaro et al. Citation2008). Nutrient cycling and maintenance of soil fertility is essential in SSA where a large portion of the agricultural land is considered to be severely degraded (Boko et al. Citation2007). The studies also provided evidence of other benefits of trees, such as control of erosion, water regulation, modification of microclimate conditions, regulation of pests, as well as provision wood and non-wood tree products. The majority of the trees and shrubs providing these benefits were hedge-grown fodder species east Africa (Angima et al. Citation2002; Kinama et al. Citation2007; Mutegi et al. Citation2008), those dispersed in crop fields to provide shade (Bayala et al. Citation2002; Bisseleua et al. Citation2013), or in shelterbelts to modify microclimate and to check wind erosion (Michels et al. Citation1998; Onyewotu et al. Citation2004) in west Africa, and those planted in woodlots for wood (Kimaro et al. Citation2007; Nyadzi et al. 2003) in southern Africa.
The positive effects of trees on ES in agricultural landscapes were consistently largest in semi-arid areas. This includes enhanced nutrient cycling, improved soil fertility and improved microclimatic conditions, control of soil erosion and pest regulation under alley cropping (Mungai et al. Citation2000; Nyamadzawo et al. Citation2012), intercrops (Akinnifesi et al. Citation2007; Makumba et al. Citation2009) and improved fallows (Chikowo et al. Citation2004a; Sileshi et al. Citation2006). Biodiversity (abundance, richness and overall diversity of soil organisms) was positively influenced by presence of trees in the semi-arid zone (Adejuyigbe et al. Citation1999; Sileshi & Mafongoya Citation2006a; Sileshi et al. Citation2008). However, the inclusion of trees on farms in semi-arid zones requires adaptive management, since these areas not only have high-response potential but also risks of trade-offs.
Trade-offs of trees on farms and agricultural landscapes and how they can be reduced
A number of studies show that while trees affected some ES positively, they negatively affected others. Whether the trees can be integrated in the farming systems to promote only benefits or if they also introduce trade-offs depend on tree management as affected by the multi-functionality of tree species, their resource use efficiency and ability to favourably modify the microclimate for crops. Trade-offs associated with microclimate, water resources and nutrient depletion were found to be especially prevalent in alley cropping and for trees dispersed in cropped plots. These trees were introduced to reduce soil erosion, improve soil fertility and provide shade. However, abounding below- and above-ground competition for water, nutrients and light outweighed their benefit to crops in some environments.
The most common trade-offs of trees on farms were linked to microclimate and water regulation. Water depletion is a major problem in the semi-arid areas and particularly in dry seasons (Ong et al. Citation2000), while shading is a notable factor in reducing yield in parklands (Bayala et al. Citation2002). In dry regions or seasons, trees compete with crops for water resources through uptake and transpiration. This process balances with the positive effect of regulation of water by better infiltration due to trees and reduced soil evaporation (Ilstedt et al. Citation2007). The balance between these effects will be most pertinent in semi-arid environments where water-supply to crops is often the most limiting factor to crop growth. For example, while increase soil organic carbon resulted from the presence of trees in semi-arid Sudan, this was accompanied by a decline in soil moisture content and crop yield (Fadl Citation2009; Fadl & Sheikh Citation2010). Trees planted for green manure, wood or gum also lead to a decline in soil moisture content and crop yield in arid (Gaafar et al. Citation2006), semiarid (Mathuva et al. Citation1998) and sub-humid (Kidanu et al. Citation2004) areas. Conversely, improved soil fertility led to increased yields in the humid region despite a decline in soil moisture (Ikerra et al. Citation1999, Citation2006; Gathumbi et al. Citation2002). The magnitude and direction of the trade-offs between ES were influenced by the arrangement of trees in the system, their subsequent management, the tree species and the underlying climatic and edaphic factors.
Competition between trees and crops can be minimized by different management practices and selection of trees that are less competitive. Management options include increasing the spacing between trees and pruning the tree canopy to improve light penetration and to reduce the demand for water by trees or pruning of the root system to reduce water uptake. Several studies demonstrate the possibility to control water uptake and transpiration through pruning and regulating phenology (Livesley et al. Citation2004; Muthuri et al. Citation2004; Namirembe et al. Citation2009). A further benefit of tree pruning is that, if pruned material is left on the field, it adds organic matter and increase nutrient return to the soil (Mathuva et al. Citation1998; Livesley et al. Citation2002). Another measure to minimize competition between trees and crops is matching the agroforestry practice to the site. For example, crops on soils with low nitrogen content benefited from nitrogen-fixing trees, while adding legumes to soils where nitrogen was not limiting led to increases in nitrogen-based emissions while not increasing yields (Chikowo et al. Citation2004a, Citation2007). Exploitation of the complementarity between trees and crops has also been proposed on deep soils, particularly for deep-rooted tree species, which are able to utilize water and nutrients from below the crop-rooting zone (Gaafar et al. Citation2006). Deep-rooted species have reduced root volume near the surface, a feature that is good for minimizing competition with adjacent crops. Sequential arrangements, on the other hand, would be beneficial in shallow soils where trees are likely to compete with crops. Risks of increased pest problems can be minimized by ensuring that the trees are not important hosts or provide recourses for crop pests.
Geographical distribution of studies and knowledge gaps
The majority of ESs were reported in semi-arid zones. The relative occurrence of studies across the agro-ecological zones compares well with their proportional land area, except for the arid zones and inland humid areas where studies were rare. The lack of studies in the arid zone can be attributed to minimal agricultural production in most arid areas while humid inland areas of Central Africa are dominated by forests. The studies seem to aggregate around areas, which have experienced pressures on natural resources resulting in, for example, land degradation and where crop and livestock production are integrated. Pressures on natural resources can, for example, be attributed to increasing population density in these areas.
The role of trees in provision of wood, fruits and nuts and non-timber tree products, such as gum, genetic resources and medicine was underreported. The low number of studies on these ESs on farms and in agricultural landscapes indicates that the trends found in this review may not fully capture farmers’ practices. For example, wood is only cited in studies on trees planted in woodlot or alley hedgerows. In contrast, there are no studies on provision of wood from dispersed trees on cropland or pastures, boundary plantings, multi-story tree gardens or other agroforestry practices common for provision of timber and non-timber products in SSA. On-farm timber and non-timber tree products have increased recently following the decline in forest cover and increasing demand for these products (De Foresta et al. Citation2013). The small number of studies on non-timber tree products, thus, does not match to the widely acknowledged potentials, e.g. of genetic diversity to improve crop productivity, production of fruits and nuts and buffer against pests and diseases. However, it conforms to earlier reports that such ESs are not well documented, especially in Africa (Crossman et al. Citation2013; De Beenhouwer et al. Citation2013).
The review identified obvious lack of studies on cultural ESs provided by trees on farms in agricultural landscapes in SSA. Cultural services provided by trees seem to be understudied on farmland despite the fact that many cultural ESs from trees are documented to be produced in the wild (Egoh et al. Citation2012). People in agricultural landscapes obtain spiritual inspiration, cultural identity, recreation, etc., through interaction with trees. Certain trees, for example, are used as venues for learning and support experiences that uphold certain moral, religious and aesthetic values in many communities. Large trees are often used to provide shade and shelter for small businesses or for meetings and training events. These ESs are underreported, with virtually no quantitative data describing how trees in agricultural landscapes realize cultural needs. Recent reviews have attributed lack of information on cultural ESs to problems of definition, differences in aesthetic preferences and the slow integration of cultural services of trees within the ES framework (MA Citation2005; Daniel et al. Citation2012; Satz et al. Citation2013).
ESs are supplied at a range of spatial scales from field-level to landscape-level. However, ESs in the studies reviewed were mainly documented at field scale (68%) and less at farm (23%) and landscape scale (9%). This highlights a potential disparity in terms of the scale at which research was conducted in relation to the scale where a particular ES is actually produced and utilized. Mismatches between scales were noted in the provisioning (food, fodder and wood), which are generally utilized at farm-level but were mostly studied at field scale. This is in line with the literature indicating that studies on ESs are often conducted at a smaller scale than where they are produced and utilized (Balvanera et al. Citation2006). Another aspect of mismatch in scale is where the ES is produced at one scale, e.g. erosion control provided as a result of farmers’ work at field and farm scale, and benefits manifested at landscape level as less sedimentation in the river is experienced by communities or hydropower companies downstream in the watershed. It is in these situations that payment for environmental services can become a useful mechanism to link those producing ESs with the people utilizing them.
Conclusion
Trees on farms and in agricultural landscapes in SSA provide ESs that support food security, agricultural productivity and sustainable natural resource management, particularly in semi-arid environments. Research on supporting and regulating ES is often aimed at improving soil fertility and adjusting growing conditions in order to increase provisioning ES for improved crop production. While food production is important for food security and income, mainly focusing on provisioning ES risks failing to appreciate that ESs are interdependent. Longer-term trends and benefits can be missed when focusing on short-term gains.
Trade-offs were mainly observed between trees and provision of certain food crops, in particular during dry periods and in semi-arid zones, brought about by depletion of water resources, exhaustion of nutrients and unfavourable alteration of microclimatic conditions. It is not known whether the trade-offs observed are caused by interactions among ESs or simultaneous responses to the same driver(s) of ecosystem change. However, the trade-offs between ESs provided by trees and other farm enterprises (e.g. crops) can be minimized by management options that reduce competition and increase complementarity among crops and trees.
Very few studies on cultural ESs and a general underreporting of certain provisioning ES, such as wood, genetic resources and medicinal products in agricultural contexts indicate knowledge gaps. Data on provisioning ES were strongly biased towards staple food production, while information on provisioning of fruits and nuts is largely lacking. The lack of data on certain ESs provided by trees in agro-ecosystems makes it difficult to establish all the synergies and trade-offs associated with trees in the landscape. In addition, most studies on ESs are carried out at field scale although the potential benefits of the ESs are at farm or landscape scales. The poor representation of certain ESs in scientific studies and that effects of trees on ESs often are studied at a lower scale than where they are benefitted could furthermore impede policy development.
Acknowledgements
This study was supported by funds allocated to the Swedish University of Agricultural Sciences by the Swedish Ministry of Foreign Affairs as part of its special effort on global food security. Funding to EB to contribute to this chapter was provided by the CGIAR research programs on Forests, Trees and Agroforestry, and Humid Tropics.
Disclosure statement
No potential conflict of interest was reported by the authors.
Additional information
Funding
References
- Adejuyigbe CO, Tian G, Adeoye GO. 1999. Soil microarthropod populations under natural and planted fallows in southwestern Nigeria. Agroforestry Syst. 47:263–272.
- Aihou K, Sanginga N, Vanlauwe B, Lyasse O, Diels J, Merckx R. 1998. Alley cropping in the moist savanna of West-Africa: I. Restoration and maintenance of soil fertility on ‘terre de barre’ soils in Bénin Republic. Agroforestry Syst. 42:213–227.
- Akinnifesi FK, Ajayi OC, Sileshi G, Chirwa PW, Chianu J. 2010. Fertiliser trees for sustainable food security in the maize-based production systems of East and Southern Africa. A review. Agron Sustainable Dev. 30:615–629.
- Akinnifesi FK, Makumba W, Kwesiga F. 2006. Sustainable maize production using gliricidia/maize intercropping in southern Malawi. Exp Agric. 42:441–457.
- Akinnifesi FK, Makumba W, Sileshi G, Ajayi OC, Mweta D. 2007. Synergistic effect of inorganic N and P fertilizers and organic inputs from Gliricidia sepium on productivity of intercropped maize in Southern Malawi. Plant Soil. 294:203–217.
- Angima SD, O’Neill MK, Omwega AK, Stott DE. 2000. Use of tree/grass hedges for soil erosion control in the central Kenyan highlands. J Soil Water Conserv. 55:478–482.
- Angima SD, Stott DE, O’neill MK, Ong CK, Weesies GA. 2002. Use of calliandra-Napier grass contour hedges to control erosion in central Kenya. Agric Ecosyst Environ. 91:15–23.
- Aweto AO, Iyanda AO. 2003. Effects of Newbouldia laevis on soil subjected to shifting cultivation in the Ibadan area, south western Nigeria. Land Degrad Dev. 14:51–56.
- Babin R, Ten Hoopen GM, Cilas C, Enjalric F, Yede, Gendre P, Lumaret J-P. 2010. Impact of shade on the spatial distribution of Sahlbergella singularis in traditional cocoa agroforests. Agric For Entomol. 12:69–79.
- Balvanera P, Pfisterer AB, Buchmann N, He JS, Nakashizuka T, Raffaelli D, Schmid B. 2006. Quantifying the evidence for biodiversity effects on ecosystem functioning and services. Ecol Lett. 9:1146–1156.
- Barrios E, Buresh RJ, Kwesiga F, Sprent JI. 1997. Light fraction soil organic matter and available nitrogen following trees and maize. Soil Sci Soc Am J. 61:826–831.
- Barrios E, Buresh RJ, Sprent JI. 1996a. Nitrogen mineralization in density fractions of soil organic matter from maize and legume cropping systems. Soil Biol Biochem. 28:1459–1465.
- Barrios E, Buresh RJ, Sprent JI. 1996b. Organic matter in soil particle size and density fractions from maize and legume cropping systems. Soil Biol Biochem. 28:185–193.
- Barrios E, Kwesiga F, Buresh RJ, Coe R, Sprent JI. 1998. Relating preseason soil nitrogen to maize yield in tree legume-maize rotations. Soil Sci Soc Am J. 62:1604–1609.
- Barrios E, Sileshi GW, Shepherd KD, Sinclair F. 2012. Agroforestry and soil health: linking trees, soil biota and ecosystem services. In: Wall DH, Bardgett RD, Behan-Pelletier V, Herrick JE, Jones TH, Ritz K, Six J, Strong DR, van der Putten WH, editors. Soil ecology and ecosystem services. Oxford (UK): Oxford University Press; p. 315–330.
- Bayala J, Heng LK, Van Noordwijk M, Ouedraogo SJ. 2008. Hydraulic redistribution study in two native tree species of agroforestry parklands of West African dry savanna. Acta Oecologica. 34:370–378.
- Bayala J, Teklehaimanot Z, Ouedraogo SJ. 2002. Millet production under pruned tree crowns in a parkland system in Burkina Faso. Agroforestry Syst. 54:203–214.
- Bennett EM, Peterson GD, Gordon LJ. 2009. Understanding relationships among multiple ecosystem services. Ecol Lett. 12:1394–1404.
- Bisseleua BHB, Fotio D, Yede, Missoup AD, Vidal S, Hector A. 2013. Shade tree diversity, cocoa pest damage, yield compensating inputs and farmers’ net returns in West Africa. PLoS One. 8:e56115.
- Boffa J-M, Taonda SJ-B, Dickey JB, Knudson DM. 2000. Field-scale influence of karité (Vitellaria paradoxa) on sorghum production in the Sudan zone of Burkina Faso. Agroforestry Syst. 49:153–175.
- Boko M, Niang I, Nyong A, Vogel C, Githeko A, Medany M, Osman-Elasha B, Tabo R, Yanda P 2007. Africa. Climate change 2007: impacts, adaptation and vulnerability. In: Contribution of Working Group II to the Fourth Assessment Report of the Intergovernmental Panel on Climate Change. Cambridge (UK): Cambridge University Press; p. 433–467.
- Bünemann EK, Smithson PC, Jama B, Frossard E, Oberson A. 2004. Maize productivity and nutrient dynamics in maize-fallow rotations in western Kenya. Plant Soil. 264:195–208.
- Burgess SSO, Turner NC, Ong CK. 1998. The redistribution of soil water by tree root systems. Oecologia. 115:306–311.
- Chaplin-Kramer R, Kremen C. 2012. Pest control experiments show benefits of complexity at landscape and local scales.pdf>. Ecol Appl. 22:1936–1948.
- Chikowo R, Mapfumo P, Leffelaar PA, Giller KE. 2007. Integrating legumes to improve N cycling on smallholder farms in sub-humid Zimbabwe: resource quality, biophysical and environmental limitations. Nutr Cycl Agroecosyst. 76:219–231.
- Chikowo R, Mapfumo P, Nyamugafata P, Giller KE. 2004a. Mineral N dynamics, leaching and nitrous oxide losses under maize following two-year improved fallows on a sandy loam soil in Zimbabwe. Plant Soil. 259:315–330.
- Chikowo R, Mapfumo P, Nyamugafata P, Giller KE. 2004b. Woody legume fallow productivity, biological N2-fixation and residual benefits to two successive maize crops in Zimbabwe. Plant Soil. 262:303–315.
- Chirwa TS, Mafongoya PL, Chintu R. 2003. Mixed planted-fallows using coppicing and non-coppicing tree species for degraded Acrisols in eastern Zambia. Agroforestry Syst. 59:243–251.
- Chirwa TS, Mafongoya PL, Mbewe DNM, Chishala BH. 2004. Changes in soil properties and their effects on maize productivity following Sesbania sesban and Cajanus cajan improved fallow systems in eastern Zambia. Biol Fertil Soils. 40:20–27.
- Crossman ND, Burkhard B, Nedkov S, Willemen L, Petz K, Palomo I, Drakou EG, Martı´n-Lopez B, McPhearson T, Boyanova K, et al. 2013. A blueprint for mapping and modelling ecosystem services. Ecosyst Serv. 4:4–14.
- Daniel TC, Muhar A, Arnberger A, Aznar O, Boyd JW, Chan KM, Costanza R, Elmqvist T, Flint CG, Gobster PH, et al. 2012. Contributions of cultural services to the ecosystem services agenda. Pnas. 109:8812–8819.
- De Beenhouwer M, Aerts R, Honnay O. 2013. A global meta-analysis of the biodiversity and ecosystem service benefits of coffee and cacao agroforestry. Agric Ecosyst Environ. 175:1–7.
- De Foresta H, Somarriba E, Temu A, Boulanger D, Feuilly H, Gauthier M. 2013. Towards the assessment of trees outside forests. Resources Assessment Working Paper 183. Rome (Italy): FAO.
- Desaeger J, Rao MR. 1999. The root-knot nematode problem in sesbania fallows and scope for managing it in western Kenya. Agroforestry Syst. 47:273–288.
- Desaeger J, Rao MR. 2000. Parasitic nematode populations in natural fallows and improved cover crops and their effects on subsequent crops in Kenya. Field Crops Res. 65:41–56.
- Egbe EA, Ladipo DO, Nwoboshi LC, Swift MJ. 1998. Potentials of Millettia thonningii and Pterocarpus santalinoides for alley cropping in humid lowlands of West Africa. Agroforestry Syst. 40:309–321.
- Egoh BN, O’Farrell PJ, Charef A, Josephine Gurney L, Koellner T, Nibam Abi H, Egoh M, Willemen L.2012. An African account of ecosystem service provision: Use, threats and policy options for sustainable livelihoods. Ecosyst Serv. 2:71–81.
- Everson CS, Everson TM, Niekerk W. 2009. Soil water competition in a temperate hedgerow agroforestry system in South Africa. Agroforestry Syst. 75:211–221.
- Fadl KEM. 2009. Growth and yield of groundnut, sesame and roselle under Acacia senegal agroforestry system in North Kordofan, Sudan. Forests, Trees and Livelihoods. 19:27–46.
- Fadl KEM, Sheikh SE. 2010. Effect of Acacia senegal on growth and yield of groundnut, sesame and roselle in an agroforestry system in North Kordofan state, Sudan. Agroforestry Syst. 78:243–252.
- Foley JA, Defries R, Asner GP, Barford C, Bonan G, Carpenter SR, Chapin FS, Coe MT, Daily GC, Gibbs HK, et al. 2005. Global consequences of land use. Science. Jul 22;309:570–574.
- Gaafar AM, Salih AA, Luukkanen O, El Fadl MA, Kaarakka V. 2006. Improving the traditional Acacia senegal-crop system in Sudan: the effect of tree density on water use, gum production and crop yields. Agroforestry Syst. 66:1–11.
- Gacheru E, Rao MR. 2005. The potential of planted shrub fallows to combat Striga infestation on maize. Int J Pest Manag. 51:91–100.
- Ganunga RP, Yerokun OA, Kumwenda JDT. 2005. Contribution of Tithonia diversifolia to yield and nutrient uptake of maize in Malawian small-scale agriculture. South Afr J Plant Soil. 22:240–245.
- Gathumbi SM, Ndufa JK, Giller KE, Cadisch G. 2002. Do Species mixtures increase above- and belowground resource capture in woody and herbaceous tropical legumes? Agron J. 94:518–526.
- George TS, Gregory PJ, Robinson JS, Buresh RJ, Jama BA. 2001. Tithonia diversifolia: variations in leaf nutrient concentration and implications for biomass transfer. Agroforestry Syst. 52:199–205.
- Gindaba J, Rozanov A, Negash L. 2005. Trees on farms and their contribution to soil fertility parameters in Badessa, eastern Ethiopia. Biol Fertil Soils. 42:66–71.
- Gindaba J, Rozanov A, Negash L. 2007. Depletion of nutrients in adjacent crop landsby Eucalyptus camaldulensis. South Afr J Plant Soil. 24:47–50.
- Girma H, Rao MR, Day R, Ogol CKPO. 2006. Abundance of insect pests and their effects on biomass yields of single vs. multi-species planted fallows. Agroforestry Syst. 68:93–102.
- Gworgwor NA. 2007. Trees to control weeds in pearl millet. Agron Sustainable Dev. 27:89–94.
- Hartemink AE, Buresh RJ, Jama B, Janssen B. 1996. Soil nitrate and water dynamics in sesbania fallows, weed fallows, and maize. Soil Sci Soc Am J. 60:568–574.
- Hartemink AE, Buresh RJ, Van Bodegom PM, Braun AR, Jama B, Janssen B. 2000. Inorganic nitrogen dynamics in fallows and maize on an Oxisol and Alfisol in the highlands of Kenya. Geoderma. 98:11–33.
- Ikerra ST, Maghembe JA, Smithson PC, Buresh RJ. 1999. Soil nitrogen dynamics and relationships with maize yields in a gliricidia–maize intercrop in Malawi. Plant Soil. 211:155–164.
- Ikerra ST, Semu E, Mrema JP. 2006. Combining Tithonia diversifolia and minjingu phosphate rock for improvement of P availability and maize grain yields on a chromic acrisol in Morogoro, Tanzania. Nutr Cycl Agroecosyst. 76:249–260.
- Ilstedt U, Malmer A, Verbeeten E, Murdiyarso D. 2007. The effect of afforestation on water infiltration in the tropics: A systematic review and meta-analysis. For Ecol Manage. 251:45–51.
- Isaac ME, Ulzen-Appiah F, Timmer VR, Quashie-Sam J. 2007. Early growth and nutritional response to resource competition in cocoa-shade intercropped systems. Plant Soil. 298:243–254.
- Jackson NA, Wallace JS. 1999. Soil evaporation measurements in an agroforestry system in Kenya. Agricult Forest Meterol. 94:203–215.
- Jama B, Nair PKR, Rao MR. 1995. Productivity of hedgerow shrubs and maize under alleycropping and block planting systems in semiarid Kenya. Agroforestry Syst. 31:257–274.
- Jama B, Palm CA, Buresh RJ, Niang A, Gachengo CN, Nziguheba G, Amadalo BA. 2000. Tithonia diversifolia as a green manure for soil fertility improvement in western Kenya: A review. Agroforestry Syst. 49:201–221.
- Jama BA, Mutegi JK, Njui AN. 2008. Potential of improved fallows to increase household and regional fuelwood supply: evidence from western Kenya. Agroforestry Syst. 73:155–166.
- Jonsson M, Raphael IA, Ekbom B, Kyamanywa S, Karungi J. 2014. Contrasting effects of shade level and altitude on two important coffee pests. J Pest Sci. 88:281–287.
- Kandji ST, Ogol CKPO, Albrecht A. 2001. Diversity of plant-parasitic nematodes and their relationships with some soil physico-chemical characteristics in improved fallows in western Kenya. Appl Soil Ecol. 18:143–157.
- Kandji ST, Ogol CKPO, Albrecht A. 2002. Influence of some agroforestry practices on the temporal structures of nematodes in western Kenya. Eur J Soil Biol. 38:197–203.
- Kang BT, Caveness FE, Tian G, Kolawole GO. 1999. Longterm alley cropping with four hedgerow species on an Alfisol in southwestern Nigeria – effect on crop performance, soil chemical properties and nematode population. Nutr Cycl Agroecosyst. 54:145–155.
- Kaya B, Nair PKR. 2001. Soil fertility and crop yields under improved-fallow systems in southern Mali. Agroforestry Syst. 52:1–11.
- Kho RM, Yacouba B, Yayé M, Katkoré B, Moussa A, Iktam A, Mayaki A. 2001. Separating the effects of trees on crops: the case of Faidherbia albida and millet in Niger. Agroforestry Syst. 52:219–238.
- Kidanu S, Mamo T, Stroosnijder L. 2004. Eucalyptus–wheat interaction on Ethiopian Nitosols. Agric Syst. 80:151–170.
- Kimaro AA, Timmer VR, Chamshama SAO, Mugasha AG, Kimaro DA. 2008. Differential response to tree fallows in rotational woodlot systems in semi-arid Tanzania: post-fallow maize yield, nutrient uptake, and soil nutrients. Agric Ecosyst Environ. 125:73–83.
- Kimaro AA, Timmer VR, Mugasha AG, Chamshama SAO, Kimaro DA. 2007. Nutrient use efficiency and biomass production of tree species for rotational woodlot systems in semi-arid Morogoro, Tanzania. Agroforestry Syst. 71:175–184.
- Kinama JM, Stigter CJ, Ong C, Nganga JK, Gichuki FN. 2007. Contour hedgerows and grass strips in erosion and runoff control on sloping land in semi-arid Kenya. Arid Land Res Manag. 21:1–19.
- Kizito F, Dragila MI, Senè M, Brooks JR, Meinzer FC, Diedhiou I, Diouf M, Lufafa A, Dick RP, Selker J, et al. 2012. Hydraulic redistribution by two semi-arid shrub species: Implications for Sahelian agro-ecosystems. J Arid Environ. 83:69–77.
- Koech EK, Whitbread R. 2006. Incidence and severity of bean rust (Uromyces appendiculatus) in alleys between Leucaena hedgerows in Kenya. New Forests. 31:385–402.
- Lehmann J, Weigl D, Peter I, Droppelmann KJ, Gebauer G, Goldbach H, Zech W. 1999. Nutrient interactions of alley cropped Sorghum bicolor and Acacia saligna in a runoff irrigation system in Northern Kenya. Plant Soil. 210:249–262.
- Livesley SJ, Gregory PJ, Buresh RJ. 2002. Competition in tree row agroforestry systems. 2. Distribution, dynamics and uptake of soil inorganic N. Plant Soil. 247:177–187.
- Livesley SJ, Gregory PJ, Buresh RJ. 2004. Competition in tree row agroforestry systems. 3. Soil water distribution and dynamics. Plant Soil. 264:129–139.
- MA. 2005. Ecosystems and human well-being: synthesis. Washington (DC): Island Press.
- Makumba W, Akinnifesi F, Janssen B. 2009. Spatial rooting patterns of gliricidia, pigeon pea and maize intercrops and effect on profile soil N and P distribution in southern Malawi. Afr J Agric Res. 4:278–288.
- Makumba W, Janssen B, Oenema O, Akinnifesi F. 2006. Influence of time of application on the performance of gliricidia prunings as a source of n for maize. Exp Agric. 42:51–63.
- Mathuva MN, Rao MR, Smithson PC, Coe R. 1998. Improving maize (Zea mays)yields in semiarid highlands of Kenya: agroforestry or inorganic fertilizers? Field Crops Res. 55:57–72.
- McIntyre BD, Riha SJ, Ong CK. 1997. Competition for water in a hedge-intercrop system. Field Crops Res. 52:151–160.
- Michels K, Lamers JPA, Buerkert A. 1998. Effects of windbreak species and mulching on wind erosion and millet yield in the Sahel. Exp Agric. 34:449–464.
- Mlambo D, Nyathi P, Mapaure I. 2005. Influence of Colophospermum mopane on surface soil properties and understorey vegetation in a southern African savanna. For Ecol Manage. 212:394–404.
- Morandin LA, Kremen C. 2013. Hedgerow restoration promotes pollinator populations and exports native bees to adjacent fields. Ecol Appl. 23:829–839.
- Mungai DN, Stigter CJ, Coulson CL, Ng’ang’a JK. 2000. Simply obtained global radiation, soil temperature and soil moisture in an alley cropping system in semi-arid Kenya. Theor Appl Climatol. 65:63–78.
- Mutegi JK, Mugendi DN, Verchot L, Kung’u JB. 2008. Combining napier grass with leguminous shrubs in contour hedgerows controls soil erosion without competing with crops. Agroforestry Syst. 74:37–49.
- Muthuri C, Ong CK, Black CR, Mati BM, Ngumi VW, Van Noordwijk M. 2004. Modelling the effects of leafing phenology on growth and water use by selected agroforestry tree species in semi-arid Kenya. Land Use Water Resour Res. 4:1–11.
- Namirembe S, Brook RM, Ong CK. 2009. Manipulating phenology and water relations in Senna spectabilis in a water limited environment in Kenya. Agroforestry Syst. 75:197–210.
- Nyadzi GI, Janssen B, Otsyina RM, Booltink HWG, Ong CK, Oenema O. 2003. Water and nitrogen dynamics in rotational woodlots of five tree species in western Tanzania. Agroforestry Syst. 59:215–229.
- Nyaga J, Barrios E, Muthuri CW, Öborn I, Matiru V, Sinclair FL. 2015. Evaluating factors influencing heterogeneity in agroforestry adoption and practices within smallholder farms in Rift Valley, Kenya. Agric Ecosyst Environ. 212:106–118.
- Nyamadzawo G, Nyamugafata P, Wuta M, Nyamangara J, Chikowo R. 2012. Infiltration and runoff losses under fallowing and conservation agriculture practices on contrasting soils, Zimbabwe. Water SA. 38:233–240.
- Odhiambo HO, Deans JD, Wilson J, Khan AAH, Sprent JI. 2001. Roots, soil water and crop yield: tree crop interactions in a semi-arid agroforestry system in Kenya. Plant Soil. 235:221–233.
- Oke DO, Odebiyi KA. 2007. Traditional cocoa-based agroforestry and forest species conservation in Ondo State, Nigeria. Agric Ecosyst Environ. 122:305–311.
- Ong CK, Black CR, Wallace JS, Khan AAH, Lott JE, Jackson NA, Howard SB, Smith DM. 2000. Productivity, microclimate and water use in Grevillea robusta-based agroforestry systems on hillslopes in semi-arid Kenya. Agric Ecosyst Environ. 80:121–141.
- Onyewotu LOZ, Stigter CJ, Oladipo EO, Owonubi JJ. 2004. Air movement and its consequences around a multiple shelterbelt system under advective conditions in semi-arid Northern Nigeria. Theor Appl Climatol. 79:255–262.
- Pumariño L, Sileshi GW, Gripenberg S, Kaartinen R, Barrios E, Muchane MN, Midega C, Jonsson M. 2015. Effects of agroforestry on pest, disease and weed control: A meta-analysis. Basic Appl Ecol. 16:573–582.
- Raudsepp-Hearne C, Peterson GD, Bennett EM. 2010. Ecosystem service bundles for analyzing tradeoffs in diverse landscapes. Pnas. 107:5242–5247.
- Rodríguez JP, Beard JDT, Bennett EM, Cumming GS, Cork SJ, Agard J, Dobson AP, Peterson GD. 2006. Trade-offs across space, time, and ecosystem services. Ecol Soc. 11:28.
- Sanou J, Zougmoré R, Bayala J, Teklehaimanot Z. 2010. Soil infiltrability and water content as affected by Baobab (Adansonia digitata L.) and Néré (Parkia biglobosa (Jacq.) Benth.) trees in farmed parklands of West Africa. Soil Use Manage. 26:75–81.
- Satz D, Gould RK, Chan KM, Guerry A, Norton B, Satterfield T, Halpern BS, Levine J, Woodside U, Hannahs N, et al. 2013. The challenges of incorporating cultural ecosystem services into environmental assessment. Ambio. Oct;42:675–684.
- Schroth G, Balle P, Peltier R. 1995a. Alley cropping groundnut with Gliricidia sepium in Côte d’ Ivoire: effects on yields, microclimate and crop diseases. Agroforestry Syst. 29:147–163.
- Schroth G, Oliver R, Balle R, Gnahoua GM, Kanchanakanti N, Leduc B, Mallet B, Peltier R, Zech W. 1995b. Alley cropping with Gliricidia sepium on a high base status soil following forest clearing: effects on soil conditions, plant nutrition and crop yields. Agroforestry Syst. 32:261–276.
- Sileshi G, Akinnifesi F, Ajayi OC, Place F. 2009. Evidence for impact of green fertilizers on maize production in sub-Saharan Africa: a meta-analysis. Nairobi. (Kenya): World Agroforestry Centre.
- Sileshi G, Kuntashula E, Mafongoya P. 2006. Effects of improved fallows on weed infestation in maize in Eastern Zambia. Zambian J Agric Sci. 8:6–12.
- Sileshi G, Mafongoya P, Chintu R, Akinnifesi F. 2008. Mixed-species legume fallows affect faunal abundance and richness and N cycling compared to single species in maize-fallow rotations. Soil Biol Biochem. 40:3065–3075.
- Sileshi G, Mafongoya PL. 2003. Effect of rotational fallows on abundance of soil insects and weeds in maize crops in eastern Zambia. Appl Soil Ecol. 23:211–222.
- Sileshi G, Mafongoya PL. 2006a. Long-term effects of improved legume fallows on soil invertebrate macrofauna and maize yield in eastern Zambia. Agric Ecosyst Environ. 115:69–78.
- Sileshi G, Mafongoya PL. 2006b. Variation in macrofaunal communities under contrasting land use systems in eastern Zambia. Appl Soil Ecol. 33:49–60.
- Sileshi G, Maghembe JA, Rao MR, Ogol CKPO, Sithanantham S. 2000. Insects feeding on Sesbania species in natural stands and agroforestry systems in southern Malawi. Agroforestry Syst. 49:41–52.
- Sinclair F. 1999. A general classification of agroforestry practice. Agroforestry Syst. 46:161–180.
- Tscharntke T, Clough Y, Bhagwat SA, Buchori D, Faust H, Hertel D, Hölscher D, Juhrbandt J, Kessler M, Perfecto I, et al. 2011. Multifunctional shade-tree management in tropical agroforestry landscapes - a review. J Appl Ecol. 48:619–629.
- Van Noordwijk M, Ha Hoang M, Neufeldt H, Öborn I, Yatich T. 2011. How trees and people can co-adapt to climate change: reducing vulnerability in multifunctional landscapes. Nairobi (Kenya):World Agroforestry Centre (ICRAF).
Appendix
Table A1. Literature references reviewed, and on which the database is built. A total of 350 scientific papers that report ecosystem services provided by trees on farms and in agricultural landscapes of Sub-Saharan Africa. The papers refer to 205 sites dispersed in 23 countries across SSA. The database will be uploaded in Dataverse (https://dataverse.harvard.edu/dataverse/icraf).
Table A2. Indicators of ecosystem services (ESs) provided by trees in sub-Saharan African agricultural landscapes. The indicators refer to the unit of study for assessing the contributions of trees to ESs. Numbers indicate the number of studies reporting the indicator for ES affected by trees. The presence of trees resulted in either increase, decrease or no effects on the indicators of ESs; indicated in , and and .
Table A3. Agroforestry practices (and other management options including trees) that have been reported from studies in agricultural landscapes in sub-Saharan Africa. A short description of the practices is given. The effects of trees on ES provision and biodiversity are summarized in and .