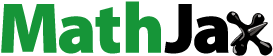
Abstract
The need for bone graft alternatives has led to the development of numerous bone graft substitutes. Here, the authors have synthesized a biodegradable poly(caprolactone-trifumarate) (PCLTF) polymer solution that could be injected into any bony defect. This polymer solution was synthesized using polycaprolactone-triol and fumaryl chloride (FCl). PCLTF is a multiple-branching, unsaturated and cross-linkable in situ material. The surface microstructure of PCLTF was investigated using a field emission scanning electron microscope. The incorporation of double bonds originating from FCl into the poly(caprolactone) backbone was confirmed in the Fourier transform infrared spectra. The in vitro cytotoxic effects of PCLTF, its leachable extracts and degradation products were evaluated in direct and indirect contact tests against human oral fibroblasts. Cell viability was evaluated using the microculture tetrazolium assay and cytotoxicity evaluations of PCLTF were tested in accordance with ISO 10993-5 standards. The results showed that there was evidence of reasonable cell growth, good cell viability and intact cell morphology after exposure to PCLTF, its extracts and degradation products. There was no evidence of critical cytotoxic effects.
Introduction
The extraction of teeth results in alveolar ridge resorption, with the buccal aspect of the ridge being affected more than the crestal region (Pietrokovski & Massler Citation1967; Devlin & Ferguson Citation1991). A systematic review reported that the alveolar ridge undergoes a mean 3.8 mm horizontal and 1.24 mm vertical reduction over 6 months (Lang et al. Citation2012). This amount of bone loss may significantly affect the amount of bone available for dental implant insertion, which is nowadays performed to replace missing teeth. To reduce bone loss and preserve bone volume, some authorities advocate alveolar ridge preservation (ARP) (Zubillaga et al. Citation2003).
Various ARP techniques have been described, using either autologous bone or bone substitutes (allogenic, xenogenic and synthetic grafts) with or without non-resorbable or resorbable barrier membranes (Douglass Citation2005; Vignoletti et al. Citation2012). Examples of bone graft materials are demineralized freeze-dried bone allograft (DFDBA) with or without calcium sulfate, corticocancellous porcine bone, bovine-derived hydroxyapatite, magnesium-enriched hydroxyapatite, calcium sulfate or medical-grade calcium sulfate hemihydrate, a biologically active silicate-based glass, collagen sponge and a sponge of polylactide–polyglycolide acid (Douglass Citation2005; Vignoletti et al. Citation2012). Most of these materials form a scaffold that preserves the socket volume to enable it eventually to be filled with naturally regenerated bone during the healing process. In general, most bone substitute scaffolds only have osteoconductive properties, but are not osteoinductive on their own. Another common problem with synthetic biomaterial such as calcium phosphate bioceramics, besides its poor mechanical properties, is that it has a slow biodegradation rate (Julien et al. Citation2007). However, even when using natural autologous bone grafts, some retention of non-vital grafted bone with the formation of connective tissue around it has been reported, which may slow down bone regeneration (Artzi et al. Citation2003).
Developing a biocompatible material as a scaffold and vehicle carrier for growth factors, which is the concept of tissue engineering, is very much desired. For example, in implant dentistry such a material can be used for ARP (Zubillaga et al. Citation2003) or to cover exposed implant surfaces (Chai Citation2009). These increased demands continue to fuel interest in improving and developing the performance of existing medical-grade polymers.
Researchers are studying biomaterials that are in an injectable form, so that they can be easily implanted in vivo and inserted into irregular osseous defects. This previously led to the development of the injectable bone cement poly(methy-methacrylate) (PMMA) (Temenoff & Mikos Citation2000). However, since this biomaterial is non-biodegradable, it is not suitable for insertion into extraction sockets as it would interfere with the eventual placement of dental implants. Therefore, a biodegradable cross-linkable and injectable scaffold would offer a great advantage in that no invasive surgery would be needed for either the application or the removal of the material.
An alternative material that can serve this purpose is polycaprolactone (PCL), an injectable and biodegradable polymer that is relatively inexpensive and has low toxicity (Kweon et al. Citation2003; Patel et al. Citation2011). This polymer consists of a highly elastic polyester structure that degrades by hydrolytic cleavage of its ester bonds in physiological conditions. Its degradation products are either metabolized via the tricarboxylic acid (Kreb's) cycle or eliminated via direct renal secretion (Woodward et al. Citation1985). However, this degradation process can take up to 2 years, which is a significant drawback clinically (Middleton & Tipton Citation2000). Various modifications, such as the addition of sebacic acid, have been reported to improve its degradation rate (Salgado et al. Citation2012). Similarly, PCL can be modified by cross-linking a functional group such as fumarate, resulting in the synthesis of polycaprolactone fumarate (PCLF) (Wang et al. Citation2009; Salgado et al. Citation2012). However, this semi-crystalline product needs to be heated to a high temperature before it can be injected into any bone defect site. As a result, necrosis or damage to the native tissue can occur (Jabbari et al. Citation2005).
To overcome these problems, the present research group has developed a resorbable polymeric solution based on PCL-triol and fumaryl chloride (FCl), named poly(caprolactone-trifumarate) (PCLTF), which may have great potential for use as a bone tissue engineering scaffold (Chai et al. Citation2006). FCl is a derivative of fumaric acid that is found in the body and is metabolized in the Krebs's cycle. PCLTF is an in situ cross-linkable polymer with multiple branching of unsaturated polyester, and contains extra non-reacted hydroxyl groups (–OH) that enhance its hydrophilicity. It has a higher degradation rate than PCL. It is a viscous liquid at room temperature and is therefore injectable without needing to be heated before application. Furthermore, because of its thick viscosity, migration of PCLTF from the injection site is prevented during the 15 min curing time. This injectable form of PCLTF has many advantages over other materials as it allows application as a bone-filling material directly into an irregular bony defect with minimal pressure.
In a previous study, this group developed two types of PCLTF, namely white and dark PCLTF scaffolds (Chai et al. Citation2004; Muhammad et al. Citation2012). Structurally, the two versions of PCLTFs are similar, with the only difference being that the opaque, dark PLCTF is produced as a result of the use of triethylamine (a catalyst used in the esterification reaction), whereas the use of potassium carbonate (K2CO3) in white PLCTF yields a translucent, white colour in the final product. The addition of K2CO3 shortens drastically the laboratory time needed to prepare the PCLTF polymer solution, from 48 h to 12 h. However, the application of K2CO3 results in polycondensation between PCL and FCl, which yields a higher molecular-weight copolymer compared with the use of triethylamine. As a result, white PCLTF has a longer degradation time than the dark polymer. Weighing up the advantages and disadvantages of these two versions of PCLTF, the authors decided to explore further the full potential of dark PCLTF for use as an extraction socket filler, because of its more favourable degradation time (Muhammad et al. Citation2012). Before this biomaterial is indicated for clinical use, it is crucial to ensure that dark PLCTF is not toxic to the body (Gomes et al. Citation2001). Therefore, it was the aim of this study to investigate the in vitro cytocompatibility of the biodegradable dark PCLTF.
Materials and methods
Sample preparation
The PCLTF polymer solution was synthesized using PCL-triol, FCl and triethylamine (Et3N), as described in a previous study (Chai et al. Citation2004). First, this mixture was stirred in an ice bath, and after 2 h, nitrogen gas was extracted. The stirring process was continued at room temperature for a total reaction time of 48 h. This process eventually formed a viscous PCLTF polymer solution. A PCLTF porous scaffold was then fabricated by first cross-linking the PCLTF polymer solution with a benzoyloxide radical, N-vinyl-2-pyrrolidone benzoyl peroxide (NVP-BP) solution, which acted as a cross-linking initiator. Following the addition of N,N-dimethyl-o-toluidine (DMT), which served as an accelerator, sodium chloride (NaCl) salt particles (sieved at 215–325 µm) were added as the porogen, finally making a porous material (Chai 2004). The resultant paste was injected into a cylindrical Teflon® mould (5 mm in diameter × 2 mm in height), which was then compressed using a glass plate to create a disc of even thickness. PCLTF cross-linked in situ and solidified within approximately 15 min. After cross-linking, the samples were removed from the moulds. The salt porogen was leached out by placing the scaffold in double-distilled water for 4 days, with water changes twice a day to prevent salt saturation. The resulting porous scaffolds were dried in a desiccator for 1 day and then sterilized in 70% ethanol overnight. Before being used in the experiments, the scaffolds were washed three times with phosphate-buffered saline (PBS).
Characterization of poly(caprolactone-trifumarate)
To characterize PCLTF, Fourier transform infrared (FTIR) spectroscopy (OMNIC 8.1 program, Nicolet 6700; Thermo Scientific, Madison, WI, USA) was employed using an internal reflection element made of diamond as the sample holder. The nuclear magnetic resonance (1H-NMR) spectra of PCL-triol and PCLTF were recorded in a 400 MHz spectrometer, using tetramethylsilane as the internal reference and dimethyl sulfoxide (DMSO) as the solvent.
The surface microstructure and the morphology of the test samples (scaffolds) were also investigated using a field emission scanning electron microscope (FESEM) (low vacuum operating mode; Quanta FEG 250; FEI, Eindhoven, the Netherlands). The test samples were dehydrated through a graded series of alcohol solutions, dried in a critical-point dryer and sputtered with a gold coater. They were viewed at an accelerating voltage of 10 kV.
Cytotoxicity tests
Following approval from the Medical Ethics Committee/IRB, Faculty of Dentistry, University of Malaya [DF OS1211/0071(P)], human gingival fibroblasts were harvested from marginal gingival tissue of extracted teeth, taken during routine orthodontic teeth extractions, and cultured for the purpose of evaluating the cytotoxic effect of PCLTF.
Cell culture was performed by expanding the fibroblasts in Dulbecco's modified Eagle's medium (LGC Scientific, Sigma, St Louis, MO, USA) supplemented with 10% (v/v) foetal bovine serum (Euroscience, Paa Austria) and 1% (v/v) antibiotics comprising of penicillin/streptomycin and amphotericin (Euroscience, PAA Austria, Fasching, Austria) in a humidified atmosphere at 37°C in 5% carbon dioxide (CO2). The confluent layer of cells obtained was designated as the ‘first passage cells’ after the cells reached 80–90% confluence. Oral fibroblasts with a passage number no greater than six were used in experiments. Cell viability was evaluated using the microculture tetrazolium (MTT) assay.
The optical density (OD) of the MTT assay is theoretically proportional to the growth rate and viability of the cells. Thus, the mean cell viability was determined by measuring the OD of the MTT assay. The percentage of cell viability was determined using the formula from the guidelines of the International Organization for Standardization (ISO) for biological evaluation of medical devices (ISO 10993-5) (ISO Citation2009):
where x is the absorbance in the test group (with PCLTF) and xc is the absorbance in the control group (without PCLTF). The tests were repeated at least three times to ensure reproducibility. Based on this reference, the cytotoxicity responses were qualitatively rated as severe, moderate, slight and non-cytotoxic when the percentage of cell viability was < 30%, 30–59%, 60–90% and > 90%, respectively (Loushine et al. Citation2011; Mukhtar-Fayyad Citation2011). Three replicate wells were used for each test and each test was repeated three times.
Direct contact cytotoxicity evaluation was preceded by seeding the human gingival oral fibroblasts on to the PCLTF discs at a density of 2 × 104 cells/cm2 in 300 µl/cm2 of culture medium in a six-well plate (Jabbari et al. Citation2005). A total volume of 1600 µl culture medium was used in each well. Cells plated with the same density but without exposure to PCLTF were used as the control. The cells were incubated at 37°C, 95% relative humidity and 5% CO2. The medium was changed once during the 72 h incubation period. The cells were incubated for 3 h in 300 µl/sample of MTT solution (2 mg/ml of thiazolyl blue tetrazolium bromide in PBS) (Sigma, St Louis, MO, USA). At the end of the incubation period, the MTT-containing medium was aspirated off and DMSO was added to dissolve the formazan crystal formed by living cells. An aliquot of 150 µl of the solution was transferred to a 96-well flat-bottomed plate, and the OD was measured with a microplate reader (Biotek ELx808 Absorbance Microplate Reader; Winooski, VT, USA).
Indirect cytotoxicity evaluations of PCLTF extract were tested in accordance with the ISO 10993-5 standards. In brief, the EtOH-sterilized discs were immersed in culture medium at a ratio of 1 ml of medium/3 cm2 surface area and incubated at 37°C, 95% relative humidity and 5% CO2. After a 24 h incubation period, the medium containing the extracts was diluted by 10 × and 100 × dilution with fresh culture medium for the indirect cytotoxicity test. Human gingival oral fibroblast culture was prepared by seeding the cells at a concentration of 1 × 104 cells/well in a 96-well plate. The cells were cultured to 80–90% confluency before the test. Once it reached the required confluency, the culture medium was replaced with 100 µl of one of three concentrations (undiluted, 10 × and 100 × diluted) of the extracts containing the medium, and incubated for 24 h, 48 h and 72 h. The cytotoxicity of extracts at each dilution was assessed by the MTT assay. Each viability measurement was normalized with the control group, where the fibroblasts were cultured in regular media without any extracts (Zhang et al. Citation2009).
The cytotoxicity test of the cross-linked PCLTF degradation products was carried out by bulk degradation of PCLTF and exposure of its degradation products to the cultured cells. The degradation process was carried out in a strong base at 37°C, which accelerated the ester hydrolysis reaction (Morrison & Boyd Citation1992; Zhang et al. Citation2009). In brief, a PCLTF sample (0.1 g) was placed in 10 ml 1 N NaOH solution and secured in a water bath at 37°C for 2 days. The sample was considered to have degraded completely when no solid material was visible in the solution. Then, the pH of the solution was adjusted to 7.4 using 1 N HCl and the solution was filtered through a cellulose acetate membrane filter (0.2 µm pore diameter). The degradation solution was then diluted in culture medium to 2 ×, 10 ×, 50 × and 100 × dilutions. Sterile PBS was diluted by the same factors and used as a negative control (Zhang et al. Citation2009).
Similarly to the test for the extraction products, confluent human gingival oral fibroblasts at a concentration of 1 × 104 cells/well in a 96-well plate were exposed to an aliquot of 100 µl of four different concentrations of degradation product-containing medium and incubated for 24 h. The viability of the cells was determined by the MTT assay as described above.
Data were analysed using Statistical Package for Social Science (SPSS) statistical software (Version 12.0; SPSS, Chicago, IL, USA). Descriptive statistics were applied where appropriate. The Mann–Whitney U test was performed for direct contact tests and the Kruskal–Wallis test for indirect cytotoxicity tests. The significance level was set at 95% (p < 0.05).
Results and discussion
The filling of an extraction socket with natural bone is the result of sequential events that begin with resorption of the socket walls, followed by recruitment and proliferation of osteoprogenitor cells from the surrounding tissues, osteoblastic differentiation, matrix formation and finally mineralization (Shin et al. Citation2003). Preservation of the alveolar ridge based on a tissue engineering approach usually involves the use of osteoconductive scaffolds. The authors' research group has developed a three-dimensional scaffold, which is a polymer solution based on PCL-triol and FCl (Chai et al. Citation2004). The resultant polymer solution was confirmed by the presence of C = C stretch and =C–H stretch at 1660–1600 cm−1 and around the region 3095–3010 cm−1, respectively, in FTIR spectra (Mahendra & Kadier Citation2012). PCLTF scaffolds fabricated from polymer solution were relatively hydrophobic, partly because of the presence of long hydrocarbon chains of PCL-triol. However, the presence of O–H stretch at a wave number of 3366.16 cm−1 indicated that the synthesized PCLTF polymer solution retained some hydroxyl groups (–OH) after reaction with FCl, hence giving some degree of hydrophilicity to the PCLTF polymer solution ().
Figure 1. Fourier transform infrared spectra of poly(caprolactone-trifumarate) (PCLTF) polymer solution: 1646.05 cm−1 (C=C stretch), 1732 cm−1 (C=O), 3400.35 cm−1 (O–H).
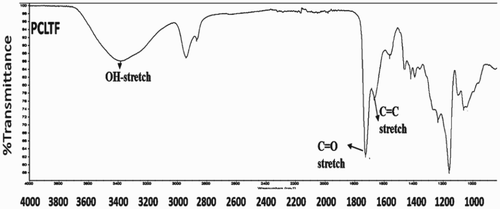
These findings were supported by the presence of a chemical shift value for the C = C–H group at 6.8 ppm relative to the tetramethylsilane (TMS) standard, which was not seen in the spectra of PCL-triol obtained by 1H-NMR (). This proved that the C = C–H group had been successfully incorporated and a PCLTF polymer solution had been synthesized.
Figure 2. 1H-Nuclear magnetic resonance spectra of the synthesized poly(caprolactone-trifumarate) (PCLTF) polymer solution: 6.8 ppm (CH = CH). PCL-triol = polycaprolactone-triol.
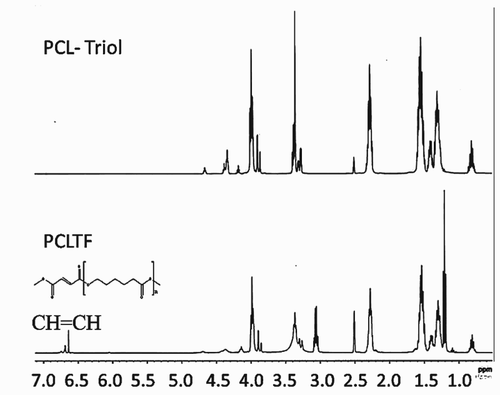
shows an FESEM micrograph of PCLTF porous scaffold. It shows the presence of pores with different shapes and sizes, ranging from 100 µm to 300 µm, and the presence of interconnections among the pores. The presence of these micropores in a scaffold improves mass transport and neovasculature formation in addition to assisting cell adhesion. To achieve this, maintaining a balance between the optimal pore size for cell migration and the specific surface area for cell attachment is essential. In agreement with the previous study (Chai et al. Citation2006), FESEM revealed that the PCLTF porous scaffolds contained pores of different sizes which were interconnected with each other. Furthermore, the weight loss of PCLTF demonstrated a higher degradation rate (30.86 ± 1.15%) than PCL (4.55 ± 0.26%) (Chai et al. Citation2004; Muhammad et al. Citation2012).
Figure 3. Field emission scanning electron microscope view of the surface microstructure and morphology of the porous poly(caprolactone-trifumarate) (PCLTF).
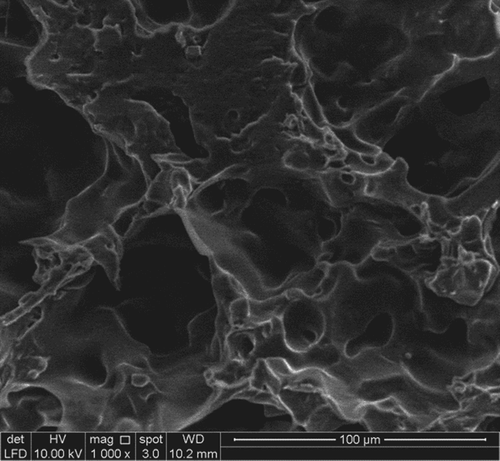
In a clinical situation, when a scaffold is placed in a dental extraction socket, the biomaterial will come into contact with the soft tissue (gingiva) before bone regeneration takes place in the socket. Because of this, human gingival fibroblasts were used to evaluate the cytocompatibility of PCLTF, its extracts and degradation products in this in vitro study. The effects of a material on cells are indicated by changes in cell morphology as well as the viability of cell growth. Cell viability can be tested using various biochemical assays, and within these different assays, changes in metabolic activity are good indicators of early cell viability (Davila et al. Citation1990). In this study, cell viability was determined using the MTT assay (Loushine et al. Citation2011).
For the direct contact test, cell metabolic activity in the MTT assay showed significantly different results between the PCLTF group and the control group (p < 0.05). The cell viability of PCLTF scaffold after a 72 h contact test was found to be 80.8 ± 2% which, according to the ISO 10993-5 standard, was considered to be slightly cytotoxic. Therefore, this biomaterial is deemed to be slightly cytotoxic, but it did not alter the normal morphological characteristics of spindle-shaped fibroblastic cells compared with the controls. This comparably normal morphological appearance of the cells, examined under phase-contrast microscope, was also seen in indirect contact tests ().
Figure 4. Phase-contrast micrograph showing the spindle-shaped fibroblastic morphology of A) (a) control and (b) poly(caprolactone-trifumarate) (PCLTF) disc treated cells (scale bar = 2 mm); B) extract products after cultivation at different time intervals; and C) degradation in different dilutions with cultivation for 24 h.
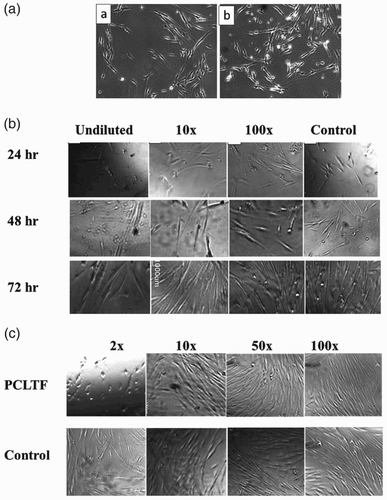
BP, NVP and DMT, which were used as cross-linking reagents, should not increase the toxicity risk of the fabricated PCLTF as these reagents were either consumed completely during the cross-linking reaction (as for BP) to form a non-toxic polymer (polyNVP), or present in a very small amount (as for DMT), where the toxic effects were negligible (Payne et al. Citation2002; Sharma et al. Citation2005; Patel et al. Citation2011). Because of this, the cytotoxic effect of the initiator could be excluded. The cytotoxicity of concern deals mainly with substances that leach out of the biomaterial and polymer. These substances often have low molecular weight, and often are additives and initiators that exhibit varying degrees of physiological activity and cell toxicity (Vajrabhaya & Sithisarn Citation1997). The slightly cytotoxic effect may be explained by the presence of salt particles that remained in the scaffold, similarly to the results reported by Kim et al. (Citation2009).
The indirect contact test of extracts evaluated the effect of the leachable product of PCLTF (Mukhtar-Fayyad Citation2011). Following ISO 10993-5 guidelines, the extracts of PCLTF in this study were obtained by incubating the specimens in culture medium at 37°C in a 5% CO2 incubator for 24 h. showed that the OD value increased gradually over time (24–72 h) in all concentrations of the extracts (p < 0.05). A significantly (p < 0.05) higher OD value, which reflected a higher cell viability, was observed in the more diluted group at 24 h, 48 h and 72 h. This observation suggests that the toxic effect reduced with dilution of the extracts. In addition, the most concentrated level of extract medium (undiluted extract) had the lowest percentage of cell viability (51.6 ± 4%), which is considered moderately cytotoxic according to the ISO 10993-5 standard. These results show that the extracts of PCLTF had an acceptable degree of biocompatibility, and the most significant cytotoxic effect was seen during the first 24 h. With time (at 48–72 h), there was evidence of continuing cell growth in all three concentrations of extracts. Although the cytotoxic effect of the undiluted extracts was moderate initially, in a clinical condition, it may be even lower as the extracts would be diluted by the surrounding tissue fluid. In addition, during the cross-linking process of the polymer, the constituents continued to integrate into the network, resulting in less diffusion of leachable products out of the network. Based on the aforementioned factors, although the initial cytotoxicity of the extract was moderate, with time, the cytotoxicity is expected to reduce gradually (Schmalz Citation1994; Gomes et al. Citation2001; Bae et al. Citation2010).
Figure 5. Results of the indirect contact test of the poly(caprolactone-trifumarate) (PCLTF) extracts. The mean value for the optical density (OD) of the microculture tetrazolium assay shows cell viability of the oral fibroblasts after the exposure in the extracts over a period of 24 h, 48 h and 72 h. #The mean cell viability of the 10×, 100× and control groups was significantly higher than the undiluted group (p < 0.05). *The mean cell viability of the 100× and control groups was significant higher than the 10× group (p < 0.05). ‡ The mean cell viability of the control was significantly higher than the 100× group (p < 0.05).
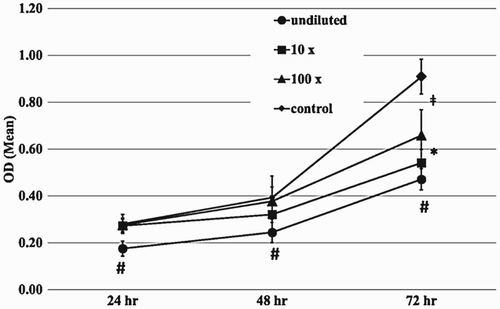
Scaffolds for bone regeneration usually degrade on a relatively long time-scale, but study of their long-term biocompatibility is difficult. The authors noticed that in vitro, PCLTF took months to gradually degrade in PBS. In this study, an accelerated degradation method was used to completely break down the networks, yielding the maximum release of degradation products, by immersing PCLTF in a strong base at 37°C. These conditions accelerate the ester hydrolysis reaction, which is more advantageous than using a diluted acid to achieve the same result. In addition, this reaction is an irreversible process. While these conditions do not exactly replicate the actual changes in the polymer in vivo and may not correlate with actual in vivo degradation time, they do simulate the physical changes expected during natural degradation on a shortened time-scale. Four different dilution factors were chosen in this study to represent a range of concentrations of the degradation product (Zhang et al. Citation2009). The degradation products from PCLTF displayed a dose-dependent cytotoxic effect. The results showed that the cell viability percentage of the fibroblasts was the lowest in a 2 × dilution of the degradation products (p < 0.05), which was considered as having moderate cytotoxicity. However, in a more diluted concentration of the degradation products, the cell viability percentage increased to 94.1 ± 11%, 96.4 ± 8% and 96.6 ± 17% at 10 ×, 50 × and 100 × dilution, respectively (), suggesting non-cytotoxic effects in the diluted conditions. Thus, the cytotoxicity effect gradually decreased when the concentrations of the degradation product were diluted.
Figure 6. Results of the indirect contact test of the poly(caprolactone-trifumarate) (PCLTF) degradation product showing the cell viability percentage after its exposure to oral fibroblasts over a period of 24 h. The cell viability percentage of the 10×, 50× and 100× diluted degradation products was significantly higher than the 2× dilution group (p < 0.05).
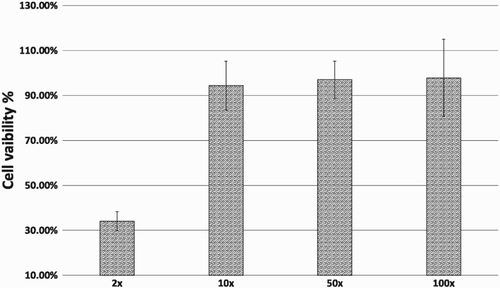
In this study, although the results showed moderate cytotoxicity of the undiluted extract and degradation products, one should understand that the results obtained in in vitro culture conditions are different from those in the in vivo homeostatic condition. Compared to the in vivo model, in vitro experiments like this one have a lack of defined mechanisms, which has a strong impact on the precision of toxicity elimination. One example of such a mechanism is the presence of the lymphatic system to help in the elimination of toxic substances (Hartung & Daston Citation2009; Loushine et al. Citation2011).
In the previous study, there was evidence of osteoblast growth, proliferation and differentiation, as well as expression of the osteogenic marker alkaline phosphatase and mineralization on the PCLTF (Chai et al. Citation2006). Within the limitations of this study, the direct contact test of PCLTF showed slight cytotoxicity. However, the indirect contact tests of the extract and degradation products showed moderate cytotoxicity initially, which reduced when diluted. Based on this early encouraging result, the potential of PCLTF for use as a bone socket filler could be further investigated in animal studies.
Conclusion
The biocompatibility of this injectable scaffold showed that the cytotoxic effect of PCLTF and its extracts, as well as its degradation products, were moderate initially, and reduced when diluted as well as with time. Within the limitations of the present study, it may be concluded that there was no critical cytotoxic effect of dark PCLTF for use as a scaffold for tissue engineering. Further preclinical studies are required to investigate the use of PCLTF as an injectable scaffold.
Disclosure statement
The authors declare that they have no conflict of interest.
Additional information
Funding
References
- Artzi Z, Givol N, Rohrer MD, Nemcovsky CE, Prasad HS, Tal H. 2003. Qualitative and quantitative expression of bovine bone mineral in experimental bone defects. Part 2: Morphometric analysis. J Periodontol. 74:1153–1160. doi: https://doi.org/10.1902/jop.2003.74.8.1153
- Bae WJ, Chang SW, Lee SI, Kum KY, Bae KS, Kim EC. 2010. Human periodontal ligament cell response to a newly developed calcium phosphate-based root canal sealer. J Endod. 36:1658–1663. doi: https://doi.org/10.1016/j.joen.2010.06.022
- Chai W. 2009. A systematic review on the management of osseous defects during dental implant placement. Ann Dent Univ Malaya. 16: 24–30.
- Chai YC, Abas WABW, Kim KH. 2004. Development and characterisation of a novel poly(caprolactone-trifumarate) macromer as scaffold for bone tissue engineering. Int Fed Med Biol Eng Proc. 323:359–362.
- Chai YC, Abas WABW, Kim KH. 2006. Osteogenic expression of bone marrow stromal cells on PCLTF scaffold: in vitro study. Asian Journal of Cell Biology. 1:40–47. doi: https://doi.org/10.3923/ajcb.2006.40.47
- Davila JC, Reddy CG, Davis PJ, Acosta D. 1990. Toxicity assessment of paraverine hydrochloride and papaverine-derived metabolites in primary cultures of rat hepatocytes. In Vitro Cell Dev Biol. 26:515–524. doi: https://doi.org/10.1007/BF02624095
- Devlin H, Ferguson M. 1991. Alveolar ridge resorption and mandibular atrophy. A review of the role of local and systemic factors. Br Dent J. 170:101–104. doi: https://doi.org/10.1038/sj.bdj.4807427
- Douglass GL. 2005. Alveolar ridge preservation at tooth extraction. J Calif Dent Assoc. 33:223–231.
- Gomes M, Reis R, Cunha A, Blitterswijk CA, De Bruijn J. 2001. Cytocompatibility and response of osteoblastic-like cells to starch-based polymers: effect of several additives and processing conditions. Biomaterials. 22:1911–1917. doi: https://doi.org/10.1016/S0142-9612(00)00377-X
- Hartung T, Daston G. 2009. Are in vitro tests suitable for regulatory use? Toxicol Sci. 111:233–237. doi: https://doi.org/10.1093/toxsci/kfp149
- ISO. 2009. Biological evaluation of medical devices. V. In: Tests for cytotoxicity: In vitro methodsIn: Iso, editor Vol10993-5 Geneva: International Organization for Standardization.
- Jabbari E, Wang S, Lu L, Gruetzmacher JA, Ameenuddin S, Hefferan TE, Currier BL, Windebank AJ, Yaszemski MJ. 2005. Synthesis, material properties, and biocompatibility of a novel self-cross-linkable poly (caprolactone fumarate) as an injectable tissue engineering scaffold. Biomacromolecules. 6:2503–2511. doi: https://doi.org/10.1021/bm050206y
- Julien M, Khairoun I, LeGeros RZ, Delplace S, Pilet P, Weiss P, Daculsi G, Bouler JM, Guicheux J. 2007. Physico-chemical-mechanical and in vitro biological properties of calcium phosphate cements with doped amorphous calcium phosphates. Biomaterials. 28:956–965. doi: https://doi.org/10.1016/j.biomaterials.2006.10.018
- Kim J, Yaszemski MJ, Lu L. 2009. Three-dimensional porous biodegradable polymeric scaffolds fabricated with biodegradable hydrogel porogens. Tissue Eng Pt C-Meth. 15:583–594. doi: https://doi.org/10.1089/ten.tec.2008.0642
- Kweon HY, Yoo MK, Park IK, Kim TH, Lee HC, Lee HS, Oh JS, Akaike T, Cho CS. 2003. A novel degradable polycaprolactone networks for tissue engineering. Biomaterials. 24:801–808. doi: https://doi.org/10.1016/S0142-9612(02)00370-8
- Lang NP, Pun L, Lau KY, Li KY, Wong M. 2012. A systematic review on survival and success rates of implants placed immediately into fresh extraction sockets after at least 1 year. Clin Oral Implants Res. 23:39–66. doi: https://doi.org/10.1111/j.1600-0501.2011.02372.x
- Loushine BA, Bryan TE, Looney SW, Gillen BM, Loushine RJ, Weller RN, Pashley DH, Tay FR. 2011. Setting properties and cytotoxicity evaluation of a premixed bioceramic root canal sealer. J Endod. 37:673–677. doi: https://doi.org/10.1016/j.joen.2011.01.003
- Mahendra P, Kadier H. 2012. Interpretation of structure in organic chemistry miscellaneous technique IJPRBS. pp. 145–157.
- Middleton JC, Tipton AJ. 2000. Synthetic biodegradable polymers as orthopedic devices. Biomaterials. 21:2335–2346. doi: https://doi.org/10.1016/S0142-9612(00)00101-0
- Morrison R, Boyd R. 1992. Organic chemistry. 6th ed. Englewood Cliffs, NJ: Prentice Hall.
- Muhammad KB, Abas WABW, Kim KH, Pingguan-Murphy B, Zain NM, AkramI H. 2012. In vitro comparative study of white and dark polycaprolactone trifumarate in situ cross-linkable scaffolds seeded with rat bone marrow stromal cells. Clinics. 67:629–637. doi: https://doi.org/10.6061/clinics/2012(06)14
- Mukhtar-Fayyad D. 2011. Cytocompatibility of new bioceramic-based materials on human fibroblast cells (MRC-5). Oral Surg Oral Med Oral Pathol Oral Radiol Endod. 112:e137–142. doi: https://doi.org/10.1016/j.tripleo.2011.05.042
- Patel H, Bonde M, Srinivasan G. 2011. Biodegradable polymer scaffold for tissue engineering. Trends Biomater Artif Organs. 25:20–29.
- Payne RG, McGonigle JS, Yaszemski MJ, Yasko AW, Mikos AG. 2002. Development of an injectable, in situ crosslinkable, degradable polymeric carrier for osteogenic cell populations. Part 2. Viability of encapsulated marrow stromal osteoblasts cultured on crosslinking poly (propylene fumarate). Biomaterials. 23:4373–4380. doi: https://doi.org/10.1016/S0142-9612(02)00185-0
- Pietrokovski J, Massler M. 1967. Alveolar ridge resorption following tooth extraction. J Prosthet Dent. 17:21–27. Epub 1967/01/01. doi: https://doi.org/10.1016/0022-3913(67)90046-7
- Salgado CL, Sanchez E, Zavaglia CAC, Granja PL. 2012. Biocompatibility and biodegradation of polycaprolactone-sebacic acid blended gels. J Biomed Mater Res A. 100:243–251. doi: https://doi.org/10.1002/jbm.a.33272
- Schmalz G. 1994. Use of cell cultures for toxicity testing of dental materials – advantages and limitations. J Dent. 22:S6–S11. doi: https://doi.org/10.1016/0300-5712(94)90032-9
- Sharma S, Khan N, Sultana S. 2005. Balsamodendron mukul suppresses benzoyl peroxide and ultraviolet light induced tumor promotional events in Swiss mice. J Photochem Photobiol B, Biol. 78:43–51. doi: https://doi.org/10.1016/j.jphotobiol.2004.09.006
- Shin H, Jo S, Mikos AG. 2003. Biomimetic materials for tissue engineering. Biomaterials. 24:4353–4364. doi: https://doi.org/10.1016/S0142-9612(03)00339-9
- Temenoff JS, Mikos AG. 2000. Injectable biodegradable materials for orthopedic tissue engineering. Biomaterials. 21:2405–2412. doi: https://doi.org/10.1016/S0142-9612(00)00108-3
- Vajrabhaya L, Sithisarn P. 1997. Multilayer and monolayer cell cultures in a cytotoxicity assay of root canal sealers. Int Endod J. 30:141–144. doi: https://doi.org/10.1046/j.1365-2591.1997.00056.x
- Vignoletti F, Matesanz P, Rodrigo D, Figuero E, Martin C, Sanz M. 2012. Surgical protocols for ridge preservation after tooth extraction. A systematic review. Clin Oral Implants Res. 23:22–38. doi: https://doi.org/10.1111/j.1600-0501.2011.02331.x
- Wang S, Yaszemski MJ, Knight AM, Gruetzmacher JA, Windebank AJ, Lu L. 2009. Photo-crosslinked poly(epsilon-caprolactone fumarate) networks for guided peripheral nerve regeneration: material properties and preliminary biological evaluations. Acta Biomater. 5:1531–1542. Epub 2009/01/28. doi: https://doi.org/10.1016/j.actbio.2008.12.015
- Woodward SC, Brewer P, Moatamed F, Schindler A, Pitt C. 1985. The intracellular degradation of poly (-caprolactone). J Biomed Mater Res. 19:437–444. doi: https://doi.org/10.1002/jbm.820190408
- Zhang Z, Feng X, Mao J, Xiao J, Liu C, Qiu J. 2009. In vitro cytotoxicity of a novel injectable and biodegradable alveolar bone substitute. Biochem Biophys Res Commun. 379:557–561. doi: https://doi.org/10.1016/j.bbrc.2008.12.084
- Zubillaga G, Hagen SV, Simon BI, Deasy MJ. 2003. Changes in alveolar bone height and width following post-extraction ridge augmentation using a fixed bioabsorbable membrane and demineralized freeze-dried bone osteoinductive graft. J Periodontol. 74:965–975. doi: https://doi.org/10.1902/jop.2003.74.7.965