Abstract
Boesenbergia rotunda, a herb in the ginger family, contains numerous beneficial compounds, such as flavonoids, flavones and cyclohexenyl chalcone derivatives, that have great potential for pharmaceutical applications. However, the low concentration of the bioactive compounds limits their commercial application. In this study, a simple and reliable Agrobacterium-mediated transformation protocol for B. rotunda cell suspension culture was successfully developed. The minimal inhibitory concentration and natural tolerance of the selective agent, hygromycin, against the cells were 20 mg l−l and 30 mg l−l in liquid media and solid media, respectively. The highest number of transformed regenerants (18 ± 0.00 per ml settled cell volume) was recorded when cells were infected with Agrobacterium tumefaciens harbouring pCAMBIA1304 for 10 min and co-cultivated for 2 days. Prolonged infection time (> 10 min) and co-cultivation period (> 2 days), however, did not increase the transformation efficiencies. The results clearly show that infection and co-cultivation periods strongly influenced the transformation efficiency in ginger. The transformed cells were recovered and showed green fluorescent signals under ultraviolet excitation. An intense blue colour was observed in the transformed cells after β-glucuronidase (GUS) histochemical staining, further confirming the functionality of the GUS enzymes in the regenerants. Polymerase chain reaction analysis of 3-, 6-, 9- and 12-month-old transformed cells confirmed that the protocol enabled stable integration of the mgfp5 gene. Moreover, the comparatively high number of transformed regenerants in this study made it possible to generate a large number of transgenic cells in a short period, which would be useful for high-throughput functional screening of enzymes involved in the biosynthetic pathways of bioactive compounds.
Introduction
Plant secondary metabolites are important sources of useful pharmaceutical drugs. However, the low amounts of these compounds in plants pose a significant challenge for drug development to meet industrial needs. The use of plant cell cultures has been widely studied for the production of secondary metabolites (Häkkinen et al. Citation2013). Although chemical synthesis is possible for several secondary metabolites, the use of toxic chemicals and extreme conditions often limits the de novo synthesis of these compounds (Wang et al. Citation2011). To overcome this problem, an effective strategy to enhance the production of beneficial secondary metabolites is essential. Over the decades, many studies have been carried out to improve the production of these high-value compounds through plant cell suspension cultures (Aoyagi Citation2011; Häkkinen et al. Citation2013). Plant cell suspension cultures offer several advantages for the production of secondary metabolites, including the ease of compound extraction and scaling up (Tepe & Sokmen Citation2007) and the simplicity of enhancing the compound production using chemical elicitors or physical stimulants (Zhao et al. Citation2010; Cai et al. Citation2011). They also offer a robust platform for the genetic engineering of metabolic pathways owing to their fast growing and homogeneous characteristics (Rao & Ravishankar Citation2002). However, yields of desired compounds may be less than ideal. As a result, there is interest in applying bioengineering approaches to enhance the productivity of desired compounds. Metabolic engineering has been used to modulate endogenous metabolic pathways or introduce new metabolic capabilities in order to increase the production of a desirable compound in plants (Farré et al. Citation2014). Despite the successes achieved in the past few years, genetic engineering of plants is still in its infancy. This may be due to the limited understanding of most secondary metabolite biosynthesis pathways (Weathers et al. Citation2010), including incomplete pathways and key regulatory mechanisms controlling the pathway fluxes (Oksman-Caldentey & Inzé Citation2004), as well as the low success rates in introducing multiple genes into plants (Chen et al. Citation2015).
An efficient plant transformation can facilitate the metabolic engineering of plants for enhanced production of a selected secondary metabolite. Agrobacterium-mediated transformation has been the most accessible tool with which to introduce heterologous DNA and manipulate gene expression in plants (Ribas et al. Citation2011). Therefore, a reliable transformation and regeneration system with a low degree of chimerism and high regeneration frequency is a prerequisite (May et al. Citation1995; Sági et al. Citation1995). There are several factors that affect the transformation efficiency, such as Agrobacterium density, infection time, co-cultivation duration and conditions (Yong et al. Citation2006; Vrbová et al. Citation2013), and temperature (Li et al. Citation2003). To date, there is no report of Agrobacterium-mediated or direct gene transformation of medicinal ginger, Boesenbergia rotunda. Boesenbergia rotunda is rich in bioactive compounds, such as flavanoids, flavones, cyclohexenyl chalcone derivatives (Trakoontivakorn et al. Citation2001; Tan et al. Citation2012) and essential oils (Jaipetch et al. Citation1982). This article reports a reliable and efficient Agrobacterium-mediated transformation protocol for B. rotunda cell suspension culture that may facilitate the development of high numbers of multiple independent transformation events in B. rotunda.
Materials and methods
Plant material and establishment of cell suspension culture
Cell suspension cultures were established according to Wong et al. (Citation2013). In brief, newly emerged buds obtained from the fresh rhizomes of B. rotunda were cultured on callus induction medium consisting of Murashige and Skoog (MS) salts (Murashige & Skoog Citation1962), 1.0 mg l−1 α-naphthaleneacetic acid (NAA), 1.0 mg l−1 6-benzyladenine (BA), 1.0 mg l−1 indole-3-acetic acid (IAA), 3% sucrose and 0.2% Gelrite® (Sigma, St Louis, MO, USA). The induced pro-embryogenic masses were then transferred on to propagation medium [MS basal medium supplemented with 3 mg l−1 2,4-dichlorophenoxyacetic acid (2,4-D), 3% sucrose and 0.2% Gelrite] for 3–4 weeks. To establish cell suspension cultures, embryogenic callus generated was cultured in liquid MS basal medium supplemented with 1 mg l−1 2,4-D, 680 µM L-glutamine and 2% sucrose. The cell suspension cultures were agitated at 80 rpm on a rotary shaker and subcultured every 14 days with replacement of fresh liquid medium at a ratio of 1:4 (old to fresh media).
Minimal inhibitory concentration of hygromycin B against Boesenbergia rotunda cells
The established cells were cultured in MS liquid (SM) or on semi-solid (SMA) selection media supplemented with 1 mg l−1 2,4-D, 680 µM L-glutamine and different concentrations of hygromycin B. Hygromycin B used in the experiments was filter-sterilized using a 0.2 µm syringe filter and stored at 4°C until use. Gelrite (Duchefa, Haarlem, The Netherlands) at 2% was added only to the semi-solid culture medium. For the SM medium, the cells were cultured in 250 ml conical flasks with continuous shaking at 80 rpm. All cultures were maintained at 25 ± 2°C under a 16 h photoperiod with a light intensity of 31.4 µmol m−2 s−1 provided by cool fluorescent lamps in a growth room. Growth and growth-inhibition of the cell suspension cultures were observed by measuring the settled cell volume (SCV) with graduated 15 ml centrifuge tubes after 20 days of culture, whereas cells plated on semi-solid medium were observed after 3 weeks of culture. The number of surviving regenerants on the agar plates was counted under a stereomicroscope.
Plant transformation
Agrobacterium tumefaciens strain LBA4404 (Hoekema et al. Citation1983) harbouring a binary vector pCAMBIA1304 (CSIRO, Dickson, Australia) was used in transforming the B. rotunda cell suspension. The plasmid includes the nptII kanamycin selective marker genes for bacteria, an hpt gene for plant selection and the reporter genes gus and mgfp5. Before transformation, mid-log phase Agrobacterium cultures with an optical density (OD600) of about 1.0 in yeast extract broth (14.0 g l−1 nutrient broth, 1.0 g l−1 yeast extract, 10 mM MgSO4 and 5.0 g l−1 sucrose, pH 7.5) were used for all transformation experiments. For infection, bacteria were collected by centrifugation at 4000 g for 10 min and resuspended in liquid medium at OD600 ≈ 1. One volume of the settled cells was submerged in four volumes of bacteria in liquid medium supplemented with 100 µM of acetosyringone (SMC) at different infection times. Bacterial broth was completely removed by careful pipetting after the incubation period. The cells were co-cultivated with Agrobacteria in 5 volumes of SMC liquid medium for different co-cultivation periods at 28°C in darkness. For post co-cultivation treatments, the cells were washed in SM medium supplemented with an appropriate concentration of hygromycin B and 300 mg l−1 cefotaxime and agitated on a rotary shaker for 1 h at 80 rpm. They were then transferred into 50 ml SM medium and maintained for 20 days at 25 ± 2°C under a 16 h photoperiod with a light intensity of 31.4 µmol m−2 s−1 provided by cool fluorescent lamps in the growth room. After 20 days of culture, the cells were plated on SMA for recovery and regeneration. Transformation efficiency was expressed as the number of regenerants per millilitre of SCV.
Green fluorescent protein visualization and β-glucuronidase histochemical assay
Putative transformed cells were visualized under 460 nm excitation wavelength with a fluorescent inverted microscope (Olympus IX71, Tokyo, Japan) equipped with a 460–490 nm emission filter. Green fluorescent protein detection was documented using a cool camera attached to the microscope. For GUS histochemical staining, cells recovered from SMA were incubated in histochemical reagent containing 0.1 M phosphate buffer (pH 7.0), 0.5 mM ferricyanide, 0.5 mM ferrocyanide, 0.1% (v/v) Triton X-100, 10.0 mM EDTA, 20% (v/v) methanol and 1.0 mM 5-bromo-3-indolyl-glucuronide (X-Gluc) (Fermentas, Hanover, MD, USA) at 37°C in darkness for 3 days until blue coloration appeared. The cells were washed with 70% (v/v) ethanol. Finally, the stained samples were transferred and fixed in formalin/acetic acid/ethanol solution, as described in Jefferson (Citation1989), before being observed under a light microscope.
Polymerase chain reaction analysis
Total genomic DNA was isolated from putative transformed cells according to a modified method based on Doyle (Citation1987). For PCR analysis, 100 ng of total DNA was added to a 20 µl PCR containing 0.2 mM of each dNTP, 1.0 mM MgCl2, 1 unit GoTaq® DNA polymerase and 0.1 µM of each primer pair for the amplification of the mgfp5 gene: 5′-AAG GAG AAG AAC TTT TCA CTG GAG-3′ and 5′-AGT TCA TCC ATG CCA TGT GTA-3′. PCR amplification was performed in a thermal cycler with an initial denaturation at 95°C for 1 min, followed by 30 cycles at 95°C for 1 min, 55°C for 1 min, 68°C for 1 min and a final extension of 68°C for 10 min. PCR products were separated on 1.0% agarose gel using electrophoresis and analysed using a Gel-Pro® imager and analyser (MicroLambda, Clarksburg, NJ, USA). The gel was scored for the presence of the mgfp5 product (≈ 700 bp).
Statistical analysis
All experiments were done in triplicate and statistically analysed using one-way analysis of variance (ANOVA). The mean values of treatments were subjected to Duncan's multiple range test at the 95% confidence level using the Statistical Package for the Social Sciences (SPSS, Chicago, IL, USA).
Results and discussion
Minimal inhibitory concentration of hygromycin B against Boesenbergia rotunda suspension cells
Successful selection of transformed cells and elimination of the non-transgenic cells is a crucial step in successful genetic engineering for plants (Veluthambi et al. Citation2003). To allow only the cells expressing the transgene to regenerate, the optimum concentration of the selective agent has to be determined. High concentrations of antibiotics are toxic to plant cells and may cause abnormalities in plant growth, whereas low concentrations can lead to false positives. The effectiveness of a selection agent can be assessed by determining the MIC and natural tolerance of the cell cultures (Parveez et al. Citation2007). The effects of different concentrations of hygromycin B against B. rotunda cell suspension are depicted in Figures and . The growth of cells was greatly affected when hygromycin B was applied in the SM for about 20 days (a). Hygromycin B at 20 mg l−l, corresponding to a lethal dose higher than 75% (LD75), was found to be effective in inhibiting the cell growth. In SMA, the LD75 of the B. rotunda cells was found at 30 mg l−l hygromycin B (b), indicating that a higher concentration of hygromycin B was required in SMA compared to SM. However, no cells survived when cultured on SMA supplemented with 50 mg l−l hygromycin B. The surviving cells were recovered on selection media, whereas non-transformed cells eventually turned brown and died (red arrows in e). Application of the appropriate concentration of hygromycin B not only enabled effective selection of transformed cells but also allowed synchronization between selection and cell growth in different developmental growth phases, i.e. propagation and regeneration of the suspension cells, which usually involve different media combinations (Ribas et al. Citation2011).
Figure 1. Inhibitory effects of different concentrations of hygromycin B against Boesenbergia rotunda cell suspension culture in (a) liquid SM and (b) solid SMA for about 20 days. Lethal dose higher than 75% (LD75) for cells cultured in SM and SMA was 20 mg l−l and 30 mg l−l, respectively. Error bars represent standard error, where n = 3. SCV = settled cell volume.
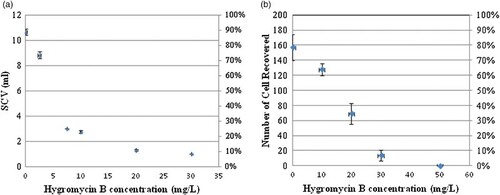
Effects of infection times and co-cultivation periods on Boesenbergia rotunda suspension cell transformation efficiency
The transfer and integration of genes is a lengthy process, and is dependent on the species and choice of explant culture conditions (Gelvin Citation2003; Mayavan et al. Citation2013). The results of this study revealed that infection time and co-cultivation period are important parameters affecting the efficiency of Agrobacterium-mediated transformation of B. rotunda cell suspension culture. To achieve optimum transformation efficiency, the influences of infection time and co-cultivation on Agrobacterium-mediated transformation of B. rotunda cell suspension culture were examined. The results revealed that the highest number of transformed regenerants (18 ± 0.00 per ml SCV) was achieved when cultures were infected with Agrobacterium for 10 min and co-cultivated for 2 days (). Longer infection times (> 10 min) and co-cultivation periods (> 2 days) did not increase the transformation efficiency. In spite of the overall successful transformation of B. rotunda suspension cell, a co-cultivation period of only 1 day resulted in low transformation efficiency. A 2–3 day co-cultivation period is optimal for many plant species, such as cotton (Sumithra et al. Citation2010), tomato (Chetty et al. Citation2013) and sugarcane (Mayavan et al. Citation2013). Dutt et al. (Citation2010) also reported that citrus explants co-cultivated for 4 or 5 days did not have an improved transformation efficiency rate, probably owing to the overgrowth of Agrobacterium, which ultimately inhibited cell growth. However, some plant species require a longer period of co-cultivation; for example, in Vanda orchid, explants co-cultivated for 4 days produced the highest transient gusA expression, compared to those co-cultivated for 1, 2 and 3 days (Gnasekaran et al. Citation2014). Similar findings were reported by De Bondt et al. (Citation1994), where a co-cultivation period of 4 days yielded a higher transformation efficiency in apple. Co-cultivation period is also dependent on the Agrobacterium strain used. For instance, Agrobacterium strain LBA4404 required 4 days for co-cultivation for optimal transformation in blueberry (Cao et al. Citation1998; Cheng et al. Citation2011).
Figure 3. Effects of infection times and co-cultivation periods on Agrobacterium-mediated transformation of Boesenbergia rotunda suspension cell. Different letters indicate significant differences at 95% confidence level based on one-way ANOVA followed by Duncan's multiple range test. Error bars represent standard error, where n = 5.
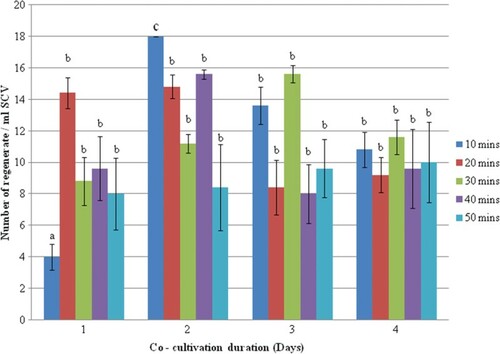
Cell regeneration and analysis of transformants
The transformed cells were recovered on the selection media, whereas non-transformed cells eventually turned brown and died (red arrows in a). Embryoid structures were observed on the putatively transformed cells after 3 weeks of selection on SM and SMA (a,b). In GUS histochemical staining, an intense blue colour was observed in the transformed cells (c). The cells were then examined for green fluorescence to determine the expression of the mgfp5 gene. All surviving cells recovered from subsequent selection in SM and SMA media emitted green fluorescence under ultraviolet examination (d). To investigate the stability of mgfp5 gene integration over 12 months, the putative 3-, 6-, 9- and 12-month-old transformed cells were propagated and analysed by performing PCR amplification using mgfp5-specific primers. A single band corresponding to about 700 bp was detected after PCR and gel analysis (), indicating the presence of the mgfp5 transgene in all samples tested.
Figure 4. (a, b) Embryoid regenerants [red (pale) arrows] and non-transformed cell (black arrows) on hygromycin selection. Bars = 1 mm (a) and 1 cm (b). (c) Putatively transformed regenerants showing gus expression in GUS histochemical assay. Bar = 1 mm. (d) Transformed cells appear as green fluorescence under ultraviolet excitation. Bar = 10 µm.
![Figure 4. (a, b) Embryoid regenerants [red (pale) arrows] and non-transformed cell (black arrows) on hygromycin selection. Bars = 1 mm (a) and 1 cm (b). (c) Putatively transformed regenerants showing gus expression in GUS histochemical assay. Bar = 1 mm. (d) Transformed cells appear as green fluorescence under ultraviolet excitation. Bar = 10 µm.](/cms/asset/88bed80e-30a1-4982-9f3d-2ae8ed7519c6/tfls_a_1051242_f0004_c.jpg)
Figure 5. Polymerase chain reaction analysis of mgfp5 gene integration in putatively transformed Boesenbergia rotunda regenerant cells. Lane 1: 100 bp DNA ladder; lane 2: no template control; lane 3: wild-type cells; lanes 4–7: 3-, 6-, 9- and 12-month-old transformed regenerants; lane 8: plasmid control.
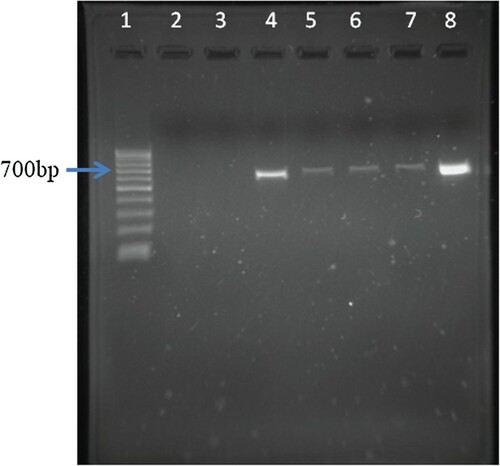
It is noteworthy that a stable Agrobacterium-mediated transformation of B. rotunda cell suspension cultures with integration of mgfp5 gene was achieved for over 12 months. The results clearly show that infection and co-cultivation strongly influence the transformation efficiency in ginger. The comparatively high number of transformed regenerants obtained by this method made it possible to generate a large number of transgenic cells in a short period, which would be useful for application in high-throughput metabolite engineering and functional analysis in B. rotunda.
Acknowledgements
The authors greatly appreciate the University of Malaya for providing support and facilities, the Malaysian Ministry of Science and Technology for a National Science Fund (NSF) doctoral scholarship to the first author, and the reviewers who helped to improve the manuscript.
Disclosure statement
No potential conflict of interest was reported by the author(s).
Additional information
Funding
References
- Aoyagi H. 2011. Application of plant protoplasts for the production of useful metabolites. Biochem Eng J. 56:1–8. doi: https://doi.org/10.1016/j.bej.2010.05.004
- Cai Z, Reidel H, Saw NMMT, Mewis I, Reineke K, Knorr D, Smetanska I. 2011. Effects of elicitors and high hydrostatic pressure on secondary metabolism of Vitis vinifera suspension culture. Process Biochem. 46:1411–1416. doi: https://doi.org/10.1016/j.procbio.2011.03.015
- Cao X, Liu Q, Rowland LJ, Hammerschlag FA. 1998. GUS expression in blueberry (Vaccinium spp.): factors influencing Agrobacterium-mediated gene transfer efficiency. Plant Cell Rep. 18:266–270. doi: https://doi.org/10.1007/s002990050569
- Chen CF, Chan KG, Tan BC, Norzulaani K. 2015. Enhancement of Agrobacterium-mediated transformation efficiency of model plant using quorum sensing molecule, N-3-oxo-octanoyl-L-homoserine-lactone. Plant Cell Tiss Organ Cult. 121:481–487. doi: https://doi.org/10.1007/s11240-015-0718-2
- Cheng M, Chang Y, Olhoft PM, Cardoza V, Lai F, Jones TJ, Wenck AR. 2011. Cells/tissues conditioning for facilitating T-DNA delivery. In: Dan Y, Ow DW, editors. Plant transformation technology revolution in last three decades: historical technology developments in plant transformation. USA: Bentham Science Publishers; p. 77–107.
- Chetty VJ, Ceballos N, Garcia D, Narváez-Vásquez J, Lopez W, Orozco-Cárdenas ML. 2013. Evaluation of four Agrobacterium tumefaciens strains for the genetic transformation of tomato (Solanum lycopersicum L.) cultivar Micro-Tom. Plant Cell Rep. 32:239–247. doi: https://doi.org/10.1007/s00299-012-1358-1
- De Bondt A, Eggermont K, Druart P, Vil MD, Goderis L, Vanderleydon J, Broekaert WF. 1994. Agrobacterium-mediated transformation of apple (Malus X domestica Borkh.): an assessment of factors affecting gene transfer during early transformation steps. Plant Cell Rep. 13:587–593. doi: https://doi.org/10.1007/BF00234517
- Doyle JJ. 1987. A rapid DNA isolation procedure for small quantities of fresh leaf tissue. Phytochem Bull. 19:11–15.
- Dutt M, Madhavaraj J, Grosser JW. 2010. Agrobacterium tumefaciens-mediated genetic transformation and plant regeneration from a complex tetraploid hybrid citrus rootstock. Sci Hortic. 123:454–458. doi: https://doi.org/10.1016/j.scienta.2009.10.010
- Farré G, Blancquaert D, Capell T, Van Der Straeten D, Christou P, Zhu C. 2014. Engineering complex metabolic pathways in plants. Annu Rev Plant Biol. 65:187–223. doi: https://doi.org/10.1146/annurev-arplant-050213-035825
- Gelvin SB. 2003. Agrobacterium-mediated plant transformation: the biology behind the “Gene-Jockeying” tool. Microbiol Mol Biol R. 67:16–37. doi: https://doi.org/10.1128/MMBR.67.1.16-37.2003
- Gnasekaran P, James Anthony JJ, Uddain J, Subramanian S. 2014. Agrobacterium-mediated transformation of the recalcitrant Vanda Kasem's Delight orchid with higher efficiency. Sci World J. 2014. Available from: https://doi.org/http://dx.doi.org/10.1155/2014/583934
- Häkkinen ST, Ritala A, Rischer H, Oksman-Caldentey KM. 2013. Medicinal plants, engineering of secondary metabolites in cell cultures. In: Sustainable food production. New York: Springer; p. 1182–1200.
- Hoekema A, Hirsch PR, Hooykaas PJJ, Schilperoort RA. 1983. A binary plant vector strategy based on separation of vir- and T-region of the Agrobacterium tumefaciens Ti-plasmid. Nature. 303:179–180. doi: https://doi.org/10.1038/303179a0
- Jaipetch T, Kanghae S, Pancharoen O, Patrock, VA, Reutrakul V, Tuntiwachwuttikul P, White AH. 1982. Constituents of Boesenbergia pandurata. Aust J Chem. 35:351–361. doi: https://doi.org/10.1071/CH9820351
- Jefferson RA. 1989. The GUS reporter gene system. Nature. 342:837–838. doi: https://doi.org/10.1038/342837a0
- Li DD, Shi W, Deng XX. 2003. Factors influencing Agrobacterium-mediated embryogenic callus transformation of Valencia sweet orange (Citrus sinensis) containing the pTA29-barnase gene. Tree Physiol. 23:1209–1215. doi: https://doi.org/10.1093/treephys/23.17.1209
- Murashige T, Skoog F. 1962. A revised medium for rapid growth and bio assays with tobacco tissue cultures. Physiol Plant. 15:473–497. doi: https://doi.org/10.1111/j.1399-3054.1962.tb08052.x
- May GD, Afza R, Mason HS, Wiecko A, Novak FJ, Arntzen CJ. 1995. Generation of transgenic banana (Musa acuminata) plants via Agrobacterium-mediated transformation. Nat Biotechnol. 13:486–492. doi: https://doi.org/10.1038/nbt0595-486
- Mayavan S, Subramanyam K, Arun M, Rajesh M, Dev GK, Sivanandhan G, Jaganath B, Manickavasagam M, Selvaraj N, Ganapathi A. 2013. Agrobacterium tumefaciens-mediated in planta seed transformation strategy in sugarcane. Plant Cell Rep. 32:1557–1574. doi: https://doi.org/10.1007/s00299-013-1467-5
- Oksman-Caldentey K-M, Inzé D. 2004. Plant cell factories in the post-genomic era: new ways to produce designer secondary metabolites. Trends Plant Sci. 9:433–440. doi: https://doi.org/10.1016/j.tplants.2004.07.006
- Parveez GKA, Abdul Majid N, Zainal A, Abdul Rasid O. 2007. Determination of minimal inhibitory concentration of selection agents for selecting transformed immature embryos of oil palm. AsPac J Mol Biol Biotechnol. 15:133–146.
- Rao RS, Ravishankar GA. 2002. Plant cell cultures: chemical factories of secondary metabolites. Biotechnol Adv. 20:101–153. doi: https://doi.org/10.1016/S0734-9750(02)00007-1
- Ribas AF, Dechamp E, Champion A, Bertrand B, Combes M, Verdeil J, Lapeyre F, Lashermes P, Etienne H. 2011. Agrobacterium-mediated genetic transformation of Coffea Arabica (L.) is greatly enhanced by using established embryogenic callus cultures. BMC Plant Biol. 11:1–15. doi: https://doi.org/10.1186/1471-2229-11-92
- Sági L, Panis B, Remy S, Schoofs H, de Smet K, Swennen R, Cammue BPA. 1995. Genetic transformation of banana and plantain (Musa spp.) via particle bombardment. Nat Biotechnol. 13:481–485. doi: https://doi.org/10.1038/nbt0595-481
- Sumithra S, Katageri IS, Vamadevaiah HM. 2010. Factors influencing regeneration and Agrobacterium mediated transformation of Gossypium herbaceaum and Gossypium hirsutum cotton genotypes. Karnataka J Agric Sci. 23:222–226.
- Tan EC, Karsani SA, Foo GT, Wong SM, Rahman NA, Khalid N, Yusof R. 2012. Proteomic analysis of cell suspension cultures of Boesenbergia rotunda induced by phenylalanine: identification of proteins involved in flavonoid and phenylpropanoid biosynthesis pathways. Plant Cell Tiss Organ Cult. 111:219–229. doi: https://doi.org/10.1007/s11240-012-0188-8
- Tepe B, Sokmen A. 2007. Production and optimisation of rosmarinic acid by Satureja hortensis L. callus cultures. Nat Prod Res. 21:1133–1144. doi: https://doi.org/10.1080/14786410601130737
- Trakoontivakorn G, Nakahara K, Shinmoto H, Takenaka M, Onishi-Kameyama M, Ono H, Yoshida M, Nagata T, Tsushida T. 2001. Structural analysis of a novel antimutagenic compound, 4 hydroxypanduratin A, and the antimutagenic activity of flavonoids in a thai spice, fingerroot (Boesenbergia pandurata Schult.) against mutagenic heterocyclic amines. J Agric Food Chem. 49:3046–3050. doi: https://doi.org/10.1021/jf010016o
- Veluthambi K, Aditya KG, Sharma A. 2003. The current status of plant transformation technologies. Curr Sci. 84:368–337.
- Vrbová M, Kotrba P, Horáček J, Smykal P, Švábová L, Vĕtrovcová M, Smykalová I, Griga M. 2013. Enhanced accumulation of cadmium in Linum usitatissimum L. plants due to overproduction of metallothionein α-domain as a fusion to β-glucuronidase protein. Plant Cell Tiss Organ Cult. 112:321–330. doi: https://doi.org/10.1007/s11240-012-0239-1
- Wang Y, Chen S, Yu O. 2011. Metabolic engineering of flavonoids in plants and microorganisms. Appl Microbiol Biotechnol. 91:949–956. doi: https://doi.org/10.1007/s00253-011-3449-2
- Weathers P, Towler M, Xu J. 2010. Bench to batch: advances in plant cell culture for producing useful products. Appl Microbiol Biotechnol. 85:1339–1351. doi: https://doi.org/10.1007/s00253-009-2354-4
- Wong SM, Salim N, Harikrishna JA and Khalid N. 2013. Highly efficient plant regeneration via somatic embryogenesis from cell suspension cultures of Boesenbergia rotunda. In Vitro Cell Dev Bio- Plant. 49:665–673. doi: https://doi.org/10.1007/s11627-013-9570-4
- Yong WTT, Abdullah JO, Mahmood M. 2006. Optimization of Agrobacterium-mediated transformation parameters for Melastomataceae spp. using green fluorescent protein (GFP) as a reporter. Sci Hortic. 109:78–85. doi: https://doi.org/10.1016/j.scienta.2006.03.005
- Zhao JL, Zhou LG, Wu JY. 2010. Effects of biotic and abiotic elicitors on cell growth and tanshinone accumulation in Salvia miltiorrhiza cell cultures. Appl Microbiol Biotechnol. 87:137–144. doi: https://doi.org/10.1007/s00253-010-2443-4