ABSTRACT
Phylloplane of a plant constitutes an important habitat for a variety of microbes many of which play important roles in plant growth. The objective of this study was to compare plant growth promoting (PGP) phylloplane bacteria of three commercially popular wheat varieties, namely Sehar, Faisalabad and Lasani, in order to assess whether better wheat variety harbours better PGP bacteria that play part in its superior yield. Phylloplane bacteria were isolated from the three wheat varieties thrice during the plant growth. The bacterial load increased with the growing season and Sehar variety carried maximum bacterial load (CFUg−1 1.1 × 109). Succession of bacterial community was also observed during the plant growth. Isolates belonging to Sehar phylloplane produced auxin in highest amounts (52.95 µg ml−1) during second sampling when plant was showing rapid growth. Many isolates from all three varieties fixed nitrogen, solubilized phosphates and some isolates also produced hydrogen cyanide. The results clearly indicated that the beneficial bacteria associated with phylloplane of better yielding variety were showing better PGP abilities when compared to their counterparts on low yielding varieties. Isolates exhibiting best PGP profiles were identified as Bacillus, Microbacterium, Acinetobacter, Proteus, Psychrobacter, Pseudomonas, Streptomyces and Kineococcus species through 16S rRNA gene sequencing.
Introduction
Wheat (Triticum aestivum) is the third-most cultivated cereal after maize and rice, with over 600 million tons harvested annually. It has high nutritional value and provides over 20% calories and proteins in human nutrition (Bushuk Citation1997). With increase in human population, development of methods to increase crop yield are of utmost importance. Improvement of soil fertility is also employed as one strategy to increase agricultural production. Among the limiting nutrients of soil, nitrogen (N) and phosphorus (P) are most important. Nitrogen assimilation into amino acids provides building block for plant's proteins. Phosphorus is a major component of nucleic acids and is very important in plant development and maturity. Indole acetic acid (IAA) is an essential natural growth promoter. Sufficiently high amounts of these nutrients are required by plants. Many microorganisms are known to effectively induce plant growth and promote its health (Hayat et al. Citation2010). Studies suggest that use of these plant growth promoting bacteria (PGPB) can be an alternative to chemicals in order to improve the growth of crop plants (Adesemoye & Kloepper Citation2009). Such microbes have agricultural significances as they can be employed in biological nitrogen fixation, mineral solubilization (e.g. phosphates), phytohormones production (e.g. IAA) as well as components of organic composts in order to improve yield and quality of agricultural crops (Higa Citation1994).
‘Phyllosphere’ refers to aerial or above ground parts of plants including leaves, stems, buds, flowers and fruits, whereas surface area of leaves constitute phylloplane. This environmental niche supports large and complex microbial communities (Inácio et al. Citation2002; Lindow & Brandl Citation2003; Whipps et al. Citation2008). It is largely inhabited by bacteria as well as archaea, filamentous fungi and yeasts. These microbes are either associated with plant surfaces as epiphytes or may reside inside tissues as endophytes (Arnold et al. Citation2000). Phyllosphere is one of the hostile environments and there has been a lot of interest in the life forms that inhabit it. Leaves are a more dominant aerial part and bacteria are dominant inhabitants of leaves. The associated bacterial load usually lies in range of 106 to 107 cells/cm2 (up to 108 cells g−1) of leaf (Beattie & Lindow Citation1995; Jacques & Morris Citation1995; Andrews & Harris Citation2000). Epiphytic bacterial communities are very dynamic and differ greatly in size among and within plants of the same species, as well as over growing season (Ercolani Citation1991). As phyllosphere is directly exposed to the environment, large fluctuations may occur in the physical and nutritional conditions resulting in variations in population types and sizes. Moreover, specific plant species and its genetic makeup influences the microbial carrying specificity and capacity of the leaves (Kinkel et al. Citation2000).
For sustainable agriculture, microbes may be employed for plant growth enhancement. Evidence supports the functional roles played by phyllosphere complex microbial communities. N2 fixing bacteria have been reported to be present in the phyllosphere of many crop plants (Miyamoto et al. Citation2004). Mwajita et al. (Citation2013) evaluated the rhizosphere, rhizoplane and phyllosphere bacteria from rice fields in Kenya for PGPB and reported that over 50% of bacterial isolates from phyllosphere were able to solubilize phosphates. Production of indole-3-acetic acid (IAA) is common among bacterial epiphytes (Brandl et al. Citation2001). Marques et al. (Citation2010) assessed plant growth promoting (PGP) abilities of six bacterial isolates on Zea mays plant and reported that all isolates produced IAA, hydrogen cyanide (HCN) and ammonia in vitro as well as in green house experiments. Normal microbiota exclusive to commercially better breeds may possess better PGP properties as compared to microbiota of lower yielding varieties and hence may enhance growth when inoculated in lower yielding varieties. The present study was undertaken to screen and compare PGP phylloplane bacteria from three commercial wheat varieties Sehar, Faisalabad and Lasani over different growing stages of the plants in order to confirm whether better yielding varieties do possess bacteria with superior PGP abilities.
Methodology
Sowing and harvesting
Seeds of three commercial wheat varieties: Sehar, Faisalabad and Lasani were obtained from Punjab Seed Corporation, Lahore. Sehar variety was the most superior one having greater growth and yield as compared to the other two varieties. The seeds were sown in pots (12 inch size) and kept in wire house in natural environment with regular watering. Plants were sampled three times at different growth stages of plants with intervals of approximately 45 days. For sampling, randomly selected plants were removed from experimental pots, root and shoot lengths were recorded and leaves were picked for bacterial isolation.
Isolation of epiphytes from phylloplane
Leaves were washed properly with autoclaved water. Cuts were treated with 70% bleach and wrapped with parafilm to avoid contamination of endophytes. Three grams of leaves were vortexed vigorously in 30 ml PBS using horizontal vortex adapter (Mobio) to ensure removal of maximum biomass. Serial dilutions were prepared and plated on N-agar plates followed by incubation at 28°C for 24–48 h. colony forming units (CFU) was counted and diverse colonies were picked and purified by quadrant-streak method. Morphological studies, gram staining, catalase and oxidase tests were performed for each isolate. Isolates were given codes with first 2 letters representing wheat variety (FD for Faisalabad, SH for Sehar and LA for Lasani), followed by ‘P’ representing phylloplane followed by the sampling number (1, 2 or 3).
Screening for PGPB
Nitrogen fixation ability
To screen enzymatically competent microorganisms that convert atmospheric nitrogen into nitrogenous compounds, nitrogen-free mannitol agar medium was used. Bacteria were cultured on the media and incubated at 28°C for 24–48 h. Following incubation, the plates were checked for the presence or absence of growth.
Phosphate solubilization ability
Phosphate solubilization by bacterial isolates was assessed based on the halo zones formation around the bacterial growth on Pikovskaya medium (Citation1948) containing calcium phosphate (2.5%) as inorganic phosphate source. Plates were inoculated and incubated at 28°C for 7 days. Following incubation, plates were observed for the formation of clear zones.
Hydrogen cyanide production
Identification of cyanogenic microorganisms was done by culturing bacteria on nutrient agar supplemented with 4.4 gL−1 glycine (Ahmad et al. Citation2008). Whatman filter paper No.1 soaked in filter sterilized solution of 2% sodium carbonate (in 0.5% picric acid) was placed over inoculated media surface aseptically. Plates were sealed with parafilm and incubated at 28°C for 4 days. After incubation, plates were observed for development of orange to red colour of the filter paper as an indication of HCN production.
Auxin production
LB-broth supplemented with tryptophan (500 µg ml−1) was inoculated and incubated at 37°C for 7 days in dark with constant shaking. After incubation, samples were centrifuged and 100 µl supernatant was mixed with 200 µl freshly prepared Salkowski reagent in a microtiter plate. The plate was incubated for 30 min in dark at room temperature for colour development. Absorbance was measured at 535 nm using Epoch BioTek plate reader (Gordon & Weber Citation1951). Concentration of IAA produced by bacterial isolates was calculated based on the standard curve achieved by processing IAA standards as described above.
Phylogenetic analysis
Bacterial isolates showing better PGP results were sent to Macrogen (Korea) for 16S rRNA gene sequencing using primers 518F (CCAGCAGCCGCGGTAATACG) and 800R (TACCAGGGTATCTAATCC) (Porsby et al. Citation2008; Bernbom et al. Citation2011). Sequences thus obtained were checked for quality with FinchTV (Geospiza) and classified through NCBI BLAST using 16S ribosomal sequence database. Sequences were aligned and neighbour-joining (NJ) phylogenetic tree (Saitou & Nei Citation1987) was made in MEGA 5.0 (Tamura et al. Citation2011).
Results
Isolation of phylloplane bacteria
Twenty-two diverse bacterial isolates were obtained from first phyllosphere sampling of the three wheat varieties. Faisalabad had the highest diversity (10) and CFUg−1 (8 × 106), followed by Lasani variety with 7 unique isolates and CFUg−1 (4.4 × 106). Sehar variety had five unique isolates but highest CFUg−1 (9.2 × 106). During second sampling of wheat leaves, 10 isolates from Faisalabad variety with CFUg−1 (4 × 106), 9 diverse isolates were recovered from Sehar and CFUg−1 was 1.76 × 107, whereas in case of Lasani variety 8 distinct isolates with 3.64 × 107 CFUg−1 was obtained. During third sampling, number of diverse colonies obtained from Faisalabad variety was highest (which increased to 13) and CFUg−1 was 2.8 × 107, followed by Lasani with 12 distinct isolates and CFUg−1 1.12 × 107. From Sehar variety, 4 isolates were obtained in third sampling with CFUg−1 4.32 × 107, which was highest in all 3 samplings.
Characterization of bacterial isolates
The morphological characteristics of bacterial isolates varied widely as shown in Tables 1S, 2S and 3S. In first sampling, bacterial isolates were mostly rod shaped and gram negative. During second sampling, most of the isolates were rod shaped and few were cocci with equal proportion of gram-negative and gram-positive bacteria. The number of gram-positive rods and gram-negative rods were equal in number in the third sampling. Number of gram-positive cocci increased and few gram-negative cocci were also found. Majority of the isolates were positive for catalase and a few were positive for oxidase test during all three samplings (Table ).
Table 1. Plant growth promoting potentials of isolates of wheat varieties during three samplings.
Nitrogen fixation by the bacterial isolates
In first sampling, 5 of 10 isolates were nitrogen fixers from Faisalabad variety, 2 of 5 from Sehar variety, whereas 5 of 7 isolates were nitrogen fixers from Lasani variety. During second sampling, seven isolates from Faisalabad, six from Sehar and seven isolates from Lasani were nitrogen fixers. Nine isolates from Faisalabad, four from Sehar and six from Lasani wheat variety were found to be fixing nitrogen in third sampling (Table ).
Phosphate solubilization
During first sampling, FDP1-3 of Faisalabad variety and SHP1-11 of Sehar variety were able to solubilize phosphates and produced clear zones in culture media plates. During second sampling, FDP2-8 isolate from Faisalabad variety, SHP2-20 isolate from Sehar and LAP2-23 isolate from Lasani were found to be phosphate solubilizers. During third sampling, FDP3-2 isolate from Faisalabad variety, SHP3-17 isolate of Saher variety and LAP3-24 isolate of Lasani variety were positive for phosphate solubilization (Table ).
HCN producing isolates
During first sampling, two bacterial isolates from Faisalabad variety (FDP1-1 and FDP1-2) were able to produce HCN. However, none of the isolates from Sehar and Lasani showed HCN production. During second sampling, isolates FDP2-1 from Faisalabad variety and LAP2-26 from Lasani were HCN producers. FDP3-5 from Faisalabad variety and LAP3-24 and LAP3-25 from Lasani variety were positive for HCN production during third sampling (Table ).
Auxin production
Majority of isolates from all of three wheat varieties were auxin producers (Table ). During first sampling, seven isolates of Faisalabad variety, four isolates of Sehar variety and five isolates of Lasani variety produced auxin in varied concentrations. Highest concentration of auxin was produced by isolate SHP1-12 from Sehar variety, up to 49.55 µg ml−1 (Figure ). During second sampling, seven isolates of Faisalabad, seven isolates of Sehar and five isolates of Lasani wheat variety were auxin producers. Highest concentration of auxin (52.95 µg ml−1) was produced by isolate SHP2-20 of Sehar variety (Figure ). During the third sampling, all isolates of the three wheat varieties showed auxin production, though at low concentrations, ranging from 1–22 µg ml−1. Highest concentration of auxin production was observed by SHP3-17 isolate from Sehar variety (22 µg ml−1) (Figure ).
Phylogenetic analysis
Selected isolates were identified using 16S rRNA gene sequencing. Genbank accession numbers are given in Table . From sampling #1, Isolate SHP1-11, SHP1-12, LAP1 and LAP1-23 were identified as Acinetobacter calcoaceticus, Bacillus stratoshphericus, Proteus mirabilis and Psychrobacter alimentarius, respectively. From sampling #2, FDP2-1, FDP2-8, SHP2-16, SHP2-17, SHP2-20, LAP2-23, LAP2-26 and LAP2-29 were identified as Pseudomonas syringae, Microbacterium natoriense, Microbacterium lemovicicum, Bacillus amyloliquefaciens, Streptomyces humidus, Microbacterium testaceum and Kineococcus radiotolerans, respectively. FDP3-2, FDP3-5, SHP3-17 and LAP3-24 selected from sampling #3 were identified as Bacillus aerius, Psychrobacter alimentarius, Microbacterium hydrocarbonoxydans and Microbacterium hydrocarbonoxydans, respectively. These results were confirmed by constructing NJ phylogenetic tree of 16S rRNA gene sequences of bacterial isolates and their nearest homologues from NCBI 16S ribosomal RNA sequences database (Figure ).
Figure 2. Auxin production by phylloplane bacterial isolates from second sampling of Seher, Faisalabad and Lasani wheat varieties. Highest auxin concentrations was found to be produced by Seher isolates ShP2-17 and ShP2-20.
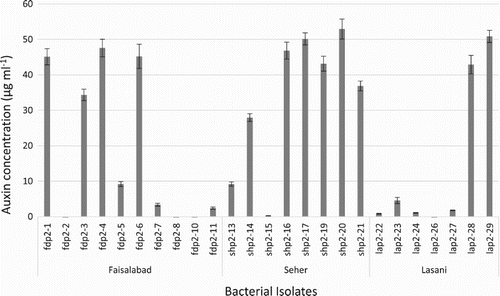
Figure 3. Auxin production by phylloplane bacterial isolates from third sampling of Seher, Faisalabad and Lasani wheat varieties. Highest auxin concentrations was found to be produced by Seher isolates ShP3-17.
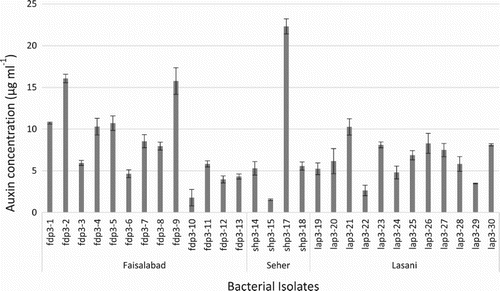
Figure 4. 16SrRNA gene sequence neighbour-joining phylogenetic tree of the selected bacterial isolates and their nearest homologue sequences from NCBI. The tree was constructed in Mega 5 and 100 replicates of bootstrap was used as measure of reliability.
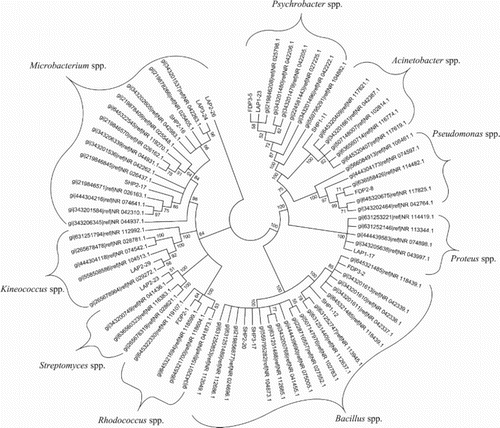
Table 2. Identity of the selected isolates based on 16S rRNA gene sequences and their Genbank accession number.
Discussion
Pakistan has an agriculture-based economy and therefore significant production of wheat is very critical. Plants' important microbiota could be explored and exploited in order to improve the growth of the plants. Three selected wheat varieties Saher, Faisalabad and Lasani had differences in growth rates and product yields with Sehar variety leading the two other varieties in both the aspects. Bacterial load (CFUg−1) was highest for Sehar variety over the course of three samplings suggesting this variety was capable of supporting larger populations of bacteria over leaf surfaces. The gradual increase in CFU and subsequent decrease in diversity suggested that succession of bacterial population occurred through the growing season. In the beginning, many diverse bacteria were present on leaves but at the end of growing season, only few ecologically competitive bacteria successfully colonized the leaves. Acinetobacter and Proteus only appeared in first sampling, Rhodococcus, Pseudomonas and Streptomyces appeared only in second sampling whereas Microbacterium and Bacillus were repeatedly present in more than one samplings. In present study, Bacillus predominated Sehar phylloplane in all three samplings and since Bacillus are known to survive hostile environments (Enya et al. Citation2007), therefore they thrived and colonized on leaves of Sehar variety even when the plants were old and dry. Moreover, Bacillus exhibited one of best PGP profiles in lab tests implying its role (as part of normal microbiota) in superiority of Sehar variety.
Vast majority of the isolates from all varieties were nitrogen fixers. As phylloplane is directly exposed to atmosphere, abundance of bacteria with ability to fix atmospheric nitrogen is logical (Knief et al. Citation2012; Mwajita et al. Citation2013). During first sampling, number of nitrogen fixers was least since seedlings were just starting to grow. Number of nitrogen fixers was greatest during second sampling as it was time of rapid growth of plants and more nitrogen in fixed form was required. Moreover, the plant and climate conditions during second sampling were perhaps most suited to nitrogen fixing bacteria. During third sampling, number of nitrogen fixers reduced. This could be because plant had already attained its maximum growth so no more fixed nitrogen was required, as well as the fact that plant was drying out and was not the same supportive for bacterial growth. Phosphate solubilization is another important attribute of plant associated microbes and all of the three varieties of wheat had bacteria associated with phylloplane that solubilized phosphate in order to make it available for plants. However, the number of phosphate solubilizing bacteria was much lower. Insoluble phosphates are abundant in soil and thus phosphate solubilizing bacteria are supposed to be greater in number in soil as compared to phylloplane (Verma et al. Citation2001). In case of HCN production, very few isolates were found to give positive results during all three samplings of all wheat varieties. HCN is effective in weed control and hence capability of plant associated bacteria to produce HCN significantly increases plant's resistance (Marques et al. Citation2010). As for auxin production, large number of isolates from all three wheat varieties significantly produced the phytohormone throughout the three sampling periods. This is in accordance with an earlier report by Lindow and Brandl (Citation2003) who stated that epiphytic bacteria on phyllosphere are known to produce this hormone. Not only the number of bacteria positive for auxin production was greatest during second sampling but the concentration of auxin produced was also maximum and it was probably due to the fact that the plant needed more auxin at that time since it was growing rapidly. Almost all isolates of Seher variety showed auxin production to highest levels which could be one of the reasons of better growth of Seher variety as compared to the rest of the two varieties.
Isolates exhibiting best PGP profiles were identified through ribotyping. Sequence homology was checked through BLAST (NCBI) and the results were confirmed by constructing phylogenetic tree. Taxonomic analysis revealed the dominance of Actinobacteria, Bacilli and Gammaproteobactera in wheat phylloplane. Faisalabad variety was predominated by Actinobacteria (50%), Gammaproteobacteria (33%) and Firmicutes (17%). Actinobacteria (33%), Gammaproteobacteria (17%) and Firmicutes (50%) contributed mainly to bacterial community associated with Sehar variety. Phylloplane of Lasani was predominated with Actinobacteria (67%) and Gammaproteobacteria (33%). Among the Actinobacteria, the genus Microbacterium was predominantly detected and phylum Firmicutes was primarily represented by genus Bacillus.
In the light of all the facts obtained from the present study it can be concluded that phyllosphere microbial community exhibits benefiting properties on plants' well-being. Succession of bacterial community occurs over growing season and presence of PGP bacteria is most pronounced at rapid growth stages of plants. Varieties with better growth and yield do possess bacteria with better PGP abilities which may be one the many reasons of the superior growth and yield. This fact may be exploited for sustainable agriculture. The phylloplane communities of other important plants and agricultural crops need to be studied in order to further establish the role of microbiota in growth and yield of superior varieties.
Disclosure statement
No potential conflict of interest was reported by the authors.
ORCID
Yasir Rehman http://orcid.org/0000-0002-4787-275X
Additional information
Funding
References
- Adesemoye A, Kloepper JW. 2009. Plant-microbes interactions in enhanced fertilizer-use efficiency. Appl Microbiol Biotechnol. 85:1–12. doi: 10.1007/s00253-009-2196-0
- Ahmad F, Ahmad I, Khan MS. 2008. Screening of free living rhizospheric bacteria for their multiple plant growth promoting activities. Microbiol Res. 163:173–181. doi: 10.1016/j.micres.2006.04.001
- Andrews JH, Harris RF. 2000. The ecology and biogeography of microorganisms on plant surfaces. Annu Rev Phytopathol. 38:145–180. doi: 10.1146/annurev.phyto.38.1.145
- Arnold AE, Maynard Z, Gilbert GS, Coley PD, Kursar TA. 2000. Are tropical fungal endophytes hyperdiverse? Ecol Lett. 3:267–274. doi: 10.1046/j.1461-0248.2000.00159.x
- Beattie GA, Lindow SE. 1995. The secret life of foliar bacterial pathogens on leaves. Annu Rev Phytopathol. 33:145–172. doi: 10.1146/annurev.py.33.090195.001045
- Bernbom N, Ng YY, Kjelleberg S, Harder T, Gram L. 2011. Marine bacteria from Danish coastal waters show antifouling activity against the marine fouling bacterium Pseudoalteromonas sp. strain S91 and zoospores of the green alga Ulva australis independent of bacteriocidal activity. Appl Environ Microbiol. 77:8557–8567. doi: 10.1128/AEM.06038-11
- Brandl MT, Quinones B, Lindow SE. 2001. Heterogeneous transcription of an indoleacetic acid biosynthetic gene in Erwinia herbicola on plant surfaces. P Natl Acad Sci USA. 98:3454–3459. doi: 10.1073/pnas.061014498
- Bushuk W. 1997. Wheat breeding for end-product use. In: Wheat: prospects for global improvement. Dordrecht: Springer; p. 203–211.
- Enya J, Koitabashi M, Shinohara H, Yoshida S, Tsukiboshi T, Negishi H, Suyama K, Tsushima S. 2007. Phylogenetic diversities of dominant culturable Bacillus, Pseudomonas and Pantoea species on tomato teaves and their possibility as biological control agents. J Phytopathol. 155:446–453. doi: 10.1111/j.1439-0434.2007.01256.x
- Ercolani GL. 1991. Distribution of epiphytic bacteria on olive leaves and the influence of leaf age and sampling time. Microb Ecol. 21:35–48. doi: 10.1007/BF02539143
- Gordon SA, Weber RP. 1951. Colorimetric estimation of indoleacetic acid. Plant Physiol. 26:192–195. doi: 10.1104/pp.26.1.192
- Hayat R, Ali S, Amara U, Khalid R, Ahmed I. 2010. Soil beneficial bacteria and their role in plant growth promotion: a review. Ann Microbiol. 60:579–598. doi: 10.1007/s13213-010-0117-1
- Higa T. 1994. Effective microorganisms: a new dimension for nature farming. In: Proceedings of the Second International Conference on Kyusei Nature Farming. Department of Agriculture.
- Inácio J, Pereira P, de Carvalhom M, Fonseca A, Amaral-Collaço MT, Spencer-Martins I. 2002. Estimation and diversity of phylloplane mycobiota on selected plants in a Mediterranean-type ecosystem in Portugal. Microb Ecol. 44:344–353. doi: 10.1007/s00248-002-2022-z
- Jacques MA, Morris CE. 1995. A review of issues related to the quantification of bacteria from the phyllosphere. FEMS Microbiol Ecol. 18:1–14. doi: 10.1111/j.1574-6941.1995.tb00158.x
- Kinkel LL, Wilson M, Lindow SE. 2000. Plant species and plant incubation conditions influence variability in epiphytic bacterial population size. Microb Ecol. 39:1–11. doi: 10.1007/s002489900182
- Knief C, Delmotte N, Chaffron S, Stark M, Innerebner G, Wassmann R, von Mering C, Vorholt JA. 2012. Metaproteogenomic analysis of microbial communities in the phyllosphere and rhizosphere of rice. ISME J. 6:1378–1390. doi: 10.1038/ismej.2011.192
- Lindow SE, Brandl MT. 2003. Microbiology of the phyllosphere. Appl Environ Microbiol. 69:1875–1883. doi: 10.1128/AEM.69.4.1875-1883.2003
- Marques AP, Pires C, Moreira H, Rangel AO, Castro PM. 2010. Assessment of the plant growth promotion abilities of six bacterial isolates using Zea mays as indicator plant. Soil Biol Biochem. 42:1229–1235. doi: 10.1016/j.soilbio.2010.04.014
- Miyamoto T, Kawahara M, Minamisawa K. 2004. Novel endophytic nitrogen-fixing clostridia from the grass Miscanthus sinensis as revealed by terminal restriction fragment length polymorphism analysis. Appl Environ Microbiol. 70:6580–6586. doi: 10.1128/AEM.70.11.6580-6586.2004
- Mwajita MR, Murage H, Tani A, Kahangi EM. 2013. Evaluation of rhizosphere, rhizoplane and phyllosphere bacteria and fungi isolated from rice in Kenya for plant growth promoters. SpringerPlus. 2:606. Epub 2013/12/19. doi: 10.1186/2193-1801-2-606
- Pikovskaya RI. 1948. Mobilization of phosphorus in soil connection with the vital activity of some microbial species. Microbiologiya. 17:362–370.
- Porsby CH, Nielsen KF, Gram L. 2008. Phaeobacter and Ruegeria Species of the Roseobacter clade colonize separate niches in a Danish Turbot (Scophthalmus maximus)-rearing farm and antagonize Vibrio anguillarum under different growth conditions. Appl Environ Microbiol. 74:7356–7364. doi: 10.1128/AEM.01738-08
- Saitou N, Nei M. 1987. The neighbor-joining method: a new method for reconstructing phylogenetic trees. Mol Biol Evol. 4:406–425.
- Tamura K, Peterson D, Peterson N, Stecher G, Nei M, Kumar S. 2011. MEGA5: molecular evolutionary genetics analysis using maximum likelihood, evolutionary distance, and maximum parsimony methods. Mol Biol Evol. 28:2731–2739. doi: 10.1093/molbev/msr121
- Verma SC, Ladha JK, Tripathi AK. 2001. Evaluation of plant growth promoting and colonization ability of endophytic diazotrophs from deep water rice. J Biotechnol. 91:127–141. doi: 10.1016/S0168-1656(01)00333-9
- Whipps JM, Hand P, Pink D, Bending GD. 2008. Phyllosphere microbiology with special reference to diversity and plant genotype. J Appl Microbiol. 105:1744–1755. doi: 10.1111/j.1365-2672.2008.03906.x