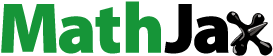
Abstract
The selection of species for enrichment planting that involves native species in a secondary lowland dipterocarps forest as one of the tropical rainforest types should be conducted to maintain the sustainable management of tropical rainforests. The research of species trial of dipterocarp species was planted under a randomized complete block design with four replications. The following parameters were examined: survival rate, diameter breast height (DBH), pilodyn penetration (PP), and stress wave velocity (SWV). The survival rate (F = 1.72; p = 0.047), DBH (F = 6.67, p < 0.0001), and pilodyn penetration (F = 14.19; p < 0.001) significantly differed at 12.5 years after planting. The survival rate of Shorea leprosula was the highest (85%) of all the species, and the survival rate of other species, namely, S. macrophylla, S. ovalis, S. johorensis, S. scaberima, and S. parvifolia, was more than 70%. The best growth of DBH parameter was observed in S. platyclados. The DBH growth of S. platyclados 12.5 years after planting were 27.62 cm (mean annual diameter increment [MADI] = 2.2 cm/year). The species whose growth in DBH was similar to those of S. platyclados were S. leprosula, S. parvivolia, and S. ovalis. However, stress wave velocity (SWV) was not significantly different among dipterocarp species (F = 1.26; p = 0.234). Pilodyn penetration (PP) was significantly correlated with DBH (Pearson’s correlations = 0.306; p < 0.001), whereas stress wave velocity was not significantly correlated with DBH and PP (p > 0.05), and their Pearson’s correlations were 0.043 and −0.012, respectively. These results suggested that each dipterocarp species varied in terms of their growth and wood properties. Thus, choosing the species for enrichment planting in secondary tropical rainforests depends on the characteristics of each species and the desired final product of forest management.
Introduction
Lowland dipterocarp forests as one of the tropical rainforest types are among the megadiverse terrestrial ecosystems (Whitmore Citation1998; Myers et al. Citation2000; Sodhi et al. Citation2004) dominated by dipterocarp species (Ashton Citation1982; Symington Citation2004). The forest production of lowland dipterocarp forests is managed through selective cutting (Appanah Citation1998; Dawkins and Philip Citation1998; MOF Citation2009; Putz et al. Citation2012). The cutting cycle is 25–30 years (MOF Citation2009), and it generally allows the cutting of all commercial tree species with a minimum diameter breast height (DBH) of >50 cm (MOF Citation2009; Finkeldey Citation2011). However, selective cutting can damage more than 50% of trees in the stand and affect the structure, species composition, and production of a logged-over area (Pinard and Putz Citation1996; Huth and Ditzer Citation2001; Kartawinata et al. Citation2001; Sist et al. Citation2003). The timber stock recoveries of the same tree species or new species harvested in the second and third cuts are only 35% and 54% of the timber volume extracted during the first harvest from the primary forest, respectively (Putz et al. Citation2012). This phenomenon suggests that timber stocks in the second and third rotations of selective logging are lower than those in the first rotation.
On the other hand, lowland dipterocarp forests have been degraded by forest fire, large-scale commercial logging, shifting cultivation, and other human activities. In Indonesia, the forest cover loss in Indonesia was 1.21 Mha year−1 from 2000 to 2012, and 38% of the forest cover loss occurs within the primary forest, especially on Kalimantan and Sumatra Islands, which are the main locations of lowland dipterocarp forests (Margono et al. Citation2014).). This event implied that wood production in dipterocarp forests decreased by 80% from 1992 to 2017 (Nawir and Murniati Citation2008; MOEF Citation2018). Conversely, in 2017, the total timber production dominated by dipterocarp wood from natural forest concessions in Indonesia was 5.4 M m3 (MOEF Citation2018) although some dipterocarps are categorized as endangered species (Ashton Citation2004; Maycock et al. Citation2012). This finding indicates that dipterocarp species are still a major target for timber harvesting from lowland dipterocarp forest concessions under the selective cutting silvicultural system.
One of the methods to prevent lowland dipterocarp forest is enrichment planting that involves native species. Many studies have discussed enrichment planting in tropical forest fragmentation (Sovu et al. Citation2010; Mangueira et al. Citation2019) and species selection for rehabilitation (Otsamo, Adjers, et al. Citation1996; Otsamo, Otsamo, et al. Citation1996; Willoughby et al. Citation2007). The parameters for species selection are the survival rate and growth (i.e. diameter) because those parameters could reflect the adaptation and ecological process of the species in the particular forest (Franklin et al. Citation1987; Schneider et al. Citation2014). The survival rate of species, especially shade-tolerant species could be used to describe ecological succession (Schneider et al. Citation2014) and the adaptation of the species in the degraded forest (Sovu et al. Citation2010; Nguyen et al. Citation2016). Moreover, the native species is mainly harvested as sawn timber, thus diameter growth is an important parameter to produce the high wood quality. However, the selection of species for planting is based on early growth, so recommending a particular species for rehabilitation is difficult (Otsamo, Adjers, et al. Citation1996; Otsamo, Otsamo, et al. Citation1996; Willoughby et al. Citation2007; Widiyatno et al. Citation2014). Furthermore, the wood properties of tropical rainforest species have yet to be fully explored, and wood properties affect wood processing and final products. Thus, the species selection for planting should consider growth, wood properties, and planting goal, such as industrial wood production, environmental protection, and rural development (Evans and Turnbull Citation2004; Montagnini Citation2005).
Enrichment planting with dipterocarps as native species in the lowland dipterocarps forest is needed to increase forest productivity and protect these species from extinction, considering that the size and wood production in tropical rainforests have declined. In the present study, the growth and wood properties of selected dipterocarp species were monitored 12.5 years after planting to find the most suitable species for rehabilitation of tropical rainforest by enrichment planting. The survival rate, DBH growth parameters, and wood properties (pilodyn penetration depth [PP] and stress wave velocity [SWV)] of dipterocarp species were measured.
Method
Study sites
This study was carried out in Central Borneo, Indonesia (00°36′–01°10′ South Latitude and 111°39′–112°25′ East Longitude; ). The research site was a lowland dipterocarp forest dominated by dipterocarp species managed with selective cutting (Tebang Pilih Tanam Indonesia, TPTI) and selective cutting enriched with line planting (Tebang Pilih Tanam Jalur, TPTJ) (MOF Citation2009). Thus, an enrichment planting in the TPTJ system is required after logging (MOF Citation2009; Kettle Citation2010; Lamb Citation2014). However, under the TPTI system, enrichment planting is conducted if the number of the stock of commercial species, especially dipterocarp species, is insufficient because the survival rate of commercial species is very low because of destructive logging methods (Appanah Citation1998).
Experimental design
The species selection trial of dipterocarps was established on a randomized complete block design with four blocks as replication and planted at a spacing of 6 m × 3 m. In this research, 23 native Dipterocarpaceae species were used: Shorea leprosula, S. platyclados, S. johorensis, S. ovalis, S. parvifolia, S. macrophylla, S. pinanga, S. scaberima, S. smithiana, S. dasyphylla, S. leavis, S. fallax, S. macroptera, S. atrivervosa, S. seminis, S. guiso, S. virescens, S. singkawang, S. blumutensis, S. lamellata, Hopea mangerawan, Dipterocarpus retusus, and D. caudiferus. Each species in every block was assigned a 3 × 10 tree plot (30 seedlings). Dipterocarp seeds were collected at the mass flowering and fruiting stages of dipterocarps from January to February 2005. The seeds were maintained in the nursery for 12 months before they were transplanted in the field. The selected species were planted with the gap planting method and the land preparation was done by cutting all the vegetations in the secondary forest with a planting hole of approximately 40 cm × 40 cm × 30 cm and a spacing of 6 m × 3 m (Widiyatno et al. Citation2014).
The following data were collected: survival rate, DBH, PP, and SWV. The survival rate was calculated by dividing the number of surviving individuals with the total number of the trees originally planted in each block. The DBH was measured at 1.3 m from the root collar by using a diameter caliper. PP, an indirect parameter used to measure wood density, was assessed in various species by using a pilodyn tester (Pilodyn 6 J Forest) in accordance with previously described methods (Ishiguri et al. Citation2008, Citation2012; Shi-jun et al. Citation2010; Wu et al. Citation2010; Hidayati et al. Citation2013; Chen et al. Citation2015; Fundova et al. Citation2018). PP was measured at the DBH on two positions for each tree without removing the bark of each tree and on the same side of all trees. Thus, the mean value of PP for each tree was calculated. The SWV of a standing tree could be measured with a commercially available hand-held stress wave timer (FAKOPP Microsecond Timer, FAKOPP Enterprise, Hungary), which is a nondestructive sampling tool, to examine the dynamic process related to the physical and mechanical properties of wood (Wang et al. Citation2000, Citation2004; Knowles et al. Citation2004; Ishiguri et al. Citation2007). The resulting signals traveling between the start and stop signals were recorded as stress wave transmission time (Treading; µs). The formula used to calculate the stress wave transmission time per length basis is expressed as follows (Wang et al. Citation2000, Citation2004; Chauhan and Walker Citation2006):
(1)
(1)
where Tm (µs/m) is the transmission time per length basis (μs/m), Treading is the average stress wave reading (μs; 6 replications per tree), and LD is the length of the distance between the first and second stop probes (m).
SWV (km/s) was calculated using the following equation (Wang et al. Citation2000, Citation2004; Nakai et al. Citation2019):
(2)
(2)
Data were analyzed through one-way analysis of variance (ANOVA) by using SPSS version 23.0 to determine the significant differences in all the variables at tα 0.05 probability. Significant differences in the parameters of dipterocarp species were further examined via Tukey’s test.
On the other hand, the clustering of group dipterocarps species was conducted to select the group species for enrichment planting in the secondary tropical rain forest. The group of dipterocarps species was analyzed by the hierarchical cluster analysis based on some characteristics i.e. survival rate, DBH, PP, and SWV by the hclust function in R package (R Developed Core Team). Moreover, the optimal cluster to find the subgroups of species within the trial was calculated by the Elbow method and the average silhouette method in R package (R Developed Core Team).
Results
Survival rate
The survival rate of dipterocarp species 12.5 years after planting was significantly different among species (F = 2.40; p = 0.03; ). The survival rate of S. leprosula was the highest (85%; ). The survival rate of other species, namely, S. macrophylla, S. ovalis, S. johorensis, S. scaberima, S. ovalis, S. fallax, S. parvifolia, and S. pinanga, more than 60% ().
Table 1. Analysis of variance of dipterocarp species 12.5 years after planting.
DBH growth
The ANOVA of DBH 12.5 years after planting showed significant differences among species (F = 6.67; p < 0.0001; ). The best growth of DBH variables was observed in S. platyclados, whose average DBH at 12.5 years after planting were 27.62 cm (mean annual diameter increment [MADI] = 2.2 cm/year) (). Meanwhile, the diameter growth of other dipterocarp species, i.e. S. macrophyla, S. ovalis, S. parvifolia, S. leprosula, S. scaberima, S. dasyphylla, and S. johorensis, was not significant difference with S. platyclados ().
PP and SWV
PP was significantly different among dipterocarp species (F = 14.19; p < 0.001), whereas SWV was not significantly different among dipterocarp species (F = 1.26; p = 0.234; ). The highest PP was observed in S. dashyphylla (33.53 mm), whereas the lowest PP was found in S. seminis (15.98 mm; ). The SWV among dipterocarp species ranged from 3.65 km/s to 4.18 km/s (). Pearson’s correlation between PP and DBH was 0.42 (p < 0.001). SWV was not significantly correlated with DBH and PP. Pearson’s correlations of SWV-DBH and SWV-PP were 0.034 and 0.016, respectively (p > 0.05; ).
Figure 4. Pairwise correlations (Pearson’s) among DBH, pilodyn penetration, and stress wave velocity of dipterocarp species 12.5 years after planting. Note: DBH: diameter at breast height (1.3 m above ground); PP: pilodyn penetration depth; SWV: stress wave velocity.
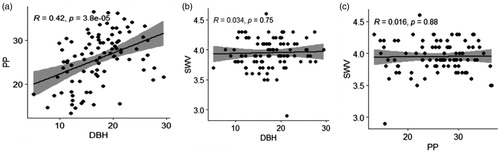
Table 2. Analysis of variance of pylodin and stress wave velocity of dipterocarp species 12.5 years after planting.
Table 3. Average Pilodyn Penetration Depth (PP), transmission time per length basis (Tm) and stress wave velocity (SWV) of dipterocarp species 12.5 years after planting.
Hierarchical cluster analysis
The twenty trees species of dipterocarps were aggregated into five groups based on four characteristic traits, i.e. survival rate, DBH, PP, and SWV, using hierarchical cluster analysis with the Elbow method and the silhouette method (). The first, second, and third groups were dominated by the species with MADI ≥ 1.5 cm/year. They were Shorea leprosula, S. macrophylla, S. ovalis, S. johorensis, S. pinanga, S. falax, S. scaberima, S, parvifolia, S. dasyphylla and S. platyclados. Meanwhile, the fourth and fifth groups were dipterocarps with MADI range 0.8–1.4 cm/years ( and ).
Discussions
Survival rate
The survival rate of dipterocarp is correlated with the availability of sunlight and moisture (Sakai et al. Citation2011, Citation2013; Daisuke et al. Citation2013; Ghazoul Citation2016). The survival rate of all dipterocarp species decreased in the early growth stage and became stable 6 years after planting when the site’s microclimate was favorable to light-demanding and shade-tolerant dipterocarp species (). This result indicated that the gap planting in our species trial increased the light intensity in the early establishment, reduced the moisture, increased the water stress, and increased the mortality of some dipterocarp species, especially shade-tolerant dipterocarps in the early growth. For instance, the survival rates of light-demanding dipterocarp species, e.g. S. leprosula, S. macrophylla, S. ovalis, S. johorensis, and S. parvifolia (Phillips et al. Citation2002), were higher than those of shade-tolerant species, e.g. Hopea mangerawan, S. leavis, and Dipterocarpus caudiferus (). This difference was due to the lower density and size of the stomata of light-demanding species, i.e. S. leprosula, than those of a shade-tolerant species, i.e. H. mangerawan. Thus, species with lower stomatal densities would use water efficiencies and more adaptive with drought condition (Ashton and Berlyn Citation1992). This result suggested that shade-tolerant species have a higher chance of survival in a shaded understory than light-demanding species. Shade-tolerant species adapt after the site’s microclimate may favor their growth whereas light-demanding species adapt in the gap planting method.
DBH growth, PP an SWV
Our result suggested that dipterocarp species had different DBH growth because of differences in sunlight requirements during early development. The highest growth (i.e. S. macrophylla, S. leprosula, S. johorenis, S. parvifolia, S. ovalis, S. scaberima, and S. dasyphylla) was detected in light-demanding and fast-growing dipterocarp species (Appanah and Weinland Citation1993, Citation1996; Phillips et al. Citation2002) except S. platyclados, which was classified as a shade-tolerant and fast-growing dipterocarp species (Sasaki Citation2006). As light-demanding dipterocarp species, they require full sunlight at the early stage of growth (Appanah and Weinland Citation1993, Citation1996; King et al. Citation2005) and succeed in large gap planting for their growth (Whitmore and Brown Citation1996). This phenomenon occurred because the net photosynthetic rate of the fast-growing dipterocarp species in gap planting was 5.7 times higher than that of the slow-growing species (Mori Citation2001). This result also indicated that the fast-growing dipterocarp species were more tolerant to drought and transplanting stress. Furthermore, S. platyclados and other species, such as shade-tolerant ones during early development, require light at least after the sapling stage (Appanah and Weinland Citation1993, Citation1996; Sasaki Citation2006). The land prepared with gap planting can cause a low survival rate, dieback, reduced high growth, and bushy crown formation (Appanah and Weinland Citation1996). This result suggested that many shade-tolerant dipterocarp species need mild environmental conditions in their early growth.
The MADI of some slow-growing dipterocarp species, namely, Dipterocarpus sp., S. guiso, S. blumutensis, S. leavis, S. macroptera, S. seminis, S. lamellata, S. singkawang, and D. caudiferus, was less than 1.2 cm/year. They are mainly categorized as heavy-hardwood dipterocarp species that are less responsive to increasing light intensity than light-hardwood dipterocarp species (Manokaran and Konchummen Citation1992). However, their MADI in our research was higher than the growth of dipterocarps (less than 0.5 cm/year) in the secondary dipterocarp forests in Sarawak (Bischoff et al. Citation2005). This finding implied that the growth of light-hardwood species was faster than that of heavy-hardwood species, and the minimum ages at which they could be harvested were less than 26 years and more than 33 years, respectively.
In term wood properties, some indicators, i.e. wood density and stiffness, which can be measured with pilodyn and FAKOPP, respectively, are used to measure the wood quality of solid and fiber products (Pilai et al. Citation2013; Defo et al. Citation2016; Ramananantoandro et al. Citation2016). Our research showed that various dipterocarp species showed significantly different PP () because of the differences in their phylogenetic group (Kenzo et al. Citation2017) and the light requirement for their growth (King et al Citation2005; Van Gelder et al. Citation2006; Rana et al. Citation2009; Ramananantoandro et al Citation2016). The highest PP was observed in S. dasyphylla, S. leprosula, and S. parvifolia, which formed a group of light-demanding and light-hardwood dipterocarp species. The high PP also indicated a low wood density because the increased PP corresponds to a decreased wood density. The slow growth of dipterocarp classified as a heavy hardwood (i.e. S. seminis, H. mangerawan, S. leavis, D. caudiferus, S. singkawang, and S. lamellate) had a low PP, indicating high-wood density (; ). Similarly, other studies have reported that slow growth is observed in high-wood density than in fast-growing species (Ramananantoandro et al. Citation2016; Muller-Landau Citation2004). In the more dense secondary forest, S. platyclados was used as a shade-tolerant species for planting because it has a high growth and a medium PP. This result suggested that different species would have varying PP.
SWV can be used to estimate stiffness (Knowles et al. Citation2004; Wu et al. Citation2010) and assess the mechanical properties of wood in standing trees (Wang et al. Citation2000). Our study showed that the SWV of dipterocarp species was similar (p > 0.05). Our result was consistent with other studies reporting no correlation between different species with SWV (Ishiguri et al. Citation2007; Citation2011; Citation2012; Makino et al. Citation2012; Karlinasari et al. Citation2018). The SWV of heavy-hardwood dipterocarp species (e.g. H. mangerawan and S. laevis) was slightly lower than that of light-hardwood dipterocarp species (e.g. S. ovalis and S. leprosula). This result implied that Tm of a heavy-hardwood species was slightly higher than that of a light-hardwood species; therefore, wood has a high quality, and wood properties improve.
Implication of the selection of dipterocarp species for enrichment planting in secondary tropical rainforests
Our result showed that each dipterocarp species has different light requirements for its growth (Appanah and Weinland Citation1996; Philipson et al. Citation2012). Dipterocarps can be divided into three groups, namely, light-demanding and fast-growing species, shade-tolerant and fast-growing species, and shade-tolerant and slow-growing species (Phillips et al. Citation2002). In terms of growth and survival rate, some dipterocarp species used to rehabilitate degraded secondary forests are S. macrophyla, S. ovalis, S. parvifolia, S. leprosula, and S. johorensis via gap planting or strip planting method because they species should not be planted under a canopy to achieve optimal growth (Adjers et al. Citation1995). However, they have PP corresponding to that of light-hardwood species, so they are widely used for plywood, specifically as face and core veneer (Martawijaya et al. Citation2005). Moreover, shade and slow-growing species, e.g. S. laevis and H. mengarawan, have shallowness the pilodyn penetration categorized as a heavy hardwood of dipterocarps. Thus, these species can be used for flooring, decking, heavy carpentry, ship building, and other similar purposes (Martawijaya et al. Citation2005; Gerard et al. Citation2017). On the other hand, the species for rehabilitating degraded secondary forest could be selected based on some traits, i.e. survival rate, DBH, PP, and SWV. Thus, clustering analysis elucidated five groups of dipterocarps species (). The first group consisted of 3 species that were S. leprosula, S. macrophylla, and S. ovalis. This group indicated that they have high survival rate, and DBH growth, but the wood quality (PP and SWV) was categorized as light-hard wood. However, the third group was only S. platyclados because this species was high growth and high wood quality, but it has a low survival rate due to the low survival rate in the degraded tropical rain forest.
Furthermore, choosing the dipterocarp species for enrichment planting in secondary forests depends on the objective of a landowner (Evans and Turnbull Citation2004; Montagnini Citation2005) and the state of the land degradation of an initially forested ecosystem (Ashton and Kelty Citation2018) to achieve a sustainable forest management. Furthermore, in secondary lowland dipterocarp forests under selective cutting, enrichment planting can be conducted if the number of the stock of commercial species, especially dipterocarp species, is insufficient (Appanah Citation1998). The advantages of enrichment by using native species in a logged forest via strip planting and gap planting are the increased biodiversity (Ashton et al. Citation2001; Kettle Citation2010) and the ability to maintain the productivity of commercial trees in tropical regions (Adjers et al. Citation1995; Appanah and Weinland Citation1996; Vincent and Davies Citation2003; Kettle Citation2010; Lamb Citation2014) and their ecological services. Enrichment planting with native species can be applied to a local environment and contribute to support biodiversity and ecosystem functions (Ashton et al. Citation2001; Thomas et al. Citation2014) because dipterocarp species are categorized as nearly threatened, vulnerable, and endangered species (Pooma et al. Citation2017).
Conclusions
The selection of species for enrichment planting that involved native species was important to maintain the diversity of late succession species and the productivity of secondary forests. Some species recommended for planting on degraded secondary lowland dipterocarp forests for based on a hierarchical cluster analysis were S. leprosula, S. macrophyla, and S. ovalis. because their survival rate, MADI were more than 67%, and >1.7 cm/year, respectively. In the more dense secondary forest, S. platyclados was used as a shade tolerant for planting because of its MADI of 2.2 cm/year. Moreover, a shade, a slow-growing, and a heavy-hardwood species, e.g. S. laevis and H. mangerawan, could be selected for enrichment planting in the dense secondary forest and construction purposes. In conclusion, the key success of planting depends on the objective and species screening of plantation. Enrichment planting should also maintain the tree density and diversity of native species to achieve the sustainable management of secondary lowland dipterocarp forest.
Acknowledgments
We would like to thank the Dean of the Faculty of Forestry for allowing us to conduct this research. In particular, we are gratitude to the R&D staff of PT SBK for their help in carrying out this experiment, especially for frequently maintaining and conducting measurements in this research.
Disclosure statement
No potential conflict of interest was reported by the author(s).
Additional information
Funding
References
- Adjers G, Hadengganan S, Kuusipalo J, Nuryanto J, Vesa L. 1995. Enrichment planting of dipterocarps in logged-over secondary forests effect of width, direction and maintenance method of planting line on selected Shorea species. For Ecol Manage. 73(1–3):259–270.
- Appanah S, Weinland G. 1993. Planting quality timber trees in Peninsular Malaysia. Kepong: Forest Research Institute Malaysia.
- Appanah S, Weinland G. 1996. Experience with planting dipterocarps in Peninsular Malaysia. In: Schulte A, Schone D, editors. Dipterocarp forest ecosystems: toward sustainable management. Singapore: World Scientific Publishing Co. Pte. Ltd; p. 411–445.
- Appanah S. 1998. Management of natural forests. In: Appanah S, Turnbull JM, editors. A review of Dipterocarps: taxonomy, ecology and silviculture. Bogor: Center for International Forestry Research; p. 133–149.
- Ashton MS, Berlyn GP. 1992. Leaf adaptations of some Shorea species to sun and shade. New Phytol. 12(4):587–596.
- Ashton PS. 1982. Dipterocarpaceae: flora Malesiana, Series I. Netherlands: Martinus Nijhoff Publishers.
- Ashton PS. 2004. Dipterocarpaceae. In: Soepadmo E, Saw LG, Chung RCK, editors. Tree flora of Sabah and Sarawak. Kuala Lumpur: Forest Research Institute Malaysia, Kuala Lumpur, Sabah Forestry Department, Sandakan and Sarawak Forestry Department, Kuching.
- Ashton MS, Gunatilleke CVS, Singhakumara BMP, Gunatilleke IAUN. 2001. Restoration pathways for rain forest in southwest Sri Lanka: a review of concepts and models. For Ecol Manage. 154(3):409–430.
- Ashton MS, Kelty MJ. 2018. The practice of silviculture: applied forest ecology. New York: John Wiley & Sons.
- Bischoff W, Newbery DM, Lingenfelder M, Schnaeckel R, Petol GH, Madani L, Ridsdale CE. 2005. Secondary succession and dipterocarp recruitment in Bornean rainforest after logging. Fore Ecol Manage. 218(1–3):174–192.
- Chauhan SS, Walker JCF. 2006. Variations in acoustic velocity and density with age, and their interrelationships in radiata pine. For Ecol Manage. 229(1-3):388–394.
- Chen Z-Q, Karlsson B, Lundqvist S-O, García Gil MR, Olsson L, Wu HX. 2015. Estimating solid wood properties using Pilodyn and acoustic velocity on standing trees of Norway spruce. Ann for Sci. 72(4):499–508.
- Daisuke H, Kenzo T, Yamauchi N, Irino KO, Kendawang JJ, Ninomiya I, Sakurai K. 2013. Effects of environmental factors on growth and mortality of Parashoreamacrophylla (Dipterocarpaceae) planted on slopes and valleys in a degraded tropical secondary forest in Sarawak, Malaysia. Soil Sci Plant Nutr. 59(2):218–228.
- Dawkins HC, Philip M. 1998. Tropical moist forest silviculture and management: a history of success and failure. Wallingford: CAB International.
- Defo M, Duchesne I, Steward J. 2016. A review of the current state of wood quality modelling and decision support systems in Canada. Canada: Canadian Wood Fibre Centre. Information Report FI-X-012.
- Evans J, Turnbull J. 2004. Plantation forestry in the tropics. New York (NY): Oxford University Press.
- Finkeldey R. 2011. Management of forest genetic resources. In: Günter S, Weber M, Stimm B, Mosandl R, editors. Silviculture in the tropics. Berlin Heidelberg: Springer-Verlag; p. 109–118.
- Franklin JF, Shugart H, Harmon ME. 1987. Tree death as an ecological process. BioScience. 37(8):550–556.
- Fundova I, Funda T, Wu HX. 2018. Non-destructive wood density assessment of Scots pine (Pinus sylvestris L.) using Resistograph and Pilodyn. PLoS One. 13(9):e0204518NY.
- Gerard J, Guibal Paradis S, Cerre J. 2017. Tropical timber atlas: technological characteristic and uses. Paris: ITTO and CIRAD.
- Ghazoul J. 2016. Dipterocarp biology, ecology, and conservation. Oxford: Oxford University Press.
- Hidayati F, Ishiguri F, Iizuka K, Makino K, Tanabe J, Marsoem SN, Na’iem M, Yokota S, Yoshizawa N. 2013. Growth characteristics, stress wave velocity, and Pilodyn penetration of 15 clones of 12-year-old Tectona grandis trees planted at two different sites in Indonesia. J Wood Sci. 59(3):249–254.
- Huth A, Ditzer T. 2001. Long-term impacts of logging in a tropical rainforest: a simulation study. For Ecol Manage. 142(1–3):33–51.
- Ishiguri F, Eizawa J, Saito Y, Iizuka K, Yokota S, Priadi D, Sumiasri N, Yoshizawa N. 2007. Variation in the wood properties of Paraserianthes falcataria planted in Indonesia. Iawa J. 28(3):339–348.
- Ishiguri F, Matsui R, Iizuka K, Yokota S, Yoshizawa N. 2008. Prediction of the mechanical properties of lumber by stress wave velocity and Pilodyn penetration of 36-year-old Japanese larch trees. Holz Roh Werkst. 66(4):275–280.
- Ishiguri F, Takeuchi M, Makino K, Wahyudi I, Takashima Y, Iizuka K, Yokota S, Yoshizawa N. 2012. Cell morphology and wood properties of Shorea acuminatissima planted in Indonesia. Iawa J. 33(1):25–38.
- Ishiguri F, Wahyudi I, Takeuchi M, Takashima Y, Iizuka K, Yokota S, Yoshizawa N. 2011. Wood properties on Pericopsis mooniana grown in a plantation in Indonesia. J Wood Sci. 57(3):241–246.
- Karlinasari L, Andini S, Worabai D, Pamungkas P, Budi SW, Siregar IZ. 2018. Tree growth performance and estimation of wood quality in plantation trials for Maesopsis eminii and Shorea spp. J for Res. 29(4):1157–1166.
- Kartawinata K, Riswan S, Gintings AN. 2001. An overview of post-extraction secondary forests in Indonesia. J Trop for Sci. 13(4):621–638.
- Kenzo T, Sano M, Yoneda R, Chann S. 2017. Comparison of wood density and water content between dry evergreen and dry deciduous forest trees in central Cambodia. JARQ. 51(4):363–374.
- Kettle CJ. 2010. Ecological considerations for using dipterocarps for restoration of lowland rainforest in Southeast Asia. Biodivers Conserv. 19(4):1137–1151.
- King DA, Davie SJ, Supardi NN, Tan S. 2005. Tree growth is related to light interception and wood density in two mixed dipterocarp forests of Malaysia. Funct Ecol. 19(3):445–453.
- Knowles LR, Hansen LW, Wendding A, Downes G. 2004. Evaluation of non-destructive methods for assessing stiffness of Douglas fir trees. N Z J for Sci. 34(1):87–101.
- Lamb D. 2014. Post-establishment enrichment of restoration plots with timber and nontimber species. In: Bozzano M, Jalonen R, Thomas E, Boshier D, Gallo L, Cavers S, Bordács S, Smith P, Loo J, editors. Genetic considerations in ecosystem restoration using native tree species. Rome: State of the World’sForest Genetic Resources–Thematic Study, FAO and Bioversity International.
- Makino K, Ishiguri F, Wahyudi I, Takashima Y, Iizuka K, Yokota S, Yoshizawa N. 2012. Wood properties of young Acacia mangium trees planted in Indonesia. For Prod J. 62(2):102–106.
- Mangueira JRSA, Holl KD, Rodrigues RR. 2019. Enrichment planting to restore degraded tropical forest fragments in Brazil. Ecosystems and People. 15(1):3–10.
- Manokaran N, Konchummen KM. 1992. Tree growth in primary lowland and hill dipterocarp forests. J Trop for Sci. 6(3):332–345.
- Margono BA, Potapov PV, Turubanova S, Stolle F, Hansen MC. 2014. Primary forest cover loss in Indonesia over 2000–2012. Nature Clim Change. 4(8):730–735.
- Martawijaya A, Kartasujana I, Kadir K, Prawira SA. 2005. Indonesian wood atlas. Vol I. Jakarta: Forest Research and Development Agency-Ministry of Forestry Republic of Indonesia. p. 171.
- Maycock CR, Kettle CJ, Khoo E, Pereira JT, Sugau JB, Nilus R, Ong RC, Amaludin NA, Newman MF, Burslem DFRP. 2012. A revised conservation assessment of dipterocarps in Sabah. Biotropica. 44(5):649–657.
- Ministry of Environment and Forestry (MOEF). 2018. State of Indonesia’s forest 2018. Jakarta: Ministry of Environment and Forestry of Indonesia.
- Ministry of Forestry (MOF). 2009. Decree no: 11/Menhut-II/2009: silvicultural system in the natural forest productions. Jakarta: Ministry of Forestry-Indonesia.
- Montagnini F. 2005. Selecting tree species for plantation. In: Mansourian S, Vallauri D, Dudley N, editors. Forest restoration in landscapes beyond planting trees. New York: Springer; p. 262–268.
- Mori T. 2001. Rehabilitation of degraded forests in Lowland Kutai, East Kalimantan, Indonesia. In: Kobayashi S, Turnbull JW, Toma T, Mori T, Majid NMNA, editors. Proceedings of the rehabilitation of degraded tropical forest ecosystems 2–4 November 1999. Bogor: Center for International Forestry Research (CIFOR); p. 17–26.
- Muller-Landau HC. 2004. Interspecific and inter-site variation in wood specific gravity of tropical trees. Biotropica. 36(1):20–32.
- Myers N, Mittermeier RA, Mittermeier CG, da Fonseca GAB, Kent J. 2000. Biodiversity hotspots for conservation priorities. Nature. 403(6772):853–858.
- Nakai K, Ishizuka M, Ohta S, Timothy J, Jasper M, Lyatura NM, Shau V, Yoshimura T. 2019. Environmental factors and wood qualities of African blackwood, Dalbergia melanoxylon, in Tanzanian Miombo natural forest. J Wood Sci. 65(1):39.
- Nawir AA, Murniati RL. 2008. Forest rehabilitation in Indonesia: where to after more than three decades? Bogor: Center for International Forestry Research (CIFOR).
- Nguyen H, Vanclay J, Herbohn J, Firn J. 2016. Drivers of tree growth, mortality and harvest preferences in species-rich plantations for smallholders and communities in the tropics. PLoS One. 11(10):e0164957
- Otsamo R, Adjers G, Hadi TS, Kuusipalo J, Otsamo A. 1996. Early performance of 12 shade tolerant tree species inter planted falcataria on imperata cylindrica grassland. J Trop for Sci. 8(3):381–394.
- Otsamo R, Otsamo A, Adjers G. 1996. Reforestation experiences with dipterocarp species on grassland. In: Schulte A, Schone D, editors. Dipterocarp forest ecosystems: toward sustainable management. Singapore: World Scientific Publishing Co. Pte. Ltd; p. 464–477.
- Philipson CD, Saner P, Marthews TR, Nilus R, Reynolds G, Turnbull LA, Hector A. 2012. Light-based regeneration niches: evidence from 21 dipterocarp species using size-specific RGRs. Biotropica. 44(5):627–636.
- Phillips PD, Yasman I, Brash TE, van Gardingen PR. 2002. Grouping tree species for analysis of forest data in Kalimantan (Indonesian Borneo). For Ecol Manage. 157(1–3):205–216.
- Pilai PKC, Pandalai RC, Dhamodaran TK, Sankaran KV. 2013. Wood density and heartwood proportion in Eucalyptus trees from intensively-managed short-rotation plantations in Kerala, India. J Trop for Sci. 25(2):220–227.
- Pinard MA, Putz FE. 1996. Retaining forest biomass by reducing logging damage. Biotropica. 28(3):278–295.
- Pooma R, Newman MF, Barstow M. 2017. Shorea laevis. Cambridge (UK): The IUCN Red List of Threatened Species.
- Putz FE, Zuidema PA, Synnott T, Peña-Claros M, Pinard MA, Sheil D, Vanclay JK, Sist P, Gourlet-Fleury S, Griscom B, et al. 2012. Sustaining conservation values in selectively logged tropical forests: the attained and the attainable. Conserv Lett. 5(4):296–303.
- Ramananantoandro R, Ramanakoto MF, Rajoelison GL, Randriamboavonjy JC, Rafidimanantsoa HP. 2016. Influence of tree species, tree diameter and soil types on wood density and its radial variation in a mid-altitude rainforest in Madagascar. Ann Sci. 73(4):1113–1124.
- Rana R, Langenfeld-Heyser R, Finkeldey R, Polle A. 2009. Functional anatomy of five endangered tropical timber wood species of the family Dipterocarpaceae. Trees. 23(3):521–529.
- Sakai A, Visaratana T, Hongthong B, Vacharangkura T. 2013. Growth performance of indigenous tree species under uneven-aged forest management in Northeast Thailand. JIRCASWork Rep. 74:1–6.
- Sakai A, Visaratana T, Vacharangkura T, Ishizuka M, Nakamura S. 2011. Growth performances of three indigenous tree species planted in a mature Acacia mangium plantation with different canopy openness under a Tropical Monsoon Climate. JARQ. 45(3):317–326.
- Sasaki S. 2006. Ecology and physiology of Dipterocarpaceae. In: Suzuki K, Ishii K, Sakurai S, Sasaki S, editors. Plantation technology in tropical forest science. Tokyo: Springer-Verlag; p. 3–22.
- Schneider T, Ashton M, Montagnini F, Milan P. 2014. Growth performance of sixty tree species in smallholder reforestation trials on Leyte, Philippines. New For. 45(1):83–96.
- Shi-Jun W, Jian-Min X, Guang-You L, Vuokko L, Bao q. L, Wei W. 2010. Use of the pilodyn for assessing wood properties in standing trees of Eucalyptus clones. J Res. 21(1):68–72.
- Sist P, Sheil D, Kartawinata K, Priyadi H. 2003. Reduced-impact logging in Indonesian Borneo: some results confirming the need for new silvicultural prescriptions. For Ecol Manage. 179(1–3):415–427.
- Sodhi NS, Posa MRC, Lee TM, Bickford D, Koh LP, Brook BW. 2004. The state and conservation of Southeast Asian biodiversity. Biodivers Conserv. 19(2):317–328.
- Sovu Tigabu M, Savadogo P, Oden PC, Xayvongsa L. 2010. Enrichment planting in a logged-over tropical mixed deciduous forest of Laos. J For Res. 21(3):273–280.
- Symington CF (Revised by Ashton PS, Appanah S). (2004). Forester’s manual of dipterocarps (Malayan forest records no 16). 2nd ed. Kuala Lumpur: Forest Research Institute Malaysia; p. 407–422.
- Thomas E, Jalonen R, Loo J, Boshier D, Gallo L, Cavers S, Bordács S, Smith P, Bozzano M. 2014. Genetic considerations in ecosystem restoration using native tree species. For Ecol Manage. 333:66–75.
- van Gelder HA, Poorter L, Sterck FJ. 2006. Wood mechanics, allometry, and life-history variation in a tropical rainforest tree community. New Phytol. 171(2):367–378.
- Vincent A, Davies SJ. 2003. Effects of nutrient addition, mulching and planting-hole size on early performance of Dryobalanops aromatica and Shorea parvifolia planted in secondary forest in Sarawak, Malaysia. For Ecol Manage. 180(1–3):261–271.
- Wang X, Divos F, Pilon C, Nrashaw BK, Ross RJ, Pellerin RF. 2004. Assessment of decay in standing timber using stress wave timing nondestructive evaluation tools: a guide for use and interpretation. Gen. Tech. Madison (WI): Forest Products Laboratory, Department of Agriculture; Rep. FPL-GTR147.
- Wang X, Ross RJ, McClellan M, Barbour RJ, Erickson JR, Forsman JW, McGinnis GD. 2000. Strength and stiffness assessment of standing trees using a nondestructive stress wave technique. Madison (WI): Forest Products Laboratory, Department of Agriculture, USA. Research Paper FPL-RP-585.
- Whitmore TC, Brown N. 1996. Dipterocarp seedling growth in rainforest canopy gaps during six and a half years. Philos Trans R Soc B. 351:1195–1205.
- Whitmore TC. 1998. An introduction to tropical rainforests. 2nd ed. New York: Oxford Univeristy Press.
- Widiyatno S, Naiem M, Purnomo S, Setiyanto PE. 2014. Early performance of 23 dipterocarp species planted in logged-over rainforest. J Trop Sci. 26(2):259–266.
- Willoughby I, Stokes V, Poole J, White JEJ, Hodge SJ. 2007. The potential of 44 native and non-native tree species for woodland creation on a range of contrasting sites in lowland Britain. Forestry. 80(5):531–553.
- Wu SJ, Xu JM, Li GY, Risto V, Lu ZH, Li BQ, Wang W. 2010. Use of the pilodyn for assessing wood properties in standing trees of Eucalyptus clones. Forestry Research. 21(1):68–72.