Abstract
Antibiotics have the remarkable power to control bacterial infections. Unfortunately, widespread use, whether regarded as prudent or not, has favored the emergence and persistence of antibiotic resistant strains of human pathogenic bacteria, resulting in a global health threat. Bacteriophages (phages) are parasites that invade the cells of virtually all known bacteria. Phages reproduce by utilizing the host cell's machinery to replicate viral proteins and genomic material, generally damaging and killing the cell in the process. Thus, phage can be exploited therapeutically as bacteriolytic agents against bacteria. Furthermore, understanding of the molecular processes involved in the viral life cycle, particularly the entry and cell lysis steps, has led to the development of viral proteins as antibacterial agents. Here we review the current preclinical state of using phage-derived endolysins, virion-associated peptidoglycan hydrolases, polysaccharide depolymerases, and holins for the treatment of bacterial infection. The scope of this review is a focus on the viral proteins that have been assessed for protective effects against human pathogenic bacteria in animal models of infection and disease.
Introduction
Antibiotics have been critical in curing minor and life threatening infections caused by bacteria. However, bacteria are remarkably resilient and over time have developed numerous approaches to withstand attack by many of the antibiotics used today. Although the evolution of resistant strains is a natural phenomenon, the overuse and misuse of antibiotics not only in human and veterinary medicine, but also in agriculture and commercial/industrial settings may have contributed to the dramatic rise in the prevalence of these antibiotic resistant bacterial strains. With few new antibiotics in the development pipeline, resistance is an increasingly serious global threat to public health.Citation1,2 If we look back before the advent of antibiotics, viruses of bacteria called phages were used as antibacterial agents against a wide range of bacterial infections. (For history of phage therapy see refs. Citation3-6). Phages have remarkable bacteriostatic and bacteriolytic activity as part of their natural lytic lifecycle, by first disrupting the bacterium's metabolism to produce new virus particles, then lysing the host cell to release the progeny.
There is little dispute in the literature that phages have the potential to be employed as antibacterial agents in healthcare. Aside from the similarity that phages and bacteriolytic antibiotics both destroy bacterial cells, there are key differences when used therapeutically. Individual phages are largely specific for one genus or species, whereas antibiotics tend to have broad antimicrobial effects on multiple species or genera. When attempting to avoid needless casualties among the commensal microflora, a phages' specificity is advantageous. However, this specificity can also be a drawback in that vigilance is required to identify the target species and selection of lytic phages for that species in order for the therapy to be effective. Since a large variety of phages exist for any given bacterium, the current trend is the use of ‘cocktail’ preparations to ensure the presence of multiple phage that target the pathogen in a variety of mechanisms, thus reducing the relatively high frequency of phage resistance exhibited by bacteria.Citation7,8 Phage therapies are also self-dosing, increasing in numbers at the site of infection only in the presence of their host bacteria. Most phages do not directly display any risk to human health or the environment. However, phages are mobile genetic elements that can interact with their bacterial host, and in some cases mobilize genes between bacteria (horizontal gene transfer).Citation9 Consequently, viral particles that possess certain properties (i.e. virulence gene transfer) may negatively affect human health by promoting bacterial virulence and pathogenicity.
Phages can mobilize genes by means of specialized or generalized transduction. During generalized transduction, segments of the degraded bacterial DNA are mistakenly packaged in newly formed viral particles instead of viral genomes. This error transfers bacterial DNA between host bacteria. Specialized transduction is restricted to temperate phages capable of lysogeny (incorporation of a viral genome into the bacterial chromosome), whereby imprecise excision of the prophage during the lytic cycle includes flanking bacterial genes that are also packaged into newly formed viral particles and transferred to other bacteria.Citation9 Therapeutic phages must lack undesirable genes (i.e., toxins), and should be virulent (unable to display lysogeny) to minimize the occurrences of transduction. Notwithstanding the pros and cons of phage therapy, the critical limiting factor for the adoption of phage therapies is the paucity of internationally recognized human clinical trials. Therefore, most countries have not approved phages as therapeutic agents in humans, with the exceptions of Russia, Georgia, and Poland.Citation4
Concurrent to phage therapies, there have been efforts to develop antibacterial agents from the bacteriostatic and bacteriolytic proteins encoded by phages.Citation10,11 To date, the most notable are the phage-encoded peptidoglycan hydrolases (PGH), called endolysins. Endolysins are in part responsible for releasing newly formed viral particles by degrading peptidoglycan, which destabilizes the cell wall and causes the host cell to burst. Some phages also encode a second PGH type, namely virion-associated peptidoglycan hydrolase (VAPGH). In contrast to endolysins, VAPGH degrade localized peptidoglycan during infection, i.e. the initial cell wall penetration. When purified, both PGH types can be bacteriolytic when applied exogenously to cells. Phages also encode polysaccharide depolymerases that degrade the macromolecule carbohydrates of the bacterial cell wall envelope. Extracellular polysaccharides can protect the bacterium from desiccation, antimicrobials, and host immune systems.Citation12 Depolymerases assist the viral particle in adsorbing, invading, and disintegrating the host bacteria, an activity that can continue even within biofilms. Holins are responsible for disruption of the cytoplasmic membrane to assist endolysins in cell lysis. The origin and remarkable diversity of endolysins,Citation10,11 VAPGH,Citation13 depolymerasesCitation14 and holinsCitation15,16 have been reviewed previously.
This review will illustrate how phage-encoded endolysins, VAPGH, polysaccharide depolymerases, and holins all have antibacterial properties that may offer new therapeutics to treat bacterial infections and disease in humans. Here we discuss recent insights into their therapeutic development, and draw attention to several preclinical animal models related to human diseases caused by bacteria, in particular those caused by antimicrobial-resistant strains.
Endolysins – Peptidoglycan Degrading Enzymes
Endolysins are responsible for degrading the bacterial cell wall peptidoglycan during the late stages of lytic phage replication, causing the cell to burst and release newly formed viral particles ().Citation16 This well-choreographed event occurs after the endolysin has accumulated in the cytoplasm, and translocated through holes formed in the plasma membrane by holins. Since the peptidoglycan layer provides structural integrity and rigidity to the cell, degrading this layer leads to cell wall instability and eventual rupture due to differences in cellular and environmental osmotic pressures (osmolysis) (). This is particularly true for Gram-positive bacteria that lack an outer membrane. Endolysin-induced lysis of Gram-negative cells is slightly more involved, requiring further disruption of the outer membrane by spanin complexes, which catalyze fusion of the inner and outer membranes. (For review on spanins see refs. Citation16, 17.)
Figure 1. Schematic of Gram-positive bacterial cell lysis by exogenously applied endolysins. Endolysin cleave bonds within the peptidoglycan and the high internal pressure of cell protrudes the plasma membrane resulting in osmolysis (lysis from without). A generalized peptidoglycan structure illustrates cleavage sites by endolysin types: glycocidases (namely glucosaminidase and muramidases), amidases, and endopeptidases (dotted box).
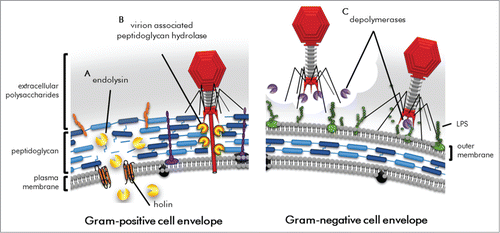
Figure 2. Schematic of bacterial cell envelope attacked by phage-encoded proteins during lytic replication. (A) Endolysins and holins work in concert for cell lysis during the final stages of replication. (B) Virion-associated peptidoglycan hydrolases are used by certain phage to puncture through the bacterial cell envelope. (C) Depolymerases degrade the extracellular polysaccharides and lipopolysaccharides that can be barriers to viral cell surface adsorption.
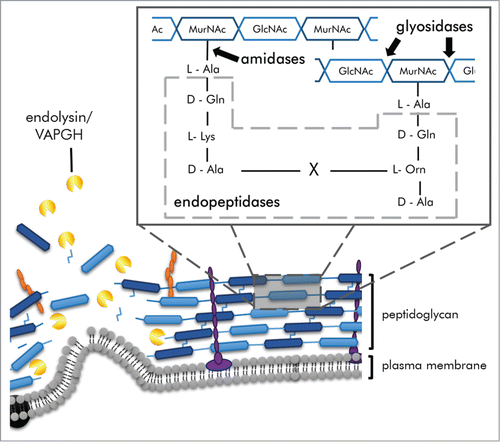
The peptidoglycan layer is unique and highly conserved among bacteria, though is substantially thicker in Gram-positive bacteria (20 – 80 nm) than in Gram-negative bacteria (10 nm).Citation18 The peptidoglycan matrix is composed of linear strands of alternating N-acetylmuramic acid (MurNAc) and N-acetylglucosamine (GlcNAc) residues (Fig. 2). Amide bonds between the MurNAc residues and the conserved first stem peptide L-alanine covalently link the linear strands to stem peptides. Stem oligopeptides are composed of L- and D- form amino acids that cross-link either to opposing stem peptides directly, or by an interpeptide bridge. (For review of peptidoglycan structures see ref. Citation18.) Endolysins are classified based on their catalytic activity site within the peptidoglycan structure: (i) glycosidase, those that cleave between glycan residues, (ii) amidase, those that cleave the amide bond between MurNAc and the first highly conserved stem L-alanine residue, and (iii) endopeptidases, which cleave peptide bonds between two amino acid residues. Glycosidases are further categorized as muramidases that cleave linkages between MurNAc and GlcNAc (similar to lysozymes), or as glucosaminidases that cleave linkages between GlcNAc and MurNAc (common in autolysins). The majority of the assigned catalytic activities in literature have been based on protein sequence homology, and not confirmed biochemically (e.g. analysis of peptidoglycan digestion products via techniques such as mass spectrometry).Citation10 In some cases, sequence analysis homologies have turned out to be inconsistent with biochemically verified catalytic sites.Citation19 (For an overview of endolysin types and activities, see refs.)Citation10,11
Endolysin derived from phages that infect Gram-positive hosts typically have a modular domain structure composed of N-terminal enzymatically active domain (EAD), and a C-terminal cell wall binding domain (CBD) connected by a short linker region (). However, proximity and orientation of these domains is not universally conserved between endolysins (i.e., a CBD can be located on the N-terminus, mid-protein, C-terminus, or be absent). Although this multi-domain organization is dominant among endolysins from Gram-positive phage, it is not common among endolysins from Gram-negative phage,Citation20-22 which are generally globular and lack CBDs.Citation23 The number of EADs in the modular endolysins from Gram-positive-infecting phage also varies, with several having two, each encoding a different catalytic activity.Citation19,24,25 For instance, the staphylococcal phage phi11 endolysin has both an endopeptidase and an amidase.Citation26 Likewise, the streptococcal phage endolysin B30 has both an endopeptidase and a muramidase EAD.Citation27 However, site-directed mutagenesis and deletion analysis of dual EAD enzymes has indicated that one of the EAD tends to be dominant and highly catalytic, whereas the other is virtually inactive in vitro. For example, both the phi11 amidase and the staphylococcal phage K endolysin, LysK, have a highly active cysteine/histidine-dependent amidohydrolase/peptidase (CHAP) EAD and poorly active amidase EAD.Citation28,29-31 Similarly, the second EAD (glucosaminidase) from the streptococcal prophage LambdaSa2 endolysin is seemingly silent, with a highly active N-terminal endopeptidase EAD.Citation32 The quandary arises when asking the question: Why would catalytically inactive domains be evolutionarily maintained? It is plausible that these domains are in fact active, but mutagenesis or deletion analysis interferes with proper folding of the protein. Additionally, lytic and biochemical assays may not recreate the microenvironment of the periplasm where endolysins encounter peptidoglycan. Donovan and Foster-Frey have discussed this in more detail with other considerations.Citation32
Cell wall binding of the endolysin is often necessary for bacteriolytic activity,Citation29,32,33 and endolysins often exhibit near species-specificity.Citation34,35 In rare cases endolysins can be serovar-specific, such as the L. monocytogenes phage endolysins Ply118 and Ply500 each unique to different serovars.Citation36,37 Endolysins with an intact CBD can also have a broad host range, such as with the streptococcal phage endolysin PlyC, which lyses several streptococcal species.Citation38 Even more promiscuous is the enterococcus phage φ1 endolysin PlyV12, which lyses E. faecalis and several streptococcal and staphylococcal species.Citation39 Uniquely, the Bacillus phage endolysin PlyG lyses both vegetative cells and germinating spores of B. anthracis. This endolysin has multiple binding domains, one for peptidoglycan of vegetative cells and another for the spore surface.Citation40 In contrast, CBD are not always required for lytic activity,Citation31,41 and in some cases CBD removal can improve bacteriolytic potency and/or lytic spectrum (see ‘Engineering novel endolysin constructs' section below for more details).
Endolysins are typically the product of a single gene in a single open reading frame. The only exception is the streptococcal C1 phage endolysin PlyC that consists of two separate proteins-a single PlyCA moiety that contains two functional EAD tethered to a ring-shaped CBD composed of 8 PlyCB subunits.Citation42 The two proteins are transcribed from two genes located in a single operon and separated by an intron-like sequence.Citation38,42 Furthermore, introns are rare in endolysin genes, but other exceptions do exist.Citation25
Engineering novel endolysin constructs
The modular structure of endolysins provides an opportunity to engineer enzymes with altered bacteriolytic activity. Exchanging the CBD of S. aureus phage endolysin Ply187 with the Src Homology 3b (SH3b) CBD, from the endolysin LysK, improved staphylolytic activity of the chimeric enzyme tenfold over native Ply187.Citation43 Likewise, exchanging the Ply187 CBD with a non-SH3b CDB from phiNM3, another S. aureus phage endolysin, creates the chimeric enzyme ClyH which yields a 14-fold increase in staphylolytic activity against the strains tested. ClyH also has a broader lytic spectrum to include staphylococcal species not lysed by the native Ply187.Citation44 There are other examples where swapping CBDs can expand a chimeric enzyme's bacteriolytic spectrum outside of the native endolysins species-specificity. For example, Becker et al. swapped the streptococcal prophage LambdaSa2 endolysin Cpl-7 CBD for the SH3b CBD from staphylococcal endolysin LysK, which drastically improved the chimeric enzymes' staphylolytic activity while maintaining the streptolytic activity.Citation30,32 Similarly, fusing additional heterologous CBD in tandem can also extend an enzyme's lytic spectrum by expanding its recognition and binding properties, as demonstrated by fluorescent-tagged Listeria endolysin CBD fusions.Citation45 However, in contrast to a CBD being required for an EAD to be active (above), for some EADs the presence of a CBD is not necessary for lytic activity. Deleting the B. anthracis phage endolysin PlyL CBD abolished anthracis-specificity and broadened lytic activity to include several Bacillus species.Citation46 Removal of the CBD may also increase endolysins potency. The truncated form of Ply187, containing just an EAD, has a lower minimum inhibitory concentration (MIC) than the full-length endolysin.Citation47 Similarly, Horgan et al. reported that the isolated CHAP domain from the endolysin LysK had a twofold higher staphylolytic activity than the full-length endolysin.Citation31 Removal of terminal region amino acids from an endolysin can also improve an enzyme's bacteriolytic potency.Citation48 An N-terminal deletion of the Clostridium difficile phage endolysin CD27L improved bacteriolytic activity, despite only removing regions outside the predicted EAD and CBD. In addition, the deletion broadened the mutant enzyme's lytic range.Citation48 The mechanism for these outcomes was not determined. Likewise, the prophage LambdaSa2 endolysin has two EADs, an endopeptidase that is a highly catalytic and a poorly catalytic glycosidase.Citation19 Removal of the entire glycosidase EAD resulted in improving the truncated enzyme's streptolytic activity over the larger parental endolysin.Citation32 However, the hypothesis that smaller enzymes are more efficient at navigating the peptidoglycan matrix making them catalytically more potent has not been demonstrated.
Some of the most dramatic changes to an endolysins' properties have occurred after fusion to a domain or peptide of non-endolysin origin. A limitation of using native endolysins therapeutically against Gram-negative bacteria is their inability to translocate across the outer membrane.Citation20-22 Yersinia pestis encodes the bacteriocin pesticin, a muramidase that can lyse closely related bacteria. Pesticin misuses the existing outer membrane Ton transport system to be transported into the periplasm and degrade the cell wall peptidoglycan.Citation49 Transport across the outer membrane requires FyuA as the transporter, and proton motive force to energize the process.Citation50 Pesticin is structurally separated into 3 domains, the N-terminal translocation domain, the receptor binding domain, and the C-terminal EAD. Buchanan and co-workers constructed a hybrid enzyme of the N-terminal domain of pesticin essential for FyuA binding, and the EAD of the E. coli phage T4 endolysin.Citation50 In a similar fashion, this hybrid enzyme was also able to bind and be actively transported into the periplasm of FyuA expressing Yersinia and uropathogenic E. coli (UPEC) strains. Once in the periplasm, peptidoglycan degradation leads to osmolysis. In addition, the hybrid enzyme was unaffected by the pesticin immunity protein (Pim), known to be harbored by many Yersinia strains.Citation50 Aritlysins is a term coined by Briers et al. that describes a group of endolysin-based enzymes designed to penetrate the outer-membrane of Gram-negative species, such as Pseudomonas aeruginosa, E. coli, and Salmonella enterica.Citation51,52 For example, Artilysin Art-175 covalently combines the P. aeruginosa phage KZ144 endolysin with the sheep myeloid antimicrobial peptide of 29 amino acids (SMAP-29).Citation51 SMAP-29 is an α-helical cathelicidin that acts rapidly to permeabilize membranes of susceptible organisms, making it a potent antimicrobial peptide (AMP) with a broad spectrum of activity against bacteria, fungi, and viruses. SMAP-29-assisted uptake of Art-175 induced cell lysis of P. aeruginosa, in contrast to SMAP-29, which did not cause cell lysis.Citation51 Unfortunately, fusion of SMAP-29 to an endolysin abolished the AMP bactericidal effect. The loss of membrane permeabilization activity might be beneficial since SMAP-29 is highly cytotoxic to human red blood cells,Citation53 and Art-175 failed to show cytotoxicity in a mouse fibroblast cell line.Citation51 Briers et al. also constructed other Artilysins by fusing Gram-negative phage endolysins with different LPS-destabilizing peptides [polycationic peptide (PCNP), hydrophobic pentapeptide (HPP), Parasin (Pa1), and lycotoxin] as single- or mixed double-peptide fusion constructs. The bacteriolytic effect on Gram-negative cells was most prominent for the PCNP fused enzymes at the N-terminus.Citation52
Certain pathogens such as S. aureus evade both antibiotics and the host immune system by residing intracellularly within their host eukaryotic cells.Citation54 Protein transduction domains (PTD) are cationic peptides that naturally facilitate protein transport across eukaryotic cell membranes.Citation55 Fusion of an endolysin to a PTD is also possible.Citation10,56 In the case with PTD-staphylolytic endolysin hybrids, the PTD assisted uptake can facilitate up to a 95% reduction in intracellular S. aureus burden over non-PTD-enzymes, in three different eukaryotic epithelial cell-lines (unpublished data).
Endolysins as therapeutic agents in animal models
The use of purified endolysins as recombinant proteins to lyse bacteria was first reported in 1959.Citation57 Since then, several endolysins have been characterized, in particular those from Gram-positive-infecting phage. To date, endolysins are not approved for therapeutic use in humans. Clinical trials are under way such as the combined Phase I (safety) and Phase II (efficacy) trial for intra-nasal use of P128 by GangaGen (ClinicalTrials.gov Identifier NCT01746654) and Phase I (safety) trial for intravenous use of PlySs2(CF-301) by ContraFect (ClinicalTrials.gov Identifier NCT02439359) are both for the treatment of S. aureus, but results have not been published. Below, is an overview of the published preclinical trials done on endolysins and endolysin-based enzymes against bacterial pathogens in animal models of human infections and diseases, organized by pathogen and summarized in .
Table 1. Summary of phage-encoded proteins used as antibacterials in animal models of human infection and disease
Streptococcal infections
Streptococci are responsible for an array of human infections, including streptococcal pharyngitis (strep throat), rheumatic fever, toxic shock-like syndrome, cellulitis, mastitis, necrotizing fasciitis, pneumonia, urinary tract infections, and meningitis in newborns.Citation58-60 The most problematic streptococci are the β-hemolytic group A streptococci (GAS) and group B streptococci (GBS), and the α-hemolytic streptococci Streptococcus pneumoniae and Streptococcus viridans.
Streptococcus pyogenes (GAS) is commonly associated with pharyngitis and impetigo,Citation61 and the first pathogen targeted to demonstrate that purified recombinant endolysin could in fact reduce the bacterial load in an animal infection model.Citation61 In this pioneering study, Nelson et al. showed that the oral administration of 24 ng (of a group C streptococcal phage endolysin PlyC, could eliminate the streptococcal burden from heavy colonized murine oral cavities within 2 h.Citation61 In a follow-up study, a single 2 mg (∼80 mg/kg) intraperitoneal injection of PlyC could rescue S. pyogenes bacteremic mice from death if given 3 h after bacterial challenge.Citation62 Similarly, a 2 mg intraperitoneal injection of the Streptococcus suis phage endolysin PlySs2, that was known to lyse several serotypes of S. pyogenes, could also rescue 95% of S. pyogenes bacteremic mice from death. Further study found that PlySs2 could also lyse methicillin-resistant S. aureus (MRSA), and is potent enough to protect mice from bacteremic death caused by mixtures of MRSA and GAS, whereas PlyC could not.Citation62 However, PlyC and PlySs2 are not endolysins encoded by S. pyogenes phages. The only S. pyogenes phage endolysin reported to be tested in an animal model is PlyPy, the gene for which was identified from within a silent prophage.Citation60 PlyPy has little similarity to other known streptococcal endolysins. Nevertheless, an intraperitoneal injection of PlyPy can still protect the majority of S. pyogenes bacteremic mice from death, similar to PlyC and PlySs2, but at a much lower dose of 0.5 mg (∼20 mg/kg).Citation60 There are several other endolysins that have been reported to have bacteriolytic activity against S. pyogenes, such as the Streptococcus agalactiae phage endolysins B30Citation27,63 and LambdaSa2 endolysin,Citation19 the Streptococcus uberis phage endolysin Ply700Citation64 and the S. pneumoniae endolysin Cpl-7.Citation65 However, these have yet to be tested in an animal model of S. pyogenes infection.
S. agalactiae (GBS) is a common colonizer of the upper respiratory and vaginal tracks of humans. In healthy adults, infections are extremely uncommon, but the elderly and patients with chronic medical conditions are susceptible to GBS bacteremia and pneumonia. GBS is particularly problematic for causing sepsis and acute inflammation of the brain and spinal cord (meningitis) among newborns.Citation58,66 Mouse S. agalactiae bacteremia is typically fatal within 3 d. Treatment with the S. dysgalactiae prophage endolysin PlySK1249, which also lyses S. agalactiae, could prolong the survival of infected mice after a single intraperitoneal treatment of 22.5 mg/kg. PlySK1249 treatment became curative when increased to 3 intraperitoneal injection treatments of 45 mg/kg over the course of the first day after GBS challenge.Citation67 GBS is also a common colonizer of the female reproductive tract, which is the primary source of neonatal infections. Washing a mouse vagina with the S. agalactiae phage endolysin PlyGBS could reduce the bacterial burden of a streptomycin-resistant S. agalactiae strain by 3-logs.Citation58 Notably, PlyGBS had an optimal lytic activity around pH 5 coinciding with the normal pH range of healthy human vaginal tracts. However, Cheng et al. considered PlyGBS to have a relatively low streptolytic activity in vitro compared to other endolysins described in the literature.Citation66 The authors improved PlyGBS lytic activity through DNA mutagenesis to create a “hyperlytic” mutant called PlyGBS90–1. This mutagenesis improved in vitro lytic activity against S. agalactiae by 28-fold. Retesting in the same vaginal decolonization model described above for PlyGBS, with a single dose of PlyGBS90–1 at the same concentration, was 10 times more potent. In addition, neither PlyGBS nor its hyperactive mutant harmed other common beneficial vaginal colonizers, such as Lactobacillus acidophilus.Citation58,66
S. pneumoniae is an α-hemolytic bacterium that colonizes the nasopharynx of most adults and children. It is also a common cause of bacterial pneumonia.Citation68,69 Reducing the burden of S. pneumoniae in the nasopharynx is thought to have an impact on the occurrence rate of pneumonia.Citation68 Topical application of the S. pneumoniae phage endolysins Pal or Cpl-1 has successfully eliminated experimentally inoculated S. pneumoniae from the nares of mice.Citation65,68 For systemic infections, a single 2 mg (∼80 mg/kg) intravenous dose of Cpl-1 given within 10 h after challenge reduced bacterial burden in the bloodstream of mice by 3-logs. Despite eliminating a significant amount of blood borne bacteria, numbers eventually recovered and mice died. Delaying treatment allowed S. pneumoniae time to colonize tissues and organs, and escape the endolysins' reach.Citation68 Switching route of treatment to an intraperitoneal injection and given within 1 h of bacterial challenge, Cpl-1 became 100% protective.Citation70 In addition, this switch reduced the curative dosage of Cpl-1 tenfold. Similar studies have also shown that intravenous infusion is not the optimal delivery method for endolysin treatments. Entenza et al. gave rats a bolus intravenous infusion of 10 mg/kg, followed by a continuous infusion of 5 mg/kg/h of Cpl-1 for 6 h, which was not enough to prevent multidrug-resistant S. pneumoniae from returning to pretreatment numbers in the blood within the 6 h. Only after a bolus treatment of 250 mg/kg followed by continuous infusion of 250 mg/kg/h for the entire 6 h was bacteria resurgence prevented.Citation71 This limited therapeutic effect of endolysin administered intravenously is likely due to the enzymes' short half-life (15–20 min) in the blood.Citation72 This is consistent with other therapeutic proteins administered intravenously.Citation68 Even the more direct intracisternal injection of Cpl-1 into the mouse brain could not dramatically prolong the bioavailability of Cpl-1 for treatment of pneumococcal meningitis, and multiple intracisternal injections proved detrimental to animal health.Citation72
Most pneumococcal endolysins depend on binding to the choline moieties of teichoic acids for catalytic activity.Citation68,73 This restricts their lytic spectrum to pneumococcal or related choline-substituted species.Citation73 The pneumococcal endolysin Cpl-7 has a CBD that is not dependent on choline-binding giving it unrestricted activity against not only pneumococcal species but against non-pneumococcal species, including S. pyogenes.Citation73,74 However, bacteriolytic activity of Cpl-7 is significantly lower than that of Cpl-1 and Pal. By inverting the Cpl-7 CBD charge from negative to positive (at neutral pH) through 15 amino acid substitutions, lytic activity of the inversion variant Cpl-7S improved fourfold. Moreover, a single 25 µg dose of Cpl-7S increased the survival rate of zebrafish (Danio rerio) embryos infected with S. pneumoniae or S. pyogenes. The mortality rate of bacterium-infected embryos was 29% for S. pneumoniae, and 35% for S. pyogenes, whereas survival rates after treatment reached 99% for pneumococcus- and 95.3% for S. pyogenes-infected.Citation73 Although Cpl-1 was not included in this model, its fourfold increase in potency over Cpl-7S in vitro suggests it may provide better protection at a lower dose. However, a chimeric of Cpl-7S EAD and Cpl-1 CBD provided an enzyme (Cpl-711) even more potent than native Cpl-1.Citation74 In a mouse model of pneumococcal bacteremia, a single intraperitoneal injection of the chimeric Cpl-711 enzyme an hour after challenge improved survival by 50% over Cpl-1 with a 0.2 mg (˜8 mg/kg) dose.Citation74 This was noteworthy because Cpl-1 and Cp1–7S have 95% similar N-terminus EAD, but by sharing the same Cpl-1 CBD, Cpl-7S EAD was catalytically more potent.
The aforementioned studies of treating pneumococcal infections in animals with endolysins, all practiced eliminating bacterial burden shortly after challenge before the onset of disease. In contrast, mice suffering severe pneumococcal pneumonia after being transnasally infected with pneumococci (instead of intraperitoneal treatments described above) and similarly treated with a single intraperitoneal injection of Cpl-1, experienced 100% survival if the treatment was administered within the first day after challenge.Citation75 However, postponing treatments to the second day reduced the chance of survival to 42%. Compared to the antibiotic amoxicillin treatment (86% chance of survival), Cpl-1 provided a slightly better survival probability. For reasons not determined, amoxicillin improved the chances of survival by 30% over Cpl-1 when given the second day after challenge.Citation75 Aerosolization has also been a delivery method of endolysins to severely ill mice. Mice suffering from pneumonia at the onset of therapy were rescued with a single treatment of 0.4 mg aerosolized Cpl-1 (in 25 mL saline) by means of a MicroSprayer.Citation69 Ten days after treatment, Cpl-1 had effectively reduced pulmonary bacterial burdens, in contrast to increasing bacterial numbers in the untreated lungs. Like many of the previously mentioned treatment regimes, a single aerosolized treatment of the endolysin was not enough to eradicate all detectable bacterial load from the lung. However, it did allow for reduction in mortality by 80% and improvements in body weight and lung tissue appearance after challenge.Citation69 Thus, complete eradication of the pathogen is not always necessary in order to experience a reduction in symptoms and improve survival. In this case, reducing the bacterial burden was likely enough to allow the mouse's immune system to cope with the remaining bacterial load.
Staphylococcal infections
The genus Staphylococcus includes several species including human commensal strains that inhabit skin and mucous membranes. Staphylococci are typically harmless, but can be opportunists causing a range of infections from relatively minor skin infections to life threatening systemic infections, such as bacteremia, endocarditis, and osteomyelitis.
Staphylococcus aureus is the most notorious of the staphylococcal pathogens, which in the past was relatively easy to treat with antibiotics. Recently, several multi-drug resistant S. aureus strains such as MRSA, vancomycin-resistant S. aureus (VRSA), and vancomycin-intermediate S. aureus (VISA) have become more prominent, making these infections harder to treat. As such, S. aureus has been a highly targeted species for endolysin technology, resulting in a large diversity of characterized enzymes tested in animal models of infection. The staphylococcal phage ΦMR11 endolysin MV-L can rapidly lyse several strains of S. aureus, including several MRSA, VRSA, and VISA variants. MV-L is also harmless to most other commensal species tested, including Staphylococcus epidermidis, a favorable mucosal inhabitant that can competitively exclude S. aureus colonization.Citation76 In humans, the nasal cavity is considered a primary source for S. aureus. Intranasal treatment with MV-L was efficient in eliminating artificially inoculated S. aureus from the nares of mice.Citation76 Similarly, nasally and orally administered CHAP(K), a truncated version of the staphylococcal phage endolysin LysK, could also effectively eliminate S. aureus from the nares of infected mice by 2-logs.Citation41 Intranasal treatment of the engineered chimeric staphylolytic enzyme ClyS, a fusion of the Twort endolysin EAD and phiNM3 CBD, could effectively decolonize MRSA from the nasal passage by 2-logs, 24 h after challenge.Citation77 As a topical ointment, ClyS was more effective than mupirocin, the antibiotic commonly prescribed for nasal S. aureus infection treatment.Citation78
Endolysins can reduce S. aureus burden in the bloodstream and prevent septic death in rodents. Treatment within 30 minutes after a systemic challenge of MRSA with a single 0.05 mg (∼2 mg/kg) intraperitoneal injection of endolysin MV-L was able to improve mouse survival by 100%. However, delay of the same treatment by an hour decreased mouse survival by 40% with further decreases the longer treatment was postponed. Furthermore, MV-L was unable to eradicate all S. aureus from the bloodstream.Citation76 Using a more potent staphylococcal endolysin (LysGH15) at the same concentration of ˜2 mg/kg offered the same level of protection from fatal bacteremia, but treatment could be extended up to an hour after MRSA challenge.Citation79 However, increasing potency was still unable to eliminate all bacterial burden. The added benefit of using LysGH15 over MV-L was its exceptional staphylolytic activity specifically toward MRSA strains. Gu et al. suggested that the increased MRSA specific potency could be a result of greater binding by LysGH15 to cell wall moieties present only on methicillin-resistant strains.Citation79 In an attempt to show this, a LysGH15 CBD fused to GFP (LysGH15B–GFP) was constructed and tested on both methicillin-resistant and methicillin-sensitive S. aureus strains. Unfortunately, this methodology was unable to show different binding affinities between the 2 strain types.Citation80 Analyzing the amino acid sequence of LysGH15, MV-L, and 2 other staphylococcal phage endolysins LysK and phi11, revealed that these enzymes all have a similar structure and encode for an N-terminal CHAP (amidohydrolase/peptidase) domain, a central amidase domain, and a C-terminal SH3b CBD. For the most part, these endolysins also share similar bacteriolytic spectrums, lysing several S. aureus strains.Citation31,81 However, MV-L, LysK and phi11 do not share LysGH15 enhanced MRSA lytic activity.
Recently, Schmelcher et al. compared eight staphylolytic endolysins that all contained a SH3b CBD to the SH3b containing bacteriocin lysostaphin, and antibiotics oxacillin and vancomycin in a bacteremia model.Citation82 The endolysins chosen were representative of multiple PGH subgroups encompassing about 60 SH3b-containing enzymes. For in vivo efficacy comparisons, mice were intraperitoneally infected with MRSA and an immunosuppressant, followed half an hour later by a 0.2 mg (∼8 mg/kg) treatment via the same route. In this model, 70% of the untreated infected mice died within 2 d. However, treatment with endolysins LysK, phi11, 80α, 2638A, or WMY could protect 100% of the animals from death, comparable to lysostaphin and vancomycin treatments. Endolysins Twort and phiSH2 were less protective rescuing only about 60% of the mice, while endolysin P68 offered no protection.Citation82 Despite the sequence diversity between the enzymes (<50 % identical) almost all of the staphylolytic enzymes tested offered some level of protection against an otherwise fatal MRSA infection. The only recombinant enzyme that did not was P68, due to protein solubility issues.
S. aureus is also the leading cause of severe endophthalmitis, a vision-threatening complication of ocular trauma and surgeries. The use of topical antibiotics does little to reduce the incidence of staphylococcal infection.Citation83 A single intravitreal injection of a chimeric staphylolytic endolysin Ply187AN-KSH3b several hours after S. aureus challenge reduced bacterial burden by 2-logs in the eye. This reduction significantly improved the outcome of endophthalmitis, and preserved up to 95% retention of the eye's retinal structural integrity. In addition, treated mice maintained their normal visual function with no significant decline in a- or b-wave amplitude. Ply187AN-KSH3b is an engineered chimeric staphylolytic enzyme, constructed by fusing the CHAP domain of staphylococcal endolysin Ply187 with the SH3b CBD of endolysin LysK. This chimera showed a greater in vitro bacteriolytic activity than either of the parental enzymes Ply187 or LysK, particularly against MRSA strains.Citation83
SAL-1 is an endolysin derived from the Staphylococcus phage SAP-1, which also shares a high degree of amino acid sequence similarity to LysK.Citation84 SAP-1 staphylolytic spectrum is similar to that of LysK, including clinical MRSA strains, but is slightly more potent. Development of SAL-1 as a therapeutic agent showed that a stabilizing serum containing calcium ions and Poloxamer 188 improved overall lytic activity against encapsulated, biofilm forming, and planktonic isolates of S. aureus, in vitro. This pre-formulation (designated SAL200) prolonged survival of MRSA infected mice after intravenous infusions were administered once a day for 3 d after a challenge. Without treatment, the infection was fatal. With treatment, survival correlated with a reduction, but not elimination of bacterial burden in the blood and splenic tissue.Citation85 The general safety of SAL200 was determined after several repeated-intravenous doses in rats and dogs.Citation86 Four weeks of repeated-doses of 100 mg/kg were well tolerated in rats and showed no signs of toxicity or abnormal behavior. In dogs, repeated-dosing over 2 weeks showed no treatment-related changes in body weight, food consumption, ophthalmology, electrocardiography, urinalysis, hematology, serum biochemistry, or organ weight. However, they did display subdued behavior, irregular respiration and experienced vomiting. Upon further investigation, repeated exposure of more than a week elicited an immune response in both the rats and dogs evident by detectable anti-SAL-1 antibodies. A decrease in blood C3 complement was also detected in the exposed dogs.Citation86 This is an important finding because complement proteins play an important role in the innate immune system, by aiding antibodies and phagocytic cells in killing foreign invaders. C3 complement levels are typically low during microbial infections, and higher during acute or chronic inflammation.Citation87 Since SAL-200 caused a decrease in C3 complement after several repeated exposures, the immune response was analogous to a microbial infection rather than inflammation to an irritant. It is hard to determine if such a response was due to the nearly unavoidable residual lipopolysaccharide endotoxin in the recombinant protein preparation, or to the enzyme. Nonetheless, the symptoms were only apparent transiently after SAL-200 injection and were considered mild.Citation86 Furthermore, C3 complement levels returned to normal after a recovery period, as would be expected when the underlying condition is resolved.Citation87
Using endolysins in combination with antibiotics can revive the efficacy of overused or less efficient antibiotics. For example, a single intraperitoneal treatment of 0.96 mg (∼38.4 mg/kg) of the fusion enzyme ClyS improved survival rates to 88% from MRSA sepsis.Citation77 ClyS is also synergistic with the antibiotics vancomycin and oxacillin. In combination, ClyS dosage could be reduced to 0.17 mg (∼6.8 mg/kg) after an intramuscular injection of 0.05 mg (∼2 mg/kg) oxacillin and still achieve the same protection against bacteremic death. Oxacillin inhibits enzymes responsible for cell wall assembly, resulting in the increased expression of autolysins (bacterial encoded PGH used to repair and remodel peptidoglycan). The combined activity of ClyS with overexpressed autolysins further accelerated the peptidoglycan destruction.Citation77
Bacillus infections
The large majority of Bacillus species are harmless to humans or animals. The species implicated in infections include the anthrax bacillus, Bacillus cereus, as well as Bacillus subtilis, Bacillus sphaericus, Bacillus alvei, Bacillus laterosporus, Bacillus megaterium, Bacillus licheniformis, and Bacillus pumilus. The clinical spectrum of infections include food poisoning, systemic infections (e.g., meningitis, endocarditis, osteomyelitis, and bacteremia), periodontal disease, and localized infections related to trauma.Citation88 Many members of this genus are spore formers, providing a means of survival during harsh conditions. Inhalation of these spores that germinate and vegetatively propagate causes an overwhelming flood of bacteria and toxins in the blood of humans. If left untreated, bacilli infections can be fatal. The γ phage endolysin PlyG can lyse both vegetative cells and germinating spores of B. anthracis, as well as other closely related bacilli. An intraperitoneal injection of PlyG was able to rescue 65% of infected mice from fatal bacilli septicemia. In addition, those mice that did not recover experienced prolonged survival.Citation88 The PGH PlyPH was isolated from a putative prophage within a bacilli genome. Although not confirmed as an endolysin, PlyPH shares a high percentage of identity to endolysins from other Bacillus phages, but not PlyG. PlyPH is unique from PlyG in its extreme resilient lytic activity between pH 4 and 8, as the majority of endolysins are most active between pH 5 to 7 with activity diminishing rapidly beyond those values. PlyPH was also highly selective for lysing B. anthracis strains, but not other bacilli such as B. cereus, B. thuringiensis, B.subtilis and B. pumilus. In a mouse model of peritonitis, almost immediately after an attenuated B. anthracis challenge, a 1.2 mg (∼48 mg/kg) intraperitoneal injection of PlyPH improved chances of survival by 40%, while all non-treated mice died.Citation89 In humans, the window of opportunity for spore-exposed individual treatment with antibiotics is 48 h. Although not tested in this study, other endolysin treatments that involved intraperitoneal injectionCitation76 suggest the window of treatment opportunity with endolysins will not be as long.
Gram-negative bacteria infections
Killing Gram-negative bacteria with exogenously applied PGH has proven considerably more difficult due to their outer membrane being highly impermeable to macromolecules, such as most endolysins.Citation90 Thus, endolysin efficacy trials in animals against Gram-negative pathogens are limited, which is disconcerting due to the fact multidrug-resistant Gram-negative pathogens are also on the rise.Citation52 Strategies to enhance outer membrane permeability using chelating agents (i.e. EDTA), or high hydrostatic pressure in combination with an endolysin have been studied.Citation21,22,91,92 Although these measures have shown that chemical and/or physical disruption of the outer membrane allows the endolysin to gain access to the protected peptidoglycan, they may not lend themselves well to human therapies. These methods have not always been successful, with some Gram-negative strains remaining insensitive to lysis by endolysins after membrane disruption.Citation92
A few phage endolysins have demonstrated bacteriolytic activity toward Gram-negative cells without the need for physical or chemical outer membrane disruption, or by enzyme modification.Citation20,21,93 For example, the SPN9CC endolysin naturally lyse only Gram-negative bacteria in the absence of an outer membrane permeabilizer.Citation93 Likewise, the Gram-positive Bacillus amyloliquefaciens phage endolysin Lys1521 can naturally lyse several P. aeruginosa and E. coli strains.Citation90,94,95 These types of endolysins tend to contain an amphipathic (both hydrophobic and hydrophilic regions) or highly cationic region, which interacts with the negatively charged surface lipopolysaccharides (LPS) to transverse the outer membrane.Citation20,22,90,94,95 The Gram-negative Acinetobacter baumannii phage endolysin LysAB2, with a predicted C-terminus amphipathic region, has broad lytic activity for both Gram-positive and Gram-negative hosts. Truncation of the amphipathic region abolishes this activity, suggesting the region is necessary for LysAB2 to be bacteriolytic of Gram-negative species.Citation20 However, harboring an amphipathic or highly positively charged amino acid region is not always necessary to destabilize the outer membrane. The endolysin OBPgp279, from Pseudomonas fluorescens phage OBP, can naturally lyse P. aeruginosa, but is devoid of any obvious amphipathic or highly positively charged regions.Citation21 Currently, it is not known how OBPgp279 transverses the outer membrane.Citation21 These aforementioned Gram-negative phage endolysins have yet to be tested therapeutically in animal models.
A. baumannii is also an opportunistic, nosocomial pathogen capable of causing severe life-threatening infections, ranging from mild skin and urinary tract infections to more severe conditions including pneumonia, meningitis, and sepsis. A. baumannii also has a high rate of resistance to antibiotics commonly used to treat Gram-negative infections, with more than 80% of Acinetobacter species considered multidrug resistant. Lood et al. screened induced prophages to obtain a diversity of 21 Gram-negative background endolysins.Citation23 The most potent in vitro, PlyF307 was further tested to protect mice from A. baumannii bacteremia. Due to the rapid onset and progression of infection, most untreated animals died within the first day. Intraperitoneal treatment with 1 mg (∼40 mg/kg) of PlyF307 within hours after challenge was able to rescue 50% of the infected mice.Citation23 Compared to rescuing mice from Gram-positive infections with endolysin as described above, which offer as much as 100% protection from death, 50% rescue from a Gram-negative infection is quite low. This suggests the outer membrane is still a formidable barrier for endolysins applied exogenously. Nonetheless, this study showed that native Gram-negative phage endolysins do offer some antibacterial properties in a mammalian model of human Gram-negative infection, without the need for additional membrane permeabilizers.
P. aeruginosa is an opportunistic, nosocomial pathogen causing urinary tract infections, respiratory infections, dermatitis, bone and joint infections, gastrointestinal infections, and bacteremia. It is particularly serious in individuals with cystic fibrosis, cancer, and severe burns.Citation96 Protein engineering has allowed endolysin-based enzymes to translocate across the outer membrane barrier of Gram-negative bacteria and control P. aeruginosa infection in animal.Citation50-52 (For details see Engineering novel endolysin constructs) Briers et al. conducted a Caenorhabditis elegans nematode gut P. aeruginosa infection model to evaluate the efficacy of a polycationic nonapeptide (PCNP)-fused endolysin called Artilysin LoGT-008.Citation52 Grazing on P. aeruginosa lawns for one day resulted in a 90% C. elegans death rate within 5 d. When infected nematodes were transferred to a buffer containing 50 µl of the (PCNP)-fused endolysin and 50 µl of EDTA, survival rate improved 63% compared to 45% from a ciprofloxacin treatment.Citation52 To get this protection it was still necessary to destabilize the outer membrane with a detergent.
Resistance to endolysins
The major opinion on the development of resistance to an endolysins catalytic activity is improbable.Citation65,77,78,88,97 There are no described resistance mechanisms or reports of bacteria losing sensitivity to ‘lysis from without’ by endolysins. Researchers have failed to identify resistant mutants after repeated exposure to sub-lethal concentrations of endolysin in vitro.Citation65,77,78,83 Even mutagenesis of endolysin-sensitive strains could not promote resistant derivatives.Citation88 Yet both approaches easily promote bacterial resistance to antibiotics.Citation65,77,78,88 This is not surprising since phage have likely evolved their viral proteins that are essential for survival over millions of years. For endolysins, this requires targeting sites within the peptidoglycan matrix that are essential for bacterial viability that cannot be modified without some consequence. For example, certain endolysins have been shown to target ligands in S. pneumoniae (specifically, choline), S. pyogenes (polyrhamnose) and B. anthracis (polysaccharide) that are all required for their viability.Citation88,98
The presumption that bacteria cannot develop resistance to externally applied endolysins may be shortsighted. Similar to certain endolysins, lysostaphin is a PGH based bacteriocin that cleaves bonds within the peptidoglycan matrix of staphylococcal species and is staphylolytic when applied exogenously to cells. However, bacterial resistance to lysostaphin is well documented.Citation99 Resistance generally results from mutations that cause the formation of monoglycine cross-bridges, thus eliminating the target site for this bacteriocins catalytic activity.Citation99 Thus, resistance to ‘lysis from without’ by a PGH applied externally is possible. From a therapeutic standpoint, lysostaphin resistance may not be a drawback since cross-bridge mutations in MRSA stains promotes a loss of the methicillin resistance phenotype.Citation99 Nonetheless, fitness costs to the bacterium do not prevent such ‘life altering’ mutations from occurring, as compensatory mutations can correct for deficits during subsequent evolutions.Citation100
Pesticin is another PGH based bacteriocin secreted by Y. pestis to lyse closely related Gram-negative competitors. Pesticin-producing cells are protected from suicide after re-uptake of the PGH by the expression of small immunity proteins that bind and inactivate the enzyme.Citation49 Bacteria are extremely resilient, and it is conceivable that these small immunity proteins already expressed by some bacterial species could evolve to inhibit endolysin activity as well. Although this has not been reported. Furthermore, the extracellular site of action for PGH makes it improbable that resistance will come from one the majority of known antimicrobial resistance mechanisms that tend to act intracellularly on invading small-molecules (i.e., reduced membrane permeability, active efflux pumps, inactivation of antimicrobials by cytoplasmic enzymes, modified site of action).Citation25 Furthermore, if endolysin resistance does occur, the large number and variety of phages and the ease of engineering endolysins provides us with a constant source of new PGHs.
Virion-Associated Peptidoglycan Hydrolases
Certain phages encode a second type of PGH called virion-associated peptidoglycan hydrolases (VAPGH; also known as virion-associated or tail-associated muralytic enzymes) (). VAPGH are a structural component of some phage particles (tails), which mediates the local hydrolysis of cell wall peptidoglycan shortly after particle adsorption. In contrast to endolysins that cause cell lysis at the end of the infection cycle, localized degradation of peptidoglycan allows the phage tail tube access to the cytoplasm for transfer of its viral DNA.Citation13 VAPGH are present in both phages infecting Gram-negative and Gram-positive bacteria, and facilitate transfer of their genomic material into a host. However, not all phage require VAPGH to accomplish genomic transfer, as many do not encode any known VAPGH genes.
VAPGH biochemical characterizations are limited, but they do share a high degree of similarity to endolysins () and likely share similar modes of action for hydrolyzing specific bonds in peptidoglycan structure (). The temperate S. aureus phage DW2 has both a putative endolysin (LysDW2) and a VAPGH (THDW2), which are both staphylolytic when individually applied exogenously to cells.Citation101 Both enzymes share an N-terminus CHAP domain, but differ in a second EAD, with LysDW2 having an amidase and THDW2 a muramidase. The major difference between the endolysin and VAPGH is that THDW2 lacks a CBD.Citation101 Likewise, the S. aureus phage ΦMR11 also encodes a putative VAPGH with a CHAP domain followed by a muramidase domain, which are independently staphylolytic, and lacks a CBD.Citation76 The S. aureus phage vB_SauS-phiIPLA88 VAPGH HydH5 is also a dual EAD enzyme,Citation102 In contrast, the S. aureus phage P68 has a VAPGH (protein P17) that has muralytic activity and a C-terminus region that acts as a substrate recognition and/or binding domain.Citation103 Since the removal of proteins and lipids from the staphylococcal cell wall does not affect binding, it was speculated that protein P17 binds to a carbohydrate component covalently attached to the peptidoglycan. Protein 17 displayed lytic activity against several clinical S. aureus strains including MRSA, some of which were resistant to P68 virion infection.Citation103 Even with the limited number of VAPGHs analyzed to date, there is already indication of a large diversity within the VAPGH family of enzymes.
Figure 3. Schematic of bacteriophage-encoded peptidoglycan hydrolase structures. Typically, an enzymatically active domain (EAD) that retains the catalytic activity is connected to cell wall binding domain (CBD) by a short linker region (blue bar). The catalytic activity, number, proximity, and orientation of domains are not conserved. (Top) streptococcal endolysin; (Middle) staphylococcal endolysin; (Bottom) staphylococcal virion-associated peptidoglycan hydrolase. Not drawn to scale.
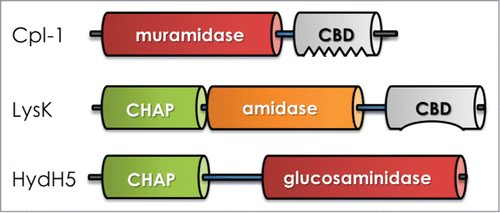
VAPGH have a modular structure, akin to endolysins, allowing protein engineering to alter their bacteriolytic properties. The lytic activity of the previously described HydH5, native full-length and 2 truncated derivatives containing only the CHAP domain, was modified via fusion to the SH3b CBD from a bacteriocin lysostaphin [known to be staphylococcal specific]. Each SH3b CBD fusion enzyme achieved higher staphylolytic activity than the native endolysin. Furthermore, HydH5 and the derivative SH3b CBD fusion enzyme were synergistic with the endolysin LysH5 encoded by the same phage presumably due to each lytic enzyme having distinct PG cut sites. These enzyme pairs together were more efficient at degrading staphylococcal peptidoglycan than individually.Citation104 Similarly, this domain swapping approach has also proven to be useful for the improvement of lytic activity of the putative VAPGH within phage K open reading frame 56.Citation105 N-terminus deletions of orf56 defined the coding region responsible for a small PGH (Lys16) that retained staphylolytic activity. Fusion of Lys16 to the lysostaphin (PGH bacteriocin) SH3b CBD generated a chimeric enzyme (P128), which displayed higher anti-staphylococcal activity than Lys16 on clinical isolates of S. aureus including MRSA.Citation105
To date, the chimeric enzyme P128 is the only VAPGH therapeutically tested in an animal model of bacterial infection. In a rat nasal decolonization model, a MRSA strain (USA300) was instilled into the nares of rats followed shortly thereafter by 2 intranasal treatments of P128 formulated as a hydrogel (50 mg/dose containing 100 μg P128), or a 2% mupirocin ointment (30 mg/dose). P128 hydrogel treatment was able to reduce MRSA colonization of rat nares by twofold, whereas the mupirocin ointment was ineffective.Citation105 The P128 hydrogel formulation was also reported to be bacteriolytic against several staphylococcal isolates recovered from the nares of healthy humans without any processing or culturing steps, suggesting in situ efficacy.Citation106 Given the PGH similarities between VAPGH and endolysins, it is likely that newly characterized VAPGH will have therapeutic capabilities against Gram-positive pathogens similar to endolysins in further animal models of bacterial infection and disease.
Polysaccharide Depolymerases – Carbohydrate-Degrading Enzymes
Many phages encode polysaccharide hydrolases (depolymerases) that degrade macromolecule carbohydrates within extracellular polysaccharides and lipopolysaccharides (LPS) surrounding bacterial cells ().Citation12,107-109 Bacterial polysaccharides encompass an overwhelming diversity, ranging in composition from single sugar residues linked together, to complex high molecular weight branching structures of 3 or more sugar residues. Polysaccharides are multifunctional, e.g. adding structural integrity to the cell, aiding in cell adherence to biotic and abiotic surfaces, and improving migration of cells in liquid and communal life in biofilms.Citation110 For many encapsulated bacteria, polysaccharides are major determinants for survival, promoting host virulence, protection from desiccation, and protection from phagocytosis and antimicrobials.Citation111-113 For phage, polysaccharides can pose as a physical barrier to the cell surface receptors needed for adsorption. Polysaccharides are classified based on their morphological localization as being either intracellular polysaccharides, structural polysaccharides (i.e. LPS), or extracellular polysaccharides (EPS). EPS are the most abundant and located outermost of the cell envelope. Depending on the bacterial species, EPS are either covalently bound to the cell surface as capsular polysaccharides, or excreted as a loose slime.
Depolymerases have only been reported in tailed dsDNA phages (order Caudovirales), which are either associated with their tail structure as tailspike proteins (TSP), or as diffusible enzymes.Citation114,115 Examples are endorhamnosidases that hydrolyze outer membrane LPS of Gram-negative species,Citation116 endolsialidases that degrade capsular polysaccharides of E. coli,Citation113 alginate lysases that degrade the capsules of P. aeruginosa,Citation117 and hyaluronidases that degrade capsules of streptococcal species.Citation113 To the best of our knowledge, polysaccharide depolymerases have not been shown to be bacteriolytic or bacteriostatic. The therapeutic attributes of depolymerases typically arise from ‘stripping away’ the extracellular polysaccharides (used by a number of bacterial pathogens) to promote host colonization (i.e., biofilm formation) and virulence and serve as protection from host immune defenses, antimicrobial agents, and phages.Citation114 Dubos and Avery demonstrated that a phage-encoded depolymerase that degraded purified capsular polysaccharides from Type III Pneumococcus, could protect mice against infection from the same bacterium.Citation118 Other pre-antibiotic era demonstrations included controlling potentially lethal pneumococcal infections in mice, rabbit,Citation119,120 and monkey,Citation121 with a partially purified preparation of depolymerases. More recently, Mushtaq et al. have shown that an intraperitoneal administration of doses as low as 0.25 mg (∼0.83 mg/kg) of the endosialidase E (endoE) given to rats early in the infection process of neurotropic strains of E. coli, improved survival of an otherwise lethal systemic infection.Citation113 The polysaccharide capsule of E. coli K1 has been shown to protect cells from humoral and cellular components of the host's immune system. Removal of this capsule with endoE during the early stages of systemic infection by E. coli K1 enabled the host's defenses to eliminate the pathogen by macrophage ingestion.Citation113,122 Surprisingly, endoE had little effect on the viability and growth rate of E. coli K1 in vitro. In a subsequent study, prompt administration of endoE could reverse the bacteremic state and prevent death of almost all rat pups tested. In untreated animals, E. coli had colonized the gastrointestinal tract, entered the bloodstream, and eventually bypassed the blood–brain barrier.Citation122 Therefore, systemic infections of encapsulated pathogens may be treated by capsule depolymerization via phage depolymerases.
Oral administration of Salmonella specific tail spike protein, P22sTsp, significantly reduced Salmonella infection in chickens. The mode of action of P22sTsp in reducing Salmonella colonization is uncertain, but may be attributed to its endorhamnosidase activity and/or binding activity of O-antigen in LPS, thus compromising cell surface structures.Citation116 The theory that removing the bacterial EPS can reduce the pathogen's ability to colonize and cause disease was tested by removing the EPS layer of the plant pathogen Erwinia amylovora.Citation123,124 Erwinia spp. phage depolymerase DpoEa1h, efficiently degrades the major EPS component amylovoan of the E. amylovora capsule. Amylovoran has been shown to be essential for infection in host plants, as amylovoran deficient mutants do not cause disease.Citation125 Expression of DpoEa1h in transgenic apple and pear plants significantly reduced fire blight (E. amylovora) susceptibility,Citation124 likely due to removal of the main virulence factor amylovoran and exposing the cells to host plant defenses.Citation123 Polysaccharide capsule is also an important virulence factor for Klebsiella pneumoniae, contributing to its resistance to antibiotics. A depolymerase encoded by Aeromonas punctata was capable of degrading capsular EPS of K. pneumoniae, which reinstated sensitivity to gentamicin.Citation126 Purified depolymerase was given as a 0.05 mg (∼2 mg/kg) intraperitoneal injection alone and in combination with 1.5 mg/kg gentamicin after the onset of acute lung infection and septicemia. The combination treatment reduced bacteria load in the lungs, liver, kidney, spleen, and blood of mice, more effectively than each treatment type alone. In addition, there was a reduction in neutrophil infiltration and inflammatory cytokines.Citation126 The latter is an example where phage enzymes can revive the functionality of over-used antibiotics.
Depolymerases may have the greatest potential as therapeutic agents to prevent or eliminate biofilms. Biofilms are found in the natural, industrial, and medical environments and consist of microorganisms embedded in a glycocalyx that is composed in part of microbial produced EPS that can serve as a structural backbone of the biofilm.Citation127,128 This superstructure protects internal bacteria from antimicrobials and host natural defense mechanisms better than their free-ranging counterparts.Citation122,128 Removal of the EPS matrix within the superstructure could be an effective means of preventing or dispersing biofilms, making the subjacent bacteria more susceptible to treatment by antimicrobials. Hughes et al. has shown that depolymerases can penetrate EPS layers to break up Enterobacter agglomerans and Serratia marcescens biofilms.Citation127 Although extensive analysis of depolymerase utility as anti-biofilm agents has yet to be demonstrated.
Holins – Cell Membrane Disturbing Proteins
During phage lysis of bacteria, several proteins are temporally regulated in parallel with the pathways of viral assembly ().Citation15,16 As mentioned, endolysins are responsible for peptidoglycan degradation at the final stages of cell lysis. Endolysins are aided by two fundamentally different pathways involving holins, namely holin-endolysin and pinholin-SAR (signal anchor-release) endolysin systems. Holins in the holin-endolysin system are phage-encoded hydrophobic membrane proteins involved in the massive permeabilization of the cytoplasmic membrane, allowing endolysins to translocate into the periplasm and attack the peptidoglycan. For phage λ, interruptions in the cytoplasmic membrane by holins occur from the formation of ‘holes’ ranging in size up to > 1μm.Citation16 The endolysins in the pinhole-SAR endolysin system are exported by the host sec system into the periplasm, and accumulate as inactive proteins tethered to the membrane by their N-terminal SAR domain. Deenergization of the cytoplasmic membrane by imbedded pinholes activates the SAR endolysins.Citation16,129 Thus, pinholins are timers for endolysin activation instead of promoting their export. Since the SAR endolysins are uniformly distributed in the periplasm before pinholin triggering, a more uniform degradation of peptidoglycan occurs causing a gradual shortening of the cells before lysis, unlike cell rupture in the holin-endolysin system.Citation16
Holins alone are unable to cause cell lysis, since destruction of the peptidoglycan is necessary to destabilize the cell wall. For example, E. coli lysis does not occur after infection with λ phage lacking an endolysin gene. Infected cells do lose their ability to support respiration and membrane potential, causing them to become leaky to normally impermeant small molecules.Citation130 Rajesh et al. were the first to demonstrate that a holin-like protein Tmp1 could be a bacteriostatic agent.Citation131 The Tmp1 gene was isolated from a goatskin surface metagenome analysis and could complement a holin-defective lambda phage and produce visible plaques on E. coli lawns.Citation131 Overexpression of Tmp1 strongly inhibited the growth of E. coli during induction, and lysates inhibited the growth of several Gram-positive food contaminants such as Kurthia gibsonii, Staphylococcus epidermidis, and Listeria innocula. The membrane-damaging property of Tmp1 is associated with its N-terminal hydrophobic transmembrane domain at the N-terminusCitation131 typical of holins.Citation132 An ORF (STY1365) in Salmonella enterica also has a putative phage holin gene that when overexpressed caused growth impairment, increased cell permeability to crystal violet, and altered the inner membrane protein profile of S. typhi cells.Citation132 These examples support the notion that holin mediated disruption of the cell membranes can provide an antibacterial effect to impede cell growth.
The lysis cassette in the S. suis phage SMP encodes the holin HolSMP and endolysin LySMP. When applied exogenously, LySMP can lyse several strains of S. suis and S. aureus and exhibit a broader lytic spectrum of activity than whole phage against bacteria investigated. LySMP can also efficiently disrupt biofilms formed by S. suis.Citation133 When applied exogenously, HolSMP alone also appears to cause weak lysis of S. aureus and B. subtilis cells. Surprisingly, holin promoted lysis occurred in strains that were insensitive to endolysin. In combination with endolysins, the holin-endolysin mixture was able to extend the spectrum of the endolysin LySMP alone, to include several more strains of multidrug-resistant S. suis and S. aureus.Citation134 This suggests that the combined use of holins and endolysins might be a potential strategy to improve lytic potency. For review on holins used in other biotechnological and biomedical applications, see ref.Citation135
Concluding Remarks
In an era of increasing emergence of multi-drug resistant pathogens and a paucity of new antibiotics, there is a growing need to rejuvenate old, and discover new antimicrobials to treat bacterial infections. In this context, the preclinical evidence is compelling for the utility of endolysins and VAPGH against an array of predominately Gram-positive bacterial pathogens, with endolysins that can target Gram-negative pathogens also on the rise. Further screening for new endolysins or engineering of endolysin-based enzymes with outer membrane transporters is ongoing and expected to expand the utility of endolysins as therapeutics for both human and animal health. Nonetheless, the recent initiation of clinical trials for two stapholytic endolysins may provide the necessary efficacy and safety validity for this class of antimicrobial agents to control multi-drug resistant bacteria in patients. As for depolymerases and holins, it is too early to know if these will have commercial antimicrobial potential, but there is evidence that holins when used in combination with endolysins can improve overall bacteriolytic potency.
Disclosure of Potential Conflicts of Interest
No potential conflicts of interest were disclosed.
References
- Michael CA, Dominey-Howes D, Labbate M. The antimicrobial resistance crisis: causes, consequences, and management. Front Public Health 2014; 2:145; PMID:25279369; http://dx.doi.org/10.3389/fpubh.2014.00145
- Cassir N, Rolain JM, Brouqui P. A new strategy to fight antimicrobial resistance: the revival of old antibiotics. Front Microbiol 2014; 5:551; PMID:25368610; http://dx.doi.org/10.3389/fmicb.2014.00551
- Kutter E, De Vos D, Gvasalia G, Alavidze Z, Gogokhia L, Kuhl S, Abedon ST. Phage therapy in clinical practice: treatment of human infections. Curr Pharm Biotechnol 2010; 11:69-86; PMID:20214609; http://dx.doi.org/10.2174/138920110790725401
- Chanishvili N. Phage therapy–history from Twort and d'Herelle through Soviet experience to current approaches. Adv Virus Res 2012; 83:3-40; PMID:22748807
- Chan BK, Abedon ST, Loc-Carrillo C. Phage cocktails and the future of phage therapy. Future Microbiol 2013; 8:769-83; PMID:23701332; http://dx.doi.org/10.2217/fmb.13.47
- Bragg R, van der Westhuizen W, Lee JY, Coetsee E, Boucher C. Bacteriophages as potential treatment option for antibiotic resistant bacteria. Adv Exp Med Biol 2014; 807:97-110; PMID:24619620
- Hyman P, Abedon ST. Bacteriophage host range and bacterial resistance. Adv Appl Microbiol 2010; 70:217-48; PMID:20359459
- Bikard D, Euler CW, Jiang W, Nussenzweig PM, Goldberg GW, Duportet X, Fischetti VA, Marraffini LA. Exploiting CRISPR-Cas nucleases to produce sequence-specific antimicrobials. Nat Biotechnol 2014; 32:1146-50; PMID:25282355; http://dx.doi.org/10.1038/nbt.3043
- Brown-Jaque M, Calero-Caceres W, Muniesa M. Transfer of antibiotic-resistance genes via phage-related mobile elements. Plasmid 2015; 79:1-7; PMID:25597519; http://dx.doi.org/10.1016/j.plasmid.2015.01.001
- Schmelcher M, Donovan DM, Loessner MJ. Bacteriophage endolysins as novel antimicrobials. Future Microbiol 2012; 7:1147-71; PMID:23030422; http://dx.doi.org/10.2217/fmb.12.97
- Oliveira H, Melo LD, Santos SB, Nobrega FL, Ferreira EC, Cerca N, Azeredo J, Kluskens LD. Molecular aspects and comparative genomics of bacteriophage endolysins. J Virol 2013; 87:4558-70; PMID:23408602; http://dx.doi.org/10.1128/JVI.03277-12
- Roach DR, Sjaarda DR, Castle AJ, Svircev AM. Host exopolysaccharide quantity and composition impact Erwinia amylovora bacteriophage pathogenesis. Appl Environ Microbiol 2013; 79:3249-56; PMID:23503310; http://dx.doi.org/10.1128/AEM.00067-13
- Rodriguez-Rubio L, Martinez B, Donovan DM, Rodriguez A, Garcia P. Bacteriophage virion-associated peptidoglycan hydrolases: potential new enzybiotics. Crit Rev Microbiol 2013; 39:427-34; PMID:22991936; http://dx.doi.org/10.3109/1040841X.2012.723675
- Yan J, Mao J, Xie J. Bacteriophage polysaccharide depolymerases and biomedical applications. BioDrugs 2014; 28:265-74; PMID:24352884; http://dx.doi.org/10.1007/s40259-013-0081-y
- Young R. Phage lysis: do we have the hole story yet? Curr Opin Microbiol 2013; 16:790-7; PMID:24113139; http://dx.doi.org/10.1016/j.mib.2013.08.008
- Young R. Phage lysis: three steps, three choices, one outcome. J Microbiol 2014; 52:243-58; PMID:24585055; http://dx.doi.org/10.1007/s12275-014-4087-z
- Rajaure M, Berry J, Kongari R, Cahill J, Young R. Membrane fusion during phage lysis. Proc Natl Acad Sci U S A 2015; 112:5497-502; PMID:25870259; http://dx.doi.org/10.1073/pnas.1420588112
- Schleifer KH, Kandler O. Peptidoglycan types of bacterial cell walls and their taxonomic implications. Bacteriol Rev 1972; 36:407-77; PMID:4568761
- Pritchard DG, Dong S, Kirk MC, Cartee RT, Baker JR. LambdaSa1 and LambdaSa2 prophage lysins of Streptococcus agalactiae. Appl Environ Microbiol 2007; 73:7150-4; PMID:17905888; http://dx.doi.org/10.1128/AEM.01783-07
- Lai MJ, Lin NT, Hu A, Soo PC, Chen LK, Chen LH, Chang KC. Antibacterial activity of Acinetobacter baumannii phage varphiAB2 endolysin (LysAB2) against both Gram-positive and Gram-negative bacteria. Appl Microbiol Biotechnol 2011; 90:529-39; PMID:21264466; http://dx.doi.org/10.1007/s00253-011-3104-y
- Walmagh M, Briers Y, dos Santos SB, Azeredo J, Lavigne R. Characterization of modular bacteriophage endolysins from Myoviridae phages OBP, 201phi2-1 and PVP-SE1. PLoS One 2012; 7:e36991; PMID:22615864; http://dx.doi.org/10.1371/journal.pone.0036991
- Walmagh M, Boczkowska B, Grymonprez B, Briers Y, Drulis-Kawa Z, Lavigne R. Characterization of five novel endolysins from Gram-negative infecting bacteriophages. Appl Microbiol Biotechnol 2013; 97:4369-75; PMID:22832988; http://dx.doi.org/10.1007/s00253-012-4294-7
- Lood R, Winer BY, Pelzek AJ, Diez-Martinez R, Thandar M, Euler CW, Schuch R, Fischetti VA. Novel phage lysin capable of killing the multidrug-resistant Gram-negative bacterium Acinetobacter baumannii in a mouse bacteremia model. Antimicrob Agents Chemother 2015; 59:1983-91; PMID:25605353; http://dx.doi.org/10.1128/AAC.04641-14
- Donovan DM, Foster-Frey J, Dong S, Rousseau GM, Moineau S, Pritchard DG. The cell lysis activity of the Streptococcus agalactiae bacteriophage B30 endolysin relies on the cysteine, histidine-dependent amidohydrolase/peptidase domain. Appl Environ Microbiol 2006; 72:5108-12; PMID:16820517; http://dx.doi.org/10.1128/AEM.03065-05
- Becker SC, Foster-Frey J, Stodola AJ, Anacker D, Donovan DM. Differentially conserved staphylococcal SH3b_5 cell wall binding domains confer increased staphylolytic and streptolytic activity to a streptococcal prophage endolysin domain. Gene 2009; 443:32-41; PMID:19422893; http://dx.doi.org/10.1016/j.gene.2009.04.023
- Navarre WW, Ton-That H, Faull KF, Schneewind O. Multiple enzymatic activities of the murein hydrolase from staphylococcal phage phi11. Identification of a D-alanyl-glycine endopeptidase activity. J Biol Chem 1999; 274:15847-56; PMID:10336488; http://dx.doi.org/10.1074/jbc.274.22.15847
- Pritchard DG, Dong S, Baker JR, Engler JA. The bifunctional peptidoglycan lysin of Streptococcus agalactiae bacteriophage B30. Microbiology 2004; 150:2079-87; PMID:15256551; http://dx.doi.org/10.1099/mic.0.27063-0
- Donovan DM, Lardeo M, Foster-Frey J. Lysis of staphylococcal mastitis pathogens by bacteriophage phi11 endolysin. FEMS Microbiol Lett 2006; 265:133-9; PMID:17054440; http://dx.doi.org/10.1111/j.1574-6968.2006.00483.x
- Sass P, Bierbaum G. Lytic activity of recombinant bacteriophage phi11 and phi12 endolysins on whole cells and biofilms of Staphylococcus aureus. Appl Environ Microbiol 2007; 73:347-52; PMID:17085695; http://dx.doi.org/10.1128/AEM.01616-06
- Becker SC, Dong S, Baker JR, Foster-Frey J, Pritchard DG, Donovan DM. LysK CHAP endopeptidase domain is required for lysis of live staphylococcal cells. FEMS Microbiol Lett 2009; 294:52-60; PMID:19493008; http://dx.doi.org/10.1111/j.1574-6968.2009.01541.x
- Horgan M, O'Flynn G, Garry J, Cooney J, Coffey A, Fitzgerald GF, Ross RP, McAuliffe O. Phage lysin LysK can be truncated to its CHAP domain and retain lytic activity against live antibiotic-resistant staphylococci. Appl Environ Microbiol 2009; 75:872-4; PMID:19047377; http://dx.doi.org/10.1128/AEM.01831-08
- Donovan DM, Foster-Frey J. LambdaSa2 prophage endolysin requires Cpl-7-binding domains and amidase-5 domain for antimicrobial lysis of streptococci. FEMS Microbiol Lett 2008; 287:22-33; PMID:18673393; http://dx.doi.org/10.1111/j.1574-6968.2008.01287.x
- Porter CJ, Schuch R, Pelzek AJ, Buckle AM, McGowan S, Wilce MC, Rossjohn J, Russell R, Nelson D, Fischetti VA, et al. The 1.6 A crystal structure of the catalytic domain of PlyB, a bacteriophage lysin active against Bacillus anthracis. J Mol Biol 2007; 366:540-50; PMID:17182056; http://dx.doi.org/10.1016/j.jmb.2006.11.056
- Kretzer JW, Lehmann R, Schmelcher M, Banz M, Kim KP, Korn C, Loessner MJ. Use of high-affinity cell wall-binding domains of bacteriophage endolysins for immobilization and separation of bacterial cells. Appl Environ Microbiol 2007; 73:1992-2000; PMID:17277212; http://dx.doi.org/10.1128/AEM.02402-06
- Eugster MR, Haug MC, Huwiler SG, Loessner MJ. The cell wall binding domain of Listeria bacteriophage endolysin PlyP35 recognizes terminal GlcNAc residues in cell wall teichoic acid. Mol Microbiol 2011; 81:1419-32; PMID:21790805; http://dx.doi.org/10.1111/j.1365-2958.2011.07774.x
- Loessner MJ, Kramer K, Ebel F, Scherer S. C-terminal domains of Listeria monocytogenes bacteriophage murein hydrolases determine specific recognition and high-affinity binding to bacterial cell wall carbohydrates. Mol Microbiol 2002; 44:335-49; PMID:11972774; http://dx.doi.org/10.1046/j.1365-2958.2002.02889.x
- Eugster MR, Loessner MJ. Wall teichoic acids restrict access of bacteriophage endolysin Ply118, Ply511, and PlyP40 cell wall binding domains to the Listeria monocytogenes peptidoglycan. J Bacteriol 2012; 194:6498-506; PMID:23002226; http://dx.doi.org/10.1128/JB.00808-12
- Nelson D, Schuch R, Chahales P, Zhu S, Fischetti VA. PlyC: a multimeric bacteriophage lysin. Proc Natl Acad Sci U S A 2006; 103:10765-70; PMID:16818874; http://dx.doi.org/10.1073/pnas.0604521103
- Yoong P, Schuch R, Nelson D, Fischetti VA. Identification of a broadly active phage lytic enzyme with lethal activity against antibiotic-resistant Enterococcus faecalis and Enterococcus faecium. J Bacteriol 2004; 186:4808-12; PMID:15231813; http://dx.doi.org/10.1128/JB.186.14.4808-4812.2004
- Yang H, Wang DB, Dong Q, Zhang Z, Cui Z, Deng J, Yu J, Zhang XE, Wei H. Existence of separate domains in lysin PlyG for recognizing Bacillus anthracis spores and vegetative cells. Antimicrob Agents Chemother 2012; 56:5031-9; PMID:22802245; http://dx.doi.org/10.1128/AAC.00891-12
- Fenton M, Casey PG, Hill C, Gahan CG, Ross RP, McAuliffe O, O'Mahony J, Maher F, Coffey A. The truncated phage lysin CHAP(k) eliminates Staphylococcus aureus in the nares of mice. Bioeng Bugs 2010; 1:404-7; PMID:21468207; http://dx.doi.org/10.4161/bbug.1.6.13422
- McGowan S, Buckle AM, Mitchell MS, Hoopes JT, Gallagher DT, Heselpoth RD, Shen Y, Reboul CF, Law RH, Fischetti VA, et al. X-ray crystal structure of the streptococcal specific phage lysin PlyC. Proc Natl Acad Sci U S A 2012; 109:12752-7; PMID:22807482; http://dx.doi.org/10.1073/pnas.1208424109
- Mao J, Schmelcher M, Harty WJ, Foster-Frey J, Donovan DM. Chimeric Ply187 endolysin kills Staphylococcus aureus more effectively than the parental enzyme. FEMS Microbiol Lett 2013; 342:30-6; PMID:23413880; http://dx.doi.org/10.1111/1574-6968.12104
- Yang H, Yu J, Wei H. Engineered bacteriophage lysins as novel anti-infectives. Front Microbiol 2014; 5:542; PMID:25360133
- Schmelcher M, Tchang VS, Loessner MJ. Domain shuffling and module engineering of Listeria phage endolysins for enhanced lytic activity and binding affinity. Microb Biotechnol 2011; 4:651-62; PMID:21535426; http://dx.doi.org/10.1111/j.1751-7915.2011.00263.x
- Low LY, Yang C, Perego M, Osterman A, Liddington RC. Structure and lytic activity of a Bacillus anthracis prophage endolysin. J Biol Chem 2005; 280:35433-9; PMID:16103125; http://dx.doi.org/10.1074/jbc.M502723200
- Loessner MJ, Gaeng S, Scherer S. Evidence for a holin-like protein gene fully embedded out of frame in the endolysin gene of Staphylococcus aureus bacteriophage 187. J Bacteriol 1999; 181:4452-60; PMID:10419939
- Mayer MJ, Garefalaki V, Spoerl R, Narbad A, Meijers R. Structure-based modification of a Clostridium difficile-targeting endolysin affects activity and host range. J Bacteriol 2011; 193:5477-86; PMID:21803993; http://dx.doi.org/10.1128/JB.00439-11
- Patzer SI, Albrecht R, Braun V, Zeth K. Structural and mechanistic studies of pesticin, a bacterial homolog of phage lysozymes. J Biol Chem 2012; 287:23381-96; PMID:22593569; http://dx.doi.org/10.1074/jbc.M112.362913
- Lukacik P, Barnard TJ, Buchanan SK. Using a bacteriocin structure to engineer a phage lysin that targets Yersinia pestis. Biochem Soc Trans 2012; 40:1503-6; PMID:23176506; http://dx.doi.org/10.1042/BST20120209
- Briers Y, Walmagh M, Grymonprez B, Biebl M, Pirnay JP, Defraine V, Michiels J, Cenens W, Aertsen A, Miller S, et al. Art-175 is a highly efficient antibacterial against multidrug-resistant strains and persisters of Pseudomonas aeruginosa. Antimicrob Agents Chemother 2014; 58:3774-84; PMID:24752267; http://dx.doi.org/10.1128/AAC.02668-14
- Briers Y, Walmagh M, Van Puyenbroeck V, Cornelissen A, Cenens W, Aertsen A, Oliveira H, Azeredo J, Verween G, Pirnay JP, et al. Engineered endolysin-based “Artilysins” to combat multidrug-resistant Gram-negative pathogens. MBio 2014; 5:e01379-14; PMID:24987094; http://dx.doi.org/10.1128/mBio.01379-14
- Dawson RM, Liu CQ. Analogues of peptide SMAP-29 with comparable antimicrobial potency and reduced cytotoxicity. Int J Antimicrob Agents 2011; 37:432-7; PMID:21377841; http://dx.doi.org/10.1016/j.ijantimicag.2011.01.007
- Hebert A, Sayasith K, Senechal S, Dubreuil P, Lagace J. Demonstration of intracellular Staphylococcus aureus in bovine mastitis alveolar cells and macrophages isolated from naturally infected cow milk. FEMS Microbiol Lett 2000; 193:57-62; PMID:11094279; http://dx.doi.org/10.1016/S0378-1097(00)00455-9
- Torchilin VP. Cell penetrating peptide-modified pharmaceutical nanocarriers for intracellular drug and gene delivery. Biopolymers 2008; 90:604-10; PMID:18381624; http://dx.doi.org/10.1002/bip.20989
- Borysowski J, Gorski A. Fusion to cell-penetrating peptides will enable lytic enzymes to kill intracellular bacteria. Med Hypotheses 2010; 74:164-6; PMID:19656633; http://dx.doi.org/10.1016/j.mehy.2009.07.006
- Freimer EH, Krause RM, Mc CM. Studies of L forms and protoplasts of group A streptococci. I. Isolation, growth, and bacteriologic characteristics. J Exp Med 1959; 110:853-74; PMID:13824817; http://dx.doi.org/10.1084/jem.110.6.853
- Cheng Q, Nelson D, Zhu S, Fischetti VA. Removal of group B streptococci colonizing the vagina and oropharynx of mice with a bacteriophage lytic enzyme. Antimicrob Agents Chemother 2005; 49:111-7; PMID:15616283; http://dx.doi.org/10.1128/AAC.49.1.111-117.2005
- Djurkovic S, Loeffler JM, Fischetti VA. Synergistic killing of Streptococcus pneumoniae with the bacteriophage lytic enzyme Cpl-1 and penicillin or gentamicin depends on the level of penicillin resistance. Antimicrob Agents Chemother 2005; 49:1225-8; PMID:15728935; http://dx.doi.org/10.1128/AAC.49.3.1225-1228.2005
- Lood R, Raz A, Molina H, Euler CW, Fischetti VA. A highly active and negatively charged Streptococcus pyogenes lysin with a rare D-alanyl-L-alanine endopeptidase activity protects mice against streptococcal bacteremia. Antimicrob Agents Chemother 2014; 58:3073-84; PMID:24637688; http://dx.doi.org/10.1128/AAC.00115-14
- Nelson D, Loomis L, Fischetti VA. Prevention and elimination of upper respiratory colonization of mice by group A streptococci by using a bacteriophage lytic enzyme. Proc Natl Acad Sci U S A 2001; 98:4107-12; PMID:11259652; http://dx.doi.org/10.1073/pnas.061038398
- Gilmer DB, Schmitz JE, Euler CW, Fischetti VA. Novel bacteriophage lysin with broad lytic activity protects against mixed infection by Streptococcus pyogenes and methicillin-resistant Staphylococcus aureus. Antimicrob Agents Chemother 2013; 57:2743-50; PMID:23571534; http://dx.doi.org/10.1128/AAC.02526-12
- Baker JR, Liu C, Dong S, Pritchard DG. Endopeptidase and glycosidase activities of the bacteriophage B30 lysin. Appl Environ Microbiol 2006; 72:6825-8; PMID:17021237; http://dx.doi.org/10.1128/AEM.00829-06
- Celia LK, Nelson D, Kerr DE. Characterization of a bacteriophage lysin (Ply700) from Streptococcus uberis. Vet Microbiol 2008; 130:107-17; PMID:18242012; http://dx.doi.org/10.1016/j.vetmic.2007.12.004
- Loeffler JM, Nelson D, Fischetti VA. Rapid killing of Streptococcus pneumoniae with a bacteriophage cell wall hydrolase. Science 2001; 294:2170-2; PMID:11739958; http://dx.doi.org/10.1126/science.1066869
- Cheng Q, Fischetti VA. Mutagenesis of a bacteriophage lytic enzyme PlyGBS significantly increases its antibacterial activity against group B streptococci. Appl Microbiol Biotechnol 2007; 74:1284-91; PMID:17186236; http://dx.doi.org/10.1007/s00253-006-0771-1
- Oechslin F, Daraspe J, Giddey M, Moreillon P, Resch G. In vitro characterization of PlySK1249, a novel phage lysin, and assessment of its antibacterial activity in a mouse model of Streptococcus agalactiae bacteremia. Antimicrob Agents Chemother 2013; 57:6276-83; PMID:24100496; http://dx.doi.org/10.1128/AAC.01701-13
- Loeffler JM, Djurkovic S, Fischetti VA. Phage lytic enzyme Cpl-1 as a novel antimicrobial for pneumococcal bacteremia. Infect Immun 2003; 71:6199-204; PMID:14573637; http://dx.doi.org/10.1128/IAI.71.11.6199-6204.2003
- Doehn JM, Fischer K, Reppe K, Gutbier B, Tschernig T, Hocke AC, Fischetti VA, Loffler J, Suttorp N, Hippenstiel S, et al. Delivery of the endolysin Cpl-1 by inhalation rescues mice with fatal pneumococcal pneumonia. J Antimicrob Chemother 2013; 68:2111-7; PMID:23633685; http://dx.doi.org/10.1093/jac/dkt131
- Jado I, Lopez R, Garcia E, Fenoll A, Casal J, Garcia P. Phage lytic enzymes as therapy for antibiotic-resistant Streptococcus pneumoniae infection in a murine sepsis model. J Antimicrob Chemother 2003; 52:967-73; PMID:14613958; http://dx.doi.org/10.1093/jac/dkg485
- Entenza JM, Loeffler JM, Grandgirard D, Fischetti VA, Moreillon P. Therapeutic effects of bacteriophage Cpl-1 lysin against Streptococcus pneumoniae endocarditis in rats. Antimicrob Agents Chemother 2005; 49:4789-92; PMID:16251333; http://dx.doi.org/10.1128/AAC.49.11.4789-4792.2005
- Grandgirard D, Loeffler JM, Fischetti VA, Leib SL. Phage lytic enzyme Cpl-1 for antibacterial therapy in experimental pneumococcal meningitis. J Infect Dis 2008; 197:1519-22; PMID:18471063; http://dx.doi.org/10.1086/587942
- Diez-Martinez R, de Paz HD, Bustamante N, Garcia E, Menendez M, Garcia P. Improving the lethal effect of cpl-7, a pneumococcal phage lysozyme with broad bactericidal activity, by inverting the net charge of its cell wall-binding module. Antimicrob Agents Chemother 2013; 57:5355-65; PMID:23959317; http://dx.doi.org/10.1128/AAC.01372-13
- Diez-Martinez R, De Paz HD, Garcia-Fernandez E, Bustamante N, Euler CW, Fischetti VA, Menendez M, Garcia P. A novel chimeric phage lysin with high in vitro and in vivo bactericidal activity against Streptococcus pneumoniae. J Antimicrob Chemother 2015; 70:1763-73; PMID:25733585
- Witzenrath M, Schmeck B, Doehn JM, Tschernig T, Zahlten J, Loeffler JM, Zemlin M, Muller H, Gutbier B, Schutte H, et al. Systemic use of the endolysin Cpl-1 rescues mice with fatal pneumococcal pneumonia. Crit Care Med 2009; 37:642-9; PMID:19114881; http://dx.doi.org/10.1097/CCM.0b013e31819586a6
- Rashel M, Uchiyama J, Ujihara T, Uehara Y, Kuramoto S, Sugihara S, Yagyu K, Muraoka A, Sugai M, Hiramatsu K, et al. Efficient elimination of multidrug-resistant Staphylococcus aureus by cloned lysin derived from bacteriophage phi MR11. J Infect Dis 2007; 196:1237-47; PMID:17955443; http://dx.doi.org/10.1086/521305
- Daniel A, Euler C, Collin M, Chahales P, Gorelick KJ, Fischetti VA. Synergism between a novel chimeric lysin and oxacillin protects against infection by methicillin-resistant Staphylococcus aureus. Antimicrob Agents Chemother 2010; 54:1603-12; PMID:20086153; http://dx.doi.org/10.1128/AAC.01625-09
- Pastagia M, Euler C, Chahales P, Fuentes-Duculan J, Krueger JG, Fischetti VA. A novel chimeric lysin shows superiority to mupirocin for skin decolonization of methicillin-resistant and -sensitive Staphylococcus aureus strains. Antimicrob Agents Chemother 2011; 55:738-44; PMID:21098252; http://dx.doi.org/10.1128/AAC.00890-10
- Gu J, Xu W, Lei L, Huang J, Feng X, Sun C, Du C, Zuo J, Li Y, Du T, et al. LysGH15, a novel bacteriophage lysin, protects a murine bacteremia model efficiently against lethal methicillin-resistant Staphylococcus aureus infection. J Clin Microbiol 2011; 49:111-7; PMID:21048011; http://dx.doi.org/10.1128/JCM.01144-10
- Gu J, Lu R, Liu X, Han W, Lei L, Gao Y, Zhao H, Li Y, Diao Y. LysGH15B, the SH3b domain of staphylococcal phage endolysin LysGH15, retains high affinity to staphylococci. Curr Microbiol 2011; 63:538-42; PMID:21947237; http://dx.doi.org/10.1007/s00284-011-0018-y
- O'Flaherty S, Coffey A, Meaney W, Fitzgerald GF, Ross RP. The recombinant phage lysin LysK has a broad spectrum of lytic activity against clinically relevant staphylococci, including methicillin-resistant Staphylococcus aureus. J Bacteriol 2005; 187:7161-4; PMID:16199588; http://dx.doi.org/10.1128/JB.187.20.7161-7164.2005
- Schmelcher M, Shen Y, Nelson DC, Eugster MR, Eichenseher F, Hanke DC, Loessner MJ, Dong S, Pritchard DG, Lee JC, et al. Evolutionarily distinct bacteriophage endolysins featuring conserved peptidoglycan cleavage sites protect mice from MRSA infection. J Antimicrob Chemother 2015; 70:1453-65; PMID:25630640; http://dx.doi.org/10.1093/jac/dku552
- Singh PK, Donovan DM, Kumar A. Intravitreal injection of the chimeric phage endolysin Ply187 protects mice from Staphylococcus aureus endophthalmitis. Antimicrob Agents Chemother 2014; 58:4621-9; PMID:24890598; http://dx.doi.org/10.1128/AAC.00126-14
- Jun SY, Jung GM, Son JS, Yoon SJ, Choi YJ, Kang SH. Comparison of the antibacterial properties of phage endolysins SAL-1 and LysK. Antimicrob Agents Chemother 2011; 55:1764-7; PMID:21263051; http://dx.doi.org/10.1128/AAC.01097-10
- Jun SY, Jung GM, Yoon SJ, Oh MD, Choi YJ, Lee WJ, Kong JC, Seol JG, Kang SH. Antibacterial properties of a pre-formulated recombinant phage endolysin, SAL-1. Int J Antimicrob Agents 2013; 41:156-61; PMID:23276502; http://dx.doi.org/10.1016/j.ijantimicag.2012.10.011
- Jun SY, Jung GM, Yoon SJ, Choi YJ, Koh WS, Moon KS, Kang SH. Preclinical safety evaluation of intravenously administered SAL200 containing the recombinant phage endolysin SAL-1 as a pharmaceutical ingredient. Antimicrob Agents Chemother 2014; 58:2084-8; PMID:24449776; http://dx.doi.org/10.1128/AAC.02232-13
- Holers VM. Complement and its receptors: new insights into human disease. Annu Rev Immunol 2014; 32:433-59; PMID:24499275; http://dx.doi.org/10.1146/annurev-immunol-032713-120154
- Schuch R, Nelson D, Fischetti VA. A bacteriolytic agent that detects and kills Bacillus anthracis. Nature 2002; 418:884-9; PMID:12192412; http://dx.doi.org/10.1038/nature01026
- Yoong P, Schuch R, Nelson D, Fischetti VA. PlyPH, a bacteriolytic enzyme with a broad pH range of activity and lytic action against Bacillus anthracis. J Bacteriol 2006; 188:2711-4; PMID:16547060; http://dx.doi.org/10.1128/JB.188.7.2711-2714.2006
- Morita M, Tanji Y, Orito Y, Mizoguchi K, Soejima A, Unno H. Functional analysis of antibacterial activity of Bacillus amyloliquefaciens phage endolysin against Gram-negative bacteria. FEBS Lett 2001; 500:56-9; PMID:11434926; http://dx.doi.org/10.1016/S0014-5793(01)02587-X
- Briers Y, Walmagh M, Lavigne R. Use of bacteriophage endolysin EL188 and outer membrane permeabilizers against Pseudomonas aeruginosa. J Appl Microbiol 2011; 110:778-85; PMID:21241420; http://dx.doi.org/10.1111/j.1365-2672.2010.04931.x
- Nakimbugwe D, Masschalck B, Deckers D, Callewaert L, Aertsen A, Michiels CW. Cell wall substrate specificity of six different lysozymes and lysozyme inhibitory activity of bacterial extracts. FEMS Microbiol Lett 2006; 259:41-6; PMID:16684100; http://dx.doi.org/10.1111/j.1574-6968.2006.00240.x
- Lim JA, Shin H, Heu S, Ryu S. Exogenous lytic activity of SPN9CC endolysin against Gram-negative bacteria. J Microbiol Biotechnol 2014; 24:803-11; PMID:24690638
- Morita M, Tanji Y, Mizoguchi K, Soejima A, Orito Y, Unno H. Antibacterial activity of Bacillus amyloliquefaciens phage endolysin without holin conjugation. J Biosci Bioeng 2001; 91:469-73; PMID:16233024; http://dx.doi.org/10.1016/S1389-1723(01)80275-9
- Orito Y, Morita M, Hori K, Unno H, Tanji Y. Bacillus amyloliquefaciens phage endolysin can enhance permeability of Pseudomonas aeruginosa outer membrane and induce cell lysis. Appl Microbiol Biotechnol 2004; 65:105-9; PMID:14714151; http://dx.doi.org/10.1007/s00253-003-1522-1
- Saussereau E, Debarbieux L. Bacteriophages in the experimental treatment of Pseudomonas aeruginosa infections in mice. Adv Virus Res 2012; 83:123-41; PMID:22748810
- Fischetti VA. Bacteriophage lytic enzymes: novel anti-infectives. Trends Microbiol 2005; 13:491-6; PMID:16125935; http://dx.doi.org/10.1016/j.tim.2005.08.007
- Pastagia M, Schuch R, Fischetti VA, Huang DB. Lysins: the arrival of pathogen-directed anti-infectives. J Med Microbiol 2013; 62:1506-16; PMID:23813275; http://dx.doi.org/10.1099/jmm.0.061028-0
- Kusuma C, Jadanova A, Chanturiya T, Kokai-Kun JF. Lysostaphin-resistant variants of Staphylococcus aureus demonstrate reduced fitness in vitro and in vivo. Antimicrob Agents Chemother 2007; 51:475-82; PMID:17101683; http://dx.doi.org/10.1128/AAC.00786-06
- Andersson DI, Hughes D. Microbiological effects of sublethal levels of antibiotics. Nat Rev Microbiol 2014; 12:465-78; PMID:24861036; http://dx.doi.org/10.1038/nrmicro3270
- Keary R, McAuliffe O, Ross RP, Hill C, O'Mahony J, Coffey A. Genome analysis of the staphylococcal temperate phage DW2 and functional studies on the endolysin and tail hydrolase. Bacteriophage 2014; 4:e28451; PMID:25105056; http://dx.doi.org/10.4161/bact.28451
- Rodriguez L, Martinez B, Zhou Y, Rodriguez A, Donovan DM, Garcia P. Lytic activity of the virion-associated peptidoglycan hydrolase HydH5 of Staphylococcus aureus bacteriophage vB_SauS-phiIPLA88. BMC Microbiol 2011; 11:138; PMID:21682850; http://dx.doi.org/10.1186/1471-2180-11-138
- Takac M, Blasi U. Phage P68 virion-associated protein 17 displays activity against clinical isolates of Staphylococcus aureus. Antimicrob Agents Chemother 2005; 49:2934-40; PMID:15980371; http://dx.doi.org/10.1128/AAC.49.7.2934-2940.2005
- Rodriguez-Rubio L, Martinez B, Rodriguez A, Donovan DM, Garcia P. Enhanced staphylolytic activity of the Staphylococcus aureus bacteriophage vB_SauS-phiIPLA88 HydH5 virion-associated peptidoglycan hydrolase: fusions, deletions, and synergy with LysH5. Appl Environ Microbiol 2012; 78:2241-8; PMID:22267667; http://dx.doi.org/10.1128/AEM.07621-11
- Paul VD, Rajagopalan SS, Sundarrajan S, George SE, Asrani JY, Pillai R, Chikkamadaiah R, Durgaiah M, Sriram B, Padmanabhan S. A novel bacteriophage Tail-Associated Muralytic Enzyme (TAME) from Phage K and its development into a potent antistaphylococcal protein. BMC Microbiol 2011; 11:226; PMID:21985151; http://dx.doi.org/10.1186/1471-2180-11-226
- Vipra AA, Desai SN, Roy P, Patil R, Raj JM, Narasimhaswamy N, Paul VD, Chikkamadaiah R, Sriram B. Antistaphylococcal activity of bacteriophage derived chimeric protein P128. BMC Microbiol 2012; 12:41; PMID:22439788; http://dx.doi.org/10.1186/1471-2180-12-41
- Bartell PF, Orr TE. Origin of polysaccharide depolymerase associated with bacteriophage infection. J Virol 1969; 3:290-6; PMID:4976560
- Bartell PF, Orr TE, Lam GK. Polysaccharide depolymerase associated with bacteriophage infection. J Bacteriol 1966; 92:56-62; PMID:4957437
- Scholl D, Adhya S, Merril C. Escherichia coli K1's capsule is a barrier to bacteriophage T7. Appl Environ Microbiol 2005; 71:4872-4; PMID:16085886; http://dx.doi.org/10.1128/AEM.71.8.4872-4874.2005
- Nwodo UU, Green E, Okoh AI. Bacterial exopolysaccharides: functionality and prospects. Int J Mol Sci 2012; 13:14002-15; PMID:23203046; http://dx.doi.org/10.3390/ijms131114002
- Kumar AS, Mody K, Jha B. Bacterial exopolysaccharides–a perception. J Basic Microbiol 2007; 47:103-17; PMID:17440912; http://dx.doi.org/10.1002/jobm.200610203
- Looijesteijn PJ, Trapet L, de Vries E, Abee T, Hugenholtz J. Physiological function of exopolysaccharides produced by Lactococcus lactis. Int J Food Microbiol 2001; 64:71-80; PMID:11252513; http://dx.doi.org/10.1016/S0168-1605(00)00437-2
- Mushtaq N, Redpath MB, Luzio JP, Taylor PW. Treatment of experimental Escherichia coli infection with recombinant bacteriophage-derived capsule depolymerase. J Antimicrob Chemother 2005; 56:160-5; PMID:15914489; http://dx.doi.org/10.1093/jac/dki177
- Sutherland IW. Polysaccharases for microbial exopolysaccharides. Carbohydrate Polymers 1999; 38:319-28; http://dx.doi.org/10.1016/S0144-8617(98)00114-3
- Casjens SR, Thuman-Commike PA. Evolution of mosaically related tailed bacteriophage genomes seen through the lens of phage P22 virion assembly. Virology 2011; 411:393-415; PMID:21310457; http://dx.doi.org/10.1016/j.virol.2010.12.046
- Waseh S, Hanifi-Moghaddam P, Coleman R, Masotti M, Ryan S, Foss M, MacKenzie R, Henry M, Szymanski CM, Tanha J. Orally administered P22 phage tailspike protein reduces salmonella colonization in chickens: prospects of a novel therapy against bacterial infections. PLoS One 2010; 5:e13904; PMID:21124920; http://dx.doi.org/10.1371/journal.pone.0013904
- Glonti T, Chanishvili N, Taylor PW. Bacteriophage-derived enzyme that depolymerizes the alginic acid capsule associated with cystic fibrosis isolates of Pseudomonas aeruginosa. J Appl Microbiol 2010; 108:695-702; PMID:19709344; http://dx.doi.org/10.1111/j.1365-2672.2009.04469.x
- Dubos R, Avery OT. Decomposition of the capsular polysaccharide of pneumococcus type III by a bacterial enzyme. J Exp Med 1931; 54:51-71; PMID:19869902; http://dx.doi.org/10.1084/jem.54.1.51
- Goodner K, Dubos R. Studies on the quantitative action of a specific enzyme in type III pneumococcus dermal infection in rabbits. J Exp Med 1932; 56:521-30; PMID:19870083; http://dx.doi.org/10.1084/jem.56.4.521
- Goodner K, Dubos R, Avery OT. The action of a specific enzyme upon the dermal infection of tabbits with type III pneumococcus. J Exp Med 1932; 55:393-404; PMID:19869998; http://dx.doi.org/10.1084/jem.55.3.393
- Francis T, Terrell EE, Dubos R, Avery OT. Experimental type III pneumococcus pneumonia in monkeys: Treatment with an enzyme which decomposes the specific capsular polysaccharide of pneumococcus type III. J Exp Med 1934; 59:641-67; PMID:19870270; http://dx.doi.org/10.1084/jem.59.5.641
- Zelmer A, Martin MJ, Gundogdu O, Birchenough G, Lever R, Wren BW, Luzio JP, Taylor PW. Administration of capsule-selective endosialidase E minimizes upregulation of organ gene expression induced by experimental systemic infection with Escherichia coli K1. Microbiology 2010; 156:2205-15; PMID:20395269; http://dx.doi.org/10.1099/mic.0.036145-0
- Kim WS, Salm H, Geider K. Expression of bacteriophage phiEa1h lysozyme in Escherichia coli and its activity in growth inhibition of Erwinia amylovora. Microbiology 2004; 150:2707-14; PMID:15289567; http://dx.doi.org/10.1099/mic.0.27224-0
- Malnoy M, Faize M, Venisse JS, Geider K, Chevreau E. Expression of viral EPS-depolymerase reduces fire blight susceptibility in transgenic pear. Plant Cell Rep 2005; 23:632-8; PMID:15375629; http://dx.doi.org/10.1007/s00299-004-0855-2
- Roach DR, Khatibi PA, Bischoff KM, Hughes SR, Donovan DM. Bacteriophage-encoded lytic enzymes control growth of contaminating Lactobacillus found in fuel ethanol fermentations. Biotechnol Biofuels 2013; 6:20; PMID:23390890; http://dx.doi.org/10.1186/1754-6834-6-20
- Bansal S, Harjai K, Chhibber S. Depolymerase improves gentamicin efficacy during Klebsiella pneumoniae induced murine infection. BMC Infect Dis 2014; 14:456; PMID:25149315; http://dx.doi.org/10.1186/1471-2334-14-456
- Hughes KA, Sutherland IW, Jones MV. Biofilm susceptibility to bacteriophage attack: the role of phage-borne polysaccharide depolymerase. Microbiology 1998; 144 (Pt 11):3039-47; PMID:9846739; http://dx.doi.org/10.1099/00221287-144-11-3039
- Bales PM, Renke EM, May SL, Shen Y, Nelson DC. Purification and characterization of biofilm-associated EPS exopolysaccharides from ESKAPE organisms and other pathogens. PLoS One 2013; 8:e67950; PMID:23805330; http://dx.doi.org/10.1371/journal.pone.0067950
- Pang T, Fleming TC, Pogliano K, Young R. Visualization of pinholin lesions in vivo. Proc Natl Acad Sci U S A 2013; 110:E2054-63; PMID:23671069; http://dx.doi.org/10.1073/pnas.1222283110
- Young R. Bacteriophage holins: deadly diversity. J Mol Microbiol Biotechnol 2002; 4:21-36; PMID:11763969
- Rajesh T, Anthony T, Saranya S, Pushpam PL, Gunasekaran P. Functional characterization of a new holin-like antibacterial protein coding gene tmp1 from goat skin surface metagenome. Appl Microbiol Biotechnol 2011; 89:1061-73; PMID:20927512; http://dx.doi.org/10.1007/s00253-010-2907-6
- Rodas PI, Trombert AN, Mora GC. A holin remnant protein encoded by STY1365 is involved in envelope stability of Salmonella enterica serovar Typhi. FEMS Microbiol Lett 2011; 321:58-66; PMID:21592194; http://dx.doi.org/10.1111/j.1574-6968.2011.02310.x
- Wang Y, Sun JH, Lu CP. Purified recombinant phage lysin LySMP: an extensive spectrum of lytic activity for swine streptococci. Curr Microbiol 2009; 58:609-15; PMID:19267155; http://dx.doi.org/10.1007/s00284-009-9379-x
- Shi Y, Li N, Yan Y, Wang H, Li Y, Lu C, Sun J. Combined antibacterial activity of phage lytic proteins holin and lysin from Streptococcus suis bacteriophage SMP. Curr Microbiol 2012; 65:28-34; PMID:22526567; http://dx.doi.org/10.1007/s00284-012-0119-2
- Saier MH, Jr., Reddy BL. Holins in bacteria, eukaryotes, and archaea: multifunctional xenologues with potential biotechnological and biomedical applications. J Bacteriol 2015; 197:7-17; PMID:25157079; http://dx.doi.org/10.1128/JB.02046-14
- Schmelcher M, Powell AM, Becker SC, Camp MJ, Donovan DM. Chimeric phage lysins act synergistically with lysostaphin to kill mastitis-causing Staphylococcus aureus in murine mammary glands. Appl Environ Microbiol 2012; 78:2297-305; PMID:22286996; http://dx.doi.org/10.1128/AEM.07050-11
- Fenton M, Ross P, McAuliffe O, O'Mahony J, Coffey A. Recombinant bacteriophage lysins as antibacterials. Bioeng Bugs 2010; 1:9-16; PMID:21327123; http://dx.doi.org/10.4161/bbug.1.1.9818
- Gu J, Zuo J, Lei L, Zhao H, Sun C, Feng X, Du C, Li X, Yang Y, Han W. LysGH15 reduces the inflammation caused by lethal methicillin-resistant Staphylococcus aureus infection in mice. Bioeng Bugs 2011; 2:96-9; PMID:21636996; http://dx.doi.org/10.4161/bbug.2.2.14883
- McCullers JA, Karlstrom A, Iverson AR, Loeffler JM, Fischetti VA. Novel strategy to prevent otitis media caused by colonizing Streptococcus pneumoniae. PLoS Pathog 2007; 3:e28; PMID:17381239; http://dx.doi.org/10.1371/journal.ppat.0030028
- Loeffler JM, Fischetti VA. Synergistic lethal effect of a combination of phage lytic enzymes with different activities on penicillin-sensitive and -resistant Streptococcus pneumoniae strains. Antimicrob Agents Chemother 2003; 47:375-7; PMID:12499217; http://dx.doi.org/10.1128/AAC.47.1.375-377.2003