Abstract
Brown fat has gained widespread attention as a potential therapeutic target to treat obesity and associated metabolic disorders. Indeed, the anti-obesity potential of multiple targets to stimulate both brown adipocyte differentiation and recruitment have been verified in rodent models. However, their therapeutic potential in humans is unknown due to the lack of a human primary brown adipocyte cell culture system. Likewise, the lack of a well-characterized human model has limited the discovery of novel targets for the activation of human brown fat. To address this current need, we aimed to identify and describe the first primary brown adipocyte cell culture system from human fetal interscapular brown adipose tissue. Pre-adipocytes isolated from non-viable human fetal interscapular tissue were expanded and cryopreserved. Cells were then thawed and plated alongside adult human subcutaneous and omental pre-adipocytes for subsequent differentiation and phenotypic characterization. Interscapular pre-adipocytes in cell culture differentiated into mature adipocytes that were morphologically indistinguishable from the adult white depots. Throughout differentiation, cultured human fetal interscapular adipocytes demonstrated increased expression of classical brown fat markers compared to subcutaneous and omental cells. Further, functional analysis revealed an elevation in fatty acid oxidation as well as maximal and uncoupled oxygen consumption in interscapular brown adipocytes compared to white control cells. These data collectively identify the brown phenotype of these cells. Thus, our primary cell culture system derived from non-viable human fetal interscapular brown adipose tissue provides a valuable tool for the study of human brown adipocyte biology and for the development of anti-obesity therapeutics.
Introduction
The incidence of obesity has reached epidemic proportions over the past decades causing a surge in associated comorbidities including type 2 diabetes, ischemic cerebrovascular disease and certain cancers.Citation1 One attractive approach for the treatment of obesity is to augment energy expenditure through enhanced brown adipose tissue (BAT) activity and prevalence or through “browning” of white adipose tissue (WAT).Citation2,3 Found mainly in the interscapular region in small mammals and human infants, brown fat is a specialized organ that burns lipids for heat generation and energy expenditure as a defense against cold and obesity. Brown adipocytes contain multiple small lipid-droplets, are packed with a high mitochondrial density, and express elevated levels of uncoupling protein 1 (Ucp1), which uncouples the electric-chemical gradient across the inner membrane from ATP synthesis. Another type of brown adipocyte, referred to as beige adipocytes, can be found in subcutaneous adipose tissue. At baseline, beige adipocytes express low levels of Ucp1 and other thermogenic genes. However, in response to stimulators such as agonists of the β-adrenergic receptor or peroxisome proliferator-activated receptor-γ (Ppar-γ), they undergo a phenotypic change to enhance mitochondrial biogenesis and increase expression of functional Ucp1Citation4 as well as other thermogenic genes.Citation3,5 Thus, both classical brown adipocytes and beige adipocytes represent potential therapeutic targets for obesity.
The ability of BAT to enhance energy expenditure provides a natural defense against obesity. However, the prevalence of BAT in human adults has not been appreciated until recently. Using positron emission tomography-computer tomography (PET-CT), researchers have detected Ucp1+ metabolically active adipocytes in the cervical, supraclavicular, axillary, and paravertebral regions of adult human subjects.Citation6-8 The activity of brown fat has been demonstrated to be inversely correlated with body mass index (BMI), although it remains unclear whether reduced BAT activity is a cause or a consequence of weight gain in humans.Citation6-9 Gene expression signature comparisons suggest that BAT around the adult neck consists of both beige and classical brown adipocytes, while infant interscapular BAT contains only classical brown adipocytes similar to those found in small mammals.Citation10-12
Studies in rodent models have led to significant progress in understanding the mechanisms underlying brown adipocyte development and activation. For example, researchers have identified a full cascade of transcriptional factors and co-factors that promote or suppress brown adipocyte development.Citation13,14 Additionally, several groups, including our own, have demonstrated that microRNAs constitute another layer of regulation in BAT development.Citation15,16 However, the significant differences between mouse and human adipocyte biology make the therapeutic potential of these and other candidates uncertain. A major bottleneck for human brown fat research is the limited availability of both adult and infant BAT and their derivative primary brown adipocyte cultures. Recently, Shingo group has established a few immortalized brown and white preadipocyte cell lines from supraclavicular adipose tissues in adults, but a cell model for interscapular brown adipocytes is still being developed.Citation17 A brown pre-adipocyte cell line, PAZ6, has been derived from human infant brown fat by virus 40 T immortalization,Citation18,19 but the mechanisms regulating differentiation and the metabolic features of the cell line can be very different from primary cell cultures. Herein, we aimed to describe a human primary brown adipocyte culture system from human fetal interscapular BAT (fiBAT). We successfully cultured these cells and demonstrate a well characterized brown phenotype described herein. We believe these cells will provide a valuable tool for both the validation of existing rodent targets and identification of novel human targets of brown fat activation and research.
Research Design and Methods
Ethics statement
All human fetal tissue was obtained from Advanced Bioscience Resources (Alameda, CA) from deceased donors as approved under exemption 4 in the HHS regulations (45 CFR Part 46). ABR follows established procedures for written informed parental consent. Zen-Bio conducted basic research in accordance with NIH guidelines and the Federal Provisions Pertaining to Research Use of Human Fetal Tissue by NIH Investigators. Zen-Bio's research related to human tissues is approved under its IRB through PearlIRB.
Materials
Sodium oleate, FCCP, antimycin, rotenone, DAPI, BODIPY, mitotracker and Oil-Red-O were purchased from Sigma (St-Louis, MO). Oligomycin was purchased from Calbiochem (Billerica, MA). Bovine serum albumin (BSA, Fraction V 7.5% cell culture grade) was obtained from Invitrogen (Carlsbad, CA). [1−14C] oleic acid was purchased from Perkin Elmer (Boston, MA). Reagents for SDS-PAGE were supplied by Bio-Rad (Hercules, CA). Anti-UCP1 (ab125480) was supplied by Abcam (Cambridge, MA) and peroxidase-conjugated goat anti-rabbit anitobody was obtained from Amersham (Piscataway, NJ). Ani-PGC1α and Anti-FABP4 were purchased from Santa Cruz Biotechnology (Dallas, TX). Fetal interscapular brown pre-adipocytes as well as adult subcutaneous and omental pre-adipocytes were provided by ZenBio (Research Triangle Park, NC).
Cell isolation
Brown adipose tissue was transported in FBS and antibiotic containing DMEM and then minced and transferred to a solution containing 1% collagenase, 1% PBS and 1% BSA for 45 minutes at 37°C with gentle inversion. The solution was then strained through a nylon mesh strainer, supplemented with serum-containing medium and centrifuged to produce a brown preadipocyte cell pellet. After 24 hours in serum-containing plating medium, cells were cultured at 37°C, 5% CO2 in pre-adipocyte medium.
Cell culture
Cryopreserved primary human pre-adipocytes isolated from adult subcutaneous, adult omental and fetal interscapular tissue were thawed and plated at a density of 40 000 cells/cm2 using DMEM/Ham's F-12 medium (1:1, v/v) supplemented with HEPES pH 7.4, fetal bovine serum 10%, penicillinandstreptomycin (1:100) and amphotericin B (1 ug/ml). Once cells reached confluence, they are induced to differentiate using differentiation medium (DMEM, FBS 10%, IMBX 0.25 mM, Dexamethasone 0.5 µM, Insulin 850nM, Indomethacin 100 uM, Rosiglitazone 1uM, penicillin and streptomycin (1:100), Fungizone/Amphotericin B(1 ug/ml)). Cells were maintained in this media for 7 days after which the media was changed to maintenance medium (DMEM, FBS 10%, Insulin 160 nM, Peniclillin and streptomycin (1:100), Fungizone/Amphotericin B (1 ug/ml)). Cells were maintained in this manner with media changed every 2 days until their use in experiments at approximately 21 days post differentiation.
Real-time PCR
RNA was extracted from pre-adipocytes and adipocytes using RNeasy (Qiagen, Valencia, CA), and quantified using a Nanodrop spectrophotometer (ThermoScientific, Wilmington, DE). cDNA was synthesized from 1μg RNA using the IScript cDNA synthesis kit (Bio-Rad Laboratories, Hercules, CA). Gene expression was determined by real-time quantitative PCR using a Prism HT7900 instrument with SYBR Green chemistry (Applied Biosystems, Foster City, CA).
Oleate oxidation
Complete [1−14C]Oleate oxidation to [14C]CO2 was measured using adapted methods described.Citation20,21 The capture of [14C]CO2 was measured after a 3 hour incubation with 300 µM oleate in the presence of 1 mM carnitine. Reactions were terminated by adding 100 ml 70% perchloric acid and [14C]CO2 was trapped in 200 ml of 1 N NaOH.
Western blotting
Cells were lysed with radioimmunoprecipitation assay (RIPA) buffer (0.5% NP-40, 0.1% SDS, 150 mM NaCl, 50mM Tris-Cl at pH 7.5). Proteins were separated by SDS-PAGE, transferred to a nitrocellulose membrane (Millipore, Billerica, MA) and probed with antibodies listed above.
Respirometry
Respirometry of cultured primary adipocytes was performed using an XF24 Extracellular Flux Analyzer (Seahorse Bioscience, North Billerica, MA). Pre-adipocytes were seeded (40 000 cells/cm2) on XF24 V7 cell culture microplates and allowed to undergo differentiation as above. On day 21 post differentiation the medium was replaced with prewarmed XF24 assay medium for 1 hour and respirometry was carried out as previously described (16). Briefly, basal respiration was assessed in untreated cells then ATP turnover was calculated in response to 10 µM oligomycin. Maximum respiratory capacity was assessed after 1 µM FCCP stimulation. Finally, mitochondrial respiration was blocked by 1 µM Rotenone and the residual OCR was considered non-mitochondrial respiration. Proton leak was calculated by subtracting the ATP turnover and the non-mitochondrial respiration components of basal respiration.
Statistical analysis
Data are expressed as mean ± SE unless otherwise indicated. Student's t-test (unpaired, 2-tailed) was used to compare 2 groups and the P value was calculated using Excel (Microsoft) or Graphpad Prism 5 (Graphpad Software). P < 0.05 was considered statistically significant.
Results
Isolation of pre-adipocytes
Isolation and preparation of human subcutaneous and omental pre-adipocytes has been previously described.Citation22 Adipose tissue was dissected away from overlying skin and connective tissue and minced and digested in a collagenase solution (). Cells were plated on tissue-culture treated plastic dishes and expanded followed by cryopreservation.
Figure 1. Isolation and characterization of human adipose tissue from various depots. (A) Schematic of procedure for isolation of adipose tissue and preadipocytes from adult human subcutaneous and omental as well as fetal interscapular sites. (B) RT-PCR of RNA isolated from adult human subcutaneous (sub), fetal interscapular (fiBAT) and adult human omental (ome) adipose tissue. UCP-1, uncoupling protein-1; ZIC1, zinc finger of the cerebellum1; PGC1-α, peroxisome proliferator-activated receptor gamma co-activator 1-α; PPAR-α, peroxisome proliferator-activated receptor α; LHX, LIM homeobox; PPAR-γ, peroxisome proliferator-activated receptor gamma.
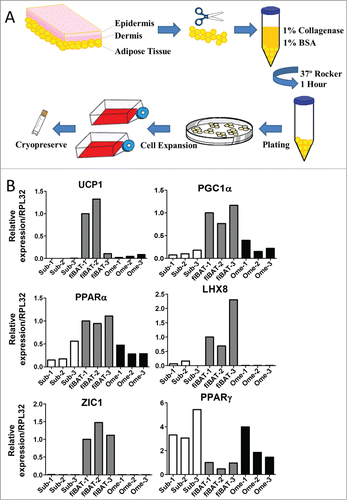
During sample processing, a portion of the tissue was set aside for RNA isolation followed by real-time PCR to evaluate expression levels of selected adipose markers (). Common brown-fat specific markers, including UCP-1, peroxisome proliferator-activated receptor gamma co-activator 1-α (PGC1-α) and peroxisome proliferator-activated receptor α (PPAR-α), were enriched in the fetal interscapular BAT (fiBAT) compared to subcutaneous and omental WAT. To further determine whether our samples were from classical or beige BAT, we examined the expression of 2 recently identified classic BAT markers, Zinc finger of the cerebellum1 (ZIC1) and LIM homeobox protein 8 (LHX8).Citation10,11,23 Both markers were highly enriched in fiBAT, indicative of fiBAT equivalent to the interscapular BAT seen in rodents. Alternatively, peroxisome proliferator-activated receptor gamma (PPARγ), a hallmark of adipogenesis, was more abundant in subcutaneous and omental WAT than in fiBAT.
Differentiation into mature adipocytes
To investigate the potential for a human fiBAT adipocyte primary culture system, we thawed and plated cryopreserved fetal interscapular pre-adipocytes. The fiBAT cells were compared to adult human subcutaneous and omental pre-adipocytes, all of which were grown to confluence and then differentiated. Prior to differentiation, cells from all depots were similar in appearance as observed by light microscopy (). After 7 days of differentiation, pre-adipocytes from all 3 depots began to take on a different morphology with sparse multi-locular lipid droplets. Cells were maintained in culture medium without induction cocktail for an additional 14 days with media changed every 2 days. At 21 days post differentiation, cells from all depots had developed clearly visible lipid droplets as determined by phase-contrast appearance as well as by staining with lipid-specific dyes including BODIPY and Oil-Red-O (). Differentiation efficiency based on visual appearance of the cells was approximately 50% for the human adult subcutaneous and fiBAT cellsand less than 10% for the human adult omental adipocytes. The differentiation efficiency of fiBAT doesn't significantly decrease within 5 passages (Data not shown).
Figure 2. Morphologic appearance of cultured primary human adipocytes. Cryopreserved primary adult human subcutaneous and omental as well as primary fetal human interscapular pre-adipocytes were thawed and plated on tissue culture dishes. Cells were grown to confluence and then differentiated. (A) Phase-contrast images of adipocytes during various stages of in vitro differentiation. (B and C) Appearance of cells after staining for lipid using BODIPY (B) and oil-red-o (C).
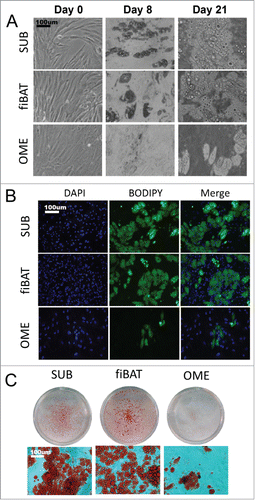
Molecular phenotype of adipocytes
To further characterize the molecular phenotype of our culture system, we performed real-time PCR to examine common BAT-specific makers during cell differentiation. Two common adipogenesis markers, FABP4 and PPARγ, were dramatically up-regulated in all 3 cell types, with highest expression in subcutaneous adipocytes (). Three BAT markers, including UCP1, CIDEA and PGC1α, were increased during differentiation, with the highest expression seen in fiBAT adipocytes (). Interestingly, the other 3 BAT markers examined, including PRDM16, LHX8 and ZIC1, were not induced during adipogenesis, but had a higher expression in fiBAT across the full differentiation time course. We have observed a significant induction of PRDM16 in mouse BAT cell differentiationCitation24 but not in our human cell culture system, highlighting a significant difference between mouse and human BAT systems. Moreover, we compared the expression of these markers in fiBAT and in the fetal BAT (). PPARγ is expressed at a higher level in our cell culture, probably due to the prolonged exposure of PPRRγ agonist; albeit at lower levels, the expression of UCP1 and CIDEA is still comparable with those in brown adipose tissue; FABP4, PGC1a and PRDM16 are expressed at similar levels. Thus, all the markers examined are well expressed in our differentiated fiBAT cells to an extent comparable with those in interscapular BAT.
Figure 3. Molecular characterization of cultured primary human adipocytes. Adult human primary subcutaneous and omental pre-adipocytes and fetal human interscapular pre-adipocytes were differentiated in culture. Total RNA was extracted at various times during differentiation for RT-PCR analysis of common adipogenic markers (A) and BAT markers (B). The expression of these markers in fiBAT and interscapular adipose tissue is compared in (C). Total protein was isolated from adipocytes at Day 0 and Day 21 of differentiation for Western blot analysis of UCP-1 (D), PGC1α and FABP4 expression (E). * (P < 0.05) Gene expression level in BAT in comparison with SUB, analyzed by Student's t-test. CIDEA, cell death-inducing DFFA-like effector a.
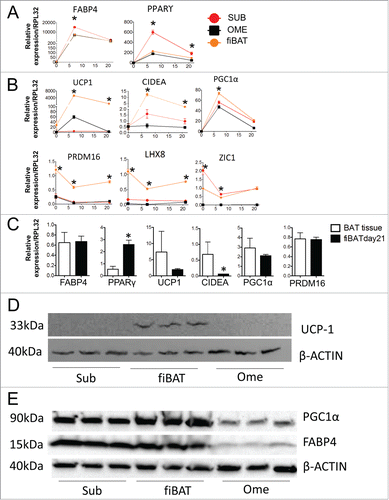
Protein was isolated from fully differentiated adipocytes for Western blotting (). UCP1 protein was undetectable in both subcutaneous and omental adipocytes but clearly present in BAT adipocytes. PGC1α could be readily detected in all 3 adipocyte systems but was most abundant in fiBAT. In contrast, FABP4 showed comparable expression in Sub and fiBAT adipocytes, but much lower levels in omental adipocytes, consistent with the reduced differentiation efficiency observed in . The real-time PCR and Western results, taken together, demonstrate that our cultured BAT adipocytes have gained key BAT molecular features.
Metabolic characterization of adipocytes
Mouse BAT adipocytes are characterized by high mitochondrial density and metabolic activity. To assess these features in human BAT adipocyte culture, we first stained adipocytes 21 days post differentiation with mitotracker dye to evaluate mitochondrial density. As shown in (), staining of adipocyte cultures revealed an elevated mitochondrial signal in our fiBAT adipocyte cultures over subcutaneous and omental controls. In addition, norepinephrine treatment induced the expression of thermogenic markers UCP1 and PGC1a (), indicating that these cells are responsive to thermogenic stimulation.
Figure 4. Metabolic characterization of cultured primary human adipocytes. Cryopreserved adult human primary subcutaneous and omental as well as primary fetal human interscapular pre-adipocytes were thawed and plated on tissue culture dishes. Cells were grown to confluence and then differentiated. (A) At day 21 of differentiation cells were stained with mitotracker. The redness in each picture is quantified with ImageJ and the quantification results are displayed in the right panel. n = 3. (B) Day 21 fiBAT cells were treated with 1 μM norepinephrine (NE) for 3 hours and real-time PCR was performed to examine the expression of UCP1 and PGC1α. (C) Day 21 primary subcutaneous and interscapular adipocytes were incubated with [1−14C] oleate. Label incorporation in CO2 was measured to assess rates of fatty acid oxidation. (D and E) The metabolic profile of cultured adipocytes (day 21) was assessed using a Seahorse XF24 extracellular flux analyzer. (D) Representative curves of the oxygen consumption rates (OCR) of adipocytes in their basal states and when treated with drugs to dissect the multiple components of the respiration process. (E) Quantitation of the OCRs from part C. * Significant (P < 0.05) ORC level for fiBAT in comparison with SUB, analyzed by Student's t-test.
![Figure 4. Metabolic characterization of cultured primary human adipocytes. Cryopreserved adult human primary subcutaneous and omental as well as primary fetal human interscapular pre-adipocytes were thawed and plated on tissue culture dishes. Cells were grown to confluence and then differentiated. (A) At day 21 of differentiation cells were stained with mitotracker. The redness in each picture is quantified with ImageJ and the quantification results are displayed in the right panel. n = 3. (B) Day 21 fiBAT cells were treated with 1 μM norepinephrine (NE) for 3 hours and real-time PCR was performed to examine the expression of UCP1 and PGC1α. (C) Day 21 primary subcutaneous and interscapular adipocytes were incubated with [1−14C] oleate. Label incorporation in CO2 was measured to assess rates of fatty acid oxidation. (D and E) The metabolic profile of cultured adipocytes (day 21) was assessed using a Seahorse XF24 extracellular flux analyzer. (D) Representative curves of the oxygen consumption rates (OCR) of adipocytes in their basal states and when treated with drugs to dissect the multiple components of the respiration process. (E) Quantitation of the OCRs from part C. * Significant (P < 0.05) ORC level for fiBAT in comparison with SUB, analyzed by Student's t-test.](/cms/asset/4768ed6a-8ac8-45d5-b121-741769a6f35f/kadi_a_1042192_f0004_c.gif)
To evaluate the functional effects of this difference, we performed substrate oxidation studies on subcutaneous and interscapular adipocyte cultures with radio-labeled oleate. Interscapular adipocytes demonstrated increased rates of oleate oxidation compared to subcutaneous adipocytes (2.3 vs 1.6 nmol/mg protein/hr; ). We next examined oxygen consumption rates (OCR) using respirometry. Both tracing and quantitation of oxygen consumption rates demonstrated equal rates of basal respiration and ATP turnover between subcutaneous and fiBAT adipocyte cultures (). The OCR observed from primary omental adipocyte cultures was significantly lower, likely a reflection of the poor differentiation capacity of these cells rather than innate differences in mitochondrial activity. Importantly, fiBAT adipocyte cultures had significantly higher rates of maximal respiration as well as oxygen consumption tied to proton leak, both features consistent with a brown fat metabolic phenotype. Thus, the adipocytes derived from our culture system are bona fide brown adipocytes.
Herein we describe a system for the isolation and culture of primary adipocytes derived from human fetal interscapular brown adipose tissue. Cells from this depot proved amenable to cryopreservation followed by thawing, expansion and differentiation into mature-appearing adipocytes. Analysis of gene and protein expression patterns during differentiation revealed enrichment in brown fat-specific genes and/or proteins including UCP-1, Cidea, LHX8 and Zic1. Compared to subcutaneous sadipocytes, the fetal interscapular cultures showed increased mitochondrial density, higher rates of fatty acid oxidation and increased maximal and uncoupled oxygen consumption. Importantly, these studies support the brown fat identity of the described human primary fetal interscapular adipocyte cell culture system. We acknowledge that there are other human brown adipocyte systems under development, such as immortalized preadipocyte line from supraclavicular adipose tissues iPS-derived adipocytes under brown adipogenic conditions and engineered human BAT cells,Citation17,25,26 but these systems are not derived from bona fide interscapular brown fat origin, their development process and biological function may be different from real brown adipocytes. Thus, the human primary brown adipocyte culture model in describe here will provide a unique value for the study of human brown fat biology.
Disclosure of Potential Conflicts of Interest
ZenBio declares that they will provide retail products described herein in a commercial capacity.
Author Contributions
SS and DX performed experiments and contributed to manuscript preparation. JPH performed experiments. BMB and JWL provided materials and contributed to the discussion. JPK and LS designed and performed experiments and wrote the manuscript.
Acknowledgments
Dr. Lei Sun is the guarantor of this work and had full access to all the data in the study and takes responsibility for the integrity of the data and the accuracy of the data analysis.
Funding
This work was supported by Duke-NUS Graduate Medical School, Singapore (JPK) and by Singapore NRF fellowship (NRF-2011NRF-NRFF 001-025) to LS.
References
- Haslam DW, James WPT. Obesity. Lancet 2005; 366:1197-209; PMID:16198769; http://dx.doi.org/10.1016/S0140-6736(05)67483-1
- Tseng Y-H, Cypess AM, Kahn CR. Cellular bioenergetics as a target for obesity therapy. Nat Rev Drug Discov 2010; 9:465-82; PMID:20514071; http://dx.doi.org/10.1038/nrd3138
- Harms M, Seale P. Brown and beige fat: development, function and therapeutic potential. Nat Med Nature Publishing Group; 2013; 19:1252-63; PMID:24100998; http://dx.doi.org/10.1038/nm.3361
- Shabalina I, Petrovic N, deJong JA, Kalinovich A, Cannon B, Nedergaard J. UCP1 in Brite/Beige adipose tissue mitochondria is functionally thermogenic. Cell Rep The Authors; 2013; 5:1196-203; PMID:24290753; http://dx.doi.org/10.1016/j.celrep.2013.10.044
- Wu J, Cohen P, Spiegelman BM. Adaptive thermogenesis in adipocytes: Is beige the new brown? Genes Dev 2013; 27:234-50; PMID:23388824; http://dx.doi.org/10.1101/gad.211649.112
- Cypess AM, Lehman S, Williams G, Tal I, Rodman D, Goldfine AB, Kuo FC, Palmer EL, Tseng YH, Doria A, et al. Identification and importance of brown adipose tissue in adult humans. N Engl J Med 2009; 360:1509-17; PMID:19357406; http://dx.doi.org/10.1097/OGX.0b013e3181ac8aa2
- Virtanen KA, Lidell ME, Orava J, Heglind M, Westergren R, Niemi T, Taittonen M, Laine J, Savisto NJ, Enerbäck S, et al. Functional brown adipose tissue in healthy adults. N Engl J Med 2009; 360:1518-25; PMID:19357407; http://dx.doi.org/10.1056/NEJMoa0808949
- Van Marken Lichtenbelt WD, Vanhommerig JW, Smulders NM, Drossaerts JMAFL, Kemerink GJ, Bouvy ND, Schrauwen P, Teule GJ. Cold-activated brown adipose tissue in healthy men. N Engl J Med 2009; 360:1500-8; PMID:19357405; http://dx.doi.org/10.1056/NEJMoa0808718
- Zingaretti MC, Crosta F, Vitali A, Guerrieri M, Frontini A, Cannon B, Nedergaard J, Cinti S. The presence of UCP1 demonstrates that metabolically active adipose tissue in the neck of adult humans truly represents brown adipose tissue. FASEB J 2009; 23:3113-20; PMID:19417078; http://dx.doi.org/10.1096/fj.09-133546
- Cypess AM, White AP, Vernochet C, Schulz TJ, Xue R, Sass CA, Huang TL, Roberts-Toler C, Weiner LS, Sze C, et al. Anatomical localization, gene expression profiling and functional characterization of adult human neck brown fat. Nat Med Nature Publishing Group; 2013; 19:635-9; PMID:23603815; http://dx.doi.org/10.1038/nm.3112
- Lidell ME, Betz MJ, Leinhard OD, Heglind M, Elander L, Slawik M, Mussack T, Nilsson D, Romu T, Nuutila P, et al. Evidence for two types of brown adipose tissue in humans. Nat Med 2013; 19:631-4; PMID:23603813; http://dx.doi.org/10.1038/nm.3017
- Jespersen NZ, Larsen TJ, Peijs L, Daugaard S, Homøe P, Loft A, de Jong J, Mathur N, Cannon B, Nedergaard J, et al. A classical brown adipose tissue mrna signature partly overlaps with brite in the supraclavicular region of adult humans. Cell Metab 2013; 17:798-805; PMID:23663743; http://dx.doi.org/10.1016/j.cmet.2013.04.011
- Spiegelman BM, Seale P, Kajimura S. Transcriptional control of brown adipocyte development and physiological function-of mice and men. Genes Dev 2009; 23:788-97; PMID:19339685; http://dx.doi.org/10.1101/gad.1779209
- Kajimura S, Seale P, Spiegelman BM. Transcriptional control of brown fat development. Cell Metab 2010; 11:257-62; PMID:20374957; http://dx.doi.org/10.1016/j.cmet.2010.03.005
- Trajkovski M, Lodish H. MicroRNA networks regulate development of brown adipocytes. Trends Endocrinol Metab 2013; 24:442-50; PMID:23809233; http://dx.doi.org/10.1016/j.tem.2013.05.002
- Sun L, Xie H, Mori MA, Alexander R, Yuan B, Hattangadi SM, Liu Q, Kahn CR, Lodish HF. Mir193b-365 is essential for brown fat differentiation. Nat Cell Biol 2011; 13:958-65; PMID:21743466; http://dx.doi.org/10.1038/ncb2286
- Shinoda K, Luijten IHN, Hasegawa Y, Hong H, Sonne SB, Kim M, Xue R, Chondronikola M, Cypess AM, Tseng YH, et al. Genetic and functional characterization of clonally derived adult human brown adipocytes. Nat Med 2015; 1-8; PMID:25774848; http://dx.doi.org/10.1038/nm.3819
- Zilberfarb V, Piétri-Rouxel F, Jockers R, Krief S, Delouis C, Issad T, Strosberg AD. Human immortalized brown adipocytes express functional beta3-adrenoceptor coupled to lipolysis. J Cell Sci 1997; 110(Pt 7):801-7; PMID:9133667. Available: http://www.ncbi.nlm.nih.gov/pubmed/9133667
- Kazantzis M, Takahashi V, Hinkle J, Kota S, Zilberfarb V, Issad T, Abdelkarim M, Chouchane L, Strosberg AD. PAZ6 cells constitute a representative model for human brown pre-adipocytes. Front Endocrinol (Lausanne) 2012; 3:13; PMID:22649407; http://dx.doi.org/10.3389/fendo.2012.00013
- Muoio DM, Noland RC, Kovalik JP, Seiler SE, Davies MN, Debalsi KL, Ilkayeva OR, Stevens RD, Kheterpal I, Zhang J, et al. Muscle-specific deletion of carnitine acetyltransferase compromises glucose tolerance and metabolic flexibility. Cell Metab 2012; 15:764-77; PMID:22560225; http://dx.doi.org/10.1016/j.cmet.2012.04.005
- Koves TR, Ussher JR, Noland RC, Slentz D, Mosedale M, Ilkayeva O, Bain J, Stevens R, Dyck JR, Newgard CB, et al. Mitochondrial overload and incomplete fatty acid oxidation contribute to skeletal muscle insulin resistance. Cell Metab 2008; 7:45-56; PMID:18177724; http://dx.doi.org/10.1016/j.cmet.2007.10.013
- Yehuda-Shnaidman E, Buehrer B, Pi J, Kumar N, Collins S. Acute stimulation of white adipocyte respiration by PKA-induced lipolysis. Diabetes 2010; 59:2474-83; PMID:20682684; http://dx.doi.org/10.2337/db10-0245
- Walden TB, Hansen IR, Timmons JA, Cannon B, Nedergaard J. Recruited vs. nonrecruited molecular signatures of brown, “brite,” and white adipose tissues. Am J Physiol Endocrinol Metab 2012; 302:E19-31; PMID:21828341; http://dx.doi.org/10.1152/ajpendo.00249.2011
- Seale P, Kajimura S, Yang W, Chin S, Rohas LM, Uldry M, Tavernier G, Langin D, Spiegelman BM. Transcriptional control of brown fat determination by PRDM16. Cell Metab 2007; 6:38-54; PMID:17618855; http://dx.doi.org/10.1016/j.cmet.2007.06.001
- Ahfeldt T, Schinzel RT, Lee Y-K, Hendrickson D, Kaplan A, Lum DH, Camahort R, Xia F, Shay J, Rhee EP, et al. Programming human pluripotent stem cells into white and brown adipocytes. Nat Cell Biol 2012; 14:209-19; PMID:22246346; http://dx.doi.org/10.1038/ncb2411
- Nishio M, Yoneshiro T, Nakahara M, Suzuki S, Saeki K, Hasegawa M, Kawai Y, Akutsu H, Umezawa A, Yasuda K, et al. Production of functional classical brown adipocytes from human pluripotent stem cells using specific hemopoietin cocktail without gene transfer. Cell Metab 2012; 16:394-406; PMID:22958922; http://dx.doi.org/10.1016/j.cmet.2012.08.001