ABSTRACT
The adipocyte-derived hormone adiponectin mediates beneficial cardiometabolic effects, and hypoadiponectinemia is a biomarker for increased metabolic and cardiovascular risk. Indeed, circulating adiponectin decreases in obesity and insulin-resistance, likely because of impaired production from white adipose tissue (WAT). Conversely, lean states such as caloric restriction (CR) are characterized by hyperadiponectinemia, even without increased adiponectin production from WAT. The reasons underlying this paradox have remained elusive, but our recent research suggests that CR-associated hyperadiponectinemia derives from an unexpected source: bone marrow adipose tissue (MAT). Herein, we elaborate on this surprising discovery, including further discussion of potential mechanisms influencing adiponectin production from MAT; additional evidence both for and against our conclusions; and observations suggesting that the relationship between MAT and adiponectin might extend beyond CR. While many questions remain, the burgeoning study of MAT promises to reveal further key insights into MAT biology, both as a source of adiponectin and beyond.
Introduction
It is now well established that white adipose tissue (WAT) is a major endocrine organ, with white adipocytes secreting numerous hormones, cytokines, lipids, and other molecules to exert diverse local and systemic effects.Citation1 Notable among these diverse endocrine factors, known as adipokines, is the hormone adiponectin. First discovered in 1995, adiponectin is the most abundant adipokine in the circulation, where it exists in distinct multimeric forms including low-molecular-weight (LMW) trimers, middle-molecular-weight (MMW) hexamers, and high-molecular-weight (HMW) complexes including dodecamers and even larger multimers. Over the past 20 years adiponectin has become established as a major topic of biomedical research that, at the time of writing (January 2016), has been featured in over 15,000 published studies. Such interest reflects the diverse biological actions of adiponectin, as well as its utility as a biomarker for increased risk of clinical conditions including insulin resistance, cardiovascular diseases, bone loss, and certain cancers.Citation2
While such extensive study has yielded great insights, many questions remain unsolved. One notable question concerns the so-called adiponectin paradox: despite being produced exclusively by adipose tissue, circulating adiponectin levels decrease in conditions of excess adiposity (i.e. obesity) but are elevated markedly in conditions of extreme leanness, such as during caloric restriction (CR) in animals and in human subjects with anorexia nervosa (AN). In obese, insulin-resistant states, hypoadiponectinemia likely results from decreased expression and secretion of adiponectin transcripts and protein in WAT.Citation3 In contrast, why circulating adiponectin increases in CR and AN has remained poorly understood.
In research published last year we revealed that, during CR, increased circulating adiponectin comes from a previously unrecognized source: bone marrow adipose tissue (MAT).Citation4 Herein we elaborate on these findings, including additional lines of evidence that further support our conclusions, and other data and clinical observations suggesting that the relationship between MAT and circulating adiponectin, both in CR and beyond, may be more complex. While there is much evidence to consider, many key questions remain to be addressed.
Increased adiponectin during CR: WAT's going on?
Four years after adiponectin was identified, Arita and colleagues made the surprising discovery that, despite being produced by adipocytes, circulating adiponectin concentrations correlate inversely with adiposity.Citation5 Thus, states of obesity and insulin resistance are characterized by hypoadiponectinemia. It is now well established that this results not from increased adiponectin clearance,Citation6 but from impaired adiponectin production from WAT.Citation3 Indeed, adiponectin has been studied extensively in the context of obesity and insulin resistance. In contrast, research into hyperadiponectinemia during CR has been more limited. The first reports of this phenomenon emerged in 2003, beginning with the observation that circulating adiponectin is significantly increased in humans with AN.Citation7 This finding coincided with a study from Phil Scherer's group, which revealed that, in lean mice, chronic CR leads to hyperadiponectinemia.Citation8 These initial reports have since been followed by numerous additional studies that, with some exceptions, further demonstrate that circulating adiponectin levels are increased in subjects with AN or during CR in lean animals or humans (). However, while this phenomenon is now well established, the underlying mechanisms are not so clear. Hyperadiponectinemia can result from increased adiponectin production and/or decreased clearance from the circulation, but few studies of AN or CR have investigated these readouts (). Indeed, only one study of AN patients has measured adiponectin transcript expression in WAT, finding this to be decreased with AN.Citation9 Surprisingly, no studies have analyzed the impact of AN on adiponectin half-life in the circulation. Whether AN alters expression or secretion of adiponectin protein in WAT also remains untested, perhaps owing to the difficulty of obtaining sufficient WAT from these extremely lean subjects. Other studies of CR in animals or humans have been similarly limited (), with none assessing secretion and only one analyzing the half-life of adiponectin, which was unaltered by CR.Citation10 Unlike for AN, animal studies of CR have more thoroughly investigated adiponectin expression in WAT; however, the results have been mixed. Thus, several reports suggest that adiponectin transcripts are increased in WAT with CR,Citation10-17 with three of these studies also finding increased expression of adiponectin protein.Citation10-12 However, Wiesenborn et al. found that, despite increased transcript expression, adiponectin protein in WAT was decreased with CR.Citation16 In contrast, studies from 6 other groups, including the Scherer lab and ourselves, find that CR is associated with unaltered or decreased expression of adiponectin mRNA in WAT.Citation4,8,18-21 In our study, adiponectin protein expression was also unaltered.Citation4
Table 1. Studies investigating the relationship between AN or CR, circulating adiponectin, WAT, and MAT.
Table 2. Impact of human lipodystrophies on WAT, MAT, and circulating adiponectin. Data for MAT and WAT phenotypes are based onCitation63,133-138 and/or studies discussed in a previous review.Citation81 Data for adiponectin are based on the references indicated in the right-most column. Circulating adiponectin concentrations (mg/L) from each cohort are shown as (median; range). aCGL1 and CGL2 are grouped together in this row because the study by Haque et al.Citation129 did not distinguish between these two classes of CGL. bIn FPLD, visceral WAT content is normal in some subjectsCitation134 but increased in others,Citation138 while in AGL the extent of WAT loss depends on the subtype of AGLCitation136 – in most subjects, loss of scWAT is severe, while visceral (intra-abdominal) WAT content can be absent, normal, or increased. cData for CGL3 are from only a single subject, and therefore the reproducibility of this observation remains unknown; however, we recently confirmed maintenance of MAT in Cav1-knockout mice.Citation49
Together, these observations paint a murky picture: although CR can lead to increased adiponectin mRNA and protein expression in WAT, it can also promote hyperadiponectinemia even when the expression of adiponectin in WAT is unaltered or even decreased. Moreover, a recent study found that, in humans, changes in circulating adiponectin during CR are not associated with changes in adiponectin secretion from WAT.Citation22 Adiponectin transcript expression in WAT is also unrelated to the changes in circulating adiponectin that occur in response to insulin or thiazolidinedione (TZD) treatment.Citation14,23 Collectively, these findings question the assumption that WAT is the key source of increased circulating adiponectin during CR, and perhaps in other contexts. But if not WAT, then what?
Digging deeper: bone marrow adipose tissue
One limitation to these studies is that their focus has been largely limited to WAT. This, perhaps, is unsurprising: WAT has been featured in over 90,000 published studies (), reflecting the widespread interest in this tissue. Brown adipose tissue (BAT), a distinct type of fat specialized for mediating adaptive thermogenesis, has also been subject to extensive research (), partly because of its promise as a therapeutic target for obesity and associated metabolic diseases.Citation24 In contrast, modern biomedical research has largely ignored MAT (). This is surprising, because adipocytes in bone marrow (BM) were identified over a century ago and MAT accounts for up to 70% of BM volume in healthy humans.Citation25 Moreover, our recent research suggests that healthy adults have over 2 kg of MAT, representing more than 10% of total adipose mass ().Citation4 MAT further increases in diverse clinical conditions, including during CR in animals and in human subjects with AN.Citation26 This is particularly striking given that such catabolic states typically feature WAT loss. Thus, both MAT and circulating adiponectin are elevated during CR and AN. This observation was the foundation for our hypothesis that MAT contributes to hyperadiponectinemia during CR; however, given the relatively limited knowledge of MAT biology, our efforts to address this hypothesis had to begin by focusing on some very basic questions.
Figure 1. MAT is under-studied, despite being a major adipose depot. Numbers of publications featuring WAT, BAT or MAT were determined by searching the PubMed database in October 2015 with the following terms: WAT, “adipose tissue” OR “adipocyte” NOT “brown adipose tissue” NOT “brown adipocyte;” BAT, brown adipose tissue OR brown adipocyte; MAT, “marrow adipose tissue” OR “marrow adipocyte” OR “yellow marrow” OR “yellow bone marrow.” Values for WAT, BAT or MAT as percentage of total adipose mass in lean, healthy humans, are based on previous publications.Citation4,96
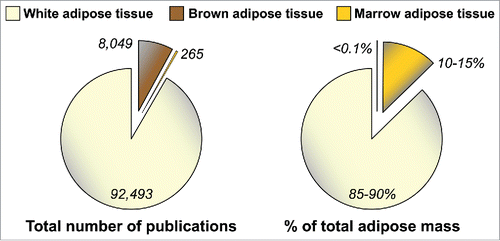
Production of adiponectin by MAT
Adiponectin expression in MAT vs WAT
The first question was whether MAT even expresses adiponectin, and how this compares to expression in WAT. Adiponectin transcript and protein expression has been reported in whole BM of long bones of miceCitation27-31 and in cultured BM adipocytes isolated from human femurs,Citation32 with mRNA expression also noted in adipocytes differentiated in vitro from mouse or human BM stromal cells.Citation33,34 Although such cultured adipocytes may not accurately mimic BM adipocyte characteristics in vivo, at least one report demonstrates that adipocytes within intact human BM express adiponectin mRNA and protein.Citation35 However, none of these previous studies analyzed intact MAT and WAT samples. Therefore, we began by characterizing MAT and WAT obtained from mice, rabbits, and humans.Citation4 In mice, techniques for isolation of intact MAT are yet to be perfected; hence, to study murine MAT we exploited the fact that BM of caudal (tail) vertebrae is essentially all MAT, with very little red, haematopoietic marrow (). We found that such caudal vertebrae express adiponectin protein at levels similar to those in inguinal WAT (iWAT), gonadal WAT (gWAT), and perirenal WAT (pWAT). In contrast, caudal vertebrae have far lower expression of other typical adipocyte markers, including peroxisome proliferator-activated receptor-γ (PPARγ), fatty-acid-binding protein 4 (FABP4), hormone-sensitive lipase (HSL), and perilipin A ().Citation4 Another study also finds that, at the mRNA level, adipocytes isolated from mouse BM express PPARγ (Pparg), FABP4 (Fabp4), and perilipin A (Plin1) at lower levels than adipocytes from WAT ().Citation36 This suggests that, in comparison to WAT, MAT expresses adiponectin transcripts and protein at higher levels than other adipocyte markers. Consistent with this, we found that, in rabbits, tibial MAT expresses adiponectin transcripts and protein at similar levels to iWAT, pWAT, and gWAT, while the expression of other typical adipocyte transcripts (e.g. Cebpa, Fabp4) and proteins (Perilipin A, FABP4) is lower than in each of these WAT depots ().Citation4
Figure 2. Characteristics of MAT in comparison to WAT. Expression or secretion of each factor, relative to WAT, is indicated as follows: greater than WAT, red circle with upward arrow; lower than WAT, green circle with downward arrow; similar to WAT, amber circle with ‘∼’; unknown, gray circle with ‘?’. Where differences refer to mRNA expression, official transcript names are used as follows: Adipoq, adiponectin; Pparg, PPARγ; Fabp4, FABP4; Cebpa, C/EBPα; Lep, leptin; Plin1, Perilipin A. All other differences refer to expression or secretion of proteins. Micrographs for caudal and tibial MAT are H&E-stained sections from mice, rabbits or humans, as indicated. The micrograph of isolated adipocytes is a phase-contrast image of adipocytes from femoral MAT, post-isolation, and is presented for schematic purposes only. Characteristics of caudal and tibial MAT are based on our previously published observations.Citation4 Characteristics of isolated BM adipocytes are based studies of MAT obtained from tibiae/femurs of mice or the iliac crest of humans.Citation36,46 These observations demonstrate that MAT expresses and secretes adiponectin, but many questions remain to be addressed. Abbreviations and other details are given in the main text.
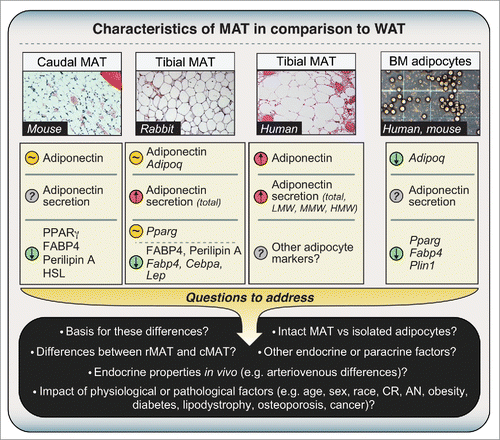
This disparity between adiponectin expression and that of other adipocyte markers is striking, especially considering that the transcription factors PPARγ and CCAAT/enhancer-binding protein-α (C/EBPα) positively regulate adiponectin expression and secretion.Citation37-42 Indeed, Fabp4 is a key transcriptional target of PPARγ; hence, decreased Fabp4 expression in MAT suggests diminished PPARγ activity. Why then would adiponectin be similarly expressed between MAT and WAT? Although the reason is unclear, other studies show that adiponectin expression can be uncoupled from that of other adipocyte genes in certain contexts.Citation39 One possibility is that the different environments of MAT and WAT are responsible for their distinct expression of adipocyte markers. For example, BM adipocytes exist in close proximity to osteoblasts, and the osteoblast-secreted factor osteocalcin stimulates adiponectin mRNA expression in adipocytes.Citation43 Thus, greater local concentrations of osteocalcin in BM might disproportionately increase adiponectin expression in MAT. Finally, differential exposure to other secreted factors that inhibit adiponectin expression and secretion, including glucocorticoids and pro-inflammatory cytokines,Citation38,44,45 might also explain why production of adiponectin is greater in MAT than in WAT.
In addition to analyzing mouse and rabbit samples, we further characterized tibial MAT and subcutaneous WAT (scWAT) from humans. We found that tibial MAT expresses adiponectin protein at higher levels than scWAT, at least in the 3 patients studied.Citation4 Together with our above results in mice and rabbits, our observations suggest that adiponectin transcripts and protein are expressed in MAT at levels similar to or greater than in WAT. However, this is at odds with two recent microarray studies by Liu et al and Poloni et al, in which adipocytes isolated from BM of mice or humans were found to have lower expression of adiponectin mRNA than adipocytes isolated from WAT ().Citation36,46 This discrepancy could relate to the fact that we analyzed intact MAT while Liu et al and Poloni et al studied isolated adipocytes. Given that MAT and WAT do not consist exclusively of adipocytes, it is possible that non-adipocyte populations contribute to adiponectin expression in whole MAT. For example, osteoblasts reportedly express adiponectin transcripts and protein,Citation27,28,47 and our human MAT samples and mouse caudal vertebrae clearly contained some ossified tissue ().Citation4 However, osteoblasts express adiponectin mRNA at only 0.01% of adipocyte levels,Citation47 whereas we found that rabbit tibial MAT expresses adiponectin mRNA at levels similar or greater than WAT, despite containing no trabecular bone.Citation4 Moreover, our observations in mice show that adiponectin protein expression in caudal vertebrae is far greater than that in lumbar vertebrae, despite these tissues having similar bone content.Citation4 Thus, we believe it unlikely that osteoblasts make any meaningful contribution to adiponectin expression in caudal vertebrae of mice or our human MAT samples. A second possibility relates to the fact that Liu et al and Poloni et al isolated adipocytes via collagenase treatment, a method that can alter cellular transcriptional profiles.Citation48 Finally, and perhaps most intriguingly, is the possibility that adiponectin expression in MAT varies across different skeletal sites. Indeed, we recently revealed that properties of BM adipocytes are region-specific, such that MAT can be classified into two broad sub-types: regulated MAT (rMAT) exists in more proximal skeletal sites and consists of adipocytes interspersed with haematopoietic BM, while constitutive MAT (cMAT) exists in more distal regions (e.g., distal tibia, caudal vertebrae) and appears histologically similar to WAT, with few visible haematopoietic cells.Citation49 These MAT subtypes also differ in their lipid composition and response to external stimuli.Citation49 Therefore, it is notable that for our adiponectin studies we analyzed more cMAT-like tissue (i.e., from tails or distal tibiae), whereas Liu et al and Poloni et al studied adipocytes from regions of rMAT (i.e. pooled from femurs/tibiae, or from the iliac crest). We have since begun to investigate adiponectin expression in adipocytes isolated from rMAT, cMAT, and WAT of rodent models, and these studies are ongoing. We have also started to analyze adiponectin protein expression in more rMAT-like samples from human femurs, finding that adiponectin is expressed in these regions; however, unlike in tibial MAT, such expression is not always higher than that in scWAT (). This supports the possibility that adiponectin expression is higher in cMAT than in rMAT. Whether these MAT subtypes differ in adiponectin expression, or indeed other endocrine properties, clearly warrants further study ().
Figure 3. Adiponectin expression in human femoral MAT. Subcutaneous WAT and MAT were isolated from the femoral heads of patients undergoing hip-replacement (Patients 1–3) or from the femoral diaphysis of an amputation patient (Patient 4). (A) Representative micrographs of H&E-stained tissue sections. Scale bar = 200 µm. (B) Total protein was isolated from scWAT and MAT of each patient and expression of the indicated proteins was assessed by immunoblotting; similar results were observed for tissue samples obtained from two other hip-replacement patients (data not shown). Expression of α-tubulin was analyzed as a loading control, although expression was sometimes variable between each tissue type. For patients 1–3, MAT and scWAT lysates were run on non-adjacent lanes of the same gel, and therefore intervening lanes have been removed for ease of comparison. Both the Institutional Review Boards of the University of Michigan and of the Veterans Affairs Hospital of Ann Arbor, MI, approved the study involving hip-replacement patients (IRB number: HUM00053722). The University of Michigan Medical School Institutional Review Boards approved the study involving lower-limb amputation patients (IRB number: HUM00060733). Methods for histology and immunoblotting are as described previously.Citation4
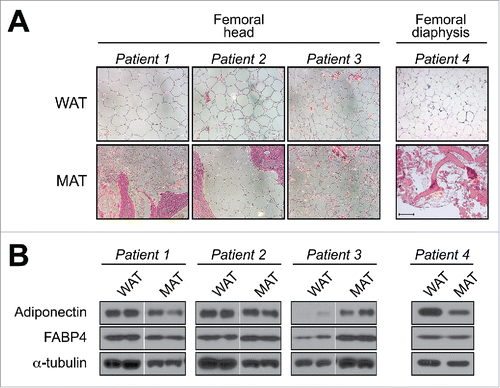
Adiponectin secretion from MAT vs WAT
Despite these inconsistencies, it is clear that BM adipocytes do express adiponectin mRNA and protein; but what about adiponectin secretion? In this case the literature is less revealing. One earlier study noted adiponectin secretion from adipocytes differentiated ex vivo from human BM,Citation50 while two more-recent papers confirm that adiponectin is secreted from primary adipocytes isolated from human femurs.Citation32,51 To build on these observations, we analyzed adiponectin secretion during ex vivo culture of tibial MAT and WAT explants obtained from rabbits or humans.Citation4 In both species, secretion of adiponectin was markedly higher from tibial MAT than from WAT, even after accounting for potential differences in total protein secretion and explant breakdown ().Citation4 For the human samples, further analysis of LMW, MMW, and HMW adiponectin showed that each of these multimeric forms is also secreted more highly from MAT than from WAT (). While this finding is striking, the mechanistic basis remains to be determined. Adiponectin secretion is regulated by numerous factors, including PPARγ, SIRT1, the endoplasmic reticulum chaperones Ero1-Lα and ERp44, the enzyme DsbA-L, and the GTPase regulator FIP1,Citation52-55 as well as the multi-ligand receptor sortilin, which directs adiponectin toward lysosomal degradation;Citation56 hence, altered expression and/or activity of these factors could account for increased adiponectin secretion from cMAT. Another possibility relates to the impact of fatty acids on adiponectin production. Specifically, we recently discovered that cMAT has a lower proportion of saturated fatty acids than rMAT or WAT.Citation49 Given that saturated fatty acids such as palmitate can suppress adiponectin transcript expression and protein secretion,Citation56 decreased exposure to saturated fatty acids might lead to increased expression and secretion of adiponectin from cMAT. Future studies must explore these possibilities and also move beyond explant studies, which can adversely affect adipose tissue biology.Citation57 Alternative approaches, such as analysis of arteriovenous differences in adiponectin concentrations across BM and WAT depots,Citation58 could be one approach to determine the relative production of adiponectin by WAT and MAT in vivo ().
Beyond the basics: MAT as a source of adiponectin during CR
Supporting evidence
Having confirmed that MAT expresses and secretes adiponectin, we next sought to determine if MAT contributes to circulating adiponectin. An ideal tool for addressing this question would be an animal model that lacks MAT but not WAT, or vice versa. Unfortunately, such a model has yet to be firmly established; however, we were extremely fortunate in that our lab had previously developed Ocn-Wnt10b mice, which express the secreted ligand Wnt10b in osteoblasts. Wnt10b promotes osteoblastogenesis, and therefore these mice have increased bone mass.Citation59 Wnt10b also inhibits adipogenesis,Citation60 leading us to speculate that Ocn-Wnt10b mice might also lack MAT. Our initial analyses of proximal tibiae, from the proximal metaphysis to the tibia-fibula junction, showed that the while Ocn-Wnt10b mice tend to have less MAT than their control littermates, this difference does not reach statistical significance.Citation4 However, additional experiments revealed that Ocn-Wnt10b mice significantly resist expansion of this MAT depot during CR.Citation4 We have since extended these analyses to whole tibiae (), revealing that MAT is also decreased in the proximal metaphysis and distal tibia of CR-fed Ocn-Wnt10b mice and that, even without CR, Ocn-Wnt10b mice have significantly less distal tibial MAT than their control littermates (). These new data also highlight that CR-associated MAT expansion occurs predominantly in the proximal tibia rather than the distal tibia. This further supports the designation of these sites as rMAT and cMAT, respectively.
Figure 4. Ocn-Wnt10b mice resist MAT expansion during CR. To determine if Ocn-Wnt10b mice resist MAT formation, we stained tibiae with osmium tetroxide and analyzed MAT volume in situ by micro-CT scanning, as described previously.Citation4 Representative images of osmium-stained tibiae from wild-type mice, fed a control or CR diet, are shown on the left of the figure; osmium-stained MAT appears as darker regions within the bones. MAT volume was then quantified for each of the indicated tibial regions and normalized to total marrow volume to give percentage of MAT volume, as shown in the graph on the right. Data in the graph are presented as mean +/− standard deviation of the following numbers of mice: WT control, n = 6; Wnt10b control, n = 4; WT CR, n = 5; and Wnt10b CR, n = 6. For each diet group, significant differences between WT and Wnt10b mice are indicated by *** (P < 0.001). Within each genotype, significant differences between control and CR diets are indicated by # (P < 0.05) or ### (P <0.001).
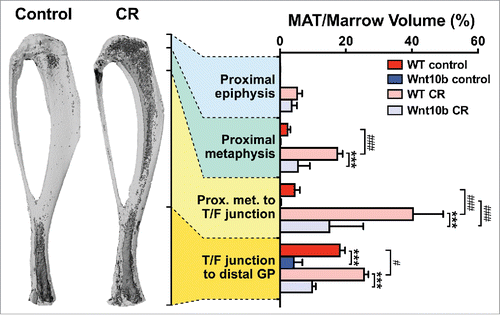
These observations demonstrate that Ocn-Wnt10b mice have moderately decreased MAT volume without CR and robustly resist MAT expansion during CR. What are the consequences for circulating adiponectin? We found no differences in control-fed mice but, strikingly, CR-associated hyperadiponectinemia is significantly blunted in the Ocn-Wnt10b mice.Citation4 Importantly, this occurs despite unaltered expression of adiponectin transcripts or protein in WAT.Citation4 It remains possible that genotype-dependent differences in adiponectin half-life or secretion from WAT contribute to this striking phenotype; however, we found that WAT expression of ERp44 and Dsb-AL, key regulators of adiponectin secretion, is unaltered by diet or genotype.Citation4 Thus, Ocn-Wnt10b mice likely resist CR-associated hyperadiponectinemia because of impaired MAT expansion, rather than altered adiponectin production from WAT.Citation4 Together these observations provide direct evidence that MAT expansion is required for hyperadiponectinemia during CR.
Other recent studies further support this conclusion. For example, Zgheib et al investigated the effects of separation-based anorexia (SBA), a unique model of CR in mice. They find that SBA causes many typical effects of CR, such as weight loss and hypoleptinemia; however, it does not lead to hyperadiponectinemia.Citation21 More recent research from this group shows that MAT expansion also fails to occur during SBA (Christophe Chauveau, personal communication). Similarly, we recently revealed that CR in rabbits leads to decreased body mass, WAT mass, and circulating leptin, but without hyperadiponectinemia or MAT expansion.Citation61 Thus, data from three distinct animal models suggest that MAT expansion is necessary for hyperadiponectinemia during CR, supporting the conclusion that MAT contributes to increased circulating adiponectin in this context.
Functional consequences
Adiponectin acts on numerous target tissues and cell types to exert diverse systemic effects on metabolic homeostasis, vascular function, inflammation, and other systems. What, then, are the consequences of MAT's contribution to hyperadiponectinemia during CR? Our studies in Ocn-Wnt10b mice reveal that, during CR, these mice not only resist hyperadiponectinemia but also have altered adaptations in skeletal muscle, including decreased expression of transcripts related to mitochondrial function and increased activity of AMP-activated protein kinase.Citation4 Beyond skeletal muscle, other responses to CR were similar between Ocn-Wnt10b and control mice, including enhanced glucose tolerance and altered hepatic transcription.Citation4 From this one could infer that the metabolic impact of MAT expansion and/or hyperadiponectinemia during CR is limited to skeletal muscle; however, there are several important caveats. Firstly, there are many other metabolic effects of CR that we did not assess, including altered energy expenditure, substrate utilization, and β-cell function, to name but a few. Secondly, while CR-associated MAT expansion and hyperadiponectinemia are blunted in the Ocn-Wnt10b mice, these effects still occur. Thus, we are currently pursuing additional approaches to determine more comprehensively the consequences of CR-associated MAT expansion.
Additional considerations
Although there is much supporting evidence, it is important to consider any observations that question the contribution of MAT to hyperadiponectinemia during CR. For example, several papers report that CR does increase adiponectin mRNA and/or protein expression in WAT (), suggesting that WAT does contribute to hyperadiponectinemia under these conditions of CR. Surprisingly, one of these studies also finds that CR leads to increased adiponectin expression in skeletal muscle, with adiponectin protein expression being higher in skeletal muscle than in visceral WAT.Citation15 From these surprising observations, the authors propose that skeletal muscle may contribute to hyperadiponectinemia during CR. Finally, in subjects with AN, both MAT and circulating adiponectin inversely correlate with bone mineral density,Citation25 and therefore one might expect BM adiposity to positively associate with circulating adiponectin in AN subjects. However, in our recent analysis of MAT and adiponectin in control and AN subjects, there was no significant association between MAT and circulating adiponectin (P. Fazeli and A. Klibanski, unpublished observations). One limitation is that these MRI-based measurements focused only on vertebral and femoral MAT,Citation4 which might hold less influence on circulating adiponectin than the more cMAT-like adipocytes within tibial MAT (). Moreover, BM adiposity alone might not sufficiently reflect the contribution of MAT to CR-associated hyperadiponectinemia. Indeed, it seems plausible that CR or AN could alter adiponectin production from MAT, thereby impacting circulating levels without alterations in total MAT mass (). However, while such knowledge is limiting in the case of WAT (), for MAT it is entirely lacking. Future research must address these questions, in particular how CR or AN impacts adiponectin secretion from these and other tissues.
Does MAT contribute to circulating adiponectin in other contexts?
Lessons from lipodystrophies
Our studies in Ocn-Wnt10b mice provide the most compelling evidence that MAT contributes to hyperadiponectinemia during CR, because of the unique ability of these mice to resist the formation of MAT but not WAT. Further insights could be gleaned from the converse phenotype, i.e., a lack of WAT but not MAT. Unfortunately, animal models with this phenotype are lacking. However, differential loss of WAT and MAT can occur in human patients with lipodystrophies, acquired or inherited conditions characterized by the impaired formation, progressive loss, or redistribution of adipose tissue.Citation62 Consequently, WAT is either partially or totally absent; however, MAT is sometimes maintained. Therefore, these conditions might provide further insights into the contributions of WAT and MAT to circulating adiponectin. For example, is circulating adiponectin greater in lipodystrophic subjects whose MAT is preserved, compared to those who lack MAT? As shown in , this is sometimes, but not always, the case. Thus, subjects with acquired partial lipodystrophy (APL) have partial loss of visceral and subcutaneous WAT, while MAT is preserved and adiponectin concentrations are normal. A similar situation exists for familial partial lipodystrophy (FPLD), although this can feature increased visceral WAT; slight decreases in circulating adiponectin were also reported in one group of FPLD subjects (). Conversely, in other classes of lipodystrophies both MAT and circulating adiponectin are decreased. These include the partial lipodystrophy associated with antiretroviral therapy for HIV, as well as congenital generalized lipodystrophies (CGL) caused by mutations in AGPAT2 (CGL1) or BSCL2 (CGL2). For CGL4, caused by PTRF mutations, MAT was detected in MRI scans of one patient,Citation63 but for most CGL4 patients MAT content has not been reported. However, we recently revealed that lack of Ptrf in mice is associated with loss of rMAT but not cMAT,Citation49 suggesting partial loss of MAT in CGL4 (). Together, these observations support the possibility that preservation of MAT is necessary if normal circulating adiponectin levels are to be maintained. However, in patients with acquired generalized lipodystrophy (AGL) and in the only known CGL3 subject, circulating adiponectin is decreased despite the presence of MAT (). Thus, the presence of MAT does not guarantee maintenance of normal circulating adiponectin concentrations.
While such observations can be informative, their interpretation is difficult because lipodystrophies are typically associated with metabolic dysregulation, such as insulin resistance and dyslipidemia, which themselves adversely affect circulating adiponectin concentrations. Moreover, analysis of MAT content in lipodsytrophic patients has been extremely limited, while essentially nothing is known about how the underlying clinical defects impact adiponectin production by MAT.
Mouse models of lipodystrophy are similarly limited, but can yield useful insights into the relationship between WAT, MAT, and adiponectin. In one notable study, Colombo et al investigated the impact of WAT transplantation in A-ZIP/F-1 mice, a well-established mouse model of lipodystrophy. In these mice WAT is absent; circulating levels of adiponectin are decreased by 98%; and those of leptin, another adipokine, are over 99% lower than in controls.Citation64 Upon transplanting wild-type scWAT into A-ZIP/F-1 mice, circulating leptin increased to 40% of wild-type concentrations, while circulating adiponectin concentrations reached only 4% of those in wild-type controls.Citation64 The authors state, “The serum levels of adiponectin achieved by WAT transplantation were very low. It is not clear why adipose tissue transplantation to a level ∼25% of wild-type WAT weight and producing nearly wild-type levels of leptin gave adiponectin levels only ∼4% of wild-type.” This suggests that, at least in this context, WAT makes only a minor contribution to circulating adiponectin. Although they would have been unaware at the time, it has since been confirmed that A-ZIP/F-1 mice also lack MAT.Citation25 Might this explain why WAT transplantation alone has only a negligible effect on circulating adiponectin?
Another recently published mouse model may provide further important insights. In an elegant approach, the Scherer lab generated mice in which expression of C/EBPα in mature adipocytes can be inducibly ablated via treatment with doxycycline.Citation42 Development of gWAT is postnatal, whereas that of scWAT occurs during embryogenesis.Citation65 Thus, perinatal treatment of these mice with doxycycline, before substantial gWAT development, leads to loss of C/EBPα in scWAT but not gWAT.Citation42 While this does not affect scWAT mass, it is associated with a 34% decrease in circulating adiponectin concentrations, leading the authors to conclude that scWAT “contributes about one-third of the adiponectin in systemic circulation.” However, it is notable that the MAT phenotype of these mice was not investigated. Indeed, we recently demonstrated that cMAT adipocytes have even greater expression of C/EBPα than adipocytes in scWAT,Citation49 which suggests that cMAT might be even more susceptible than scWAT to C/EBPα deletion. Therefore, in the Scherer lab's unique mouse model it seems highly plausible that C/EBPα ablation would impair adiponectin production from MAT, thereby leading to decreased circulating adiponectin. We eagerly await studies addressing this possibility.
Insulin receptor dysfunction and hyperadiponectinemia
The above studies underscore the potential of monogenic human diseases and transgenic mouse models to clarify our knowledge of MAT's contribution to circulating adiponectin. This applies not only to conditions of decreased circulating adiponectin, but also to states characterized by hyperadiponectinemia. In particular, marked increases in circulating adiponectin occur in patients with insulin receptoropathies caused by insulin receptor antibodies or mutations in the insulin receptor.Citation66,67 Hyperadiponectinemia also occurs in FIRKO mice, which lack the insulin receptor in adipose tissue.Citation68 Thus, insulin receptor dysfunction is associated with increased circulating adiponectin. While the underlying reasons remain unclear, several observations support the possibility that MAT plays a role. For example, insulin directly suppresses adiponectin expression in human MAT,Citation32 suggesting that insulin receptor dysfunction might increase MAT adiponectin production. Moreover, MAT expansion occurs in conditions of hypoinsulinemia, such as CR and type 1 diabetes,Citation25 which suggests that insulin might suppress MAT formation. Thus, there is clear rationale for investigating if MAT is increased in FIRKO mice or in humans with insulin receptoropathies, as this might explain the idiopathic hyperadiponectinemia that occurs in these conditions ().
Figure 5. Potential relationships between MAT and circulating adiponectin in health and disease. Circulating adiponectin is represented as low-molecular-weight (LMW) trimers, middle-molecular-weight (MMW) hexamers, and high-molecular-weight (HMW) dodecamers, although HMW forms may consist of even larger multimers. MAT content varies in ‘normal’ physiological and developmental contexts, with further decreases or increases occurring in adverse or pathological conditions, as indicated. In some cases decreases or increases in MAT are paralleled by similar changes in circulating adiponectin (e.g. decreases in CGL1, CGL2, CGL4; increases in CR, AN), while in other conditions MAT and circulating adiponectin change in opposite directions (e.g., heart failure; obesity). Future studies must address the relative contributions of rMAT and cMAT (represented here by micrographs of rMAT and cMAT from rabbits), as well as how these diverse physiological and clinical conditions impact not just MAT content, but also MAT function.
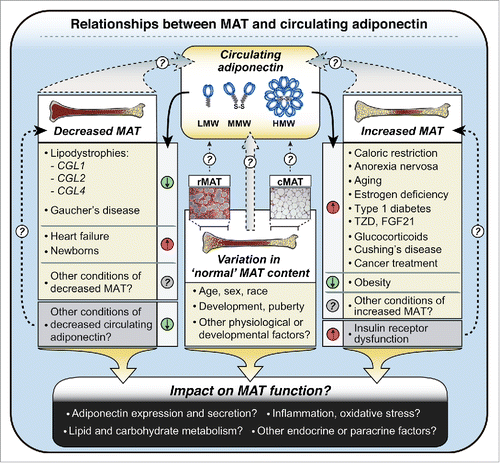
Associations between MAT and adiponectin: not restricted to caloric restriction
Our studies to date have focused on the contribution of MAT and WAT to hyperadiponectinemia during CR. However, increases in both MAT and circulating adiponectin also occur in many other diverse conditions, including aging, estrogen deficiency, type I diabetes, and in response to pharmacological agents such as TZDs, glucocorticoids and fibroblast growth factor-21 (FGF21) ().Citation8,25,36,69-73 Conversely, both MAT and circulating adiponectin are decreased in Gaucher's disease.Citation74-76 This suggests that loss of MAT may lead to hypoadiponectinemia, consistent with our above discussion of lipodystrophies (). Whether these conditions also feature a significant positive correlation between MAT and circulating adiponectin has yet to be established; however, such an association has been noted in patients with Cushing's Disease.Citation77 Finally, our recent work further reveals that both MAT and circulating adiponectin increase in patients undergoing chemotherapy or radiotherapy for cancer.Citation4 Based on these observations, it is tempting to speculate that MAT influences circulating adiponectin levels in states other than CR (). This relationship might also extend beyond adverse clinical conditions. Indeed, ethnic differences in healthy adults have been reported for both circulating adiponectin and BM adiposity, with each of these being higher in Caucasians than in adults of African origin.Citation78,79 Moreover, a positive correlation between MAT volume and serum adiponectin was recently reported in healthy Caucasian girls.Citation80
The above evidence suggests that MAT might contribute to circulating adiponectin beyond CR, both in clinical contexts and in healthy populations (). However, it must be emphasized that, in other conditions, there is discordance between MAT content and circulating adiponectin. For example, heart failure is associated with hyperadiponectinemia, despite MAT loss.Citation81,82 Similarly, circulating adiponectin concentrations in newborn humans are 2- to 3-fold higher than in healthy adults,Citation83 even though MAT is essentially absent in newborns.Citation81 Finally, in adult humans it is well established that circulating adiponectin levels are higher in females than in males;Citation5 however, most studies to date suggest that males have more MAT than females.Citation25 Thus, while increases in MAT are paralleled by increased circulating adiponectin in many conditions, this relationship is not universal ().
MAT and adiponectin in obesity
This discordance is perhaps most notable in the case of obesity and insulin resistance. When we began to study the relationship between MAT and circulating adiponectin it had not yet been established if MAT volume was altered in such adverse metabolic conditions; however, more recent studies report increased MAT in high-fat-diet-fed miceCitation84-86 and in humans with visceral obesity and dyslipidemia or type 2 diabetes.Citation87-89 Unfortunately none of these studies assessed circulating adiponectin, and therefore it remains unclear if this is related to MAT content in such conditions. The clinical studies were also limited to small groups of subjects and therefore await verification in larger cohorts. Nevertheless, these findings raise the question: if MAT truly is a source of adiponectin, then why does circulating adiponectin decrease in obese, insulin-resistant states, when MAT increases?
Although this question might quickly spring to mind, by this logic one would also question if WAT is a source of adiponectin; after all, obesity is defined by excessive WAT accumulation, yet this has not cast doubt on the contribution of WAT to circulating adiponectin. Of course, it is now well established that obesity and insulin resistance lead to WAT dysfunction, including excessive inflammation and oxidative stress, which impairs adiponectin production from WAT. Increasing evidence demonstrates that obesity and insulin resistance also promote oxidative stress and inflammation within BM.Citation90-92 Adipocytes in MAT might be particularly susceptible to such stress, given their relatively high expression of proinflammatory genes.Citation36 Thus, it seems likely that obesity would also lead to adipocyte dysfunction within MAT, thereby compromising production of adiponectin. Determining how obesity impacts MAT function must therefore be a priority of future research ().
Concluding Perspectives
Our research to date provides compelling evidence that MAT contributes to increased circulating adiponectin during CR. The basis of this phenomenon might be further clarified by studies beyond MAT, including more widespread analysis of adiponectin secretion from WAT and its clearance from the circulation, each of which has been largely ignored (). However, given the limited study of MAT (), it is not surprising that many of the key questions are focused on this tissue. For example, to what extent does adiponectin expression and secretion vary between rMAT, cMAT, and WAT, and what are the underlying mechanisms ()? Moreover, are these characteristics affected by CR, obesity, aging, lipodystrophies, or other physiological or pathological conditions in which MAT and/or circulating adiponectin is altered ()? Similarly, to what degree does the relationship between MAT and circulating adiponectin extend to other physiological or pathological conditions ()?
The function of MAT as an endocrine organ could have enormous implications. Our studies in Ocn-Wnt10b mice suggest that MAT expansion contributes to CR-associated adaptations in skeletal muscle,Citation4 demonstrating that MAT can exert systemic effects. However, whether this is through adiponectin or other endocrine factors remains to be determined, as does the full extent of MAT's systemic actions during CR. The endocrine impact of MAT in other contexts, beyond CR, also demands further investigation.
While it remains possible that MAT produces other endocrine factors, thus far we have focused on adiponectin. Here, a major point of interest would be to determine if MAT influences circulating adiponectin in healthy humans. As mentioned above, one study reports a positive relationship between MAT and adiponectin in healthy girls, albeit in a rather limited context.Citation80 Several genome-wide association studies have identified genetic variants that influence circulating adiponectin,Citation93-95 and therefore it would be informative to investigate if these variants also impact MAT formation or function. Indeed, huge insights into MAT biology, beyond its relationship to adiponectin, might be gleaned from more widespread analysis of MAT across larger human populations. Achieving this goal would not be straightforward. For example, determination of MAT content non-invasively relies on MRI, which is expensive and time consuming, making large-scale studies difficult. Even if this were achievable, would such measurements of MAT content alone be sufficient to inform us about the contribution of MAT to circulating adiponectin, or indeed to other physiological or pathological phenomena? As for WAT in obesity, alterations in MAT function, perhaps undetectable by MRI, might have a far greater impact than changes in MAT content alone (). This underscores the need to more directly analyze MAT function though both preclinical and clinical studies, including establishing the global characteristics of MAT in comparison to other adipose depots.
Clearly, there is much work to be done; however, interest in MAT formation and function is growing and research in this field is gathering momentum. The future therefore holds great promise for further expanding our understanding of MAT, not only as a source of adiponectin, but also more broadly in the context of health and disease.
Abbreviations
AGL | = | acquired generalized lipodystrophy |
AN | = | anorexia nervosa |
APL | = | acquired partial lipodystrophy |
BAT | = | brown adipose tissue |
BM | = | bone marrow |
C/EBPα | = | CCAAT/enhancer-binding protein-α; |
CGL | = | congenital generalized lipodystrophy |
cMAT | = | constitutive MAT |
CR | = | caloric restriction |
FABP4 | = | fatty-acid-binding protein 4 |
FGF21, | = | fibroblast growth factor-21 |
FPLD, | = | familial partial lipodystrophy |
HMW | = | High-molecular-weight adiponectin |
HSL | = | Hormone-sensitive lipase |
LMW, | = | Low-molecular-weight adiponectin |
MAT | = | marrow adipose tissue |
MMW | = | Middle-molecular-weight adiponectin |
PPARγ, | = | peroxisome proliferator-activated receptor-γ; |
rMAT | = | regulated MAT |
SBA | = | separation-based anorexia |
TZD | = | thiazolidinedione |
WAT | = | white adipose tissue |
iWAT | = | inguinal WAT |
gWAT | = | gonadal WAT |
pWAT | = | perirenal WAT |
Acknowledgments
We are grateful to Dr. Katherine A Gallagher (Department of Vascular Surgery, University of Michigan Hospital) and Dr. Joshua D. Miller (Department of orthopedic Surgery, University of Michigan Hospital) for providing the WAT and femoral MAT samples characterized in .
Funding
This work was supported by grants from the National Institutes of Health (USA) to E.L.S. (K99-DE024178), A.A.B. (T32-DK101357), and O.A.M. (R24-DK092759). W.P.C. is supported by a Career Development Award (MR/M021394/1) from the Medical Research Council (UK), and by a Chancellor's Fellowship from the University of Edinburgh.
References
- Romacho T, Elsen M, Rohrborn D, Eckel J. Adipose tissue and its role in organ crosstalk. Acta physiologica 2014; 210:733-53; PMID:24495317; http://dx.doi.org/10.1111/apha.12246
- Scherer PEE. Adiponectin: basic and clinical aspects [Special Issue]. Best Pract Res Clin Endocrinol Metab 2014; 28:1-130; PMID:24417940; http://dx.doi.org/10.1016/j.beem.2013.11.004
- Ye R, Scherer PE. Adiponectin, driver or passenger on the road to insulin sensitivity? Mol Metab 2013; 2:133-41; PMID:24049728; http://dx.doi.org/10.1016/j.molmet.2013.04.001
- Cawthorn WP, Scheller EL, Learman BS, Parlee SD, Simon BR, Mori H, Ning X, Bree AJ, Schell B, Broome DT, et al. Bone marrow adipose tissue is an endocrine organ that contributes to increased circulating adiponectin during caloric restriction. Cell Metab 2014; 20:368-75; PMID:24998914; http://dx.doi.org/10.1016/j.cmet.2014.06.003
- Arita Y, Kihara S, Ouchi N, Takahashi M, Maeda K, Miyagawa J, Hotta K, Shimomura I, Nakamura T, Miyaoka K, et al. Paradoxical decrease of an adipose-specific protein, adiponectin, in obesity. Biochem Biophys Res Commun 1999; 257:79-83; PMID:10092513; http://dx.doi.org/10.1006/bbrc.1999.0255
- Halberg N, Schraw TD, Wang ZV, Kim JY, Yi J, Hamilton MP, Luby-Phelps K, Scherer PE. Systemic fate of the adipocyte-derived factor adiponectin. Diabetes 2009; 58:1961-70; PMID:19581422; http://dx.doi.org/10.2337/db08-1750
- Delporte ML, Brichard SM, Hermans MP, Beguin C, Lambert M. Hyperadiponectinaemia in anorexia nervosa. Clin Endocrinol (Oxf) 2003; 58:22-9; PMID:12519408; http://dx.doi.org/10.1046/j.1365-2265.2003.01702.x
- Combs TP, Berg AH, Rajala MW, Klebanov S, Iyengar P, Jimenez-Chillaron JC, Patti ME, Klein SL, Weinstein RS, Scherer PE. Sexual differentiation, pregnancy, calorie restriction, and aging affect the adipocyte-specific secretory protein adiponectin. Diabetes 2003; 52:268-76; PMID:12540596; http://dx.doi.org/10.2337/diabetes.52.2.268
- Dolezalova R, Lacinova Z, Dolinkova M, Kleiblova P, Haluzikova D, Housa D, Papezova H, Haluzik M. Changes of endocrine function of adipose tissue in anorexia nervosa: comparison of circulating levels versus subcutaneous mRNA expression. Clin Endocrinol (Oxf) 2007; 67:674-8; PMID:17953628; http://dx.doi.org/10.1111/j.1365-2265.2007.02944.x
- Qiao L, Lee B, Kinney B, Yoo HS, Shao J. Energy intake and adiponectin gene expression. Am J Physiol Endocrinol Metab 2011; 300:E809-16; PMID:21325106; http://dx.doi.org/10.1152/ajpendo.00004.2011
- Zhu M, Lee GD, Ding L, Hu J, Qiu G, de Cabo R, Bernier M, Ingram DK, Zou S. Adipogenic signaling in rat white adipose tissue: modulation by aging and calorie restriction. Exp Gerontol 2007; 42:733-44; PMID:17624709; http://dx.doi.org/10.1016/j.exger.2007.05.011
- Rohrbach S, Aurich AC, Li L, Niemann B. Age-associated loss in adiponectin-activation by caloric restriction: lack of compensation by enhanced inducibility of adiponectin paralogs CTRP2 and CTRP7. Mol Cell Endocrinol 2007; 277:26-34; PMID:17716811; http://dx.doi.org/10.1016/j.mce.2007.07.005
- Park S, Komatsu T, Hayashi H, Trindade LS, Yamaza H, Chiba T, Shimokawa I. Divergent regulation of adipose tissue metabolism by calorie restriction and inhibition of growth hormone signaling. Exp Gerontol 2009; 44:646-52; PMID:19646410; http://dx.doi.org/10.1016/j.exger.2009.07.002
- Ding Q, Ash C, Mracek T, Merry B, Bing C. Caloric restriction increases adiponectin expression by adipose tissue and prevents the inhibitory effect of insulin on circulating adiponectin in rats. J Nutr Biochem 2012; 23:867-74; PMID:21852089; http://dx.doi.org/10.1016/j.jnutbio.2011.04.011
- Dai Y, Pang J, Gong H, Fan W, Zhang TM. Roles and tissue source of adiponectin involved in lifestyle modifications. J Gerontol A Biol Sci Med Sci 2013; 68:117-28; PMID:22562959; http://dx.doi.org/10.1093/gerona/gls131
- Wiesenborn DS, Menon V, Zhi X, Do A, Gesing A, Wang Z, Bartke A, Altomare DA, Masternak MM. The effect of calorie restriction on insulin signaling in skeletal muscle and adipose tissue of Ames dwarf mice. Aging (Albany NY) 2014; 6:900-12; PMID:25411241
- Chen JH, Ouyang C, Ding Q, Song J, Cao W, Mao L. A Moderate Low-Carbohydrate Low-Calorie Diet Improves Lipid Profile, Insulin Sensitivity and Adiponectin Expression in Rats. Nutrients 2015; 7:4724-38; PMID:26110252; http://dx.doi.org/10.3390/nu7064724
- Higami Y, Pugh TD, Page GP, Allison DB, Prolla TA, Weindruch R. Adipose tissue energy metabolism: altered gene expression profile of mice subjected to long-term caloric restriction. FASEB journal : official publication of the Federation of American Societies for Experimental Biology 2004; 18:415-7; PMID:14688200
- Wang Z, Al-Regaiey KA, Masternak MM, Bartke A. Adipocytokines and lipid levels in Ames dwarf and calorie-restricted mice. The journals of gerontology Series A, Biological sciences and medical sciences 2006; 61:323-31; PMID:16611697; http://dx.doi.org/10.1093/gerona/61.4.323
- Tahmoorespur M, Ghazanfari S, Nobari K. Evaluation of adiponectin gene expression in the abdominal adipose tissue of broiler chickens: feed restriction, dietary energy, and protein influences adiponectin messenger ribonucleic acid expression. Poult Sci 2010; 89:2092-100; PMID:20852099; http://dx.doi.org/10.3382/ps.2010-00772
- Zgheib S, Mequinion M, Lucas S, Leterme D, Ghali O, Tolle V, Zizzari P, Bellefontaine N, Legroux-Gerot I, Hardouin P, et al. Long-term physiological alterations and recovery in a mouse model of separation associated with time-restricted feeding: a tool to study anorexia nervosa related consequences. PLoS One 2014; 9:e103775; PMID:25090643; http://dx.doi.org/10.1371/journal.pone.0103775
- Wang X, You T, Murphy K, Lyles MF, Nicklas BJ. Addition of Exercise Increases Plasma Adiponectin and Release from Adipose Tissue. Med Sci Sports Exerc 2015
- Rasouli N, Yao-Borengasser A, Miles LM, Elbein SC, Kern PA. Increased plasma adiponectin in response to pioglitazone does not result from increased gene expression. Am J Physiol Endocrinol Metab 2006; 290:E42-E6; PMID:16118250; http://dx.doi.org/10.1152/ajpendo.00240.2005
- Rosen ED, Spiegelman BM. What we talk about when we talk about fat. Cell 2014; 156:20-44; PMID:24439368; http://dx.doi.org/10.1016/j.cell.2013.12.012
- Fazeli PK, Horowitz MC, Macdougald OA, Scheller EL, Rodeheffer MS, Rosen CJ, Klibanski A. Marrow fat and bone–new perspectives. J Clin Endocrinol Metab 2013; 98:935-45; PMID:23393168; http://dx.doi.org/10.1210/jc.2012-3634
- Devlin MJ. Why does starvation make bones fat? Am J Hum Biol 2011; 23:577-85; PMID:21793093; http://dx.doi.org/10.1002/ajhb.21202
- Berner HS, Lyngstadaas SP, Spahr A, Monjo M, Thommesen L, Drevon CA, Syversen U, Reseland JE. Adiponectin and its receptors are expressed in bone-forming cells. Bone 2004; 35:842-9; PMID:15454091; http://dx.doi.org/10.1016/j.bone.2004.06.008
- Shinoda Y, Yamaguchi M, Ogata N, Akune T, Kubota N, Yamauchi T, Terauchi Y, Kadowaki T, Takeuchi Y, Fukumoto S, et al. Regulation of bone formation by adiponectin through autocrine/paracrine and endocrine pathways. J Cell Biochem 2006; 99:196-208; PMID:16598753; http://dx.doi.org/10.1002/jcb.20890
- DiMascio L, Voermans C, Uqoezwa M, Duncan A, Lu D, Wu J, Sankar U, Reya T. Identification of adiponectin as a novel hemopoietic stem cell growth factor. J Immunol 2007; 178:3511-20; PMID:17339446; http://dx.doi.org/10.4049/jimmunol.178.6.3511
- Thorrez L, Van Deun K, Tranchevent LC, Van Lommel L, Engelen K, Marchal K, Moreau Y, Van Mechelen I, Schuit F. Using ribosomal protein genes as reference: a tale of caution. PLoS One 2008; 3:e1854; PMID:18365009; http://dx.doi.org/10.1371/journal.pone.0001854
- Lattin JE, Schroder K, Su AI, Walker JR, Zhang J, Wiltshire T, Saijo K, Glass CK, Hume DA, Kellie S, et al. Expression analysis of G Protein-Coupled Receptors in mouse macrophages. Immunome Res 2008; 4:5; PMID:18442421; http://dx.doi.org/10.1186/1745-7580-4-5
- Uchihashi K, Aoki S, Shigematsu M, Kamochi N, Sonoda E, Soejima H, Fukudome K, Sugihara H, Hotokebuchi T, Toda S. Organotypic culture of human bone marrow adipose tissue. Pathol Int 2010; 60:259-67; PMID:20403027; http://dx.doi.org/10.1111/j.1440-1827.2010.02511.x
- Ugarte F, Ryser M, Thieme S, Fierro FA, Navratiel K, Bornhauser M, Brenner S. Notch signaling enhances osteogenic differentiation while inhibiting adipogenesis in primary human bone marrow stromal cells. Exp Hematol 2009; 37:867-75 e1; PMID:19540436; http://dx.doi.org/10.1016/j.exphem.2009.03.007
- Xiao L, Sobue T, Esliger A, Kronenberg MS, Coffin JD, Doetschman T, Hurley MM. Disruption of the Fgf2 gene activates the adipogenic and suppresses the osteogenic program in mesenchymal marrow stromal stem cells. Bone 2010; 47:360-70; PMID:20510392; http://dx.doi.org/10.1016/j.bone.2010.05.021
- Yokota T, Meka CS, Medina KL, Igarashi H, Comp PC, Takahashi M, Nishida M, Oritani K, Miyagawa J, Funahashi T, et al. Paracrine regulation of fat cell formation in bone marrow cultures via adiponectin and prostaglandins. J Clin Invest 2002; 109:1303-10; PMID:12021245; http://dx.doi.org/10.1172/JCI0214506
- Liu LF, Shen WJ, Ueno M, Patel S, Kraemer FB. Characterization of age-related gene expression profiling in bone marrow and epididymal adipocytes. BMC Genomics 2011; 12:212; PMID:21545734; http://dx.doi.org/10.1186/1471-2164-12-212
- Iwaki M, Matsuda M, Maeda N, Funahashi T, Matsuzawa Y, Makishima M, Shimomura I. Induction of adiponectin, a fat-derived antidiabetic and antiatherogenic factor, by nuclear receptors. Diabetes 2003; 52:1655-63; PMID:12829629; http://dx.doi.org/10.2337/diabetes.52.7.1655
- Maeda N, Takahashi M, Funahashi T, Kihara S, Nishizawa H, Kishida K, Nagaretani H, Matsuda M, Komuro R, Ouchi N, et al. PPARgamma ligands increase expression and plasma concentrations of adiponectin, an adipose-derived protein. Diabetes 2001; 50:2094-9; PMID:11522676; http://dx.doi.org/10.2337/diabetes.50.9.2094
- Gustafson B, Jack MM, Cushman SW, Smith U. Adiponectin gene activation by thiazolidinediones requires PPAR gamma 2, but not C/EBP alpha-evidence for differential regulation of the aP2 and adiponectin genes. Biochem Biophys Res Commun 2003; 308:933-9; PMID:12927809; http://dx.doi.org/10.1016/S0006-291X(03)01518-3
- Park B-H, Qiang L, Farmer SR. Phosphorylation of C/EBP{beta} at a consensus extracellular signal-regulated kinase/glycogen synthase kinase 3 site is required for the induction of adiponectin gene expression during the differentiation of Mouse fibroblasts into adipocytes. Mol Cell Biol 2004; 24:8671-80; http://dx.doi.org/10.1128/MCB.24.19.8671-8680.2004
- Qiao L, Maclean PS, Schaack J, Orlicky DJ, Darimont C, Pagliassotti M, Friedman JE, Shao J. C/EBPalpha regulates human adiponectin gene transcription through an intronic enhancer. Diabetes 2005; 54:1744-54; PMID:15919796; http://dx.doi.org/10.2337/diabetes.54.6.1744
- Wang QA, Tao C, Jiang L, Shao M, Ye R, Zhu Y, Gordillo R, Ali A, Lian Y, Holland WL, et al. Distinct regulatory mechanisms governing embryonic versus adult adipocyte maturation. Nat Cell Biol 2015; 17:1099-111; PMID:26280538; http://dx.doi.org/10.1038/ncb3217
- Ferron M, Hinoi E, Karsenty G, Ducy P. Osteocalcin differentially regulates beta cell and adipocyte gene expression and affects the development of metabolic diseases in wild-type mice. Proc Natl Acad Sci U S A 2008; 105:5266-70; PMID:18362359; http://dx.doi.org/10.1073/pnas.0711119105
- Degawa-Yamauchi M, Moss KA, Bovenkerk JE, Shankar SS, Morrison CL, Lelliott CJ, Vidal-Puig A, Jones R, Considine RV. Regulation of adiponectin expression in human adipocytes: effects of adiposity, glucocorticoids, and tumor necrosis factor alpha. Obes Res 2005; 13:662-9; PMID:15897474; http://dx.doi.org/10.1038/oby.2005.74
- Simons PJ, van den Pangaart PS, van Roomen CP, Aerts JM, Boon L. Cytokine-mediated modulation of leptin and adiponectin secretion during in vitro adipogenesis: evidence that tumor necrosis factor-alpha- and interleukin-1beta-treated human preadipocytes are potent leptin producers. Cytokine 2005; 32:94-103; PMID:16213747; http://dx.doi.org/10.1016/j.cyto.2005.08.003
- Poloni A, Maurizi G, Serrani F, Mancini S, Zingaretti MC, Frontini A, Cinti S, Olivieri A, Leoni P. Molecular and functional characterization of human bone marrow adipocytes. Exp Hematol 2013; 41:558-66 e2; PMID:23435314; http://dx.doi.org/10.1016/j.exphem.2013.02.005
- Kajimura D, Lee HW, Riley KJ, Arteaga-Solis E, Ferron M, Zhou B, Clarke CJ, Hannun YA, Depinho RA, Guo EX, et al. Adiponectin regulates bone mass via opposite central and peripheral mechanisms through FoxO1. Cell Metab 2013; PMID:23684624
- Hayman DM, Blumberg TJ, Scott CC, Athanasiou KA. The effects of isolation on chondrocyte gene expression. Tissue Eng 2006; 12:2573-81; PMID:16995790; http://dx.doi.org/10.1089/ten.2006.12.2573
- Scheller EL, Doucette CR, Learman BS, Cawthorn WP, Khandaker S, Schell B, Wu B, Ding SY, Bredella MA, Fazeli PK, et al. Region-specific variation in the properties of skeletal adipocytes reveals regulated and constitutive marrow adipose tissues. Nat Commun 2015; 6:7808; PMID:26245716; http://dx.doi.org/10.1038/ncomms8808
- Ryden M, Dicker A, Gotherstrom C, Astrom G, Tammik C, Arner P, Le Blanc K. Functional characterization of human mesenchymal stem cell-derived adipocytes. Biochem Biophys Res Commun 2003; 311:391-7; PMID:14592427; http://dx.doi.org/10.1016/j.bbrc.2003.10.010
- Hozumi A, Osaki M, Sakamoto K, Goto H, Fukushima T, Baba H, Shindo H. Dexamethasone-induced plasminogen activator inhibitor-1 expression in human primary bone marrow adipocytes. Biomed Res 2010; 31:281-6; PMID:21079357; http://dx.doi.org/10.2220/biomedres.31.281
- Liu M, Zhou L, Xu A, Lam KS, Wetzel MD, Xiang R, Zhang J, Xin X, Dong LQ, Liu F. A disulfide-bond A oxidoreductase-like protein (DsbA-L) regulates adiponectin multimerization. Proc Natl Acad Sci U S A 2008; 105:18302-7; PMID:19011089; http://dx.doi.org/10.1073/pnas.0806341105
- Wang ZV, Schraw TD, Kim JY, Khan T, Rajala MW, Follenzi A, Scherer PE. Secretion of the adipocyte-specific secretory protein adiponectin critically depends on thiol-mediated protein retention. Mol Cell Biol 2007; 27:3716-31; PMID:17353260; http://dx.doi.org/10.1128/MCB.00931-06
- Qiang L, Wang H, Farmer SR. Adiponectin secretion is regulated by SIRT1 and the endoplasmic reticulum oxidoreductase Ero1-L alpha. Mol Cell Biol 2007; 27:4698-707; PMID:17452443; http://dx.doi.org/10.1128/MCB.02279-06
- Carson BP, Del Bas JM, Moreno-Navarrete JM, Fernandez-Real JM, Mora S. The rab11 effector protein FIP1 regulates adiponectin trafficking and secretion. PLoS One 2013; 8:e74687; PMID:24040321; http://dx.doi.org/10.1371/journal.pone.0074687
- Karki S, Chakrabarti P, Huang G, Wang H, Farmer SR, Kandror KV. The multi-level action of fatty acids on adiponectin production by fat cells. PLoS One 2011; 6:e28146; PMID:22140527; http://dx.doi.org/10.1371/journal.pone.0028146
- Gesta S, Lolmede K, Daviaud D, Berlan M, Bouloumie A, Lafontan M, Valet P, Saulnier-Blache JS. Culture of human adipose tissue explants leads to profound alteration of adipocyte gene expression. Horm Metab Res 2003; 35:158-63; PMID:12734776; http://dx.doi.org/10.1055/s-2003-39070
- Frayn KN, Coppack SW. Assessment of white adipose tissue metabolism by measurement of arteriovenous differences. Methods Mol Biol 2001; 155:269-79; PMID:11293078
- Bennett CN, Ouyang H, Ma YL, Zeng Q, Gerin I, Sousa KM, Lane TF, Krishnan V, Hankenson KD, MacDougald OA. Wnt10b increases postnatal bone formation by enhancing osteoblast differentiation. J Bone Miner Res 2007; 22:1924-32; PMID:17708715; http://dx.doi.org/10.1359/jbmr.070810
- Cawthorn WP, Bree AJ, Yao Y, Du B, Hemati N, Martinez-Santibanez G, MacDougald OA. Wnt6, Wnt10a and Wnt10b inhibit adipogenesis and stimulate osteoblastogenesis through a beta-catenin-dependent mechanism. Bone 2012; 50:477-89; PMID:21872687; http://dx.doi.org/10.1016/j.bone.2011.08.010
- Cawthorn WP, Scheller EL, Parlee SD, Pham HA, Learman BS, Redshaw CM, Sulston RJ, Burr AA, Das AK, Simon BR, et al. Expansion of bone marrow adipose tissue during caloric restriction is associated with increased circulating glucocorticoids and not with hypoleptinemia. Endocrinology 2015:en20151477
- Garg A, Agarwal AK. Lipodystrophies: disorders of adipose tissue biology. Biochim Biophys Acta 2009; 1791:507-13; PMID:19162222; http://dx.doi.org/10.1016/j.bbalip.2008.12.014
- Rajab A, Straub V, McCann LJ, Seelow D, Varon R, Barresi R, Schulze A, Lucke B, Lutzkendorf S, Karbasiyan M, et al. Fatal cardiac arrhythmia and long-QT syndrome in a new form of congenital generalized lipodystrophy with muscle rippling (CGL4) due to PTRF-CAVIN mutations. PLoS genetics 2010; 6:e1000874; PMID:20300641; http://dx.doi.org/10.1371/journal.pgen.1000874
- Colombo C, Cutson JJ, Yamauchi T, Vinson C, Kadowaki T, Gavrilova O, Reitman ML. Transplantation of adipose tissue lacking leptin is unable to reverse the metabolic abnormalities associated with lipoatrophy. Diabetes 2002; 51:2727-33; PMID:12196465; http://dx.doi.org/10.2337/diabetes.51.9.2727
- Wang QA, Tao C, Gupta RK, Scherer PE. Tracking adipogenesis during white adipose tissue development, expansion and regeneration. Nat Med 2013; 19:1338-44; PMID:23995282; http://dx.doi.org/10.1038/nm.3324
- Semple RK, Soos MA, Luan J, Mitchell CS, Wilson JC, Gurnell M, Cochran EK, Gorden P, Chatterjee VK, Wareham NJ, et al. Elevated plasma adiponectin in humans with genetically defective insulin receptors. J Clin Endocrinol Metab 2006; 91:3219-23; PMID:16705075; http://dx.doi.org/10.1210/jc.2006-0166
- Semple RK, Halberg NH, Burling K, Soos MA, Schraw T, Luan J, Cochran EK, Dunger DB, Wareham NJ, Scherer PE, et al. Paradoxical elevation of high-molecular weight adiponectin in acquired extreme insulin resistance due to insulin receptor antibodies. Diabetes 2007; 56:1712-7; PMID:17325257; http://dx.doi.org/10.2337/db06-1665
- Bluher M, Michael MD, Peroni OD, Ueki K, Carter N, Kahn BB, Kahn CR. Adipose tissue selective insulin receptor knockout protects against obesity and obesity-related glucose intolerance. Dev Cell 2002; 3:25-38; PMID:12110165; http://dx.doi.org/10.1016/S1534-5807(02)00199-5
- Isobe T, Saitoh S, Takagi S, Takeuchi H, Chiba Y, Katoh N, Shimamoto K. Influence of gender, age and renal function on plasma adiponectin level: the Tanno and Sobetsu study. Eur J Endocrinol 2005; 153:91-8; PMID:15994750; http://dx.doi.org/10.1530/eje.1.01930
- Imagawa A, Funahashi T, Nakamura T, Moriwaki M, Tanaka S, Nishizawa H, Sayama K, Uno S, Iwahashi H, Yamagata K, et al. Elevated serum concentration of adipose-derived factor, adiponectin, in patients with type 1 diabetes. Diabetes Care 2002; 25:1665-6; PMID:12196453; http://dx.doi.org/10.2337/diacare.25.9.1665
- Berg AH, Combs TP, Du X, Brownlee M, Scherer PE. The adipocyte-secreted protein Acrp30 enhances hepatic insulin action. Nat Med 2001; 7:947-53; PMID:11479628; http://dx.doi.org/10.1038/90992
- Kharitonenkov A, Wroblewski VJ, Koester A, Chen YF, Clutinger CK, Tigno XT, Hansen BC, Shanafelt AB, Etgen GJ. The metabolic state of diabetic monkeys is regulated by fibroblast growth factor-21. Endocrinology 2007; 148:774-81; PMID:17068132; http://dx.doi.org/10.1210/en.2006-1168
- Wei W, Dutchak PA, Wang X, Ding X, Wang X, Bookout AL, Goetz R, Mohammadi M, Gerard RD, Dechow PC, et al. Fibroblast growth factor 21 promotes bone loss by potentiating the effects of peroxisome proliferator-activated receptor gamma. Proc Natl Acad Sci U S A 2012; 109:3143-8; PMID:22315431; http://dx.doi.org/10.1073/pnas.1200797109
- Langeveld M, Scheij S, Dubbelhuis P, Hollak CE, Sauerwein HP, Simons P, Aerts JM. Very low serum adiponectin levels in patients with type 1 Gaucher disease without overt hyperglycemia. Metabolism 2007; 56:314-9; PMID:17292718; http://dx.doi.org/10.1016/j.metabol.2006.10.014
- Maas M, Hollak CE, Akkerman EM, Aerts JM, Stoker J, Den Heeten GJ. Quantification of skeletal involvement in adults with type I Gaucher's disease: fat fraction measured by Dixon quantitative chemical shift imaging as a valid parameter. AJR Am J Roentgenol 2002; 179:961-5; PMID:12239046; http://dx.doi.org/10.2214/ajr.179.4.1790961
- Rosenthal DI, Mayo-Smith W, Goodsitt MM, Doppelt S, Mankin HJ. Bone and bone marrow changes in Gaucher disease: evaluation with quantitative CT. Radiology 1989; 170:143-6; PMID:2909087; http://dx.doi.org/10.1148/radiology.170.1.2909087
- Geer EB, Shen W, Strohmayer E, Post KD, Freda PU. Body composition and cardiovascular risk markers after remission of Cushing's disease: a prospective study using whole-body MRI. J Clin Endocrinol Metab 2012; 97:1702-11; PMID:22419708; http://dx.doi.org/10.1210/jc.2011-3123
- Schnitzler CM, Mesquita J. Bone marrow composition and bone microarchitecture and turnover in blacks and whites. J Bone Miner Res 1998; 13:1300-7; PMID:9718199; http://dx.doi.org/10.1359/jbmr.1998.13.8.1300
- Hulver MW, Saleh O, MacDonald KG, Pories WJ, Barakat HA. Ethnic differences in adiponectin levels. Metabolism 2004; 53:1-3; PMID:14681833; http://dx.doi.org/10.1016/j.metabol.2003.07.002
- Newton AL, Hanks LJ, Davis M, Casazza K. The relationships among total body fat, bone mineral content and bone marrow adipose tissue in early-pubertal girls. BoneKEy Rep 2013; 2
- Scheller EL, Rosen CJ. What's the matter with MAT? Marrow adipose tissue, metabolism, and skeletal health. Ann N Y Acad Sci 2014; 1311:14-30; PMID:24650218; http://dx.doi.org/10.1111/nyas.12327
- George J, Patal S, Wexler D, Sharabi Y, Peleg E, Kamari Y, Grossman E, Sheps D, Keren G, Roth A. Circulating adiponectin concentrations in patients with congestive heart failure. Heart 2006; 92:1420-4; PMID:16621874; http://dx.doi.org/10.1136/hrt.2005.083345
- Mantzoros C, Petridou E, Alexe DM, Skalkidou A, Dessypris N, Papathoma E, Salvanos H, Shetty G, Gavrila A, Kedikoglou S, et al. Serum adiponectin concentrations in relation to maternal and perinatal characteristics in newborns. Eur J Endocrinol 2004; 151:741-6; PMID:15588241; http://dx.doi.org/10.1530/eje.0.1510741
- Styner M, Thompson WR, Galior K, Uzer G, Wu X, Kadari S, Case N, Xie Z, Sen B, Romaine A, et al. Bone marrow fat accumulation accelerated by high fat diet is suppressed by exercise. Bone 2014; 64:39-46; PMID:24709686; http://dx.doi.org/10.1016/j.bone.2014.03.044
- Doucette CR, Horowitz MC, Berry R, MacDougald OA, Anunciado-Koza R, Koza RA, Rosen CJ. A High Fat Diet Increases Bone Marrow Adipose Tissue (MAT) But Does Not Alter Trabecular or Cortical Bone Mass in C57BL/6J Mice. J Cell Physiol 2015; 230:2032-7; PMID:25663195; http://dx.doi.org/10.1002/jcp.24954
- Lecka-Czernik B, Stechschulte LA, Czernik PJ, Dowling AR. High bone mass in adult mice with diet-induced obesity results from a combination of initial increase in bone mass followed by attenuation in bone formation; implications for high bone mass and decreased bone quality in obesity. Mol Cell Endocrinol 2015; 410:35-41; PMID:25576855; http://dx.doi.org/10.1016/j.mce.2015.01.001
- Baum T, Yap SP, Karampinos DC, Nardo L, Kuo D, Burghardt AJ, Masharani UB, Schwartz AV, Li X, Link TM. Does vertebral bone marrow fat content correlate with abdominal adipose tissue, lumbar spine bone mineral density, and blood biomarkers in women with type 2 diabetes mellitus? J Magnetic Resonance Imaging: JMRI 2012; 35:117-24; PMID:22190287; http://dx.doi.org/10.1002/jmri.22757
- Bredella MA, Gill CM, Gerweck AV, Landa MG, Kumar V, Daley SM, Torriani M, Miller KK. Ectopic and serum lipid levels are positively associated with Bone Marrow fat in obesity. Radiology 2013; PMID:23861502
- Schafer AL, Li X, Schwartz AV, Tufts LS, Wheeler AL, Grunfeld C, Stewart L, Rogers SJ, Carter JT, Posselt AM, et al. Changes in vertebral bone marrow fat and bone mass after gastric bypass surgery: A pilot study. Bone 2015; 74:140-5; PMID:25603463; http://dx.doi.org/10.1016/j.bone.2015.01.010
- Halade GV, El Jamali A, Williams PJ, Fajardo RJ, Fernandes G. Obesity-mediated inflammatory microenvironment stimulates osteoclastogenesis and bone loss in mice. Exp Gerontol 2011; 46:43-52; PMID:20923699; http://dx.doi.org/10.1016/j.exger.2010.09.014
- Mangialardi G, Spinetti G, Reni C, Madeddu P. Reactive oxygen species adversely impacts bone marrow microenvironment in diabetes. Antioxidants Redox Signaling 2014; 21:1620-33; PMID:25089632; http://dx.doi.org/10.1089/ars.2014.5944
- Gallagher KA, Joshi A, Carson WF, Schaller M, Allen R, Mukerjee S, Kittan N, Feldman EL, Henke PK, Hogaboam C, et al. Epigenetic changes in bone marrow progenitor cells influence the inflammatory phenotype and alter wound healing in type 2 diabetes. Diabetes 2015; 64:1420-30; PMID:25368099; http://dx.doi.org/10.2337/db14-0872
- Richards JB, Waterworth D, O'Rahilly S, Hivert MF, Loos RJ, Perry JR, Tanaka T, Timpson NJ, Semple RK, Soranzo N, et al. A genome-wide association study reveals variants in ARL15 that influence adiponectin levels. PLoS Genet 2009; 5:e1000768; PMID:20011104; http://dx.doi.org/10.1371/journal.pgen.1000768
- Jee SH, Sull JW, Lee JE, Shin C, Park J, Kimm H, Cho EY, Shin ES, Yun JE, Park JW, et al. Adiponectin concentrations: a genome-wide association study. Am J Hum Genet 2010; 87:545-52; PMID:20887962; http://dx.doi.org/10.1016/j.ajhg.2010.09.004
- Dastani Z, Hivert MF, Timpson N, Perry JR, Yuan X, Scott RA, Henneman P, Heid IM, Kizer JR, Lyytikainen LP, et al. Novel loci for adiponectin levels and their influence on type 2 diabetes and metabolic traits: a multi-ethnic meta-analysis of 45,891 individuals. PLoS Genet 2012; 8:e1002607; PMID:22479202; http://dx.doi.org/10.1371/journal.pgen.1002607
- Cypess AM, Lehman S, Williams G, Tal I, Rodman D, Goldfine AB, Kuo FC, Palmer EL, Tseng YH, Doria A, et al. Identification and importance of brown adipose tissue in adult humans. N Engl J Med 2009; 360:1509-17; PMID:19357406; http://dx.doi.org/10.1056/NEJMoa0810780
- Pannacciulli N, Vettor R, Milan G, Granzotto M, Catucci A, Federspil G, De Giacomo P, Giorgino R, De Pergola G. Anorexia nervosa is characterized by increased adiponectin plasma levels and reduced nonoxidative glucose metabolism. J Clin Endocrinol Metab 2003; 88:1748-52; PMID:12679468; http://dx.doi.org/10.1210/jc.2002-021215
- Housova J, Anderlova K, Krizova J, Haluzikova D, Kremen J, Kumstyrova T, Papezova H, Haluzik M. Serum adiponectin and resistin concentrations in patients with restrictive and binge/purge form of anorexia nervosa and bulimia nervosa. J Clin Endocrinol Metab 2005; 90:1366-70; PMID:15598689; http://dx.doi.org/10.1210/jc.2004-1364
- Bosy-Westphal A, Brabant G, Haas V, Onur S, Paul T, Nutzinger D, Klein H, Hauer M, Muller MJ. Determinants of plasma adiponectin levels in patients with anorexia nervosa examined before and after weight gain. Eur J Nutr 2005; 44:355-9; PMID:15793670; http://dx.doi.org/10.1007/s00394-005-0533-3
- Modan-Moses D, Stein D, Pariente C, Yaroslavsky A, Ram A, Faigin M, Loewenthal R, Yissachar E, Hemi R, Kanety H. Modulation of adiponectin and leptin during refeeding of female anorexia nervosa patients. J Clin Endocrinol Metab 2007; 92:1843-7; PMID:17327386; http://dx.doi.org/10.1210/jc.2006-1683
- Dostalova I, Kavalkova P, Haluzikova D, Lacinova Z, Mraz M, Papezova H, Haluzik M. Plasma concentrations of fibroblast growth factors 19 and 21 in patients with anorexia nervosa. J Clin Endocrinol Metab 2008; 93:3627-32; PMID:18559909; http://dx.doi.org/10.1210/jc.2008-0746
- Karczewska-Kupczewska M, Straczkowski M, Adamska A, Nikolajuk A, Otziomek E, Gorska M, Kowalska I. Insulin sensitivity, metabolic flexibility, and serum adiponectin concentration in women with anorexia nervosa. Metabolism 2010; 59:473-7; PMID:19846178; http://dx.doi.org/10.1016/j.metabol.2009.07.036
- Terra X, Auguet T, Aguera Z, Quesada IM, Orellana-Gavalda JM, Aguilar C, Jimenez-Murcia S, Berlanga A, Guiu-Jurado E, Menchon JM, et al. Adipocytokine levels in women with anorexia nervosa. Relationship with weight restoration and disease duration. Int J Eat Disord 2013; 46:855-61; PMID:23881663; http://dx.doi.org/10.1002/eat.22166
- Misra M, Miller KK, Almazan C, Ramaswamy K, Aggarwal A, Herzog DB, Neubauer G, Breu J, Klibanski A. Hormonal and body composition predictors of soluble leptin receptor, leptin, and free leptin index in adolescent girls with anorexia nervosa and controls and relation to insulin sensitivity. J Clin Endocrinol Metab 2004; 89:3486-95; PMID:15240636; http://dx.doi.org/10.1210/jc.2003-032251
- Nogueira JP, Maraninchi M, Lorec AM, Corroller AB, Nicolay A, Gaudart J, Portugal H, Barone R, Vialettes B, Valero R. Specific adipocytokines profiles in patients with hyperactive and/or binge/purge form of anorexia nervosa. Eur J Clin Nutr 2010; 64:840-4; http://dx.doi.org/10.1038/ejcn.2010.66
- Amitani H, Asakawa A, Ogiso K, Nakahara T, Ushikai M, Haruta I, Koyama K, Amitani M, Cheng KC, Inui A. The role of adiponectin multimers in anorexia nervosa. Nutrition 2013; 29:203-6; PMID:23237649; http://dx.doi.org/10.1016/j.nut.2012.07.011
- Tagami T, Satoh N, Usui T, Yamada K, Shimatsu A, Kuzuya H. Adiponectin in anorexia nervosa and bulimia nervosa. J Clin Endocrinol Metab 2004; 89:1833-7; PMID:15070952; http://dx.doi.org/10.1210/jc.2003-031260
- Iwahashi H, Funahashi T, Kurokawa N, Sayama K, Fukuda E, Okita K, Imagawa A, Yamagata K, Shimomura I, Miyagawa JI, et al. Plasma adiponectin levels in women with anorexia nervosa. Horm Metab Res 2003; 35:537-40; PMID:14517770; http://dx.doi.org/10.1055/s-2003-42655
- Bredella MA, Fazeli PK, Daley SM, Miller KK, Rosen CJ, Klibanski A, Torriani M. Marrow fat composition in anorexia nervosa. Bone 2014
- Fazeli PK, Bredella MA, Freedman L, Thomas BJ, Breggia A, Meenaghan E, Rosen CJ, Klibanski A. Marrow fat and preadipocyte factor-1 levels decrease with recovery in women with anorexia nervosa. J Bone Miner Res 2012; PMID:22508185
- Fazeli PK, Bredella MA, Misra M, Meenaghan E, Rosen CJ, Clemmons DR, Breggia A, Miller KK, Klibanski A. Preadipocyte factor-1 is associated with marrow adiposity and bone mineral density in women with anorexia nervosa. J Clin Endocrinol Metab 2010; 95:407-13; PMID:19850693; http://dx.doi.org/10.1210/jc.2009-1152
- Ecklund K, Vajapeyam S, Feldman HA, Buzney CD, Mulkern RV, Kleinman PK, Rosen CJ, Gordon CM. Bone marrow changes in adolescent girls with anorexia nervosa. J Bone Miner Res 2010; 25:298-304; PMID:19653811; http://dx.doi.org/10.1359/jbmr.090805
- Bredella MA, Fazeli PK, Miller KK, Misra M, Torriani M, Thomas BJ, Ghomi RH, Rosen CJ, Klibanski A. Increased bone marrow fat in anorexia nervosa. J Clin Endocrinol Metab 2009; 94:2129-36; PMID:19318450; http://dx.doi.org/10.1210/jc.2008-2532
- Shinmura K, Tamaki K, Saito K, Nakano Y, Tobe T, Bolli R. Cardioprotective effects of short-term caloric restriction are mediated by adiponectin via activation of AMP-activated protein kinase. Circulation 2007; 116:2809-17; PMID:18040027; http://dx.doi.org/10.1161/CIRCULATIONAHA.107.725697
- Wang Z, Masternak MM, Al-Regaiey KA, Bartke A. Adipocytokines and the regulation of lipid metabolism in growth hormone transgenic and calorie-restricted mice. Endocrinology 2007; 148:2845-53; PMID:17347312; http://dx.doi.org/10.1210/en.2006-1313
- Fenton JI, Nunez NP, Yakar S, Perkins SN, Hord NG, Hursting SD. Diet-induced adiposity alters the serum profile of inflammation in C57BL/6N mice as measured by antibody array. Diabetes, obesity & metabolism 2009; 11:343-54; http://dx.doi.org/10.1111/j.1463-1326.2008.00974.x
- Varady KA, Allister CA, Roohk DJ, Hellerstein MK. Improvements in body fat distribution and circulating adiponectin by alternate-day fasting versus calorie restriction. J Nutr Biochem 2010; 21:188-95; PMID:19195863; http://dx.doi.org/10.1016/j.jnutbio.2008.11.001
- Harvey AE, Lashinger LM, Otto G, Nunez NP, Hursting SD. Decreased systemic IGF-1 in response to calorie restriction modulates murine tumor cell growth, nuclear factor-kappaB activation, and inflammation-related gene expression. Mol Carcinog 2013; 52:997-1006; PMID:22778026; http://dx.doi.org/10.1002/mc.21940
- Zhu M, Miura J, Lu LX, Bernier M, DeCabo R, Lane MA, Roth GS, Ingram DK. Circulating adiponectin levels increase in rats on caloric restriction: the potential for insulin sensitization. Exp Gerontol 2004; 39:1049-59; PMID:15236764; http://dx.doi.org/10.1016/j.exger.2004.03.024
- Sharma N, Castorena CM, Cartee GD. Greater insulin sensitivity in calorie restricted rats occurs with unaltered circulating levels of several important myokines and cytokines. Nutr Metab (Lond) 2012; 9:90; PMID:23067400; http://dx.doi.org/10.1186/1743-7075-9-90
- Varady KA, Roohk DJ, Loe YC, McEvoy-Hein BK, Hellerstein MK. Effects of modified alternate-day fasting regimens on adipocyte size, triglyceride metabolism, and plasma adiponectin levels in mice. J Lipid Res 2007; 48:2212-9; PMID:17607017; http://dx.doi.org/10.1194/jlr.M700223-JLR200
- Piccio L, Stark JL, Cross AH. Chronic calorie restriction attenuates experimental autoimmune encephalomyelitis. J Leukoc Biol 2008; 84:940-8; PMID:18678605; http://dx.doi.org/10.1189/jlb.0208133
- Jiang HY, Koike T, Li P, Wang ZH, Kawata Y, Oshida Y. Combined effects of short-term calorie restriction and exercise on insulin action in normal rats. Horm Metab Res 2010; 42:950-4; PMID:20938891; http://dx.doi.org/10.1055/s-0030-1267174
- Fontana L, Klein S, Holloszy JO. Effects of long-term calorie restriction and endurance exercise on glucose tolerance, insulin action, and adipokine production. Age 2010; 32:97-108; PMID:19904628; http://dx.doi.org/10.1007/s11357-009-9118-z
- Villareal DT, Fontana L, Das SK, Redman L, Smith SR, Saltzman E, Bales C, Rochon J, Pieper C, Huang M, et al. Effect of two-year caloric restriction on Bone metabolism and Bone mineral density in non-obese younger adults: a randomized clinical trial. J Bone Miner Res 2015; [Epub ahead of print]; PMID:26332798; http://dx.doi.org/10.1002/jbmr.2701
- Weiss EP, Racette SB, Villareal DT, Fontana L, Steger-May K, Schechtman KB, Klein S, Holloszy JO. Improvements in glucose tolerance and insulin action induced by increasing energy expenditure or decreasing energy intake: a randomized controlled trial. Am J Clin Nutr 2006; 84:1033-42; PMID:17093155
- Wolfe BE, Jimerson DC, Orlova C, Mantzoros CS. Effect of dieting on plasma leptin, soluble leptin receptor, adiponectin and resistin levels in healthy volunteers. Clin Endocrinol (Oxf) 2004; 61:332-8; PMID:15355449; http://dx.doi.org/10.1111/j.1365-2265.2004.02101.x
- Karschin J, Lagerpusch M, Enderle J, Eggeling B, Muller MJ, Bosy-Westphal A. Endocrine determinants of changes in insulin sensitivity and insulin secretion during a weight cycle in healthy men. PLoS One 2015; 10:e0117865; PMID:25723719; http://dx.doi.org/10.1371/journal.pone.0117865
- Haque WA, Shimomura I, Matsuzawa Y, Garg A. Serum adiponectin and leptin levels in patients with lipodystrophies. J Clin Endocrinol Metab 2002; 87:2395; PMID:11994394; http://dx.doi.org/10.1210/jcem.87.5.8624
- Antuna-Puente B, Boutet E, Vigouroux C, Lascols O, Slama L, Caron-Debarle M, Khallouf E, Levy-Marchal C, Capeau J, Bastard JP, et al. Higher adiponectin levels in patients with Berardinelli-Seip congenital lipodystrophy due to seipin as compared with 1-acylglycerol-3-phosphate-o-acyltransferase-2 deficiency. J Clin Endocrinol Metab 2010; 95:1463-8; PMID:20097706; http://dx.doi.org/10.1210/jc.2009-1824
- Shastry S, Delgado MR, Dirik E, Turkmen M, Agarwal AK, Garg A. Congenital generalized lipodystrophy, type 4 (CGL4) associated with myopathy due to novel PTRF mutations. Am J Med Genet Part A 2010; 152A:2245-53; PMID:20684003; http://dx.doi.org/10.1002/ajmg.a.33578
- Dwianingsih EK, Takeshima Y, Itoh K, Yamauchi Y, Awano H, Malueka RG, Nishida A, Ota M, Yagi M, Matsuo M. A Japanese child with asymptomatic elevation of serum creatine kinase shows PTRF-CAVIN mutation matching with congenital generalized lipodystrophy type 4. Mol Genet Metab 2010; 101:233-7; PMID:20638880; http://dx.doi.org/10.1016/j.ymgme.2010.06.016
- Kim CA, Delepine M, Boutet E, El Mourabit H, Le Lay S, Meier M, Nemani M, Bridel E, Leite CC, Bertola DR, et al. Association of a homozygous nonsense caveolin-1 mutation with Berardinelli-Seip congenital lipodystrophy. J Clin Endocrinol Metab 2008; 93:1129-34; PMID:18211975; http://dx.doi.org/10.1210/jc.2007-1328
- Garg A, Peshock RM, Fleckenstein JL. Adipose tissue distribution pattern in patients with familial partial lipodystrophy (Dunnigan variety). J Clin Endocrinol Metab 1999; 84:170-4; PMID:9920078
- Huang JS, Mulkern RV, Grinspoon S. Reduced intravertebral bone marrow fat in HIV-infected men. AIDS 2002; 16:1265-9; PMID:12045492; http://dx.doi.org/10.1097/00002030-200206140-00009
- Misra A, Garg A. Clinical features and metabolic derangements in acquired generalized lipodystrophy: case reports and review of the literature. Medicine (Baltimore) 2003; 82:129-46; PMID:12640189; http://dx.doi.org/10.1097/00005792-200303000-00007
- Koutkia P, Grinspoon S. HIV-associated lipodystrophy: pathogenesis, prognosis, treatment, and controversies. Annu Rev Med 2004; 55:303-17; PMID:14746523; http://dx.doi.org/10.1146/annurev.med.55.091902.104412
- Mory PB, Crispim F, Freire MB, Salles JE, Valerio CM, Godoy-Matos AF, Dib SA, Moises RS. Phenotypic diversity in patients with lipodystrophy associated with LMNA mutations. Eur J Endocrinol 2012; 167:423-31; PMID:22700598; http://dx.doi.org/10.1530/EJE-12-0268