ABSTRACT
Obesity is becoming a global epidemic, predisposing to Type 2 diabetes, cardiovascular disease, fatty liver disease, pulmonary disease, osteoarthritis and cancer. Therefore, understanding the biology of adipocyte expansion in response to overnutrition is critical to devising strategies to treat obesity, and the associated burden of morbidity and mortality. Through exploratory patch-clamp experiments in freshly isolated primary murine and human adipocytes, we recently determined that SWELL1/LRRC8a, a leucine-rich repeat containing transmembrane protein, functionally encoded an ion channel signalling complex (the volume-regulated anion channel, or VRAC) on the adipocyte plasma membrane. The SWELL1-/LRRC8 channel complex activates in response to increases in adipocyte volume and in the context of obesity. SWELL1 is also required for insulin-PI3K-AKT2 signalling to regulate adipocyte growth and systemic glycaemia. This commentary delves further into our working models for the molecular mechanisms of adipocyte SWELL1-mediated VRAC activation, proposed signal transduction mechanisms, and putative impact on adipocyte hypertrophy during caloric excess.
Obesity is a major public health problem, predisposing to high cholesterol, diabetes and hypertension and inflicting health-care costs over 100 billion dollars in the US alone. It is characterized by a tremendous increase in adipose tissue that is in large part due to massive volumetric expansion of the constituent adipocytes [Citation1,Citation2]. This adipocyte expansion has been long associated with metabolic disease and insulin resistance [Citation1,Citation3–Citation6], implicating adipocyte-size dependent regulation of insulin signalling and growth. Studies in cultured adipocytes show that lipid droplet growth increases local tissue stiffness to mechanically-stimulate lipogenesis, and adipocyte growth in neighbouring cells via MEK signalling [Citation7–Citation9], suggesting that obesity may beget obesity in a positive-feedback, feed-forward manner [Citation7]. Indeed, elegant studies in primary, mature, human adipocytes also reveal evidence of adipocyte mechano-signalling in human adipose tissue [Citation10]. Taken together, this literature suggests that, in the setting of obesity, adipocyte expansion may activate an undiscovered mechano-sensitive molecule that modulates adipocyte growth and intracellular signalling. We recently discovered that SWELL1 (LRRC8a), a member of the Leucine Rich Repeat Containing protein family, is a required component of a volume-sensitive and putatively mechano-sensitive ion channel/signalling molecule that is activated in the setting of adipocyte hypertrophy and regulates adipocyte size, insulin signalling and systemic glycaemia via a novel SWELL1-PI3K-AKT2-GLUT4 signalling axis. Adipocyte-specific SWELL1 ablation disrupts insulin-PI3K-AKT2 signalling, inducing insulin resistance and glucose intolerance in vivo [Citation11]. These data identify SWELL1 as a component of a cell-autonomous sensor of adipocyte size and a positive regulator of adipocyte insulin signalling, growth and glucose homeostasis, particularly in the setting of obesity (). Thus, SWELL1 may be a component of the molecular mechano-sensor responsible for the mechano-sensitive nature of adipocytes described by others previously [Citation7–Citation10].
SWELL1/LRRC8a, a macromolecular mechano-sensitive complex?
While it is now established that SWELL1 functionally encodes the volume-regulated anion channel (VRAC) or the swell-activated chloride current (ICl,SWELL) in multiple cell types, such as common cell lines [Citation12,Citation13], adipocytes [Citation11], pancreatic β-cells [Citation14,Citation15], nodose neurons [Citation16], astrocytes [Citation17] and spermatozoa [Citation18], it remains unclear whether SWELL1-mediated VRAC is mechano-sensitive, responsive to osmolarity, ionic strength, or other stimuli [Citation19]. Recent work using purified LRRC8 proteins reconstituted in lipid droplets demonstrates that VRAC channels are only activated by substantial, rather non-physiological reductions in ionic strength, and not mechanical or osmotic stimuli [Citation20]. These findings imply that the physiological function of VRAC is to sense ionic strength. However, there are a number of papers, spanning over a decade, that demonstrate VRAC activation in response to purely mechanical forces, such as pulling on β1-integrins with magnetic microparticles in cardiac myocytes [Citation21,Citation22] and with shear force or positive pressure inflation in endothelial cells [Citation23–Citation26] (for excellent reviews see [Citation19,Citation27]). A key difference in these studies is measuring VRAC activity in an intact cell versus in a minimalist system that is devoid of the requisite interacting proteins necessary for SWELL1 to function within a physiological cellular context. However, this is not to say that VRAC is not, or cannot, be activated by prominent reductions in ionic strength in intact cells as this has also been shown very elegantly in endothelial cells [Citation28]. Zhang et al. (2017) demonstrated that SWELL1-mediated VRAC activates in response to positive pressure ‘inflation’ with pipette solution (with as little as 1 mmHg pressure) after achieving whole-cell configuration [Citation11,Citation29]. Under these conditions, both ionic and osmotic gradients equilibrate within a minute of cell dialysis with pipette solution and remain constant thereafter. The subsequent VRAC activation observed upon mechanical adipocyte swelling must, therefore, occur independent from changes in ionic or osmotic strength. It is notable that whole-cell adipocyte capacitance (which is a measure of total surface area) prior to mechanical swelling is identical to the capacitance measured after swelling. This indicates that the highly caveolated adipocyte plasma membrane (PM), enriched in caveolin-1, is unfolding upon positive pressure swelling (). We surmise that this PM unfolding and caveolae flattening may form part of a mechano-sensitive activation mechanism for SWELL1-mediated VRAC (). In support of this hypothesis, there is prior evidence that caveolae are required for VRAC activation [Citation30–Citation32], that caveolae provide ‘membrane reserves’ to regulate VRAC activation [Citation33], and that caveolae are mechano-sensors that flatten into the plasma membrane in response to mechanical stimuli [Citation34,Citation35]. Moreover, similar to SWELL1, caveolin-1 regulates insulin-PI3K-AKT signalling [Citation36–Citation41] and adipocyte lipid content [Citation42–Citation44]. Indeed, we showed that Cav1 and SWELL1 interact, probably via GRB2, in microdomains in the adipocyte membrane [Citation11]. Curiously, endothelial cells, like adipocytes, are highly enriched in caveolae, and exhibit mechano-activatable VRAC [Citation25], suggesting the presence of a similar SWELL1-GRB2-Cav1 mechano-sensitive macromolecular complex in the endothelium as well. Taken together, these data support the concept that purified LRRC8 channels are not intrinsically mechano-sensitive when reconstituted in a minimalist system. However, in a native cellular environment SWELL1/LRRC8a form macromolecular complexes with interacting proteins (GRB2, Cav1, and possibly cytoskeletal proteins, integrins) that confer mechano-sensitivity [Citation19] and mechano-signalling properties to regulate insulin-AKT2 signalling, growth, and possibly other signalling pathways as well.
Figure 2. SWELL1-mediated VRAC activation by mechanical adipocyte swelling. Mechanical swelling increases adipocyte size without a measurable change in total surface area (as assessed by total cell capacitance) implicating caveolae flattening as a mechanism of VRAC activation in adipocytes. LRR: Leucine Rich Repeat; Cav1: Caveolin-1.

SWELL1/LRRC8a: channel, scaffolding protein, insulin signalling rheostat
Historically, VRAC has been studied for decades using hypotonic swelling as the primary stimulus for VRAC activation and for observing regulatory volume decrease (RVD) [Citation45]. Accordingly, the primary function ascribed to VRAC, for which it was ultimately named, was to regulate cytoplasmic volume in response to hypotonic swelling. However, SWELL1 is broadly expressed, and VRAC also ubiquitously present in cell types that are never exposed to a hypotonic environment, nor to significant drops intracellular ionic strength – i.e. adipocytes, pancreatic β-cells, endothelial cells. Therefore, the true physiological function of the SWELL1 channel complex may have little to do with cellular cytoplasmic volume regulation under physiological conditions in most mammalian cells. The SWELL1 loss-of-function experiments presented in Zhang et al. instead reveal a signalling mechanism in which the SWELL1 complex, via the C-terminal leucine-rich repeat domain (LRRD), regulates insulin-PI3K-AKT2 signalling by titrating the interaction of a negative regulator, GRB2, with the insulin signalling pathway (IRS1). The working model based on these data, is that SWELL1 physically restrains GRB2 from inhibiting AKT2 signalling, in an insulin-dependent manner (). In the absence of SWELL1, GRB2 is free to fully suppress insulin-AKT2 signalling (). Consistent with this model, selective GRB2 ablation can fully rescue insulin-AKT2 signalling in SWELL1 KO adipocytes () [Citation11].
Figure 3. Adipocyte SWELL1/VRAC complex regulates insulin-GRB2-PI3K-AKT2 signalling and senses mechanical forces via caveolae flattening to tune insulin-AKT2 signalling by modulating GRB2-IRS1 interactions. (a) In WT adipocytes, SWELL1 restrains GRB2 from suppression of PI3K-AKT2 signalling. (b) SWELL1 ablation releases GRB2 to fully inhibit insulin-PI3K-AKT2 signalling. (c) GRB2 knock-down (KD) in SWELL1 KO adipocytes fully rescues insulin-PI3K-AKT2 signalling. (d) Working model: adipocyte expansion activates the SWELL1/VRAC complex to disinhibit insulin-PI3K-AKT2 via GRB2, and thereby support lipogenesis and continued adipocyte growth, in a feed-forward manner, potentiating adipocyte hypertrophy.
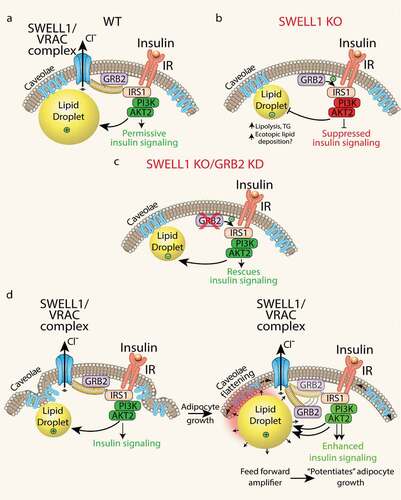
Outstanding questions are whether: 1. SWELL1/LRRC8 complex functions merely as a scaffolding protein for components of the insulin signalling transduction machinery, since LRRD are known to provide surfaces for protein–protein interactions [Citation46–Citation49]; or 2. SWELL1 forms a mechano-sensitive, macromolecular complex that senses cell expansion via conformational changes stimulated by caveolae flattening, etc. (as evidenced by current activation) and then transduce GRB2-dependent modulation of insulin signalling by physically destabilizing the GRB2-IRS1 interaction – thereby ‘tuning’ insulin-AKT2 signalling in response to adipocyte growth. This may establish a feed-forward mechanism to potentiate adipocyte growth during times of nutrient excess (). Although scenario #2 is inferred by Zhang et al., these studies largely focused on SWELL1 loss-of-function experiments in vitro and in vivo. In animal studies, we used high-fat diet models to induce physiological adipocyte expansion and presumably activate SWELL1-mediated signalling. However, these approaches do not allow for tracking SWELL1 signalling in real-time during adipocyte growth, and the hypothesized unfolding of the highly caveolated adipocyte plasma membrane as proposed in our working model () – thus these specific models must be formally tested. Indeed, a prediction of this model is that induction of SWELL1 protein expression, SWELL1-LRRC8 activity and VRAC current is a feature of early, normoglycemic obesity that supports healthy adipocyte expansion and maintains insulin-sensitivity [Citation11,Citation50]. Consequently, reduced SWELL1 activity and associated VRAC currents in the setting of later stage, hyperglycaemic obesity may contribute to insulin resistance and exacerbate impairments in systemic glycaemia, as shown by Xie et al. (2017) [Citation50]. Consistent with this hypothesis, Inoue et al. (2010) [Citation51], the first group to measure VRAC in freshly isolated mature white adipocytes, showed that VRAC is indeed significantly reduced 2–3 fold in the KKAy polygenic Type 2 diabetes model compared to either control KKAa or C57 mice. These data lend further support to the notion that SWELL1 is permissive for insulin-signalling [Citation11,Citation14], potentially bridges adipocyte expansion with the regulation of insulin-AKT2 signalling [Citation7–Citation11], and may become deficient in T2D states to drive diabetes pathogenesis [Citation50,Citation51]. From an overarching cell biology perspective, this model is physiologically relevant in a multitude of cell types and tissues whereby the SWELL1 complex may integrate cellular growth or mechanical force inputs to tune insulin-AKT or mTOR signalling, including endothelium, cardiac muscle, skeletal muscle, and macrophages to name just a few. Finally, a critical unanswered question that arises from the above working model is whether SWELL1-mediated regulation of insulin-AKT2 signalling is mediated purely via SWELL1-dependent GRB2-IRS1 protein–protein interactions or if SWELL1-LRRC8 channel activity (osmolite or chloride conductance) also contributes. Indeed, this is an area of ongoing investigation in the laboratory and has been recently facilitated by a deluge of novel cryo-electron microscopy data of LRRC8a and LRRC8a/c hexamers within the past year [Citation52–Citation55].
In summary, SWELL1 is a component of a novel, broadly expressed, ion channel signalling complex that we hypothesize senses adipocyte growth, putatively via plasma membrane and caveolae unfolding mechanisms to regulate insulin-PI3K-AKT2 signalling and glucose metabolism. Future studies of this fascinating signalling molecule will broaden our mechanistic understanding of its endogenous physiological activation mechanisms, intracellular localization, interaction partners, and regulation of intracellular signalling and systemic energy homeostasis.
Additional information
Funding
References
- Farnier C, Krief S, Blache M, et al. Adipocyte functions are modulated by cell size change: potential involvement of an integrin/ERK signalling pathway. Int J Obes Relat Metab Disord. 2003;27:1178–1186.
- Kim SM, Lun M, Wang M, et al. Loss of white adipose hyperplastic potential is associated with enhanced susceptibility to insulin resistance. Cell Metab. 2014;20:1049–1058.
- Salans LB, Knittle JL, Hirsch J. The role of adipose cell size and adipose tissue insulin sensitivity in the carbohydrate intolerance of human obesity. J Clin Invest. 1968;47:153–165.
- Heinonen S, Saarinen L, Naukkarinen J, et al. Adipocyte morphology and implications for metabolic derangements in acquired obesity. Int J Obes (Lond). 2014.
- Weyer C, Foley JE, Bogardus C, et al. Enlarged subcutaneous abdominal adipocyte size, but not obesity itself, predicts type II diabetes independent of insulin resistance. Diabetologia. 2000;43:1498–1506.
- Lonn M, Mehlig K, Bengtsson C, et al. Adipocyte size predicts incidence of type 2 diabetes in women. FASEB J. 2010;24:326–331.
- Ben-Or Frank M, Shoham N, Benayahu D, et al. Effects of accumulation of lipid droplets on load transfer between and within adipocytes. Biomech Model Mechanobiol. 2014;14(1):15–28.
- Shoham N, Girshovitz P, Katzengold R, et al. Adipocyte stiffness increases with accumulation of lipid droplets. Biophys J. 2014;106:1421–1431.
- Shoham N, Gottlieb R, Sharabani-Yosef O, et al. Static mechanical stretching accelerates lipid production in 3T3-L1 adipocytes by activating the MEK signaling pathway. Am J Physiol Cell Physiol. 2012;302:C429–441.
- Pellegrinelli V, Heuvingh J, Du Roure O, et al. Human adipocyte function is impacted by mechanical cues. J Pathol. 2014;233:183–195.
- Zhang Y, Xie L, Gunasekar SK, et al. SWELL1 is a regulator of adipocyte size, insulin signalling and glucose homeostasis. Nat Cell Biol. 2017;19:504–517.
- Qiu Z, Dubin AE, Mathur J, et al. SWELL1, a plasma membrane protein, is an essential component of volume-regulated anion channel. Cell. 2014;157:447–458.
- Voss FK, Ullrich F, Münch J, et al. Identification of LRRC8 heteromers as an essential component of the volume-regulated anion channel VRAC. Science. 2014;344:634–638.
- Kang C, Xie L, Gunasekar SK, et al. SWELL1 is a glucose sensor regulating beta-cell excitability and systemic glycaemia. Nat Commun. 2018;9:367.
- Stuhlmann T, Planells-Cases R, Jentsch TJ. LRRC8/VRAC anion channels enhance beta-cell glucose sensing and insulin secretion. Nat Commun. 2018;9:1974.
- Wang, R., et al. The volume-regulated anion channel (LRRC8) in nodose neurons is sensitive to acidic pH. JCI Insight. 2017;2:e90632.
- Formaggio F, Saracino E, Mola MG, et al. LRRC8A is essential for swelling-activated chloride current and for regulatory volume decrease in astrocytes. FASEB J. 2019;33:101–113.
- Luck JC, Puchkov D, Ullrich F, et al. LRRC8/VRAC anion channels are required for late stages of spermatid development in mice. J Biol Chem. 2018;293:11796–11808.
- Strange K, Yamada T, Denton JS. A 30-year journey from volume-regulated anion currents to molecular structure of the LRRC8 channel. J Gen Physiol. 2019;151:100–117.
- Syeda R, Qiu Z, Dubin AE, et al. LRRC8 proteins form volume-regulated anion channels that sense ionic strength. Cell. 2016;164:499–511.
- Browe DM, Baumgarten CM. Stretch of beta 1 integrin activates an outwardly rectifying chloride current via FAK and Src in rabbit ventricular myocytes. J Gen Physiol. 2003;122:689–702.
- Browe DM, Baumgarten CM. EGFR kinase regulates volume-sensitive chloride current elicited by integrin stretch via PI-3K and NADPH oxidase in ventricular myocytes. J Gen Physiol. 2006;127:237–251.
- Barakat AI, Leaver EV, Pappone PA, et al. A flow-activated chloride-selective membrane current in vascular endothelial cells. Circ Res. 1999;85:820–828.
- Nakao M, Ono K, Fujisawa S, et al. Mechanical stress-induced Ca2+ entry and Cl- current in cultured human aortic endothelial cells. Am J Physiol. 1999;276:C238–249.
- Nilius B, Droogmans G. Ion channels and their functional role in vascular endothelium. Physiol Rev. 2001;81:1415–1459.
- Romanenko VG, Davies PF, Levitan I. Dual effect of fluid shear stress on volume-regulated anion current in bovine aortic endothelial cells. Am J Physiol Cell Physiol. 2002;282:C708–718.
- Osei-Owusu J, Yang J, Vitery MDC, et al. Molecular biology and physiology of volume-regulated anion channel (VRAC). Curr Top Membr. 2018;81:177–203.
- Voets T, Droogmans G, Raskin G, et al. Reduced intracellular ionic strength as the initial trigger for activation of endothelial volume-regulated anion channels. Proc Natl Acad Sci U S A. 1999;96:5298–5303.
- Zhang Y, Tong D, Mishra A, et al. Isolation and patch-clamp of primary adipocytes. Methods Mol Biol. 2017;1566:145–150.
- Trouet D, Carton I, Hermans D, et al. Inhibition of VRAC by c-Src tyrosine kinase targeted to caveolae is mediated by the Src homology domains. Am J Physiol Cell Physiol. 2001;281:C248–256.
- Trouet D, Hermans D, Droogmans G, et al. Inhibition of volume-regulated anion channels by dominant-negative caveolin-1. Biochem Biophys Res Commun. 2001;284:461–465.
- Trouet D, Nilius B, Jacobs A, et al. Caveolin-1 modulates the activity of the volume-regulated chloride channel. J Physiol. 1999;520(Pt 1):113–119.
- Kozera L, White E, Calaghan S, et al. Caveolae act as membrane reserves which limit mechanosensitive I(Cl,swell) channel activation during swelling in the rat ventricular myocyte. PLoS One. 2009;4:e8312.
- Nassoy P, Lamaze C. Stressing caveolae new role in cell mechanics. Trends Cell Biol. 2012;22:381–389.
- Sinha B, Köster D, Ruez R, et al. Cells respond to mechanical stress by rapid disassembly of caveolae. Cell. 2011;144:402–413.
- Boothe T, Lim GE, Cen H, et al. Inter-domain tagging implicates caveolin-1 in insulin receptor trafficking and Erk signaling bias in pancreatic beta-cells. Mol Metab. 2016;5:366–378.
- Cohen AW, Razani B, Wang XB, et al. Caveolin-1-deficient mice show insulin resistance and defective insulin receptor protein expression in adipose tissue. Am J Physiol Cell Physiol. 2003;285:C222–235.
- Gustavsson J, Parpal S, Karlsson M, et al. Localization of the insulin receptor in caveolae of adipocyte plasma membrane. FASEB J. 1999;13:1961–1971.
- Kimura A, Mora S, Shigematsu S, et al. The insulin receptor catalyzes the tyrosine phosphorylation of caveolin-1. J Biol Chem. 2002;277:30153–30158.
- Sedding DG, Hermsen J, Seay U, et al. Caveolin-1 facilitates mechanosensitive protein kinase B (Akt) signaling in vitro and in vivo. Circ Res. 2005;96:635–642.
- Foti M, Porcheron G, Fournier M, et al. The neck of caveolae is a distinct plasma membrane subdomain that concentrates insulin receptors in 3T3-L1 adipocytes. Proc Natl Acad Sci U S A. 2007;104:1242–1247.
- Briand N, Prado C, Mabilleau G, et al. Caveolin-1 expression and cavin stability regulate caveolae dynamics in adipocyte lipid store fluctuation. Diabetes. 2014;63:4032–4044.
- Cohen AW, Razani B, Schubert W, et al. Role of caveolin-1 in the modulation of lipolysis and lipid droplet formation. Diabetes. 2004;53:1261–1270.
- Pilch PF, Liu L. Fat caves: caveolae, lipid trafficking and lipid metabolism in adipocytes. Trends Endocrinol Metab. 2011;22:318–324.
- Strange K, Emma F, Jackson PS. Cellular and molecular physiology of volume-sensitive anion channels. Am J Physiol. 1996;270:C711–730.
- Abascal F, Zardoya R. LRRC8 proteins share a common ancestor with pannexins, and may form hexameric channels involved in cell-cell communication. Bioessays. 2012;34:551–560.
- de Wit J, Hong W, Luo L, et al. Role of leucine-rich repeat proteins in the development and function of neural circuits. Annu Rev Cell Dev Biol. 2011;27:697–729.
- Kobe B, Deisenhofer J. The leucine-rich repeat: a versatile binding motif. Trends Biochem Sci. 1994;19:415–421.
- Kobe B, Kajava AV. The leucine-rich repeat as a protein recognition motif. Curr Opin Struct Biol. 2001;11:725–732.
- Xie, L., et al. Induction of adipose and hepatic SWELL1 expression is required for maintaining systemic insulin-sensitivity in obesity. Channels. 2017;11(6):673–677.
- Inoue H, Takahashi N, Okada Y, et al. Volume-sensitive outwardly rectifying chloride channel in white adipocytes from normal and diabetic mice. Am J Physiol Cell Physiol. 2010;298:C900–909.
- Deneka D, Sawicka M, Lam AKM, et al. Structure of a volume-regulated anion channel of the LRRC8 family. Nature. 2018.
- Kasuya G, Nakane T, Yokoyama T, et al. Cryo-EM structures of the human volume-regulated anion channel LRRC8. Nat Struct Mol Biol. 2018;25:797–804.
- Kern DM, Oh S, Hite RK, et al. Cryo-EM structures of the DCPIB-inhibited volume-regulated anion channel LRRC8A in lipid nanodiscs. eLife. 2019;8. pii: e42636.
- Kefauver JM, Serra I, Scorciapino MA, et al. Structure of the human volume regulated anion channel. eLife. 2018;7. pii: e38461.