ABSTRACT
Critical-size defects (CSDs) are challenging oral clinical issues that need to be solved. Adipose-derived mesenchymal stem cells (ADSCs) and gene therapy offer a new target to solve these issues. Consequently, ADSCs attract more and more attention because of advantages such as easy obtainability and no ethical concerns. TNF receptor-associated factor 6 (TRAF6) is a significant binding protein both of tumour necrosis factor superfamily and of the toll/interleukin-1 receptor superfamily. Evidence is accumulating that TRAF6 inhibited osteoclast formation and promoted the proliferation of multiple myeloma cell lines and bone resorption. Here, we reported that overexpression of TRAF6 enhanced the proliferation, migration and osteogenesis of ADSCs through Raf-Erk-Merk-Hif1a pathway. Cell sheet of ADSCs combined with TRAF6 accelerated the healing of CSDs. In a word, TRAF6 enhanced osteogenesis, migration and proliferation through Raf-Erk-Merk-Hif1a pathway.
KEYWORDS:
1. Introduction
Bone loss represents a challenging oral clinical issue, especially in large defects such as critical-size defects (CSDs), where surgical intervention is needed because of its lack of self-regeneration [Citation1]. To facilitate CSD healing, regenerative medicine and gene therapy are widely applied nowadays [Citation2].
Human mesenchymal stem cell (hMSC) is a kind of self-renewing multipotent cell derived from different tissues, including bone marrow, adipose tissue, blood, cord, pulp and periodontal ligaments [Citation3,Citation4]. hMSCs could differentiate into any types of cells or organism and be able to self-renew [Citation5,Citation6]. Bone-marrow stromal cells (BMSCs) are obtained from adult bone with a pluripotent differentiation and can usually be used in bone tissue engineering [Citation7]. In contrast to BMSCs, adipose-derived mesenchymal stem cells (ADSCs) have several advantages such as easy obtainability, abundant sources, low technique sensitivity and no ethical issues [Citation8]. However, low osteogenic differentiation is an obstacle to applying ADSCs to bone tissue engineering [Citation9]. Many efforts have been made to improve the osteogenic tendency, including gene therapy [Citation10–12].
Tumor necrosis factor (TNF) receptor-associated factor 6 (TRAF6) is a ubiquitin ligase which is a significant binding protein of both TNF superfamily and toll/IL-1 receptor (TIR) superfamily [Citation13,Citation14]. It was first recognized as the initiation of innate immunity and adaptive immune responses induced by the recognition of different molecular pathogens [Citation14,Citation15]. C-terminal domain and N-terminal domain were special domains of TRAF6 with its functions as an E3 ubiquitin ligase by integrating with various kinases and regulating signalling pathways [Citation16]. TRAF6 acts as the intersection of the activation of the NF-κB signalling pathway and the mitogen-activated protein kinase signalling pathway [Citation17]. Osteoclasts are defective in TRAF6-deficient mice [Citation18]. Interferon-γ-induced TRAF6 degradation also inhibited osteoclast formation [Citation19]. TRAF6 silencing inhibits the proliferation of MM in cell lines and primary cells but inhibits the formation of osteoclasts and bone resorption [Citation20]. However, whether TRAF6 influenced osteogenesis of ADSCs remains unknown.
In our study, we hypothesized that TRAF6 influenced the osteogenesis of ADSCs and bone formation through Raf-Erk-Mek-hif-1a pathway.
2. Results
2.1. Identification of ADSCs
Flow cytometry and multi-lineage potential were used to identify the characteristics of ADSCs. As shown in , the expression of CD29, CD44 and CD90 was positive, while the expression of CD34 and CD45 was negative. Furthermore, it has been confirmed that the cells differentiate osteoblasts () and adipocytes ().
Figure 1. Characteristics of ADSCs. a: The special surface markers including CD29, CD34, CD44, CD45 and CD90 were detected by flow cytometry analysis. b: Osteogenesis was detected by alizarin red stanning. c: Osteogenesis was detected by ALP stanning. d: Adipogenesis was detected by oil red stanning.
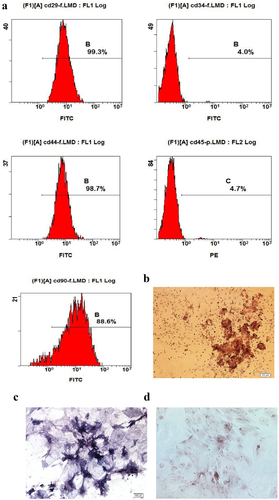
2.2. Concentration of TRAF6 on ADSCs
To detect the effect of TRAF6 on ADSCs, overexpression of TRAF6 was cloned from Sangon Company (Sangon, Shanghai). Immunofluorescence method was used to verify the optimum concentration, and the result showed that 500 ng was an optimum concentration (). Quantitative real-time polymerase chain reaction (qRT-PCR) and Western blot elucidated that TRAF6 successfully transfected into ADSCs and prolonged the RNA () and protein level ().
Figure 2. Verification of concentration of TRAF6 vector. a: 500 ng vector of TRAF6 was detected as an optimum concentration by immunofluorescence. b: Transfection efficacy was detected by qRT-PCR. c: Transfection efficacy was detected by Western blot. A t-test was used to compare p-ex and TRAF6. Data are expressed as mean ± SD referred to the control (*p<0.05) P-EX: blank vector; TRAF6: vector of overexpression of TRAF6.
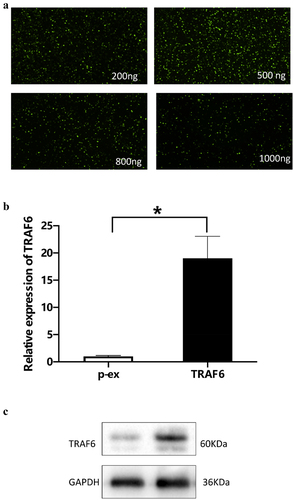
2.3. Effects of TRAF6 on ADSCs
TRAF6 promoted osteogenesis detection by alkaline phosphatase (ALP) and Alizarin red stanning (). CCK-8 results showed that TRAF6 enhanced proliferation on ADSCs after transfecting for 24 h (). As shown in , TRAF6 accelerated the migration through wound healing.
Figure 3. The effects of TRAF6 on proliferation, migration and osteogenesis of ADSCs. a: The osteogenesis detected by ALP. b: The osteogenesis detected by AR. c: The proliferation rate analysed by CCK-8 after transfection for 24 h. d: The migration rate analysed by wound healing. A t-test was used to compare p-ex and TRAF6. Data are expressed as mean ± SD referred to the control (*p<0.05); P-EX: blank vector; TRAF6: vector of overexpression of TRAF6, ALP: alkaline phosphatase, AR: alizarin red.
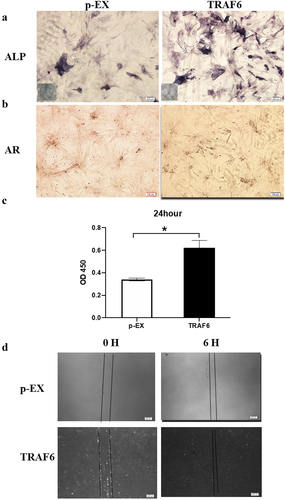
2.4. Mechanisms of TRAF6 on ADSCs
TRAF6 promoted the RNA expression of TRAF6, ALP and Runx2 (); meanwhile, it promoted the protein level of Runx2 and ALP. TRAF6 also overexpressed the protein level of p-Raf, p-Mek, p-Erk and Hif-1a, which means TRAF6 could activate the Raf-Mek-Erk-Hif-1a pathway ().
Figure 4. The mechanism of TRAF6 promoting osteogenic differentiation. a: The mRNA expression level of ALP, RUNX2 and TRAF6 was detected by qRT-PCR. b: The protein expression level of HIF1a, ALP, RUNX2 and TRAF6 was detected by Western blot. A t-test was used to compare p-ex and TRAF6. Data are expressed as mean ± SD referred to the control (*p<0.05) P-EX: blank vector; TRAF6: vector of overexpression of TRAF6.
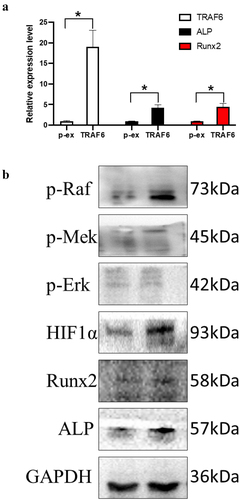
2.5. Osteogenesis effect of TRAF6 in vivo
Bone defect was exhibited and filled with cell membrane after transfecting TRAF6. TRAF6 promoted the healing of bone defect () and bone volume/tissue volume (BV/TV) and bone surface area tissue volume ratio (BS/TV), while inhibited BS/BV ().
Figure 5. The effect of TRAF6 on osteogenesis of ADSCs in vitro. a: The healing of bone defect on ADSCs detected by micro-CT. b: The osteointegration parameters detected by micro-CT. A t-test was used to compare p-ex and TRAF6. Data are expressed as mean ± SD referred to the control (*p<0.05) P-EX: blank vector; TRAF6: vector of overexpression of TRAF6.
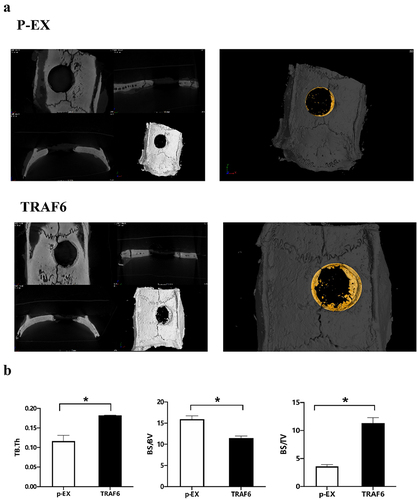
2.6. Histological observations and analysis
Haematoxylin and eosin (HE) stanning showed a higher new bone formation in the TRAF6 overexpression group (). Results of Van Gieson (VG) stanning declared a stained darker and increased in collagen deposition bone tissue in the TRAF6 overexpression group (). Results of Toluidine blue (TB) stanning dedicated that a much new bone formation in the TRAF6 overexpression group ().
Figure 6. The osteogenic effect of TRAF6 was analysed by histomorphology staining. a: The osteogenic effect of TRAF6 was analysed by HE stanning. b: The osteogenic effect of TRAF6 was analysed by VG staining. c: The osteogenic effect of TRAF6 was analysed by TB staining. P-EX: blank vector; TRAF6: vector of overexpression of TRAF6. HE: Hematoxylin and eosin; VG: Van Gieson; TB: Toluidine blue.
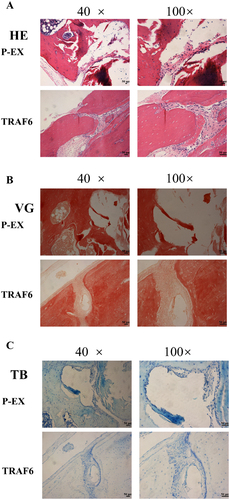
3. Discussion
In our study, we found that overexpression of TRAF6 promoted osteogenesis, proliferation and migration of ADSCs through Raf-Mek-Erk-Hif-1a signal pathway. Meanwhile, TRAF6 induced the new bone formation in a CDS model of rat through ADSC cell sheet.
In fact, CSDs, which are usually resulting from bone tumours, bone diseases and injuries, will not come into being the new bone of the patients or animal models completely. At the same time, new bone formation is a complex process involving mesenchymal stem cells (MSC) and a variety of biological factors [Citation21,Citation22]. Professor Nilveus has developed and described models of critical-size supra-alveolar periodontal defects [Citation23] and subsequent models of critical-size supra-alveolar perialveolar defects [Citation24] for the evaluation of periodontal and alveolar bone regeneration techniques. ADSCs were defined as a potential seed cell for regenerative medicine because of their easy accessibility and no ethics properties [Citation25,Citation26]. Although there are obstacles to improving the osteogenic differentiation of ADSCs, some efforts have been made to improve the osteogenesis of ADSCs. For instance, Zhang et al. found that circRNA-vgll3/miR-326-5p/integrin α5 (Itga5) pathway promoted the osteogenesis of ADSCs [Citation27]. Liu et al. showed a much more osteogenic differentiation tendency of miR-145-5p inhibitors on ADSCs [Citation28]. GLP-1 enhanced osteoblastic mineralization through the higher expression of OCN, Runx2 and collagen I [Citation29]. Receptor activator of NF-kB ligan (RANKL) is the most important cytokine for inducing osteoclast differentiation and affecting its survival, maturation and function [Citation30]. The binding of RANKL to RANK occurs after the recruitment of tTRAFs besides TRAF6 [Citation31]. TRAF6 is a ubiquitin ligase binding both TNF superfamily and TIR superfamily. The vital roles of TRAF6 in bone growth and bone remodelling were determined by osteoclast differentiation and bone resorption pit assays [Citation32,Citation33]. TRAF6 plays a vital role in NF-KB signalling pathway and is an upstream of LPS-induced osteoclastogenesis [Citation34,Citation35]. The bone loss that occurs in periodontitis depends on RANKL produced by osteoblasts and periodontal ligament cells [Citation36]. Meanwhile, some scholars have found that TRAF6 was upregulated during osteoclastogenesis after LPS-inducing osteoclast [Citation35,Citation37]. SiRNA of TRAF6 inhibited the proliferation and promoted the apoptosis on myeloma and multiple myeloma (MM) cells [Citation38]. HIF-1α is a critical factor for bone formation [Citation39]. Osteoblasts stayed on the surface of new bone and sensed a decrease in oxygen levels, and HIF-1α is a vital mediator during this process [Citation40]. As shown in , Erk and Mek are upstream of Hif-1a [Citation41], which is in accordance with our results. Our results showed that TRAF6 promoted the protein level of p-Raf, p-Erk, p-Mek and HIF-1α.
In summary, TRAF6 induced osteogenesis, proliferation and migration but inhibited the adipogenesis in vivo and in vitro by overexpression of Raf-Mek-Erk-Hif-1a pathway. In conclusion, TRAF6 promoted the osteogenic differentiation and proliferation and migration on ADSCs through Raf-Mek-Erk-Hif-1a pathway but inhibited adipogenic differentiation. In vivo, TRAF6 in cell sheet could induce new bone formation. All results indicated that TRAF6 could be an inducible factor for bone defect. Our data also offer a clinical application of ADSC and shed new light on the gene therapy of TRAF6 to accelerate CDS wound healing and attenuate new bone formation.
4. Materials and methods
4.1. Ethics and animals
All animal experiments were supervised and approved by the Animal Ethics Committee of School of Stomatology of the Fourth Military Medical University (kq-011). All procedures were performed according to the ARRIVE guidelines (https://arriveguidelines.org). All methods were in accordance with relevant guidelines including the revised Animals (Scientific Procedures) Act 1986 in the UK and Directive 2010/63/EU in Europe.
4.2. Animal model
Twenty-four Sprague–Dawley male rats (250 g ± 10 g) were selected and adaptive fed in a specific pathogen-free condition, which was 21°–23°C temperature, 30–60% relative humidity and 12 h light/dark in the Experimental Animal Center of the School of Stomatology, the Fourth Military Medical University. All rats were randomly divided into two groups (n = 12). Model of critical-sized calvaria defect was exhibited as previously described [Citation42] with sterile conditions under anaesthesia. Group of p-ex was transfected with blank vectors and group of TRAF6 which was transfectedinto the overexpression vector of TRAF6. After 4 weeks, rats were sacrificed with overdose of anaesthesia and skulls were selected for micro-CT and HE, VG and TB.
4.3. Micro-CT
After implantation for 4 weeks, the new bone in the defective area was reconstructed by the micro-CT system. Using the medium resolution mode, the samples were scanned at a thickness of 0.018 mm of each slice, with 1,024 reconstruction matrix together with a 200 ms integration time. After 3D reconstruction, BMD, BV/TV, Tb.N, Tb.Th and Tb.Sp were automatically determined in order to verify the osteoporotic model, and BMD and BV/TV values in the defect regions were used to evaluate new bone formation by auxiliary software (Scanco Medical, Bassersdorf, Switzerland).
4.4. Histomorphology section and stanning
After operation for 4 weeks, bone tissue was fixed with 4% paraformaldehyde overnight, and then embedded in paraffin, and sect continuously at 4 µm. These slices were stained with HE, VG and TG as previously described.
4.5. Isolation, culture and characterization of ADSCs
ADSCs were isolated from groin fat of young rats as previously described [Citation43]. Adherent cells were cultured in a α-MEM medium with 10% FBS and 1% penicillin–streptomycin. To identify the characteristics of ADSCs, flow cytometry analysis and multi-lineage potential assay were used according to methods previously reported.
4.6. Cell transfection
First, 1 × 106 ADSCs were seeded into a six-well plate. Cells were washed with 1× PBS three times and then transfected with 500 ng p-ex or 500 ng TRAF6 using Lipofectamine 3000. Transfection efficiencies were detected by qRT-PCR and Western blot.
4.7. Cell proliferation assay
1 × 103 ADSCs were seeded into a 96-well plate. Cells were washed with 1× PBS three times and then transfected with 500 ng p-ex or 500 ng TRAF6 using Lipofectamine 3000. Ten microlitres of cck-8 regents was added into the plate and then incubated in an incubator for 30 min after 24 h. OD value of 450 nm was measured by microplate reader.
4.8. Osteogenic induction, ALP and Alizarin assay
Osteogenic induction medium which included 10% foetal bovine serum, 10 μL 10−8 mol/L dexamethasone sodium phosphate, 1 mL 100 U/mL penicillin, 1 mL 100 U/mL streptomycin and 1 mL 0.1 mmol/L L-ascorbic acid phosphate was added into a six-well plate after transfection. After inducing for 7 days, ALP stanning was done as previously described. Alizarin assay was used to detect the calcium deposits after 21 days of induction.
4.9. Adipogenic induction and oil red assay
Adipogenic induction medium which included 10% foetal bovine serum, 1 mL 100 U/mL penicillin and streptomycin, 5 μL 10−8 mol/L dexamethasone sodium phosphate and 250 µl 0.5 mmol/L IBMX was added into a six-well plate after transfection. After inducing for 7 days, oil red stanning was done as previously described.
4.10. Scratch test
A scratch line was made using a 10 µl pipette tip. Then, the pictures were captured by a microscope and the plate was removed from the incubator after transfection. The pictures were captured again by a microscope after transfection for 6 h. The pictures were disposed by ImageJ.
4.11. RNA isolation and quantitative real-time PCR
Cells were washed three times by 1× PBS after transfection and then digested by TRIzol. Five-hundred microlitres of trichloromethane was added and centrifuged at 4°C at 12,000 rpm for 15 min. One-hundred microlitres of isopropanol was added after gently absorbing the middle layer and centrifuged at 4°C at 9000 rpm for 10 min. RNA sedimented at the bottom after being washed by 70% ethyl alcohol at 4°C at 9000 rpm for 5 min, centrifuging twice. Concentration of RNA was qualified by NanoDrop and transcripted into cDNA by PrimeScript Master Mix (Takara). Relative expression of genes was detected using TB Green® Premix Ex TaqTM II (Takara) by 2−ΔΔCt method. Primer sequence is shown in .
Table 1. Sequencing of primers.
4.12. Western blot
Protein was extracted by RIPA (Takara) and quantified with BCA (bicinchoninic acid). Proteins were separated with sodium dodecyl sulphate–polyacrylamide gel and transferred to PVDF membrane (Millipore, USA). After blocking with 5% skimmed milk, the membranes were incubated overnight with each primary antibody including ALP, RUNX2, TRAF6, p-Raf, p-Erk, p-Mel and HIF1α (Abcam, 1:1000 dilution). Relative secondary antibody (Abcam, 1:5000 dilution) was incubated for 2 h. ECL kit (Solarbio, China) was used to develop the target bands, and the GAPDH was a normalization.
4.13. Statistical analysis
GraphPad Prism 7.0 was used for statistical analysis by t-test. Besides, P value < 0.05 is considered statistically significant, and all experiments were repeated at least three times.
Abbreviations
ADSCS, adipose-derived stem cells; CSD, critical-size defects; TRAF6, TNF-receptor-associated factor 6; TNF, tumour necrosis factor; TIR, toll/IL-1 receptor; BV/TV, bone volume/tissue volume; BS/TV, bone surface area tissue volume ratio; HE, hematoxylin and eosin; VG, Van Gieson; TB, toluidine blue
Author contributions
Liuxiangdong carried out all the experiments, collection and analysis of data, and wrote the manuscript. Zhengjiang and Songshuang performed experimental guidance and data analysis. Chenzijun, Wangyuxi and Zhangsijia contributed to collection of ADSCs. Luqn and Songyingliang conceived and designed the study. All authors read and approved the final manuscript.
Supplemental Material
Download MS Word (3.5 MB)Disclosure statement
No potential conflict of interest was reported by the authors.
Data availability statement
The data that support the findings of this study are openly available in Adipocyte at URL.
Supplementary material
Supplemental data for this article can be accessed online at https://doi.org/10.1080/21623945.2023.2193280.
Additional information
Funding
References
- Vajgel A, Mardas N, Farias BC, et al. A systematic review on the critical size defect model. Clin Oral Implants Res. 2014;25(8):879–11.
- Zhang S, Chuah SJ, Lai RC, et al. MSC exosomes mediate cartilage repair by enhancing proliferation, attenuating apoptosis and modulating immune reactivity. Biomaterials. 2018;156:16–27.
- de Melo Pereira D, Eischen-Loges M, Birgani ZT, et al. Proliferation and osteogenic differentiation of hMscs on biomineralized collagen. Front Bioeng Biotechnol. 2020;8:554565.
- Zuk PA, Zhu M, Mizuno H, et al. Multilineage cells from human adipose tissue: implications for cell-based therapies. Tissue Eng. 2001;7(2):211–228.
- Zakrzewski W, Dobrzynski M, Szymonowicz M, et al. Stem cells: past, present, and future. Stem Cell Res Ther. 2019;10(1):68.
- Xue K, Jiang Y, Zhang X, et al. Hypoxic ADSCs-derived EVs promote the proliferation and chondrogenic differentiation of cartilage stem/progenitor cells. Adipocyte. 2021;10(1):322–337.
- Sivaraj KK, Jeong HW, Dharmalingam B, et al. Regional specialization and fate specification of bone stromal cells in skeletal development. Cell Rep. 2021;36(2):109352.
- Harasymiak-Krzyzanowska I, Niedojadlo A, Karwat J, et al. Adipose tissue-derived stem cells show considerable promise for regenerative medicine applications. Cell Mol Biol Lett. 2013;18(4):479–493.
- Rada T, Reis RL, Gomes ME. Adipose tissue-derived stem cells and their application in bone and cartilage tissue engineering. Tissue Eng Part B Rev. 2009;15(2):113–125.
- Xu X, Fang K, Wang L, et al. Local application of semaphorin 3A combined with adipose-derived stem cell sheet and anorganic bovine bone granules enhances bone regeneration in type 2 diabetes mellitus rats. Stem Cells Int. 2019;2019:2506463.
- Li KC, Chang YH, Hsu MN, et al. Baculovirus-mediated miR-214 knockdown shifts osteoporotic ASCs differentiation and improves osteoporotic bone defects repair. Sci Rep. 2017;7(1):16225.
- Lo SC, Li KC, Chang YH, et al. Enhanced critical-size calvarial bone healing by ASCs engineered with Cre/loxP-based hybrid baculovirus. Biomaterials. 2017;124:1–11.
- Dou Y, Tian X, Zhang J, et al. Roles of TRAF6 in central nervous system. Curr Neuropharmacol. 2018;16(9):1306–1313.
- Ishida T, Mizushima S, Azuma S, et al. Identification of TRAF6, a novel tumor necrosis factor receptor-associated factor protein that mediates signaling from an amino-terminal domain of the CD40 cytoplasmic region. J Biol Chem. 1996;271(46):28745–28748. DOI:10.1074/jbc.271.46.28745
- Akira S, Takeda K, Kaisho T. Toll-like receptors: critical proteins linking innate and acquired immunity. Nat Immunol. 2001;2(8):675–680.
- Georg I, Diaz-Barreiro A, Morell M, et al. BANK1 interacts with TRAF6 and MyD88 in innate immune signaling in B cells. Cell Mol Immunol. 2020;17(9):954–965.
- Vallabhapurapu S, Karin M. Regulation and function of NF-kappaB transcription factors in the immune system. Annu Rev Immunol. 2009;27:693–733.
- Naito A, Azuma S, Tanaka S, et al. Severe osteopetrosis, defective interleukin-1 signalling and lymph node organogenesis in TRAF6-deficient mice. Genes Cells. 1999;4(6):353–362. DOI:10.1046/j.1365-2443.1999.00265.x
- Feng X. Ranking intracellular signaling in osteoclasts. IUBMB Life. 2005;57(6):389–395.
- Chen H, Li M, Sanchez E, et al. Combined TRAF6 targeting and proteasome blockade has anti-myeloma and anti-bone resorptive effects. Mol Cancer Res. 2017;15(5):598–609. DOI:10.1158/1541-7786.MCR-16-0293
- Raphael-Mizrahi B, Gabet Y. The cannabinoids effect on bone formation and bone healing. Curr Osteoporos Rep. 2020;18(5):433–438.
- Bartold M, Gronthos S, Haynes D, et al. Mesenchymal stem cells and biologic factors leading to bone formation. J Clin Periodontol. 2019;46(Suppl 21):12–32.
- Wikesjo UM, Nilveus R. Periodontal repair in dogs. Healing patterns in large circumferential periodontal defects. J Clin Periodontol. 1991;18(1):49–59.
- Wikesjo UM, Susin C, Qahash M, et al. The critical-size supraalveolar peri-implant defect model: characteristics and use. J Clin Periodontol. 2006;33(11):846–854.
- Bacakova L, Zarubova J, Travnickova M, et al. Stem cells: their source, potency and use in regenerative therapies with focus on adipose-derived stem cells - a review. Biotechnol Adv. 2018;36(4):1111–1126. DOI:10.1016/j.biotechadv.2018.03.011
- Mazini L, Rochette L, Amine M, et al. Regenerative capacity of Adipose Derived Stem Cells (ADSCs), comparison with Mesenchymal Stem Cells (MSCs). Int J Mol Sci. 2019;20(10):2523.
- Zhang D, Ni N, Wang Y, et al. CircRNA-vgll3 promotes osteogenic differentiation of adipose-derived mesenchymal stem cells via modulating miRNA-dependent integrin alpha5 expression. Cell Death Differ. 2021;28(1):283–302.
- Liu X, Zhu W, Wang L, et al. MiR-145-5p suppresses osteogenic differentiation of adipose-derived stem cells by targeting semaphorin 3A. Vitro Cell Dev Biol Anim. 2019;55(3):189–202.
- Li Y, Fu H, Wang H, et al. GLP-1 promotes osteogenic differentiation of human ADSCs via the Wnt/GSK-3beta/beta-catenin pathway. Mol Cell Endocrinol. 2020;515:110921.
- Theill LE, Boyle WJ, Penninger JM. RANK-L and RANK: t cells, bone loss, and mammalian evolution. Annu Rev Immunol. 2002;20:795–823.
- Walsh MC, Choi Y. Biology of the TRANCE axis. Cytokine Growth Factor Rev. 2003;14(3–4):251–263.
- Wang K, Li S, Gao Y, et al. BCL3 regulates RANKL-induced osteoclastogenesis by interacting with TRAF6 in bone marrow-derived macrophages. Bone. 2018;114:257–267.
- Darnay BG, Besse A, Poblenz AT, et al. Trafs in RANK signaling. Adv Exp Med Biol. 2007;597:152–159.
- Walsh MC, Lee J, Choi Y. Tumor necrosis factor receptor- associated factor 6 (TRAF6) regulation of development, function, and homeostasis of the immune system. Immunol Rev. 2015;266(1):72–92.
- He L, Duan H, Li X, et al. Sinomenine down-regulates TLR4/TRAF6 expression and attenuates lipopolysaccharide-induced osteoclastogenesis and osteolysis. Eur J Pharmacol. 2016;779:66–79.
- Tsukasaki M. RANKL and osteoimmunology in periodontitis. J Bone Miner Metab. 2021;39(1):82–90.
- Ye F, Zhou Q, Tian L, et al. The protective effect of berberine hydrochloride on LPSinduced osteoclastogenesis through inhibiting TRAF6Ca2+calcineurinNFATcl signaling pathway. Mol Med Rep. 2017;16(5):6228–6233.
- Huang H, Sun Z, Wang X, et al. The effect of marrow stromal cells on TRAF6 expression levels in myeloma cells. Oncol Lett. 2017;14(2):1464–1470.
- Merceron C, Ranganathan K, Wang E, et al. Hypoxia-inducible factor 2alpha is a negative regulator of osteoblastogenesis and bone mass accrual. Bone Res. 2019;7:7.
- Xu WN, Zheng HL, Yang RZ, et al. HIF-1alpha regulates glucocorticoid-induced osteoporosis through PDK1/AKT/mTOR signaling pathway. Front Endocrinol. 2019;10:922.
- Zhang Y, Jiang X, Qin X, et al. RKTG inhibits angiogenesis by suppressing MAPK-mediated autocrine VEGF signaling and is downregulated in clear-cell renal cell carcinoma. Oncogene. 2010;29(39):5404–5415. DOI:10.1038/onc.2010.270
- Liang L, Song Y, Li L, et al. Adipose-derived stem cells combined with inorganic bovine bone in calvarial bone healing in rats with type 2 diabetes. J Periodontol. 2014;85(4):601–609. DOI:10.1902/jop.2013.120652
- Wang L, Ding F, Shi S, et al. Hypermethylation in calca promoter inhibited ASC osteogenic differentiation in rats with type 2 diabetic mellitus. Stem Cells Int. 2020;2020:5245294.