ABSTRACT
Blocking antibodies against immunosuppressive molecules have shown promising results in cancer patients. However, there are not enough data to define those conditions dictating treatment efficacy. In this scenario, IL-10 is a cytokine with controversial effects on tumor growth. Thus, our aim was to characterize in which setting IL-10 blockade may potentiate the beneficial effects of a therapeutic vaccine In the IL-10-expressing B16-OVA and TC-1 P3 (A15) tumor models, therapeutic vaccination with tumor antigens plus the TLR7 ligand Imiquimod increased IL-10 production. Although blockade of IL-10 signal with anti-IL-10R antibodies did not inhibit tumor growth, when combined with vaccination it enhanced tumor rejection, associated with stronger innate and adaptive immune responses. Interestingly, a similar enhancement on immune responses was observed after simultaneous vaccination and IL-10 blockade in naive mice. However, when using vaccines containing as adjuvants the TLR3 ligand poly(I:C) or anti-CD40 agonistic antibodies, despite tumor IL-10 expression, anti-IL-10R antibodies did not provide any beneficial effect on tumor growth and antitumor immune responses. Of note, as opposed to Imiquimod, vaccination with this type of adjuvants did not induce IL-10 and correlated with a lack of in vitro IL-10 production by dendritic cells (DC). Finally, in B16-OVA-bearing mice, blockade of IL-10 during therapeutic vaccination with a multiple adjuvant combination (MAC) with potent immunostimulatory properties but still inducing IL-10 led to superior antitumor immunity and complete tumor rejection. These results suggest that for therapeutic antitumor vaccination, blockade of vaccine-induced IL-10 is more relevant than tumor-associated IL-10.
Abbreviations
DC | = | dendritic cells |
APC | = | antigen presenting cells |
MAC | = | multiple adjuvant combination |
Introduction
Immunotherapy based on antibodies blocking immunosuppressive molecules is yielding promising results, although there are still patients who do not respond.Citation1 Similarly, results obtained with antitumor vaccination are far from being optimal.Citation2 Thus, combination of both strategies might provide a higher benefit, as demonstrated in pre-clinical models.Citation3 For these reasons, characterization of those situations amenable to treatment combinations is clearly needed to enhance therapeutic success. Interleukin 10 (IL-10) is an immunosuppressive element considered as potential target for inhibition in immunotherapeutic strategies. IL-10 is a cytokine traditionally considered anti-inflammatory (reviewed in Citation4), with a prominent role on monocytes and DC. It inhibits proinflammatory cytokine production and antigen presentation required for induction of Th1 responses. IL-10 is produced by antigen presenting cells (APC) and by different lymphocyte subsets, including Foxp3+ regulatory T cells, Tr1 cells, Th2 T cells, as well as Th1 cells.Citation5 Under normal circumstances, IL-10 is produced in response to activation stimuli to counteract the potential harmful effects of inflammatory cytokines which may result in immune damage.Citation6 However, in some pathological situations such as chronic viral infectionsCitation7 or tumors (reviewed in Citation8), prolonged IL-10 production leads to immunosupression and the lack of efficient immune responses, thereby allowing microbial persistence or tumor growth. Indeed, in this last case, IL-10 can also be produced by tumor cells, resulting not only in immunosuppression, but also in the activation of an autocrine loop which leads to a higher proliferation of malignant cells.Citation9
Besides this scenario, some therapies, such as vaccines containing immunostimulatory adjuvants, have been shown to induce the production of IL-10 by APC, resulting in diminished Th1 immune responses.Citation10,11 Indeed, although vaccines based on combinations of selected tumor antigens with adjuvants inducing optimal APC activation may lead to proper antitumor T cell priming,Citation12-14 this may be accompanied by activation of regulatory feed-back mechanisms, such as IL-10 production.Citation15 This has led to the hypothesis that combination of these immune-activating strategies with blockade of IL-10 may have a higher immunotherapeutic effect. However, in some tumor models it has been shown that IL-10 has a beneficial effect on antitumor responses through different mechanisms.Citation16-19 Due to the potential different sources of IL-10 in the setting of antitumor therapeutic vaccination as well as the opposite results described for IL-10,Citation20,21 in the present manuscript we analyzed the effect of IL-10 blockade during therapeutic vaccination using different adjuvants and tumor models, as well as the mechanisms responsible for such effect.
Here, we show that IL-10 blockade may have an antitumor effect when combined with therapeutic vaccination, and it mainly depends on adjuvant ability to induce IL-10, whereas no effect is observed in the absence of immunotherapy, even in the case of IL-10- containing-tumors.
Results
Therapeutic vaccination with TLR7 ligand Imiquimod enhances IL-10 production
IL-10 is a cytokine traditionally considered immunosuppressive, which has been found in many patient tumors,Citation8 although it has shown controversial effects on tumor growth. Since in the context of therapeutic vaccination IL-10 can be also induced by the vaccine,Citation11 we analyzed the effect of IL-10 blockade during therapeutic vaccination. We thus used the B16-OVA tumor model, where IL-10 production has been demonstratedCitation9,22-24 and a vaccine consisting in the model antigen OVA plus Imiquimod, a TLR7 ligand used in vaccination protocols in clinical trials.Citation25-28 Imiquimod administration in a murine breast cancer model induced IL-10.Citation29 Thus, we first tested the ability of our Imiquimod-containing vaccine to induce IL-10 in the B16-OVA tumor model. We found that levels of IL-10 in tumor tissue slightly increased 2 d after Imiquimod + OVA administration (), without reaching statistical significance. By contrast, a clear increase in IL-10 concentrations was observed in serum of treated mice (), confirming the ability of this vaccine to induce IL-10.
Figure 1. IL-10 induction after vaccination with tumor antigens + Imiquimod in tumor-bearing mice. C57BL/6 mice (n = 4–6/group) with 5 mm B16-OVA tumors were vaccinated by i.t. injection of OVA plus topical application of Imiquimod. (A) Two days later tumors were obtained, homogenized and IL-10 measured by ELISA. (B) Serum was also obtained at the day of sacrifice and IL-10 was measured, including also samples from control non-tumor bearing mice (naïve). (C) Mice bearing 5 mm TC-1 P3(A15) tumors were immunized with EDA-HPVE7 plus Imiquimod and serum IL-10 measured as in B. Results are representative of two-three independent experiments (*, P <0.05; **, P < 0.01).
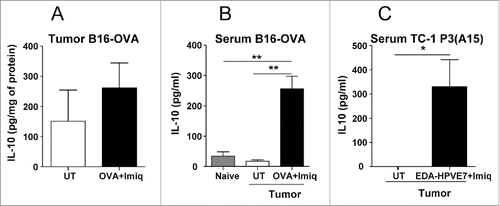
In order to confirm these findings in another tumor model and with a different vaccine we used the poorly immunogenic TC-1 P3(A15) sub-line.Citation30 This subline, generated after three cycles of immunoselection in vaccinated mice, has lower MHC class I expression, making it resistant to vaccines effective in the original more immunogenic TC-1 cells. Vaccines able to target the subline rely mainly on CD8 T cells and NK cells. Original TC-1 tumors have been shown to contain IL-10-producing macrophages, whereas it is hardly produced by tumor cells.Citation31 These features made this subline more suitable to test the efficacy of our vaccination strategy.
We vaccinated tumor-bearing mice with Imiquimod plus the EDA-HPVE7 immunogen. This immunogen, which we had previously demonstrated to be useful in the treatment of TC-1 tumors, Citation32 contains HPVE7 protein bound to the 91-amino acid extra domain A (EDA) from fibronectin, a TLR4 ligand which targets antigens to DC and induces its maturation, promoting the induction of T cell responses. As in B16-OVA-bearing mice, no clear differences were observed in tumor homogenates (data not shown), whereas serum IL-10 levels increased after vaccination (). Thus, these results show that Imiquimod-based vaccines induce IL-10 production in tumor-bearing mice.
Blockade of IL-10 during therapeutic vaccination enhances its antitumor effect
Once characterized the presence of IL-10 in the tumors and the ability of the vaccine to induce this cytokine we tested the antitumor efficacy of combining vaccination with IL-10 blockade. Mice bearing 5 mm B16-OVA tumors received three weekly cycles of Imiquimod, co-administered with OVA and anti-IL-10R antibodies. Vaccination alone decreased tumor growth as compared with control isotype-treated mice, prolonging animal survival for one week approximately, but no tumor rejections were observed. Moreover, no effect was observed when mice were treated only with anti-IL-10R antibodies, despite basal tumor IL-10 expression. By contrast, combination therapy not only led to a decreased tumor growth (p < 0.001; Vac + anti-IL-10R vs. remaining groups), but also induced rejection of three out of eight tumors (p < 0.001; Vac + anti-IL-10R vs. remaining groups) ().
Figure 2. IL-10 blockade enhances the antitumor effect of therapeutic vaccination in B16-OVA and TC-1 P3(A15) tumor-bearing mice. C57BL/6 mice (n = 8/group) bearing 5 mm B16-OVA (A) or TC-1 P3(A15) (B) tumors were vaccinated with three weekly cycles of topical Imiquimod administration plus i.t. immunogen injection (OVA or EDA-HPVE7, respectively), combined with i.p. injection of neutralizing anti-IL-10R antibodies or an isotype control. Control groups of animals treated with anti-IL-10R or isotype antibodies were also included. Tumor growth and animal survival was monitored twice per week. Results are representative of two independent experiments.
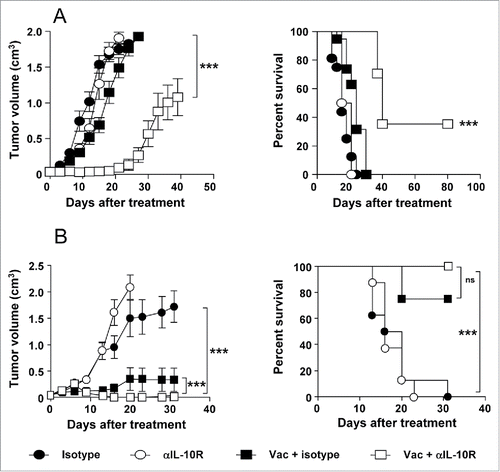
Similarly, in mice bearing TC-1 P3(A15) tumors, no beneficial effect was observed after administration of anti-IL-10R. However, in vaccinated groups, although the effect was not as clear as in B16-OVA tumors due to higher vaccine efficacy (p < 0.001; vaccinated groups vs. Isotype or anti-IL-10R groups), simultaneous vaccination and IL-10 blockade decreased tumor growth (p <0.001; Vac + anti-IL-10R vs. Vac + Isotype) and increased survival from 75% to 100% (), although this parameter did not reach statistical significance. Thus, although IL-10 blockade does not have any beneficial effect as stand-alone, when combined with Imiquimod-based vaccines results in a higher therapeutic antitumor effect.
Antitumor effect of therapeutic vaccination plus IL-10 blockade is associated with stronger innate and adaptive immunity
To characterize mechanisms responsible for the improved antitumor effect of the combined therapy, B16-OVA tumor-bearing mice were treated with the same vaccination immunotherapy with or without IL-10 blockade and 2 d after vaccination, tumor draining lymph nodes were collected to analyze innate immunity. Since co-stimulatory molecules like CD86 reflect phenotypic maturation status of DC and the prototypical Th1-polarizing cytokine IL-12 favors induction of anti-tumor IFN-γ-producing cells, we measured these parameters in DC. Blockade of IL-10 signal in vaccinated mice significantly increased CD86 expression, as compared with vaccinated mice which received control antibodies. Moreover, a higher percentage of IL-12+ DC was observed after IL-10 blockade (). Concerning NK cells, a higher proportion of these cells (data not shown) and of IFN-γ-producing NK cells were detected in mice vaccinated and receiving anti-IL-10R ().
Figure 3. IL-10 blockade enhances vaccine-induced antitumor innate and adaptive responses in tumor-bearing mice. C57BL/6 mice (n = 4–6/group) bearing 5 mm B16-OVA tumors received a single vaccination with topical Imiquimod administration plus i.t. OVA injection, combined with i.p. injection of neutralizing anti-IL-10R antibodies or an isotype control. (A) They were sacrificed 2 d after vaccination and CD86 and intracellular IL-12 were determined by flow cytometry in DC from tumor-draining lymph nodes. (B) In the same animals as in A, lymphocytes were cultured with the NK-sensitive YAC-1 cells line and IFN-γ production measured by ELISPOT. (C) Equivalent groups were sacrificed one week after vaccination and cells from tumor-draining lymph nodes were stimulated with OVA(257–264) peptide or B16-OVA tumor cells to measure IFN-γ-producing cells by ELISPOT. (D) OVA(257–264)-specific CD8+ T cells from mice shown in C were analyzed by flow cytometry measuring the percentage of IFN-γ+TNF-α+ and IFNγ+TNF- α +IL-2+ CD8+ T cells after 5 h of stimulation with OVA(257–264). (E) Mice with B16-OVA tumors (n=10/group) were depleted of CD8+, CD4+ or NK cells and vaccinated with OVA + Imiquimod plus IL-10 blockade. As controls mice were left untreated or vaccinated in the absence of any cell depletion. Tumor volume was measured in these animals. Results are representative of two independent experiments.
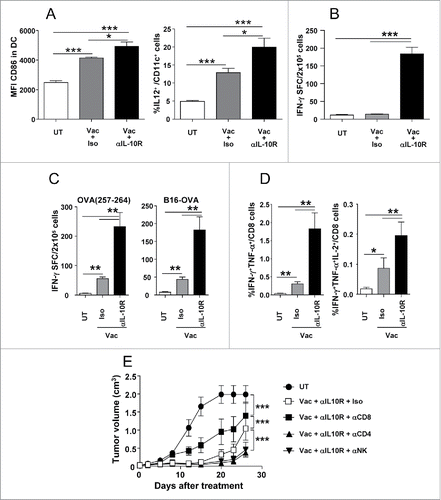
To study adaptive immune responses, mice were analyzed after one week of treatment, measuring responses against peptide OVA(257–264) or B16-OVA tumor cells. Vaccination alone induced weak responses above those found in untreated animals. By contrast, when combined with IL-10 blockade, it clearly induced the strongest responses against all antigens (), in accordance with their enhanced antitumor efficacy. Further analysis by flow cytometry of T cells confirmed this enhanced activation, shown as a higher percentage of OVA(257–264)-specific CD8 T cells producing double- (IFN-γ/TNF-α) and triple- (IFN-γ/TNF- α/IL-2) cytokines (). These results demonstrate that IL-10 blockade enhances innate functions with potential direct antitumor activity as well as those which result in stronger activation of Th1-adaptive responses, which would explain the higher therapeutic efficacy.
In order to characterize the role of cell subsets responsible for the enhanced antitumor effect observed in mice subjected to vaccination and IL-10 blockade, we carried out depletion experiments in mice with B16-OVA tumors. Depletion of CD8 cells, although still retained some antitumor activity, clearly impaired treatment efficacy. Interestingly, depletion of CD4 or NK cells somehow delayed tumor growth (). These data suggest that although IL-10 blockade improves the activity of several cell subsets, CD8 cells are main effectors involved in tumor rejection when using this strategy.
IL-10 blockade lacks antitumor effect when used in combination with adjuvants not inducing IL-10
Having demonstrated that IL-10 blockade improves antitumor effects of the vaccine and induction of immune responses, we wanted to discriminate whether these effects were due to blockade of IL-10 produced by the tumor, induced by the vaccine or both. To test this we blocked IL-10 signal during vaccination in naive tumor-free mice. Blockade with anti-IL-10R antibodies induced more potent responses, both against OVA protein and OVA(257–264) CD8 epitope (). Regarding responses against OVA(257–264), we could also observe a higher number of OVA(257–264)/H-2Kb tetramer-specific T cells, as well as increased effector functions, such as CD107 expression, and to lesser extent the simultaneous production of IFN-γ and TNF-α (Fig. S1). Equivalent results were observed after immunization of naive mice with Imiquimod plus the EDA-HPVE7 immunogen, where blockade with anti-IL-10R increased vaccine-induced CD8 responses against HPVE7(49–57) peptide (). These results suggest that blockade of IL-10 signal during vaccination with Imiquimod enhances antitumor responses, even in the absence of tumor-derived IL-10.
Figure 4. IL-10 blockade enhances (T)cell responses in an adjuvant-dependent manner. (A) C57BL/6 mice (n = 4 /group) immunized with OVA s.c. and topical Imiquimod cream application at the immunization site, combined with i.p. injection of isotype or anti-IL-10R antibodies. One week later their splenocytes were stimulated with CD8 epitope OVA(257–264) or OVA protein and IFNγ-producing cells evaluated by ELISPOT. (B) Mice were immunized with Imiquimod plus EDA-HPVE7 protein as in A with or without IL-10 blockade and responses against HPV E7(49–57) peptide were measured by ELISPOT. (C) C57BL/6 mice (n = 4) were immunized with OVA s.c. plus adjuvants CpG, poly(I:C) or anti-CD40 agonistic antibodies or with EDA-OVA plus control or anti-IL-10R blocking antibodies. One week later mice were sacrificed and their splenocytes were stimulated with CD8+ epitope OVA(257–264) and IFNγ-producing cells evaluated by ELISPOT. Results show the difference between peptide-stimulated minus unstimulated cells and are representative of two-three independent experiments.
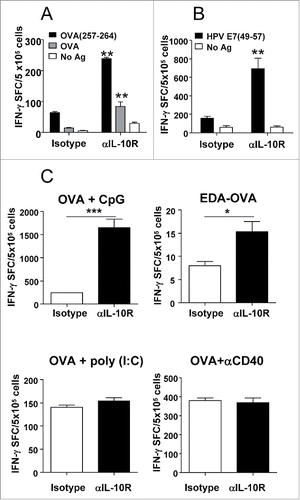
Next, to discriminate the role of tumor-derived IL-10 from that derived from the vaccine we searched for adjuvants which, when used in vaccination in naive mice, were not affected by IL-10 blockade. Vaccination experiments showed that when using OVA plus adjuvant CpG (TLR9 ligand) and to a lesser extent, using EDA-OVA (OVA covalently bound to the TLR4 ligand EDA), T cell responses were potentiated in the presence of IL-10 blockade. By contrast, no effect was observed when using poly(I:C) (TLR3 ligand) or agonistic anti-CD40 antibodies as adjuvants ().
IL-10 blockade enhances T cell responses when using Imiquimod or CpG as adjuvants and this blockade also improves antitumor effect of immunotherapy based on Imiquimod () or CpG.Citation33 We thus studied the antitumor effect of IL-10 blockade in conjunction with poly(I:C) and anti-CD40-based vaccines, conditions where blockade of IL-10 does not result in enhanced T cell responses in naive mice. Despite IL-10 expression in the B16-OVA model, blockade of IL-10 in combination with poly(I:C) did not affect tumor growth or animal survival when compared with mice treated with this immunotherapy plus control antibodies (). Similarly, when anti-CD40 antibodies were used as adjuvants, equivalent tumor growth and survival was observed in mice vaccinated and treated with anti-IL-10R or control antibodies ().
Figure 5. IL-10 blockade lacks antitumor effect when used in combination with adjuvants not inducing IL-10. C57BL/6 mice (n = 8/group) bearing 5 mm B16-OVA tumors were left untreated or received three weekly cycles of therapeutic vaccination consisting of i.t. injection of OVA plus poly(I:C) (A) or agonistic anti-CD40 antibodies (B), combined with i.p. injection of neutralizing anti-IL-10R antibodies or an isotype control. Tumor growth and animal survival was monitored twice per week. (C) Mice (n = 5/group) with 5 mm B16-OVA tumors were vaccinated with OVA+Imiquimod, OVA+poly(I:C) or left untreated. Two days later IL-10 content in tumor homogenates and serum was determined by ELISA. (D) Purified splenic CD11c+ DC from naïve C57BL/6 mice were stimulated in 96-well plates for 1 d with Imiquimod, CpG, poly(I:C), anti-CD40 or left unstimulated and IL-10 content in the supernatants was determined by ELISA. Results are representative of two-three independent experiments.
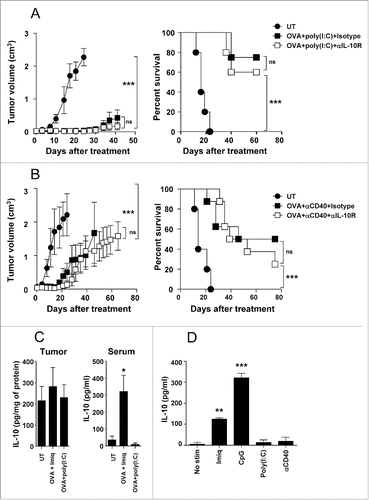
In order to understand the different antitumor effects obtained when blocking IL-10 signal in the presence of adjuvants we analyzed their ability to induce IL-10 in vivo. Vaccination of tumor-bearing mice using poly(I:C) did not increase tumor or serum IL-10, as opposed to Imiquimod, which upregulated IL-10 as previously found (). Moreover, since these differences in serum IL-10 could be detected at an early time-point after adjuvant administration, we measured in vitro IL-10 induction by cells of innate immunity. We found that in vitro stimulation of DC with poly(I:C) or anti-CD40 did not induce IL-10, whereas clear levels of IL-10, although of different magnitude, were detected in the presence of CpG and Imiquimod (). Similar in vitro analyses of IL-10 production by macrophages showed also a superior capacity of Imiquimod vs. poly(I:C) (Fig. S2). These results suggest that adjuvant ability to induce IL-10 is the factor dictating the enhanced efficacy provided by IL-10 blockade during vaccination.
Inhibition of IL-10 in a MAC-based immunotherapy results in complete tumor rejection
We previously demonstrated that therapeutic vaccination using antigen targeting through EDA, despite using a much lower antigen dose (∼100 μg), rejected about 50% of B16-OVA tumors if combined with a multiple adjuvant combination MAC, which was associated to the induction of strong antitumor responses.Citation34 We showed that in this stringent model, the adjuvant combination had immunopotentiating properties on DC, which included increased IL-12 production. This strategy includes adjuvants which benefit from IL-10 blockade (Imiquimod and EDA) as well as those which do not (poly(I:C) and anti-CD40). Since blockade of IL-10 when using Imiquimod as a single adjuvant-based vaccination therapy enhanced T cell responses and leads to increased tumor rejection, we analyzed the antitumor effect of IL-10 blockade when using the vaccine based on EDA-OVA+MAC administration. We first tested in naive mice the effect of IL-10 blockade during immunization with EDA-OVA+MAC. IL-10 blockade resulted in superior responses against OVA(257–264) and OVA protein (). Finally, treatment experiments were conducted in mice with established B16-OVA tumors. We found that inhibition of IL-10 in animals vaccinated with EDA-OVA+MAC resulted in complete tumor rejection ().
Figure 6. Inhibition of IL-10 when using a MAC-based therapeutic vaccine enhances antitumor responses resulting in complete tumor rejection. (A) C57BL/6 mice (n = 4) were immunized with EDA-OVA + MAC plus control or anti-IL-10R blocking antibodies. One week later mice were sacrificed and their splenocytes were stimulated with CD8 epitope OVA(257–264) or OVA protein and IFN-γ-producing cells evaluated by ELISPOT. (B) C57BL/6 mice (n = 8/group) bearing 5 mm B16-OVA tumors received three weekly cycles of vaccination with EDA-OVA + MAC, combined with i.p. injection of neutralizing anti-IL-10R antibodies or an isotype control. Tumor growth and animal survival was monitored twice per week. Results are representative of two independent experiments.
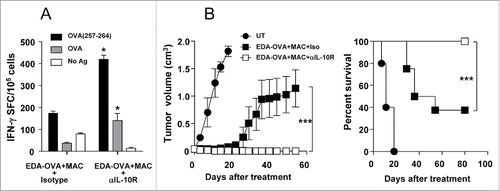
Discussion
Tumor immunotherapy based on the administration of antibodies against immunosuppressive molecules has been successful, but only in a proportion of patients.Citation1 Therefore, it is crucial to define those patients or settings where these strategies may provide a higher therapeutic benefit. In this context, IL-10 is a cytokine whose activity in antitumor immune responses is controversial. Although some authors report an immunosuppressive effect,Citation10,33 in other cases it has been demonstrated to possess a beneficial role.Citation18,19 Since these effects have been reported in different settings, in the present study we focused on the role of IL-10 during therapeutic vaccination. Our results show that in models of IL-10-containing tumors, blockade of IL-10 signal during therapeutic vaccination has a clear antitumor effect, which is associated with an enhancement of innate and adaptive immune responses. Interestingly, this enhancement of immune responses and concomitant antitumor effect was not observed when using all vaccination strategies, since it was adjuvant-dependent. Indeed, only when using those adjuvants which induced IL-10, a beneficial antitumor effect was observed. This is somehow surprising, because in the tumor models used for these studies there are additional IL-10 sources, such as tumor or stromal cells. However, despite the presence of tumor-associated IL-10, its blockade does not provide any benefit when used with adjuvants such as poly(I:C) or anti-CD40 antibodies. All these results suggest that in the setting of therapeutic vaccination, beneficial effects of IL-10 blockade are related to inhibition of vaccine-induced but not tumor-associated IL-10, and this is adjuvant-dependent.
There are examples of tumor models where antibody or genetic ablation of IL-10 shows an antitumor effect associated with enhanced immunity, even in the absence of additional vaccination. Tumors expressing HY can be rejected by IL-10 KO female miceCitation35,36 and similarly, in original TC-1 tumors, which contain IL-10 produced by macrophages, inhibition of IL-10 with Abs or in IL-10 KO mice also results in tumor growth delay and higher T cell infiltration. Citation31 In both models, just by inhibiting IL-10 present in the tumor, DC and Th1 activation is rescued, suggesting higher tumor immunogenicity. In our models however, there are not spontaneous tumor rejections, even after blockade of IL-10. Thus, depending on the priming situation (immunogenic tumors which spontaneously prime responses vs. non-immunogenic tumors which require exogenous antigen and vaccination), vaccine-induced IL-10 may be more relevant.
There are several potential IL-10 sources in our models. We have previously shown that B16-OVA tumors contain IL-10 Citation24 and it has been described that B16 tumor cells in culture produce IL-10.Citation9 However, we have not detected IL-10 by ELISA in supernatants of our B16-OVA cells, even after in vitro stimulation with IL-10-inducing TLR ligands (data not shown), despite TLR expression in these cells.Citation37 CD4+ T cells have been reported as IL-10 producers after Imiquimod administration Citation29 in a breast tumor model, suggesting that these cells may also produce IL-10 in our model. However, the early detection of IL-10 after Imiquimod administration (1–2 d) suggests that innate immunity may play an important role in IL-10 production. It has been shown that some TLR ligands induce IL-10 production by DC,Citation38 which would inhibit their antigen-presenting functions and the concomitant induction of T cell responses. Our in vitro experiments show the different ability of adjuvants to induce IL-10 by DC, associated to in vivo results of serum IL-10 and susceptibility to IL-10 blockade. In this line, it has been demonstrated that adjuvants not only determine IL-10 production by DC, but also dictate their sensitivity to be inhibited by IL-10, modulating thus their functional properties.Citation39 Indeed, MyD88-independent adjuvants such as poly(I:C), are resistant to IL-10 inhibitory effects, whereas DC activated through MyD88-dependent adjuvants (Imiquimod or CpG) can be inhibited by this cytokine. Thus, these results may suggest DC as a potential IL-10 source and at the same time may explain why anti-IL-10R antibodies, even in the presence of tumor IL-10, do not provide any benefit when using poly(I:C) or anti-CD40 as adjuvants.
Although IL-10 has been considered an immunosuppressive cytokine, it has been also reported to enhance CD8+ T cell functions.Citation18,40 In our model, adjuvant-induced IL-10 would timely act in an autocrine manner on APC, inhibiting their functions in the T cell priming phase, without effect during the effector phase, where APC may not longer be necessary. Indeed, when we blocked IL-10 at day 7 during the effector phase of in vivo killing experiments, once responses have been induced, no enhancement of responses were observed (Fig. S3), reinforcing the idea that, in therapeutic vaccination, IL-10 blockade would be more relevant to enhance APC functions during the priming phase. Indeed, these results agree with recently reported data showing that IL-10 has a dual role on CD8 T cell responses, initially inhibiting DC in the priming of these responses, but being necessary for their maintenance after postclonal expansion,Citation41 in accordance also with beneficial effect of IL-10 through enhancement of antitumor functional properties of effector CD8 T cells.Citation18,40
Vaccination strategies based on single adjuvants have shown poorer efficacy than those using adjuvant combinations. We had previously demonstrated that vaccination with MAC plus antigen targeting through EDA, despite low antigen dose administration, had a high antitumor therapeutic efficacy, mainly due to the effect of CD8 T cells potently primed by highly activated DC.Citation34 This adjuvant combination contains Imiquimod, EDA, poly(I:C) and anti-CD40 adjuvants, some of them inducing IL-10. Thus, while maintaining the ability to produce IL-10, this combination has enhanced immunostimulatory properties. Therefore it was of interest to analyze the effect of IL-10 blockade in this setting. We found more potent immune responses which lead to complete tumor rejection, as compared with partial rejection obtained in the absence of IL-10 blockade. In line with these results, in vitro experiments using human DC have demonstrated that LPS inhibits poly(I:C)-induced IL-12 production and this was mediated by IL-10 induced by LPS.Citation15 As occurred in our case, blockade of IL-10 in their context enhanced those responses induced by poly(I:C). Combination of these strategies (adjuvant mixtures plus blockade of immunosuppression), on the one side allows enhancing immunity, and on the other side inhibits negative feed-back mechanisms, leading to an overall higher immunity.
In summary, we have found that during therapeutic vaccination of poorly immunogenic tumors, blockade of IL-10 enhances the efficacy of the antitumor immune response and concomitant tumor rejection by inhibiting IL-10 induced by the vaccine. Although some murine tumor models may show a partial benefit only with IL-10 blockade, they correspond to immunogenic tumors, a situation uncommon in cancer patients, suggesting thus that strategies aimed at blocking IL-10 would obtain their highest benefit not when applied as single therapies, but in the context of therapeutic maneuvers which induce this cytokine.
Materials and methods
Peptides and antigens
CD8+ epitope peptides OVA(257–264) and HPV16 E7(49–57), with a purity >95%, were obtained from NeoMPS. Proteins containing the EDA from fibronectin EDA-OVA and EDA-HPV were produced as described.Citation32,42 Endotoxin levels in these proteins were below 0.2 EU/μg protein. OVA protein (low endotoxin) was purchased from Hyglos (321001).
Mice
Female C57BL/6 (Harlan; Barcelona, Spain) were maintained in pathogen-free conditions and treated according to guidelines of our institution, after study approval by the review committee.
Cell lines
B16-OVA tumor cells, obtained from Dr. G. Kroemer (Paris, France), were grown in DMEM containing 10% fetal calf serum and antibiotics. TC-1 P3 (A15) cells, Citation30 obtained from Dr. T.-C. Wu (Baltimore, MD, USA) and the NK-sensitive cell line YAC-1 (ATCC, Manassas, VA) were grown in RPMI 1640 medium containing 10% fetal calf serum and antibiotics.
Immunization of mice
Naive mice were injected subcutaneously with OVA protein (0.5 mg/mouse) or EDA-containing immunogens (EDA-OVA or EDA-HPVE7) (2 nanomoles) on day 0 combined with the following adjuvants: Imiquimod cream (722710.6, Meda Aldara™; topical application; 2.5 mg/mouse) on days 0, 1 and 2; poly(I:C) (27–4732; Amersham; 50 μg/mouse s.c.) on day 0; CpG 1668 (Sigma; 50 μg/mouse s.c.) on day 0 and agonistic FGK45.5 anti-CD40 antibodies (BE0016–2, BioXcell; 50 μg/mouse s.c.) on day 0. On day 7 they were sacrificed and splenocytes were obtained for immunological analysis. Additionally, they received 500 μg i.p.of anti-IL-10R (1B1.3A; BE0050, BioXcell) and the corresponding isotype control antibodies (BE0088, BioXcell;) on day 0.
Tumor treatment experiments
Mice were injected intradermally with 105 B16-OVA cells and when the tumor diameter reached 4–5 mm, they received three weekly cycles of immunotherapy consisting of OVA protein (intratumor; 0.5 mg/mouse) on day 0 combined with adjuvants as described above. Some groups received administrations of anti-IL-10R or isotype antibodies (500 μg i.p.) on days 0 and 7. In some experiments, mice were vaccinated with EDA-OVA plus a MAC.Citation34 Treatment experiments of TC-1 tumors included injection of EDA-HPVE7 protein plus Imiquimod administered as above. For depletion experiments, mice received i.p. injections of 200 μg of anti-CD8 (H35.17.2; a kindly gift of Dr. C. Leclerc; Paris, France), -CD4+ (GK1.5; BE003–1, BioXcell) -NK1.1 (PK136; BE0036, BioXcell) or isotype control (BE0088, BioXcell) antibodies on days –1, 0, 1 and 6, being 0 the day when treatment starts. Untreated mice challenged with tumor cells were used as positive controls of tumor growth. Tumor volume was calculated according to the formula: V= (length × widthCitation2)/2. Mice were killed when tumor diameter reached 17 mm.
ELISPOT
Cells producing IFN-γ were enumerated by ELISPOT (551083, BD-Biosciences) as described.Citation34 For T-cell responses, splenocytes or lymph node cells were stimulated with peptides OVA(257–264), HPV16 E7(49–57) (1 μg/mL), OVA protein (10 μg/mL) or 4×104 irradiated (20,000 rads) tumor cells. When measuring NK cell derived production of IFNγ, splenocytes were incubated with 4×104 irradiated-YAC-1 cells.
Flow cytometry
To analyze NK cells and DC, spleens or tumor-draining lymph nodes were obtained 2 d after vaccination, treated with collagenase and DNAse for 15 min and homogenized. Then, cells were first incubated for 10 min with Fc Block™ (553142, BD-Biosciences) and stained with specific antibodies. For NK cell analysis, cells were stained with anti-CD69-FITC (557392), anti-CD3-PE (555275) and anti-NK1.1-APC-labeled (175941) antibodies. DC were analyzed using anti-CD11c-APC (550261), anti-IAb (either PE- (06355A) or FITC- (553551) and anti-CD86-FITC (553691) antibodies. To measure IL-12 production, DC were incubated for 5 h with GolgiStop (554724, BD-Biosciences) and labeled with anti-CD11c-APC, anti-IAb-FITC. Then, after fixation and permeabilization, they were labeled with anti-IL-12 p70-PE (554479). For T cell analyses, spleens or tumor-draining lymph nodes were obtained one week after vaccination and OVA(257–264)/H-2Kb tetramer-specific T cells were enumerated as described,Citation34 using a 1/100 tetramer dilution. T-cell activation was analyzed after stimulation of splenocytes with 1 μυσmL of OVA(257–264) in the presence of GolgiStop and GolgiPlug (555029, BD-Biosciences) with or without anti-CD107a and CD107b-FITC antibodies (553793 and 558758 respectively), for 4 h. Then cells were labeled with anti-CD8+-APC (553035) and fixed, permeabilized and stained with anti-IFNγ-PE (562020). For double/triple cytokine analysis, after surface staining with anti-CD8-FITC (553030), cells were stained with anti-IFNγ-PE (562020), anti-TNF-α-PE-Cy7 (557644) and anti-IL-2-APC (554229) labeled antibodies. All antibodies were from BD-Biosciences, except anti-NK1.1 (e-Bioscience). Expression of the different markers was analyzed with a FACSCalibur flow cytometer (Becton Dickinson) and Flowjo software (Tree Star Inc.).
Measurement of IL-10 by ELISA
In vivo produced IL-10 was determined in tumor homogenates and serum from treated mice obtained 2 d after vaccination. In vitro produced IL-10 was determined from supernatants of splenic DC obtained after purification with anti-CD11c-conjugated magnetic beads (130–052–001, Miltenyi). For these experiments, five wells per condition containing 105 DC were cultured for 24 h in 96-well plates with the following adjuvants: Imiquimod (tlrl-imq, Invivogen (10 μg/mL)), poly(I:C) (25 μg/mL), CpG (5 μg/mL), anti-CD40 (50 μg/mL). In all cases IL-10 was measured using OptEIA Set (555252, BD-Biosciences).
Statistical analysis
Tumor growth was fitted to a third order polynomial and compared with the Extra sum-of-squares F test. Survival curves of animals treated with different protocols were plotted according to the Kaplan–Meier method and were compared using the log-rank test. Immune responses were analyzed using non-parametric Kruskal–Wallis and Mann–Whitney U tests. P <0.05 was taken to represent statistical significance.
Disclosure of potential conflicts of interest
No conflicts of interest exist regarding this manuscript.
1075113_Supplemental_Figures.pdf
Download PDF (72.6 KB)Acknowledgments
Authors thank Drs. Kroemer, Wu and Leclerc for their kindly gift of tumor cells and antibodies.
Funding
This study is supported by Ministerio de Economia y Competitividad/Instituto de Salud Carlos III (grant PI14/00343)
References
- Shin DS, Ribas A. The evolution of checkpoint blockade as a cancer therapy: what's here, what's next? Curr Opin Immunol 2015; 33:23-35; PMID:25621841; http://dx.doi.org/10.1016/j.coi.2015.01.006
- Melero I, Gaudernack G, Gerritsen W, Huber C, Parmiani G, Scholl S, Thatcher N, Wagstaff J, Zielinski C, Faulkner I et al. Therapeutic vaccines for cancer: an overview of clinical trials. Nat Rev Clin Oncol 2014; 11:509-24; PMID:25001465; http://dx.doi.org/10.1038/nrclinonc.2014.111
- Curran MA, Montalvo W, Yagita H, Allison JP. PD-1 and CTLA-4 combination blockade expands infiltrating T cells and reduces regulatory T and myeloid cells within B16 melanoma tumors. Proc Natl Acad Sci U S A 2010; 107:4275-80; PMID:20160101; http://dx.doi.org/10.1073/pnas.0915174107
- Pestka S, Krause CD, Sarkar D, Walter MR, Shi Y, Fisher PB. Interleukin-10 and related cytokines and receptors. Annu Rev Immunol 2004; 22:929-79; PMID:15032600; http://dx.doi.org/10.1146/annurev.immunol.22.012703.104622
- Saraiva M, O'Garra A. The regulation of IL-10 production by immune cells. Nat Rev Immunol 2010; 10:170-81; PMID:20154735; http://dx.doi.org/10.1038/nri2711
- O'Garra A, Barrat FJ, Castro AG, Vicari A, Hawrylowicz C. Strategies for use of IL-10 or its antagonists in human disease. Immunol Rev 2008; 223:114-31; PMID:18613832; http://dx.doi.org/10.1111/j.1600-065X.2008.00635.x
- Brooks DG, Trifilo MJ, Edelmann KH, Teyton L, McGavern DB, Oldstone MB. Interleukin-10 determines viral clearance or persistence in vivo. Nat Med 2006; 12:1301-9; PMID:17041596; http://dx.doi.org/10.1038/nm1492
- Moore KW, de Waal Malefyt R, Coffman RL, O'Garra A. Interleukin-10 and the interleukin-10 receptor. Annu Rev Immunol 2001; 19:683-765; PMID:11244051; http://dx.doi.org/10.1146/annurev.immunol.19.1.683
- Sredni B, Weil M, Khomenok G, Lebenthal I, Teitz S, Mardor Y, Ram Z, Orenstein A, Kershenovich A, Michowiz S et al. Ammonium trichloro(dioxoethylene-o,o')tellurate (AS101) sensitizes tumors to chemotherapy by inhibiting the tumor interleukin 10 autocrine loop. Cancer Res 2004; 64:1843-52; PMID:14996748; http://dx.doi.org/10.1158/0008-5472.CAN-03-3179
- Jarnicki AG, Conroy H, Brereton C, Donnelly G, Toomey D, Walsh K, Sweeney C, Leavy O, Fletcher J, Lavelle EC et al. Attenuating regulatory T cell induction by TLR agonists through inhibition of p38 MAPK signaling in dendritic cells enhances their efficacy as vaccine adjuvants and cancer immunotherapeutics. J Immunol 2008; 180:3797-806; PMID:18322186; http://dx.doi.org/10.4049/jimmunol.180.6.3797
- Darrah PA, Hegde ST, Patel DT, Lindsay RW, Chen L, Roederer M, Seder RA. IL-10 production differentially influences the magnitude, quality, and protective capacity of Th1 responses depending on the vaccine platform. J Exp Med 2010; 207:1421-33; PMID:20530206; http://dx.doi.org/10.1084/jem.20092532
- Ahonen CL, Doxsee CL, McGurran SM, Riter TR, Wade WF, Barth RJ, Vasilakos JP, Noelle RJ, Kedl RM. Combined TLR and CD40 triggering induces potent CD8+ T cell expansion with variable dependence on type I IFN. J Exp Med 2004; 199:775-84. Epub 2004 Mar 8; PMID:15007094; http://dx.doi.org/10.1084/jem.20031591
- Celis E. Toll-like receptor ligands energize peptide vaccines through multiple paths. Cancer Res 2007; 67:7945-7; PMID:17804699; http://dx.doi.org/10.1158/0008-5472.CAN-07-1652
- Wells JW, Cowled CJ, Farzaneh F, Noble A. Combined triggering of dendritic cell receptors results in synergistic activation and potent cytotoxic immunity. J Immunol 2008; 181:3422-31; PMID:18714014; http://dx.doi.org/10.4049/jimmunol.181.5.3422
- Bogunovic D, Manches O, Godefroy E, Yewdall A, Gallois A, Salazar AM, Marie I, Levy DE, Bhardwaj N. TLR4 engagement during TLR3-induced proinflammatory signaling in dendritic cells promotes IL-10-mediated suppression of antitumor immunity. Cancer Res 2011; 71:5467-76; PMID:21730023; http://dx.doi.org/10.1158/0008-5472.CAN-10-3988
- Berman RM, Suzuki T, Tahara H, Robbins PD, Narula SK, Lotze MT. Systemic administration of cellular IL-10 induces an effective, specific, and long-lived immune response against established tumors in mice. J Immunol 1996; 157:231-8; PMID:8683120
- Zheng LM, Ojcius DM, Garaud F, Roth C, Maxwell E, Li Z, Rong H, Chen J, Wang XY, Catino JJ et al. Interleukin-10 inhibits tumor metastasis through an NK cell-dependent mechanism. J Exp Med 1996; 184:579-84; PMID:8760811; http://dx.doi.org/10.1084/jem.184.2.579
- Mumm JB, Emmerich J, Zhang X, Chan I, Wu L, Mauze S, Blaisdell S, Basham B, Dai J, Grein J et al. IL-10 elicits IFNgamma-dependent tumor immune surveillance. Cancer Cell 2011; 20:781-96; PMID:22172723; http://dx.doi.org/10.1016/j.ccr.2011.11.003
- Tanikawa T, Wilke CM, Kryczek I, Chen GY, Kao J, Nunez G, Zou W. Interleukin-10 ablation promotes tumor development, growth, and metastasis. Cancer Res 2012; 72:420-9; PMID:22123924; http://dx.doi.org/10.1158/0008-5472.CAN-10-4627
- Groux H, Bigler M, de Vries JE, Roncarolo MG. Inhibitory and stimulatory effects of IL-10 on human CD8+ T cells. J Immunol 1998; 160:3188-93; PMID:9531274
- Mocellin S, Panelli MC, Wang E, Nagorsen D, Marincola FM. The dual role of IL-10. Trends Immunol 2003; 24:36-43; PMID:12495723; http://dx.doi.org/10.1016/S1471-4906(02)00009-1
- Seo N, Hayakawa S, Takigawa M, Tokura Y. Interleukin-10 expressed at early tumour sites induces subsequent generation of CD4(+) T-regulatory cells and systemic collapse of antitumour immunity. Immunology 2001; 103:449-57; PMID:11529935; http://dx.doi.org/10.1046/j.1365-2567.2001.01279.x
- Bouabe H, Liu Y, Moser M, Bosl MR, Heesemann J. Novel highly sensitive IL-10-beta-lactamase reporter mouse reveals cells of the innate immune system as a substantial source of IL-10 in vivo. J Immunol 2011; 187:3165-76; PMID:21844394; http://dx.doi.org/10.4049/jimmunol.1101477
- Diaz-Valdes N, Basagoiti M, Dotor J, Aranda F, Monreal I, Riezu-Boj JI, Borras-Cuesta F, Sarobe P, Feijoo E. Induction of monocyte chemoattractant protein-1 and interleukin-10 by TGFbeta1 in melanoma enhances tumor infiltration and immunosuppression. Cancer Res 2011; 71:812-21; PMID:21159663; http://dx.doi.org/10.1158/0008-5472.CAN-10-2698
- Shackleton M, Davis ID, Hopkins W, Jackson H, Dimopoulos N, Tai T, Chen Q, Parente P, Jefford M, Masterman KA et al. The impact of imiquimod, a Toll-like receptor-7 ligand (TLR7L), on the immunogenicity of melanoma peptide vaccination with adjuvant Flt3 ligand. Cancer Immun 2004; 4:9; PMID:15384929
- Adams S, O'Neill DW, Nonaka D, Hardin E, Chiriboga L, Siu K, Cruz CM, Angiulli A, Angiulli F, Ritter E et al. Immunization of malignant melanoma patients with full-length NY-ESO-1 protein using TLR7 agonist imiquimod as vaccine adjuvant. J Immunol 2008; 181:776-84; PMID:18566444; http://dx.doi.org/10.4049/jimmunol.181.1.776
- Feyerabend S, Stevanovic S, Gouttefangeas C, Wernet D, Hennenlotter J, Bedke J, Dietz K, Pascolo S, Kuczyk M, Rammensee HG et al. Novel multi-peptide vaccination in Hla-A2+ hormone sensitive patients with biochemical relapse of prostate cancer. Prostate 2009; 69:917-27; PMID:19267352; http://dx.doi.org/10.1002/pros.20941
- Goldinger SM, Dummer R, Baumgaertner P, Mihic-Probst D, Schwarz K, Hammann-Haenni A, Willers J, Geldhof C, Prior JO, Kundig TM et al. Nano-particle vaccination combined with TLR-7 and -9 ligands triggers memory and effector CD8(+) T-cell responses in melanoma patients. Eur J Immunol 2012; 42:3049-61; PMID:22806397; http://dx.doi.org/10.1002/eji.201142361
- Lu H, Wagner WM, Gad E, Yang Y, Duan H, Amon LM, Van Denend N, Larson ER, Chang A, Tufvesson H et al. Treatment failure of a TLR-7 agonist occurs due to self-regulation of acute inflammation and can be overcome by IL-10 blockade. J Immunol 2010; 184:5360-7; PMID:20308630; http://dx.doi.org/10.4049/jimmunol.0902997
- Cheng WF, Hung CF, Lin KY, Ling M, Juang J, He L, Lin CT, Wu TC. CD8+ T cells, NK cells and IFN-gamma are important for control of tumor with downregulated MHC class I expression by DNA vaccination. Gene Ther 2003; 10:1311-20; PMID:12883527; http://dx.doi.org/10.1038/sj.gt.3301982
- Bolpetti A, Silva JS, Villa LL, Lepique AP. Interleukin-10 production by tumor infiltrating macrophages plays a role in Human Papillomavirus 16 tumor growth. BMC Immunol 2010; 11:27; PMID:20525400; http://dx.doi.org/10.1186/1471-2172-11-27
- Mansilla C, Berraondo P, Durantez M, Martinez M, Casares N, Arribillaga L, Rudilla F, Fioravanti J, Lozano T, Villanueva L et al. Eradication of large tumors expressing human papillomavirus E7 protein by therapeutic vaccination with E7 fused to the extra domain a from fibronectin. Int J Cancer 2012; 131:641-51; PMID:21898393; http://dx.doi.org/10.1002/ijc.26412
- Vicari AP, Chiodoni C, Vaure C, Ait-Yahia S, Dercamp C, Matsos F, Reynard O, Taverne C, Merle P, Colombo MP et al. Reversal of tumor-induced dendritic cell paralysis by CpG immunostimulatory oligonucleotide and anti-interleukin 10 receptor antibody. J Exp Med 2002; 196:541-9; PMID:12186845; http://dx.doi.org/10.1084/jem.20020732
- Aranda F, Llopiz D, Diaz-Valdes N, Riezu-Boj JI, Bezunartea J, Ruiz M, Martinez M, Durantez M, Mansilla C, Prieto J et al. Adjuvant combination and antigen targeting as a strategy to induce polyfunctional and high-avidity T-cell responses against poorly immunogenic tumors. Cancer Res 2011; 71:3214-24; PMID:21402711; http://dx.doi.org/10.1158/0008-5472.CAN-10-3259
- Halak BK, Maguire HC, Jr., Lattime EC. Tumor-induced interleukin-10 inhibits type 1 immune responses directed at a tumor antigen as well as a non-tumor antigen present at the tumor site. Cancer Res 1999; 59:911-7; PMID:10029084
- Yang AS, Lattime EC. Tumor-induced interleukin 10 suppresses the ability of splenic dendritic cells to stimulate CD4 and CD8 T-cell responses. Cancer Res 2003; 63:2150-7; PMID:12727833
- Yang HZ, Cui B, Liu HZ, Mi S, Yan J, Yan HM, Hua F, Lin H, Cai WF, Xie WJ et al. Blocking TLR2 activity attenuates pulmonary metastases of tumor. PLoS One 2009; 4:e6520; PMID:19654875; http://dx.doi.org/10.1371/journal.pone.0006520
- Samarasinghe R, Tailor P, Tamura T, Kaisho T, Akira S, Ozato K. Induction of an anti-inflammatory cytokine, IL-10, in dendritic cells after toll-like receptor signaling. J Interferon Cytokine Res 2006; 26:893-900; PMID:17238832; http://dx.doi.org/10.1089/jir.2006.26.893
- Chang J, Kunkel SL, Chang CH. Negative regulation of MyD88-dependent signaling by IL-10 in dendritic cells. Proc Natl Acad Sci U S A 2009; 106:18327-32; PMID:19815506; http://dx.doi.org/10.1073/pnas.0905815106
- Emmerich J, Mumm JB, Chan IH, LaFace D, Truong H, McClanahan T, Gorman DM, Oft M. IL-10 directly activates and expands tumor-resident CD8(+) T cells without de novo infiltration from secondary lymphoid organs. Cancer Res 2012; 72:3570-81; PMID:22581824; http://dx.doi.org/10.1158/0008-5472.CAN-12-0721
- Fu C, Liang X, Cui W, Ober-Blobaum JL, Vazzana J, Shrikant PA, Lee KP, Clausen BE, Mellman I, Jiang A. Beta-Catenin in dendritic cells exerts opposite functions in cross-priming and maintenance of CD8+ T cells through regulation of IL-10. Proc Natl Acad Sci U S A 2015; 112:2823-8; PMID:25730849; http://dx.doi.org/10.1073/pnas.1414167112
- Lasarte JJ, Casares N, Gorraiz M, Hervas-Stubbs S, Arribillaga L, Mansilla C, Durantez M, Llopiz D, Sarobe P, Borras-Cuesta F et al. The extra domain A from fibronectin targets antigens to TLR4-expressing cells and induces cytotoxic T cell responses in vivo. J Immunol 2007; 178:748-56; PMID:17202335; http://dx.doi.org/10.4049/jimmunol.178.2.748