ABSTRACT
High-grade gliomas are notoriously heterogeneous regarding antigen expression, effector responses, and immunosuppressive mechanisms. Therefore, combinational immune therapeutic approaches are more likely to impact a greater number of patients and result in longer, durable responses. We have previously demonstrated the monotherapeutic effects of miR-124, which inhibits the signal transducer and activator of transcription 3 (STAT3) immune suppressive pathway, and immune stimulatory 4–1BB aptamers against a variety of malignancies, including genetically engineered immune competent high-grade gliomas. To evaluate potential synergy, we tested an immune stimulatory aptamer together with microRNA-124 (miRNA-124), which blocks tumor-mediated immune suppression, and found survival to be markedly enhanced, including beyond that produced by monotherapy. The synergistic activity appeared to be not only secondary to enhanced CD3+ cell numbers but also to reduced macrophage immune tumor trafficking, indicating that a greater therapeutic benefit can be achieved with approaches that both induce immune activation and inhibit tumor-mediated immune suppression within the central nervous system (CNS) tumors.
Abbreviations
CNS | = | Central nervous system |
GEMMs | = | genetically engineered murine models |
STAT3 | = | signal transducer and activator of transcription 3 |
VEGF | = | vascular endothelial growth factor |
Introduction
Given the heterogeneity of glioblastoma, combinatorial approaches that simultaneously enhance immune effector responses and control glioblastoma-mediated immune suppression are most likely to achieve long-term durable responses. One promising immunotherapeutic being tested in cancer patients is the anti-4–1BB antibody, which strongly activates and promotes the survival of cytotoxic CD8+ T cells. However, this antibody has dose-limiting toxicity secondary to cytokine storm. As with the antibody, the 4–1BB oligonucleotide RNA aptamer engages the 4–1BB costimulatory receptor on CD8+ T cells, with similar effects but reduced toxicity.Citation1 In order to optimize 4–1BB engagement of the aptamer with T cells within the tumor microenvironment, the 4–1BB aptamer was conjugated to a second aptamer that binds to vascular endothelial growth factor (VEGF), a stromal product highly expressed within glioblastomas. Systemic administration of this bi-specific VEGF/4–1BB aptamer conjugate (designated VEGF-4–1BB) engenders antitumor immunity against multiple tumor types, including a modest, yet statistically significant, therapeutic effect in a heterogeneous murine model of high-grade glioma.Citation2
We have also reported that miR-124 binds mRNAs encoding multiple nodes along the signal transducer activator of transcription 3 (STAT3) pathway—a key molecular hub of tumor-mediated immune suppression. Therapy with miR-124 induced marked increases in the median survival time in a variety of murine glioma model systems, and the responses correlated with marked decreases of glioma-infiltrating Tregs and marked increases of TNF-α and IFNγ-secreting CD4+ and CD8+ T cells. miR-124 exerts its primary therapeutic effect through the immune system, as shown by ablation of its efficacy in nude mouse models and T cell depletion studies.Citation3 Although there may be some degree of immune activation and immune cell trafficking into the glioblastoma microenvironment, we have previously documented that these immune effector responses are anergic,Citation4 which is consistent with immune effectors becoming exhausted within the tumor microenvironment.Citation5 We therefore hypothesized that a combined approach that stimulates immune responses and blocks immune suppression would exert an additive therapeutic effect and would enhance immune responses within the glioma microenvironment.
Materials and methods
Glioma models
Creation of the transgenic Ntv-a mouse has been previously described.Citation6,7,8 The mice are mixtures of the following strains: C57BL/6, BALB/C, FVB/N, and CD1. We have previously described, including the immunological characteristics, the transduction of the PDGF and STAT3 genes to induce high-grade gliomas in these mice.Citation9 To transfer genes via RCAS vectors, transfected DF-1 producer cells (1×105 cells in 1 to 2 µL of PBS) were injected into the right frontal brain lobe of Ntv-a mice from an entry point just anterior to the coronal suture of the skull, using a gas-tight Hamilton syringe. Mice were injected within 24 to 72 h after birth, because the population of nestin-positive cells producing TVA receptors diminishes progressively with time. For co-injection of RCAS-PDGFB and RCAS-STAT3, equal numbers of DF-1 cells were injected. On post-natal day 21, littermates were randomized into a treatment arm and therapy was initiated. The mice were compassionately killed 90 d after injection or sooner if they demonstrated neurological morbidity related to tumor burden, including hydrocephalus or disability of motor function. Their brains were fixed in formalin, embedded in paraffin, sectioned for immunohistochemical analysis, and analyzed for tumor formation. Histological verification of tumor formation and determination of low- or high-grade type was performed by the study's neuropathologist (GNF). High-grade tumors were distinguished by the presence of microvascular proliferation, mitotic activity, and necrosis. The animal experiments described in this research were approved by The Institutional Animal Care and Use Committee at The University of Texas MD Anderson Cancer Center (Protocols 08–06–11632 and 08–06–11832).
Aptamers, miR-124, and 4–1BB antibodies
The VEGF-4–1BB aptamer was created with a 2′-fluoro-pyrimidine modified-dimeric 4–1BB RNA aptamer transcribed in vitro from a DNA template Citation10 extended at the 3′ end with a linker sequence (5′-UCCCGCUAUAAGUGUGCAUGAGAAC-3′), which was annealed to a VEGFCitation11 chemically synthesized (IDT, Colarville, IA) aptamer via a complementary linker sequence engineered at their three ends. Equimolar amounts of 4–1BB and VEGF aptamers were mixed, heated to 75°C, and cooled to room temperature. Annealing efficiency, monitored by agarose gel electrophoresis was >80%. The VEGF-4–1BB conjugated aptamer is designed to provide co-stimulation in areas of VEGF expression. 100 pmoles of aptamer conjugate were administered by tail vein injection. The 4–1BB antibody (3H3) was purchased from eBioscience (San Diego, CA), and 800 pmoles of the antibody were used for each intraperitoneal injection. Two different concentrations of the 4–1BB aptamer were used in the experiments; 100 pmoles of the 4–1BB aptamer (1X) is equivalent to the concentration of the VEGF-4–1BB conjugated aptamer used, whereas 800 pmoles of the 4–1BB aptamer (8X) is the equivalent to the concentration of the 4–1BB antibody used. The miR-124 duplex that mimics miR-124a (sense: 5′-UAAGGCACGCGGUGAAUGCCA-3′, antisense: 3′-UAAUUCCGUGCGCCACUUACG-5′) and the scramble control miRNA duplex (sense: 5′-AGUACUGCUUACGAUACGGTT-3′, antisense: 3′-TTUCAUGACG AAUGCUAUGCC-5′) were synthesized (SynGen, San Carlos, CA). The treatment cohorts consisted of 20 µg of the miR-124 duplex in 10 µL of PBS mixed with the vehicle (80 µL PBS containing 10 µL lipofectamine 2000; Invitrogen) or the vehicle control (90 µL PBS + 10 µL lipofectamine 2000).
Treatment schema and animal randomization
After receiving the injection of the PDGF-B + STAT3 constructs as described, littermates were randomized and placed into the treatment or control groups. This methodology of using RCAS/Ntv-a mice to determine treatment efficacy has been described previously.Citation9,12
Immunohistochemistry
Mouse brains were paraffin-embedded, and 4-µm brain sections were used for immunohistochemical analysis. The Thermo Scientific PT Module (Thermo Fisher Scientific, Fremont, CA, USA) with citrate buffer was used for antigen retrieval. A rabbit polyclonal antibody to VEGF (1:100; Millipore, Temecula, CA), a mouse monoclonal antibody to CD3 (1:200; Abcam, Cambridge, MA), a primary antibody to FoxP3 (1:50; eBioscience, San Diego, CA), or an antibody to the macrophage and microglia-restricted cell surface glycoprotein F4/80 Citation13,14 (1:50; Biolegend, San Diego, CA) were used. Staining was performed using the Lab Vision Immunohistochemical Autostainer 360 (Thermo Fisher Scientific). Immunoreactive staining was visualized using an avidin–biotin complex technique with diaminobenzidine (Invitrogen, Carlsbad, CA, USA) as the chromogenic substrate and hematoxylin as the counterstain. Slides were subjected to biotin-labeled secondary antibody staining (biotinylated link universal solution; DAKO) for 60 min at room temperature. Finally, streptavidin-horseradish peroxidase (DAKO) was added and the slides were incubated for 30 min at room temperature. Diaminobenzidine (DAKO) was used as the chromogen, and color development was stopped by gently dipping slides in distilled water. A negative control treated with antibody diluents only was included with each set of slides. Two independent observers (L-YK, GNF) quantitatively evaluated expression by analyzing the tumors using high-power microscope fields (max: x40 objective lens and x10 eyepiece) of each specimen in the regions with the highest relative positive staining for that individual specimen. The observers examined each tumor in a blinded fashion and in duplicate. Each observer recorded the absolute number of cells with positive staining. The duplicate numbers were then averaged for the final number of cells with positive expression per specimen.
Immune effector assays
Splenocytes (2 × 107 cells/mL) from surviving mice treated with control, miR-124, and/or VEGF-4–1BB aptamer were incubated with 2 mM carboxy-fluorescein diacetate succinimidyl ester (CFSE, Invitrogen) in PBS at 25°C for 5 min. The CFSE-labeled splenocytes (5 × 105) were cultured in the presence of pre-coated (1 ug/mL anti-CD3/anti-CD28 in 500 uL of RPMI1640 medium) plates for T cell proliferation. The cells were cultured for 72 h in 48-well plates, and proliferation was assessed by the CFSE dilution profile of the labeled target cells acquired on a FACS Caliber (BD Biosciences, CA). In addition, the effector function of stimulated T cells of the miR-124/VEGF-4–1BB aptamer-treated mice was measured by intracellular cytokine production by stimulating cells for 6 h in the presence of 50 ng/mL PMA, 500 ng/mL ionomycin (Sigma-Aldrich) and 2 uM monensin (GolgiStopTM, BD Sciences). The cells were then incubated with an Fc-blocker and FITC-conjugated anti-CD4+ and APC-conjugated anti-CD8+ for surface staining, followed by intracellular staining using PE-conjugated anti-mouse IFNγ, PE-conjugated anti-mouse IL-2, or PE-conjugated anti-mouse TNF-α in the FIX/PERM buffers (BD PharMingen) according to the manufacturer's instructions.
Statistics
Unpaired, two-sided two sample t-tests were performed between individual treatment groups using GraphPad Prism V-5.00 (GraphPad Software Inc., La Jolla, CA). p values of less than or equal to 0.05 were considered statistically significant. The log-rank test for trend was applied to the survival curve to determine statistical significance.
Results
MiR-124 is synergistic with VEGF-targeted 4–1BB aptamer conjugate
We have previously demonstrated therapeutic efficacy of miR-124 in both immune competent clonotypic GL261 gliomas and in genetically engineered murine models (GEMMs) of heterogeneous high-grade glioma.Citation3 MiR-124 targets multiple nodes along the STAT3 pathway, but despite marked therapeutic effects, the animals still ultimately succumbed to tumor progression. In order to further potentiate the therapeutic effect of miR-124, we tested a combinational approach with VEGF-4–1BB aptamer conjugate that targets 4–1BB costimulation to the tumor lesion.Citation1 Since the GEMMs more closely recapitulate the human heterogeneous biology, we elected to screen the combinational approach in this more stringent murine model. The median survival time for the scramble control was 39.5 d, for miR-124 was 66.5 d, for VEGF-4–1BB was 65.5 d and for the combination of miRNA-124 + VEGF-4–1BB was 89.5 d (; p = 0.04). To further ascertain if the conjugation of the 4–1BB aptamer to VEGF was required to optimize the therapeutic benefit, we treated the GEMMs with the 4–1BB aptamer at the equivalency used in the conjugated aptamer, denoted as 1X. Although there was an increase in median survival to 42 d, this was not statistically different relative to untreated PBS controls (median survival = 34 d) (). We also evaluated whether increasing the dose of the 4–1BB aptamer, denoted 8X, could approximate the therapeutic effect of the 4–1BB mAb. Equivalency studies with the 4–1BB aptamer and the 4–1BB antibody demonstrated similar therapeutic results, with median survival times of 71 and 69 d, respectively. These results were not therapeutically different from those obtained using the VEGF-4–1BB aptamer alone in this model system, with a median survival time of 65.5 d; however, escalating the dose of the 4–1BB aptamer and 4–1BB mAbs has been shown to be associated with toxicity.Citation15
Figure 1. The combination of miR-124 with VEGF-4–1BB had an additive therapeutic effect in a genetically-engineered murine model (GEMM) of high-grade glioma. (A). Treatment schema of GEMM mice treated with either miR124, VEGF-4–1BB or the combination of both. (B). Graph of the Kaplan–Meier survival estimate demonstrates improved survival in miR-124 + VEGF-4–1BB-treated Ntv-a mice transfected with the RCAS-PDGFB and RCAS-STAT3 transgenes (n = 10 mice/group) compared with monotherapy (p = 0.04 by log-rank tests). Repeat of this experiment demonstrated similar findings with the combination treatment of VEGF-4–1BB and miRNA-124 resulting in a median survival time of 75.5 d (p = 0.0335 by log-rank test). (C). Treatment schema of GEMM mice treated with various amounts of 4–1BB aptamer versus 4–1BB antibody. (D). Graph of the Kaplan–Meier survival estimate demonstrates improved survival with the 4–1BB aptamer monotherapy, but only at concentrations (8X) equivalent to that of the 4–1BB antibody (n = 9 mice/group) (p = 0.0966 by log-rank tests). Even at optimal dosing, immune stimulation alone never achieved a similar level of therapeutic efficacy as was observed with the combination of immune stimulation and inhibition of tumor-mediated immune suppression.
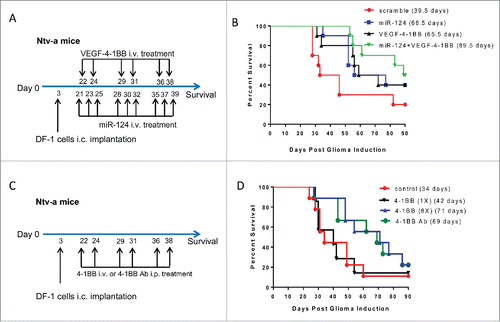
Heterogeneous VEGF glioma expression and therapeutic response
The VEGF-4–1BB aptamer was designed to provide costimulation within the microenvironment based on its co-localization to VEGF-expressing tumor areas. Previously, the VEGF-4–1BB aptamer was shown to demonstrate marked therapeutic effects in clonotypic models of malignancy with homogeneous VEGF expression. However, this is rarely the case within human glioblastomas, and thus the question arises as to whether VEGF-4–1BB can assert a therapeutic effect in models that have more heterogeneous expression, which more completely recapitulate human biology. The GEMMs of glioma demonstrate heterogeneous expression of VEGF—specifically as islands within the high-grade gliomas (). When these mice were treated with a miRNA scramble control, miR-124, VEGF-4–1BB, or miR-124 + VEGF-4–1BB, VEGF expression in the glioma microenvironment was not different among the treatment groups (), indicating that the differences in survival were not secondary to a VEGF expression bias.
Figure 2. Glioma biology in the setting of miR-124 and VEGF-4–1BB treatment. (A). Representative VEGF staining of a high-grade glioma induced in Ntv-a mice transfected with RCAS-PDGFB and RCAS-STAT3 transgenes demonstrates an island of expression (left panel bar scale r = 12.5 μm ; right panel bar scale = 100 μm). Arrows denote localized areas of positive VEGF staining. (B). Summarized analysis of the presence of VEGF in the tumors of mice treated with scramble control (n = 7), miR-124 (n = 9), VEGF-4–1BB (n = 7), or miR-124 + VEGF-4–1BB (n = 9). (C). Summary graph demonstrates the incidence of high- and low-grade gliomas on the basis of hematoxylin and eosin staining features of necrosis and neovascular proliferation in Ntv-a mice transfected with RCAS-PDGFB and RCAS-STAT3 transgenes and subsequently treated with scramble control (n = 9), miR-124 (n = 10), VEGF-4–1BB (n = 10), or the combination of miR-124 + VEGF-4–1BB (n = 10) from .
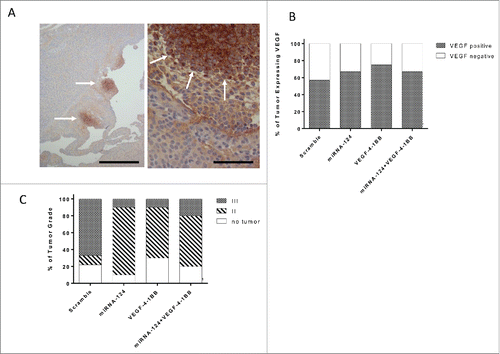
Immune modulatory influence of immune modulatory oligonucleotides on glioma grade
We have previously demonstrated that in the GEMMs of glioma that there is significant plasticity of glioma grade,Citation9 similar to malignant transformation seen in human glioma patients. The glioma grade plasticity has been previously documented in response to agents that target STAT3,Citation9,12 such as miRNA-124.Citation3 As such, we assessed glioma grade in each treatment group succumbing to the tumor to ascertain whether the immune modulatory agent had sculpted the malignancy. In the scramble-controlled cohort, high-grade gliomas predominated. As we previously described, high-grade malignant features were mostly absent in animals that had been treated with miRNA-124 who succumbed to death from infiltrating low-grade gliomas and hydrocephalus. Similarly, the relative incidence of low-grade gliomas in animals treated with the VEGF-4–1BB aptamers was also increased, but the two in combination did not alter further the glioma grade (). This indicates/suggests that the therapeutic effect observed with the combined approach was not secondary to an absolute eradication of the glioma or an alteration in the relative incidence of malignant transformation/progression.
miR-124 and VEGF-4–1BB in combination enhances immune trafficking to the glioma microenvironment
To further evaluate induced systemic immunological effector responses, we subsequently analyzed both the CD4+ and CD8+ compartments for proliferative and effector responses during the therapeutic window in the Ntv-A mice. miR-124 induced minimal CD4+ and CD8+ T cell proliferation, whereas the VEGF-4–1BB aptamer induced a more robust 26% and 13% increase in CD4+ and CD8+ proliferation, respectively; however, this was not further enhanced by the combination (data not shown). As monotherapies, both the VEGF-4–1BB aptamer and miR-124 enhanced TNF-α, IL-2, and IFNγ responses in the CD4+ and CD8+ immune compartments; however, an additive effect was observed with the combination only in the IFNγ effector responses. Recently, STAT3 has been shown to inhibit the accumulation of immune cells by the tumor,Citation16 by downregulation of the CXCR3/CXCL10 axis. To ascertain if there were any differences between the treatment groups at the time of death, the gliomas were subsequently stained for innate (F4/80) and adaptive (CD3) immune responses in the tumor microenvironment. We have previously shown that STAT3-targeting approaches reduce glioma infiltration of F4/80 macrophages, which are negative prognosticators for survival.Citation12 As anticipated,Citation4,17 there were 59% and 43% decreases in the glioma microenvironment of the tumor-supportive macrophage with the STAT3-targeting miR-124 and VEGF-4–1BB aptamer, respectively (; p = 0.0053 and 0.0661, respectively by two-sample t-tests). This was further decreased (to 71.4%) using the combination of miR-124 and VEGF-4–1BB compared with the scramble control (p = 0.0014 by two-sample t-tests, but not significant differently compared with monotherapy with either miR-124 treatment or VEGF-4–1BB aptamer treatment (). In contrast, the number of CD3 T cells significantly increased relative to the control by treatment with miR-124 (p = 0.0486 by two-sample t-tests), the VEGF-4–1BB aptamer (p = 0.0261 by two-sample t-tests), or the combination of miR-124 and the VEGF-4–1BB aptamer (p = 0.0132 by two-sample t-tests) (). Tregs were suppressed in gliomas treated with miR-124, VEGF-4–1BB, and the combination of miR-124 and VEGF-4–1BB, to a similar extent (data not shown).
Figure 3. Intratumoral immune responses of miR-124 and VEGF-4–1BB treatment. (A). Representative specimen from the brain of an Ntv-a mouse transfected with the RCAS-PDGFB and RCAS-STAT3 transgenes demonstrating F4/80 immune infiltration upon treatment with miR-124 + VEGF-4–1BB versus monotherapy (bar scale = 100 μm). (B). Summary graph of the treatment groups, including scramble control (n = 7), miR-124 (n = 9), VEGF-4–1BB (n = 7), and the combination of miR-124 + VEGF-4–1BB (n = 9); p = 0.0014 by unpaired, two-sided two sample t-tests. (C). Representative specimen from the brain of an Ntv-a mouse transfected with the RCAS-PDGFB and RCAS-STAT3 transgenes demonstrating CD3+ immune infiltration upon treatment with miR-124 + VEGF-4–1BB versus monotherapy (bar scale = 100 μm). (D). Summary graph of the treatment groups including scramble control (n = 6), miR-124 (n = 6), VEGF-4–1BB (n = 5), and the combination of miR-124 + VEGF-4–1BB (n = 5); p = 0.0132 by unpaired, two-sided two sample t-tests.
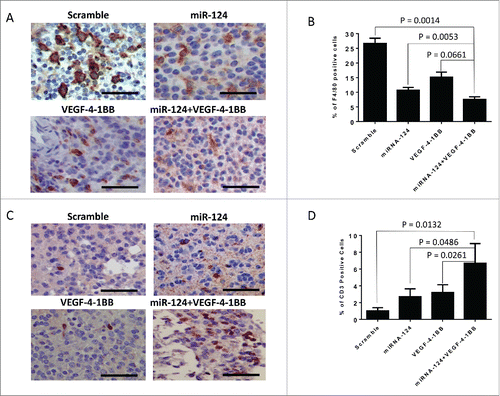
Discussion
High-grade malignant gliomas are noted for marked heterogeneity of both effector responses and immune suppressive mechanisms.Citation18 As such, it is unlikely that a single immune therapeutic strategy will assert a long-term durable response in most patients because of the plasticity and redundancy of mechanisms. Optimal antitumor immune responses require an antigenic target, immune activation, trafficking to the tumor microenvironment, and maintenance of effector function. Meaningful strides in the treatment of glioblastoma utilizing immunotherapy will probably require a multi-modality approach. In this manuscript, we show that a previously described immune therapeutic that targets immune suppression and inhibits effector function, miRNA-124,Citation3 has improved survival when used in combination with an immune stimulatory aptamer—VEGF-4–1BB. During our initial screening process, other co-stimulatory aptamers were tested for synergistic therapeutic activity withmiRNA-124, but they failed to reveal therapeutic synergy, indicating that the selection of combinations will need to be carefully considered and vetted. We additionally noted that the therapeutic efficacy is further potentiated when the 4–1BB aptamer is targeted to the glioma microenvironment, even if the target is heterogeneously expressed, as is VEGF. This is further supported by our finding of increased immune trafficking to the tumor microenvironment with the combination. We did determine if immune modulation impacted the grade of glioma since we have previously shown this to be the case for miR-124 Citation3 and that grade correlates with survival in these models.Citation12 Monotherapy with the VEGF-4–1BB aptamer also demonstrated an impact on the incidence of high-grade gliomas but this was not further potentiated with the combination.
We do not rule out the possibility that the combination may also affect the viability and/or activation status of various T cell subsets as a mechanism of synergistic activity. Furthermore, there may be immune stimulatory effects on dendritic cells in vivo and increased vascular permeability of tumor vasculature through the effects of VEGF-4–1BB on endothelial cells, which has not been excluded in this study. Typically, antibodies have been therapeutically utilized clinically as a means for modulating the immune system. The development and clinical manufacture of these therapeutics can be challenging and expensive. miRNAs and oligonucleotide-based aptamers offer an alternative platform that can bind their targets with specificity and avidity comparable with antibodies.Citation3,19 Unlike antibodies, both of these agents can be synthesized by a relatively simple and cost-effective cell-free chemical process. Ultimately, we envision that these therapeutics will advanced to clinical trials as combinatorial approaches, as we have described.
Disclosure of potential conflicts of interest
No potential conflicts of interest were disclosed.
Author contributions
Conception and design: EG, ABH. Development of methodology: L-Y K, GR, GC, EG, ABH. Acquisition of data: L-Y K, JW. Analysis and interpretation of data: L-Y K, GF, SZ. Writing, reviewing, and revision of manuscript: L-Y K, EG, ABH.
Acknowledgments
We offer special thanks to David M. Wildrick, Ph.D., Lamonne Crutcher, and Audria Patrick for their editorial and administrative support.
Funding
This study was supported by NIH grants P30 CA16672, CA1208113, K08 NS070928, and P50CA127001, and funds provided by the Ben and Catherine Ivy Foundation and the M.D. Anderson GBM Moonshot program.
References
- Pastor F, Kolonias D, McNamara JO, 2nd, Gilboa E. Targeting 4-1BB costimulation to disseminated tumor lesions with bi-specific oligonucleotide aptamers. Mol Ther 2011; 19:1878-86; PMID:21829171; http://dx.doi.org/10.1038/mt.2011.145
- Schrand B, Berezhnoy A, Brenneman R, Williams A, Levay A, Kong LY, Rao G, Zhou S, Heimberger AB, Gilboa E. Targeting 4-1BB costimulation to the tumor stroma with bispecific aptamer conjugates enhances the therapeutic index of tumor immunotherapy. Cancer Immunol Res 2014; 2:867-77; PMID:24938283; http://dx.doi.org/10.1158/2326-6066.CIR-14-0007
- Wei J, Wang F, Kong LY, Xu S, Doucette T, Ferguson SD, Yang Y, McEnery K, Jethwa K, Gjyshi O et al. miR-124 Inhibits STAT3 Signaling to Enhance T Cell-Mediated Immune Clearance of Glioma. Cancer Res 2013; 73:3913-26; PMID:23636127; http://dx.doi.org/10.1158/0008-5472.CAN-12-4318
- Hussain SF, Yang D, Suki D, Aldape K, Grimm E, Heimberger AB. The role of human glioma-infiltrating microglia/macrophages in mediating antitumor immune responses. Neuro Oncol 2006; 8:261-79; PMID:16775224; http://dx.doi.org/10.1215/15228517-2006-008
- Twyman-Saint Victor C, Rech AJ, Maity A, Rengan R, Pauken KE, Stelekati E, Benci JL, Xu B, Dada H, Odorizzi PM et al. Radiation and dual checkpoint blockade activate non-redundant immune mechanisms in cancer. Nature 2015; 520:373-7; PMID:25754329; http://dx.doi.org/10.1038/nature14292
- Holland EC, Varmus HE. Basic fibroblast growth factor induces cell migration and proliferation after glia-specific gene transfer in mice. Proc Natl Acad Sci U S A 1998; 95:1218-23; PMID:9448312; http://dx.doi.org/10.1073/pnas.95.3.1218
- Dai C, Celestino JC, Okada Y, Louis DN, Fuller GN, Holland EC. PDGF autocrine stimulation dedifferentiates cultured astrocytes and induces oligodendrogliomas and oligoastrocytomas from neural progenitors and astrocytes in vivo. Genes Dev 2001; 15:1913-25; PMID:11485986; http://dx.doi.org/10.1101/gad.903001
- Shih AH, Dai C, Hu X, Rosenblum MK, Koutcher JA, Holland EC. Dose-dependent effects of platelet-derived growth factor-B on glial tumorigenesis. Cancer Res 2004; 64:4783-9; PMID:15256447; http://dx.doi.org/10.1158/0008-5472.CAN-03-3831
- Doucette TA, Kong LY, Yang Y, Ferguson SD, Yang J, Wei J, Qiao W, Fuller GN, Bhat KP, Aldape K et al. Signal transducer and activator of transcription 3 promotes angiogenesis and drives malignant progression in glioma. Neuro Oncol 2012; 14:1136-45; PMID:22753228; http://dx.doi.org/10.1093/neuonc/nos139
- Berezhnoy A, Cstro I, Levay A, Malek TR, Gilboa E. Aptamer-targeted inhibition of mTOR in T cell enhances antitumor immunity. J Clin Invest 2014; 124(1):188-97; PMID:24292708; http://dx.doi.org/10.1172/JCI69856
- Ruckman J, Green LS, Beeson J, Waugh S, Gillette WL, Henninger DD, Claesson-Welsh L, Janjić N. 2′-Fluoropyrimidine RNA-based aptamers to the 165-amino acid form of vascular endothelial growth factor (VEGF165). Inhibition of receptor binding and VEGF-induced vascular permeability through interactions requiring the exon 7-encoded domain. J Biol Chem 1998; 273:20556-67; PMID:9685413; http://dx.doi.org/10.1074/jbc.
- Kong LY, Wu AS, Doucette T, Wei J, Priebe W, Fuller GN, Qiao W, Sawaya R, Rao G, Heimberger AB. Intratumoral mediated immunosuppression is prognostic in genetically engineered murine models of glioma and correlates to immunotherapeutic responses. Clin Cancer Res 2010; 16:5722-33; PMID:20921210; http://dx.doi.org/10.1158/1078-0432.CCR-10-1693
- Prinz M, Priller J. Microglia and brain macrophages in the molecular age: from origin to neuropsychiatric disease. Nat Rev Neurosci 2014; 15:300-12; PMID:24713688; http://dx.doi.org/10.1038/nrn3722
- Ye XZ, Xu SL, Xin YH, Yu SC, Ping YF, Chen L, Xiao HL, Wang B, Yi L, Wang QL et al. Tumor-associated microglia/macrophages enhance the invasion of glioma stem-like cells via TGF-beta1 signaling pathway. J Immunol 2012; 189:444-53; PMID:22664874; http://dx.doi.org/10.4049/jimmunol.1103248
- Schrand B, Berezhnoy A, Brenneman R, Williams A, Levay A, Gilboa E. Reducing toxicity of 4-1BB costimulation: targeting 4-1BB ligands to the tumor stroma with bi-specific aptamer conjugates. Oncoimmunology 2015; 4:e970918; PMID:25949891; http://dx.doi.org/10.4161/21624011.2014.970918
- Yue C, Shen S, Deng J, Priceman SJ, Li W, Huang A, Yu H. STAT3 in CD8+ T cells inhibits their tumor accumulation by down-regulating CXCR3/CXCL10 axis. Cancer Immunol Res 2015; 3(8):864-70; PMID:26025380; http://dx.doi.org/10.1158/2326-6066.CIR-15-0014
- Wu A, Wei J, Kong LY, Wang Y, Priebe W, Qiao W, Sawaya R, Heimberger AB. Glioma cancer stem cells induce immunosuppressive macrophages/microglia. Neuro Oncol 2010; 12:1113-25; PMID:20667896; http://dx.doi.org/10.1093/neuonc/noq082
- Doucette TA, Rao G, Rao A, Shen L, Aldape K, Wei J, Dziurzynski K, Gilbert M, Heimberger AB. Immune heterogeneity of glioblastoma subtypes: extrapolation from the cancer genome atlas. Cancer Immunol Res 2013; 1:112-22; PMID:24409449; http://dx.doi.org/10.1158/2326-6066.CIR-13-0028
- Keefe AD, Pai S, Ellington A. Aptamers as therapeutics. Nat Rev Drug Discov 2010; 9:537-50; PMID:20592747; http://dx.doi.org/10.1038/nrd3141