ABSTRACT
Neutrophils play a major role in cancer biology and both pro- and antitumoral functions of tumor-infiltrating neutrophils have been described. We have shown that tumors, by releasing G-CSF into the bloodstream, prime circulating neutrophils to form neutrophil extracellular traps (NETs) and we have detected the presence of NETs within the tumor microenvironment. Here, we report, using PAD4-deficient mice with a defect in neutrophil chromatin decondensation and NET formation, that the priming of neutrophils toward NETosis favors tumor growth. Interestingly, in a tumor model that does not release G-CSF and in which neutrophils are not primed for NETosis, PAD4-deficiency did not reduce tumor growth. However, supplying exogenous G-CSF to the wild-type (WT) host promoted intratumoral NETosis and tumor growth. Taken together, our results suggest that the priming of neutrophils for NETosis by the tumor or its environment leads to the accumulation of intratumoral NETs and a growth advantage to the tumor. Our work unveiled a pro-tumoral role for NETs which strengthens their potential as a new target in the fight against cancer.
KEYWORDS:
Introduction
Tumor-infiltrating neutrophils contribute to a significant proportion of the inflammatory component of the tumor but their role in cancer biology is still unclear. Although high numbers of blood and intratumoral neutrophils in a variety of solid tumors have been reported to predict poor clinical outcome,Citation1,2 both pro- and antitumoral functions are attributed to tumor-associated neutrophils.
In the last decade, the identification of NETs as a new mechanism to trap pathogens has given us a new perspective on neutrophils.Citation3 NETosis is an active process leading to the release of extracellular chromatin containing not only DNA and histones but also granular proteins. NETs have been characterized as proinflammatory, procoagulant and associated with a variety of thrombotic diseases.Citation4 The release of chromatin from neutrophils is driven by peptidylarginine deiminase 4 (PAD4), which in activated neutrophils translocates to the nucleus and through the citrullination of histones induces chromatin decondensation that is followed by chromatin release.Citation5
PAD4 is abundantly expressed in neutrophils, but is also upregulated in many cancers where it negatively regulates tumor suppressor genes of the p53 pathway and is thus involved in tumorigenesis.Citation6 We previously showed that cancer, through the release of G-CSF, can prime neutrophils toward NETosis and identified NETs as a new player in cancer-associated thrombosis.Citation7 NETs could play a role in many steps of tumor progression and their release could enhance tumor growth/invasion, or induce cytotoxicity.Citation8 Using PAD4-deficient mice, which fail to release NETs,Citation9,10 we now address the role of the host PAD4/NETs in PAD4 WT tumors and report that the systemic priming of neutrophils induces the accumulation of NETting neutrophils in the tumor microenvironment. Moreover, the formation of NETs in the tumor environment promotes cancer growth. Our results indicate that prevention of NET production could be used as a new strategy to reduce tumor growth and suggest that drugs or conditions that promote NETosis would have the opposite effect.
Results
Presence of NETs increases Lewis lung carcinoma (LLC) tumor growth
NETs have been observed in both murine and human tumors: sarcomas, pancreatic adenocarcinoma and LLC lung carcinomas Citation11-14 and have been shown to promote metastasis following septicemia in mice.Citation15 To evaluate if the presence of PAD4/NETs in the host affects primary tumor growth, we compared tumor progression in WT mice to PAD4-deficient mice whose neutrophils are defective in NET formation. Subcutaneous injection of LLC cells revealed an approximately 35% reduction in tumor growth in PAD4−/− hosts compared to WT mice (). Confocal microscopy confirmed the presence of neutrophil-rich areas containing neutrophils with citrullinated histone H3 (H3Cit) and NETs in the tumors obtained from the WT mice, whereas no H3Cit signal or NETs could be observed in the tumors from PAD4−/− mice (). Moreover, tumor analysis by Western blot showed an increasing accumulation of neutrophils that was similar in both genotypes as the tumor progressed. The increase in neutrophil infiltration was associated with an upregulation of H3Cit but only in the tumors from WT mice (). H3Cit was not detected in the PAD4−/− hosts. This shows that neutrophils, rather than cancer cells, are the primary source of H3Cit in these tumors and suggests that the presence of NETs in the tumor promotes tumor growth. To control for differences in neutrophil accumulation between the genotypes, we quantified the amount of neutrophils by Western blot () and flow cytometry (). A similar amount of intratumoral neutrophils was observed in WT and PAD4−/− tumors with both techniques.
Figure 1. PAD4-deficiency with impaired NETosis reduces LLC tumor growth. LLC cells were injected in 17 WT or 16 PAD4−/− mice and tumors were allowed to grow. (A) Tumor growth was evaluated until day 17 post-implantation, unless mice were moribund or tumors necrotic and mice were sacrificed. A reduction in tumor size and weight was observed in PAD4−/− compared to WT mice. *p <0.05 (B) Confocal analysis of tumor sections revealed the presence of NETs in tumors from WT mice, as identified by extracellular citrullinated histone H3 (H3Cit) and diffused DNA staining (arrows), which was absent in the tumors from PAD4−/− hosts. (C) Western blot analysis of tumor lysates obtained on indicated days post-implantation showed neutrophil accumulation in both genotypes and increasing H3Cit content only in the tumors from WT mice as the tumors progressed. At day 17 post-implantation, a similar amount of Ly6G was observed in the tumor lysate of both genotypes (n = 4–6; p >0.05). (D) FACS analysis of digested tumors also revealed a similar amount of neutrophils in both genotypes (n = 4).
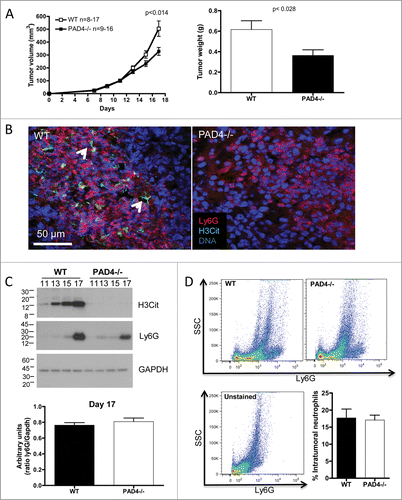
B16 melanoma growth is not affected by the host's PAD4-deficiency
To examine whether NETs modulate the growth of different types of solid tumors, we evaluated the growth of B16 melanoma tumors in WT and PAD4−/− mice. To our surprise, the B16 tumor growth after subcutaneous injection in PAD4−/− mice was indistinguishable from that observed in WT mice (). Interestingly, confocal microscopy unveiled the presence of very few NETs in the neutrophil-rich area of B16 tumors from WT mice (). Moreover, the comparison of similar sized 17-d-old LLC and B16 tumors showed a comparable recruitment of neutrophils in both tumor types but a much greater amount of H3Cit was observed in the LLC tumors () suggesting that the LLC tumors promote more NET induction than B16 tumors. Since PAD4 has been shown to be overexpressed in a variety of tumors,Citation16,17 we assessed whether the low expression of H3Cit observed in B16 tumors could be due to citrullination of histone H3 in the cancer cells. PAD4 mRNA analysis revealed the expression of higher levels of PAD4 in B16 tumor cells compared to LLC cells (). Moreover, H3Cit was found in B16 tumors from PAD4-deficient mice whereas no H3Cit could be observed in LLC tumors from these mice (). These results suggest that the small accumulation of H3Cit in B16 tumors is likely due to the cancer cells themselves. Together, our results indicate that a tumor-specific environment coordinates the predisposition of neutrophils to NET formation and that NET presence favors tumor growth.
Figure 2. Host PAD4-deficiency does not affect the growth of B16 melanoma. B16F10 cells were injected in 20 WT and 22 PAD4−/− mice and tumors were allowed to grow. (A) Tumor growth was evaluated until day 17 post-implantation, unless mice were moribund or tumors necrotic and mice were sacrificed. No difference in tumor growth was observed between the genotypes (p >0.05). (B) Confocal analysis of tumor sections revealed only rare NETs in tumors from WT mice (arrow), as observed by extracellular H3Cit, which was absent in the tumor from PAD4−/− hosts. (C) At day 17 post-implantation, LLC and B16 tumors in WT animals reached similar weights. Comparative analysis of their lysates by Western blot showed similar neutrophil infiltration (n = 4) but a much higher amount of H3Cit in the LLC tumors than in the B16 tumors. (D) B16F10 and LLC cells were assessed for PAD4 expression by RT-PCR. B16 cells showed higher expression of PAD4. (E) Western blot analysis of 17-d tumors from PAD4-deficient mice showed H3Cit expression in B16 tumors whereas no H3Cit can be observed in LLC tumors.
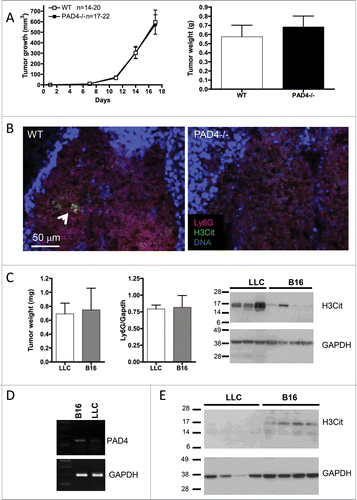
LLC and B16 melanoma tumors exhibit different neutrophil phenotypes
Since only a small amount of H3Cit and NETs could be observed within the neutrophilic areas present in B16 compared to LLC tumors from WT mice, we hypothesized that the different types of cancer must induce different neutrophil phenotypes. Analysis of tumor-infiltrating neutrophils by flow cytometry revealed the presence of more activated CD11bhigh neutrophils in the LLC compared to B16 tumors (). This result again suggests that LLC tumors generate an environment different from B16 tumors, which affects neutrophils' maturation and function.
Figure 3. Neutrophils from LLC and B16 tumor-bearing WT mice have a different phenotype. LLC or B16F10 cells were injected and tumors were allowed to grow. (A) At day 17 post-implantation, tumors were digested for FACS analysis. CD11b and Ly6G staining revealed a different phenotype of the tumor-infiltrating neutrophils with more CD11bhigh neutrophils in the LLC tumors (n = 6). (B) Plasma analysis showed upregulation of G-CSF levels in LLC tumor-bearing mice, both WT and PAD4−/−, as the tumor grew whereas G-CSF levels remained low in all B16 tumor-bearing mice (n = 4–9). (C) At day 16 and 17 post-implantation, neutrophils from LLC and B16 tumor-bearing WT mice were isolated. Wright–Giemsa staining revealed a typical neutrophil nuclear morphology in mice bearing both type of tumors (left). However, more neutrophils isolated from LLC tumor-bearing mice produced NETs compared to neutrophils isolated from B16 tumor-bearing mice, under both unstimulated and LPS-stimulated conditions (right, n = 4;* p <0.05,**p <0.01).
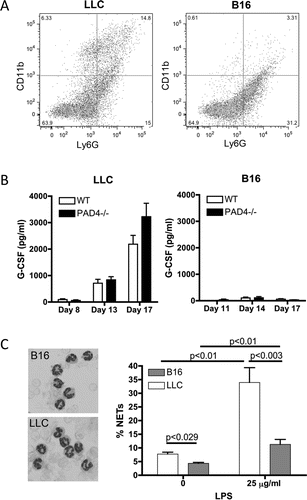
We have previously shown that several types of tumors, including LLC, release G-CSF into the circulation, which increases neutrophil number and primes them toward NETosis.Citation7 We therefore evaluated the presence of G-CSF in B16 melanomas compared to LLC tumor-bearing mice. Plasma analysis showed only a minor amount of G-CSF in the B16 tumor-bearing mice and the level did not increase with tumor growth (). In contrast, LLC tumor-bearing mice presented a continuous increase in plasma G-CSF over time. No difference in G-CSF levels was found between the two genotypes of mice. Moreover, 14.17 ± 0.88 pg/mL was found in the supernatant of LLC cells in culture for 48 h whereas none was detect in B16 cells indicating that the LLC tumor cells themselves are responsible for the accumulation of G-CSF in the circulation. Peripheral blood neutrophils isolated from LLC tumor-bearing mice had a higher propensity for NET formation, with and without LPS stimulation, than neutrophils from B16 tumor-bearing mice (). This indicates that the systemic G-CSF release by the LLC tumor favors the priming of neutrophils and intratumoral NETosis.
Priming of neutrophils toward NETosis by G-CSF promotes the formation of NETs in the B16 tumors with concomitant increase in tumor growth
To evaluate if the priming of neutrophils toward NET formation by G-CSF would be sufficient to induce NETosis in the tumor and promote tumor growth, we treated B16 tumor-bearing WT and PAD4−/− mice with G-CSF. We have previously shown that G-CSF treatment of non-tumor-bearing WT mice was sufficient to prime neutrophils toward NETosis.Citation7 B16 tumor cells were injected subcutaneously and allowed to grow. Strikingly, after daily administration of G-CSF, B16 tumors in WT mice grew significantly faster than the tumors in PAD4−/− mice (). As expected, G-CSF treatment also increased peripheral neutrophil counts to a similar extent in both genotypes. Tumor analysis revealed an increase in CD11bhigh tumor-infiltrating neutrophils () and a higher amount of H3Cit in tumors of mice treated with G-CSF (). Since the increase in H3Cit was observed only in tumors from WT mice and not in tumors from PAD4−/− mice, the H3Cit likely represents accumulation of primed neutrophils/NETs in the tumors. A high amount of H3Cit staining was also observed in neutrophil-rich areas of B16 tumors from WT mice only after the G-CSF treatment (). Taken together, our results indicate that priming of neutrophils toward NETosis favors the accumulation of NETting neutrophils in the tumor microenvironment and that the priming promotes tumor growth.
Figure 4. G-CSF treatment induces intratumoral accumulation of NETing neutrophils and promotes B16 tumor growth in WT but not PAD4−/− mice. B16F10 cells were injected in 11 WT and 12 PAD4−/− mice and tumors were allowed to grow. At day 9 post-implantation, when tumors reached 30–50 mm3, daily G-CSF treatment was initiated and tumor growth was monitored. Tumor growth was evaluated until day 17 post-implantation, unless mice were moribund or tumors necrotic and mice were sacrificed. (A) G-CSF treatment of B16 tumor-bearing mice increases tumor growth in WT mice compared to PAD4−/− mice. As the initial volume of the tumors at the beginning of the treatment varies, data are presented as the ratio of the tumor volume at the indicated day (V1) compared to the volume at the initiation of G-CSF treatment (V0). The curves are compared by a non-linear regression analysis (p = 0.008). Neutrophil counts were increased by G-CSF treatment but no significant difference in the number of circulating neutrophils was observed between both genotypes (n = 9–10). (B) FACS analysis of the 17-d digested tumors from WT mice showed an increased percentage of CD11bhigh neutrophils in the B16 tumors when the tumor-bearing WT mice were treated with G-CSF. This percentage was similar to that seen in LLC tumors from untreated mice (n = 5–6). (C) Western blot showed increased H3Cit in 17-d tumor lysate of B16 tumors from WT mice treated with G-CSF than from untreated mice (left) whereas this was not observed in PAD4−/− mice (right). (D) Confocal microscopy confirmed the presence of higher amounts of H3Cit and NETs in the B16 tumors from WT mice treated with G-CSF that was absent in B16 tumors from PAD4−/− mice similarly treated.
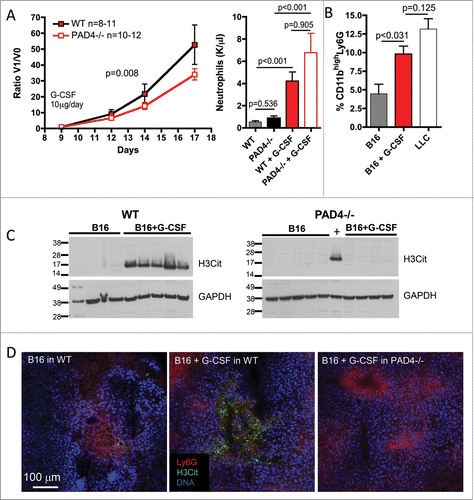
Discussion
We show that a cancer that induces the accumulation of NETing neutrophils in the tumor promotes its own growth. Moreover, we observed that the systemic priming of neutrophils toward NETosis by G-CSF alone is sufficient to induce the accumulation of NETs in an established low-NET tumor and enhance its growth. Our work unveils a novel pro-tumoral role of neutrophils i.e., formation of NETs.
Recently, we observed NETs in LLC tumors.Citation12,13 NETs have also been observed in human Ewing sarcomasCitation11 and pancreatic adenocarcinoma.Citation14 Interestingly, LLC tumor, Ewing sarcomas and pancreatic carcinomas have all been reported to express G-CSF.Citation7,18,19 Moreover, pancreatic carcinomas are associated with myeloid systemic and intratumoral accumulations.Citation20 In cancer patients, increase in neutrophil count and elevated blood G-CSF have been frequently observed and associated with poor outcome.Citation2,21 The systemic activation of neutrophils and neutrophilia may also initiate cancer-associated thrombosis as demonstrated in mice.Citation7 Importantly, our results demonstrate that G-CSF does not have to be locally produced in the tumor to prime neutrophils toward NETosis. Indeed, the systemic neutrophil priming following G-CSF infusion in the B16 melanoma model results in the accumulation of NETs in the tumor and increases tumor growth. This suggests that a systemic increase in G-CSF, such as upon infection or trauma, for example, could prime neutrophils toward NETosis. Moreover, besides cancer, neutrophils can be primed under many other conditions, such as hypoxia, and by many inflammatory diseases producing reactive oxygen species (ROS) or activating cytokines such as diabetes.Citation22 These conditions may therefore increase tumor growth, even if the cancer itself did not prime the neutrophils. In addition, the tumor environment may produce other factors that attract neutrophils and induce NETosis locally independently of G-CSF. Indeed, tumors may secrete a wide range of cytokines and chemokines such as TNF-α, interleukin-1, and CXCL2 that attract neutrophils and contribute to intratumoral NETosis.Citation21,23,24 Moreover, tumors are prothrombotic and the interaction of intratumoral neutrophils with the activated endothelium or platelets via P-selectin would also induce NETosis.Citation25 Our work and that of others Citation15 indicates that a closer monitoring of neutrophil counts and activation and a deeper understanding of the tumor environment and the factors that drive NETosis should be of prime importance in the fight against cancer.
The mechanism(s) through which NETing neutrophils/NETs provide a growth advantage to the tumor remains to be elucidated. NETs are procoagulantCitation26 and could therefore promote tumor growth through local thrombin generation.Citation27 The NETting process could also have a predominant role in the generation of a beneficial extracellular matrix by delivering proteinases and could also favor the release of inflammatory and angiogenic factors. For example, NETs have been shown to contain and activate matrix metalloproteinases,Citation28 preserve the enzymatic activity of elastaseCitation29 and recruit platelets,Citation30 important cells for angiogenesis.Citation31 Neutrophils were described to suppress the cytotoxic CD8+ antitumoral response, which is associated with the generation of ROS.Citation32 ROS are inducers of NETs and thus NETs could be implicated. Interestingly, it has been shown that neutrophils from chronic granulomatous disease patients, which harbor NADPH oxidase mutations, and myeloperoxidase (MPO)-deficient neutrophils fail to suppress lymphocyte function Citation33 and also do not make NETs.Citation34-36 Thus, NETs likely play a role in several aspects of tumor biology.
Our work shows a tumor-promoting effect of NETs in mice at a late stage of tumor development. It has been shown that the neutrophil phenotype evolves with tumor progression. Their presence appears mostly at the periphery of the tumor at early stages and they are found to be scattered between cancer cells later on, where they acquire a pro-tumoral phenotype.Citation37 It is therefore possible that as the tumor progresses and more cytokines are produced, more neutrophils are attracted and accumulated, leading to an increase in intratumoral NETs, favoring tumor growth. The presence of intravascular NETs in a model of sepsis was shown to increase experimental metastasis.Citation15 Since these observations rely on syngeneic tumor cell transplant or experimental metastasis models, it would be of interest to evaluate the effects of neutrophils/NETs in spontaneous tumor models.
Our results clearly show a substantial amount of NETs in the tumor and their absence in the PAD4-deficient mice. PAD4 is mostly expressed in neutrophils and to a lesser extent in other white blood cells.Citation6 Although the effect of G-CSF observed in our study is likely due to neutrophils, the implication of the effect of PAD4 from other white blood cells on tumor growth cannot be excluded. The development of neutrophil-specific PAD4-deficient mice would further extend our knowledge of the effects of NETs in cancer. We also believe that further development and testing of PAD4-specific inhibitorsCitation38 in mouse cancer models would be of prime importance.
In conclusion, our study has revealed a new tumor-promoting activity of neutrophils mediated by PAD4/NETs and may explain the poor prognosis associated with neutrophil accumulation within the tumor environment. We have previously shown that cancers can prime neutrophils toward NETosis, which induces thrombosis.Citation7 Here, we show that NETs also promote tumor growth. Taken together, these findings in mice suggest deleterious effects of NETs in cancer patients. We propose that the prevention of NET formation with PAD4 inhibitors or enhancement of NET clearance could contribute a new facet to our current treatments of cancer.
Methods
Cell lines and reagents
The LLC and B16F10 (B16) cell lines were purchased from ATCC and maintained in high-glucose Dulbecco's Modified Eagle's Medium (DMEM) + L-GlutaMAX-1 [supplemented with 10% (v/v) FCS, 10 mM HEPES buffer]. LLC and B16F10 cells originated from C57BL/6 mice. All cell lines were obtained from and characterized by ATCC according to the cell line authentication testing (growth curve analysis; mycoplasma, bacteria, and fungi contamination; DNA profiling; and species confirmation) and were used within 6 mo after resuscitation. All cell culture products were purchased from Life Technologies/Invitrogen.
Animals
All animal procedures were performed using 6- to 8-week-old C57BL/6 female mice (The Jackson Laboratory). PAD4−/− miceCitation9 have been backcrossed to C57BL/6J for more than seven generations. All experimental procedures involving mice were approved by the Institutional Animal Care and Use Committee of Boston Children's Hospital.
Induction of solid tumors
LLC (5 × 105) or B16F10 (2 × 105) cells were inoculated subcutaneously in the right flank. The tumor volume was calculated using the formula V = lw2 × 0.4 where l is the length and w the width. Mice were sacrificed at the indicated time or when tumors were ulcerated or if moribund. For G-CSF treatment, only mice bearing 30–50 mm3 tumors (day 9) were subcutaneously injected between the scapulae with 10 μg of recombinant human G-CSF (Neupogen®, Amgen) daily. Blood was collected using EDTA-coated capillaries and analyzed with a Hemavet hematology analyzer (Drew Scientific). At sacrifice, blood was collected into 3.2% sodium citrate, centrifuged at 6,000 rpm for plasma collection and centrifuged again at 12000 rpm to remove any contaminating cells.
Confocal microscopy
Tumors were harvested from sacrificed animals and embedded in OCT. Cryosections were fixed in zinc fixative (100 mM Tris-HCl, 37 mM zinc chloride, 23 mM zinc acetate, 3.2 mM calcium acetate) and permeabilized. 40 µm sections were incubated with antibodies against H3Cit (Abcam) and Ly6G (BD Biosciences) and then Alexa Fluor-conjugated secondary antibodies (Invitrogen) counterstained with Hoechst-33342. Images were acquired with Olympus Fluoview software using the Olympus IX 81 confocal microscope.
Western blot analysis
Equal amounts of protein per sample were analyzed on a 4–20% SDS-PAGE gel and transferred onto Immobilon PVDF membranes using a Mini-Trans Blot Electrophoretic Transfer Cell System (Bio-Rad Laboratories). The membranes were blocked and probed with rat anti-Ly6G (BD PharMingen), rabbit anti-histone H3 (citrulline 2, 8, 17) (Abcam) or GAPDH antibodies (Life Technology) followed by horseradish peroxidase-conjugated anti-rabbit, anti-rat or anti-mouse IgG (Bio-Rad Laboratories). Detection was carried out with a Pierce ECL Western Blotting Substrate (Thermo Scientific). Quantifications were executed using Image J software.
Flow cytometry analysis
The tumor was dissected into approximately 2 mm fragments followed by agitation in 1 mg/mL collagenase (Invitrogen), 100 µg/mL hyaluronidase (Sigma, St Louis, MO), and 20 mg/mL DNase (Sigma) in RPMA for 2–3 h at 37 degrees. The digest was filtered through 100 µm nylon mesh to remove macroscopic debris, and resuspended in PBS. Cells were stained with anti-Ly6G and anti-CD11b antibodies and assessed by flow cytometry using a FACSCanto II (BD Biosciences) and analyzed using FlowJo software.
RNA isolation and semiquantitative PCR
Total RNA was isolated from isolated peripheral blood neutrophils using Trizol reagent according to the manufacturer's instructions (Invitrogen, Inc.). Two micrograms of total RNA was reverse-transcribed using the Omniscript reverse transcriptase (Qiagen) and amplified with Hot Start Taq (Qiagen) using the following conditions: an initial 15 min activation step at 95°C, 94°C for 1 min, 55°C for 1 min, and 72°C for 1 min, followed by a final extension step at 72°C for 10 min. The primers used for PCR amplification are (5′-GGCTACACAACCTTCGGCAT-3′) for sense murine PAD4 and (5′-GCTGCTTTCACCTGTAGGGT-3′) for antisense, (5′-CAAAGTTGTCATGGATGACC-3′) for sense GAPDH and (5′-CCATGGAGAAGGATGGGG-3′) for antisense. Thirty-five cycles of amplification were done in a thermal cycler (RoboCycler Gradient 96, Stratagene). PCR assays using equal amounts of RNAs were reverse-transcribed and amplified for 30 and 35 cycles to confirm that the amplification was in the linear range. PCR products were analyzed by electrophoresis on 1.8% agarose gels using ethidium bromide staining and UV illumination.
Peripheral blood neutrophil isolation and NETs induction
Mice were exsanguinated into PBS containing 1% BSA and 15 mM EDTA. Neutrophils were isolated and NET formation was evaluated as previously described.Citation7 Briefly, blood cells were layered onto a Percoll gradient followed by red blood cell hemolysis. Isolated peripheral blood neutrophils were seeded into 96-wells before stimulation with lipopolysaccharides (LPS) (from Klebsiella pneumoniae). DNA was stained with Hoechst-33342 (Invitrogen) and cells were fixed with 2% paraformaldehyde before visualization using an epifluorescent Axiovert microscope (Zeiss). NETs were counted from six different fields in triplicate wells and expressed as a percentage of NET-forming cells per total number of cells in the field.
Quantification of G-CSF
1 × 106 LLC or B16F10 cells were cultured in their respective media for 48 h. Plasma and cell culture supernatant G-CSF was determined using the Quantikine mouse G-CSF ELISA (R&D Systems) according to the manufacturer's instructions. The sensitivity of the kit is 5 pg/mL.
Statistical analysis
Data are represented as means ± SEM and were analyzed by a two-sided Mann–Whitney test performed between groups. Non-linear curve fit comparison was done between groups to compare tumor growth following treatment. All p values were considered significant below 0.05.
Disclosure of potential conflicts of interest
No potential conflicts of interest were disclosed.
Acknowledgment
We thank Stephen Cifuni for his assistance and help with the G-CSF studies.
Funding
This work was supported by the National Heart, Lung, and Blood Institute of the National Institutes of Health grant R01HL102101 (to D.D.W.).
References
- Dumitru CA, Lang S, Brandau S. Modulation of neutrophil granulocytes in the tumor microenvironment: mechanisms and consequences for tumor progression. Seminars Cancer Biol 2013; 23:141-8; http://dx.doi.org/10.1016/j.semcancer.2013.02.005
- Shen M, Hu P, Donskov F, Wang G, Liu Q, Du J. Tumor-associated neutrophils as a new prognostic factor in cancer: a systematic review and meta-analysis. Plos One 2014; 9:e98259; PMID:24906014; http://dx.doi.org/10.1371/journal.pone.0098259
- Brinkmann V, Reichard U, Goosmann C, Fauler B, Uhlemann Y, Weiss DS, Weinrauch Y, Zychlinsky A. Neutrophil extracellular traps kill bacteria. Science 2004; 303:1532-5; PMID:15001782; http://dx.doi.org/10.1126/science.1092385
- Martinod K, Wagner DD. Thrombosis: tangled up in NETs. Blood 2014; 123:2768-76; PMID:24366358; http://dx.doi.org/10.1182/blood-2013-10-463646
- Wang Y, Li M, Stadler S, Correll S, Li P, Wang D, Hayama R, Leonelli L, Han H, Grigoryev SA et al. Histone hypercitrullination mediates chromatin decondensation and neutrophil extracellular trap formation. J Cell Biol 2009; 184:205-13; PMID:19153223; http://dx.doi.org/10.1083/jcb.200806072
- Wang S, Wang Y. Peptidylarginine deiminases in citrullination, gene regulation, health and pathogenesis. Biochim Biophys Acta 2013; 1829:1126-35; PMID:23860259; http://dx.doi.org/10.1016/j.bbagrm.2013.07.003
- Demers M, Krause DS, Schatzberg D, Martinod K, Voorhees JR, Fuchs TA, Scadden DT, Wagner DD. Cancers predispose neutrophils to release extracellular DNA traps that contribute to cancer-associated thrombosis. Proc Natl Acad Sci U S A 2012; 109:13076-81; PMID:22826226; http://dx.doi.org/10.1073/pnas.1200419109
- Demers M, Wagner DD. Neutrophil extracellular traps: A new link to cancer-associated thrombosis and potential implications for tumor progression. Oncoimmunol 2013; 2:e22946; PMID:23526174; http://dx.doi.org/10.4161/onci.22946
- Li P, Li M, Lindberg MR, Kennett MJ, Xiong N, Wang Y. PAD4 is essential for antibacterial innate immunity mediated by neutrophil extracellular traps. J Exp Med 2010; 207:1853-62; PMID:20733033; http://dx.doi.org/10.1084/jem.20100239
- Martinod K, Demers M, Fuchs TA, Wong SL, Brill A, Gallant M, Hu J, Wang Y, Wagner DD. Neutrophil histone modification by peptidylarginine deiminase 4 is critical for deep vein thrombosis in mice. Proc Natl Acad Sci U S A 2013; 110:8674-9; PMID:23650392; http://dx.doi.org/10.1073/pnas.1301059110
- Berger-Achituv S, Brinkmann V, Abed UA, Kuhn LI, Ben-Ezra J, Elhasid R, Zychlinsky A. A proposed role for neutrophil extracellular traps in cancer immunoediting. Front Immunol 2013; 4:48; PMID:23508552; http://dx.doi.org/10.3389/fimmu.2013.00048
- Demers M, Wagner DD. NETosis: a new factor in tumor progression and cancer-associated thrombosis. Semin Thromb Hemost 2014; 40:277-83; PMID:24590420; http://dx.doi.org/10.1055/s-0034-1370765
- Ho-Tin-Noe B, Carbo C, Demers M, Cifuni SM, Goerge T, Wagner DD. Innate immune cells induce hemorrhage in tumors during thrombocytopenia. Am J Pathol 2009; 175:1699-708; PMID:19729481; http://dx.doi.org/10.2353/ajpath.2009.090460
- Boone BA, Orlichenko L, Schapiro NE, Loughran P, Gianfrate GC, Ellis JT, Singhi AD, Kang R, Tang D, Lotze MT et al. The receptor for advanced glycation end products (RAGE) enhances autophagy and neutrophil extracellular traps in pancreatic cancer. Cancer Gene Therapy 2015; 22(6):326-34; PMID:25908451
- Cools-Lartigue J, Spicer J, McDonald B, Gowing S, Chow S, Giannias B, Bourdeau F, Kubes P, Ferri L. Neutrophil extracellular traps sequester circulating tumor cells and promote metastasis. J Clin Invest 2013; 123(8):3446-58; PMID:23863628; http://dx.doi.org/10.1172/JCI67484
- Chang X, Han J. Expression of peptidylarginine deiminase type 4 (PAD4) in various tumors. Mol Carcinogenesis 2006; 45:183-96; PMID:16355400; http://dx.doi.org/10.1002/mc.20169
- Chang X, Han J, Pang L, Zhao Y, Yang Y, Shen Z. Increased PADI4 expression in blood and tissues of patients with malignant tumors. BMC Cancer 2009; 9:40; PMID:19183436; http://dx.doi.org/10.1186/1471-2407-9-40
- Morales-Arias J, Meyers PA, Bolontrade MF, Rodriguez N, Zhou Z, Reddy K, Chou AJ, Koshkina NV, Kleinerman ES. Expression of granulocyte-colony-stimulating factor and its receptor in human Ewing sarcoma cells and patient tumor specimens: potential consequences of granulocyte-colony-stimulating factor administration. Cancer 2007; 110:1568-77; PMID:17694551; http://dx.doi.org/10.1002/cncr.22964
- Joshita S, Nakazawa K, Sugiyama Y, Kamijo A, Matsubayashi K, Miyabayashi H, Furuta K, Kitano K, Kawa S. Granulocyte-colony stimulating factor-producing pancreatic adenosquamous carcinoma showing aggressive clinical course. Intern Med 2009; 48:687-91; PMID:19420814; http://dx.doi.org/10.2169/internalmedicine.48.1900
- Khaled YS, Ammori BJ, Elkord E. Increased levels of granulocytic myeloid-derived suppressor cells in peripheral blood and tumour tissue of pancreatic cancer patients. J Immunol Res 2014; 2014:879897; PMID:24741628; http://dx.doi.org/10.1155/2014/879897
- Sionov RV, Fridlender ZG, Granot Z. The Multifaceted Roles Neutrophils Play in the Tumor Microenvironment. Cancer Microenvironment 2014; 8(3):125-58; PMID:24895166; http://dx.doi.org/10.1007/s12307-014-0147-5
- Wong SL, Demers M, Martinod K, Gallant M, Wang Y, Goldfine AB, Kahn CR, Wagner DD. Diabetes primes neutrophils to undergo NETosis, which impairs wound healing. Nat Med 2015; 21:815-9; PMID:26076037; http://dx.doi.org/10.1038/nm.3887
- Keshari RS, Jyoti A, Dubey M, Kothari N, Kohli M, Bogra J, Barthwal MK, Dikshit M. Cytokines induced neutrophil extracellular traps formation: implication for the inflammatory disease condition. PloS One 2012; 7:e48111; PMID:23110185; http://dx.doi.org/10.1371/journal.pone.0048111
- Marcos V, Zhou Z, Yildirim AO, Bohla A, Hector A, Vitkov L, Wiedenbauer EM, Krautgartner WD, Stoiber W, Belohradsky BH et al. CXCR2 mediates NADPH oxidase-independent neutrophil extracellular trap formation in cystic fibrosis airway inflammation. Nat Med 2010; 16:1018-23; PMID:20818377; http://dx.doi.org/10.1038/nm.2209
- Etulain J, Martinod K, Wong SL, Cifuni SM, Schattner M, Wagner DD. P-selectin promotes neutrophil extracellular trap formation in mice. Blood 2015; 126:242-6; PMID:25979951; http://dx.doi.org/10.1182/blood-2015-01-624023
- Massberg S, Grahl L, von Bruehl ML, Manukyan D, Pfeiler S, Goosmann C, Brinkmann V, Lorenz M, Bidzhekov K, Khandagale AB et al. Reciprocal coupling of coagulation and innate immunity via neutrophil serine proteases. Nat Med 2010; 16:887-96; PMID:20676107; http://dx.doi.org/10.1038/nm.2184
- Green D, Karpatkin S. Role of thrombin as a tumor growth factor. Cell Cycle 2010; 9:656-61; PMID:20190559; http://dx.doi.org/10.4161/cc.9.4.10729
- Carmona-Rivera C, Zhao W, Yalavarthi S, Kaplan MJ. Neutrophil extracellular traps induce endothelial dysfunction in systemic lupus erythematosus through the activation of matrix metalloproteinase-2. Annals Rheumatic Dis 2015; 74(7):1417-24; PMID:24570026; http://dx.doi.org/10.1136/annrheumdis-2013-204837
- Kolaczkowska E, Jenne CN, Surewaard BG, Thanabalasuriar A, Lee WY, Sanz MJ, Mowen K, Opdenakker G, Kubes P. Molecular mechanisms of NET formation and degradation revealed by intravital imaging in the liver vasculature. Nat Commun 2015; 6:6673; PMID:25809117; http://dx.doi.org/10.1038/ncomms7673
- Fuchs TA, Brill A, Duerschmied D, Schatzberg D, Monestier M, Myers DD, Jr, Wrobleski SK, Wakefield TW, Hartwig JH, Wagner DD. Extracellular DNA traps promote thrombosis. Proc Natl Acad Sci U S A 2010; 107:15880-5; PMID:20798043; http://dx.doi.org/10.1073/pnas.1005743107
- Kisucka J, Butterfield CE, Duda DG, Eichenberger SC, Saffaripour S, Ware J, Ruggeri ZM, Jain RK, Folkman J, Wagner DD. Platelets and platelet adhesion support angiogenesis while preventing excessive hemorrhage. Proc Natl Acad Sci U S A 2006; 103:855-60; PMID:16418262; http://dx.doi.org/10.1073/pnas.0510412103
- Lu T, Gabrilovich DI. Molecular pathways: tumor-infiltrating myeloid cells and reactive oxygen species in regulation of tumor microenvironment. Clin Cancer Res 2012; 18:4877-82; PMID:22718858; http://dx.doi.org/10.1158/1078-0432.CCR-11-2939
- El-Hag A, Clark RA. Immunosuppression by activated human neutrophils. Dependence on the myeloperoxidase system. J Immunol 1987; 139:2406-13; PMID:2821114; http://dx.doi.org/0022-1767/87/1397-2406S02.00
- Fuchs TA, Abed U, Goosmann C, Hurwitz R, Schulze I, Wahn V, Weinrauch Y, Brinkmann V, Zychlinsky A. Novel cell death program leads to neutrophil extracellular traps. J Cell Biol 2007; 176:231-41; PMID:17210947; http://dx.doi.org/10.1083/jcb.200606027
- Metzler KD, Fuchs TA, Nauseef WM, Reumaux D, Roesler J, Schulze I, Wahn V, Papayannopoulos V, Zychlinsky A. Myeloperoxidase is required for neutrophil extracellular trap formation: implications for innate immunity. Blood 2011; 117:953-9; PMID:20974672; http://dx.doi.org/10.1182/blood-2010-06-290171
- Papayannopoulos V, Metzler KD, Hakkim A, Zychlinsky A. Neutrophil elastase and myeloperoxidase regulate the formation of neutrophil extracellular traps. J Cell Biol 2010; 191:677-91; PMID:20974816; http://dx.doi.org/10.1083/jcb.201006052
- Mishalian I, Bayuh R, Levy L, Zolotarov L, Michaeli J, Fridlender ZG. Tumor-associated neutrophils (TAN) develop pro-tumorigenic properties during tumor progression. Cancer Immunol Immunother 2013; 62:1745-56; PMID:24092389; http://dx.doi.org/10.1007/s00262-013-1476-9
- Lewis HD, Liddle J, Coote JE, Atkinson SJ, Barker MD, Bax BD, Bicker KL, Bingham RP, Campbell M, Chen YH et al. Inhibition of PAD4 activity is sufficient to disrupt mouse and human NET formation. Nat Chem Biol 2015; 11:189-91; PMID:25622091; http://dx.doi.org/10.1038/nchembio.1735