ABSTRACT
Adoptive transfer of tumor-infiltrating lymphocytes (TIL) has shown promising yet sometimes suboptimal results in clinical trials for advanced cancer, underscoring the need for approaches improving efficacy and safety. Six implantable syngeneic tumor cell lines of the Syrian hamster were used to initiate TIL cultures. TIL generated from tumor fragments cultured in human interleukin-2 (IL-2) for 10 d were adoptively transferred into tumor-bearing hamsters with concomitant intratumoral injections of oncolytic adenovirus (Ad5-D24) for the assessment of antitumor efficacy. Pancreatic cancer (HapT1) and melanoma (RPMI 1846) TIL exhibited potent and tumor-specific cytotoxicity in effector-to-target (E/T) assays. MHC Class I blocking abrogated the cell killing of RPMI 1846 TIL, indicating cytotoxic CD8+ T-cell activity. When TIL were combined with Ad5-D24 in vitro, HapT1 tumor cell killing was significantly enhanced over single agents. In vivo, the intratumoral administration of HapT1 TIL and Ad5-D24 resulted in improved tumor growth control compared with either treatment alone. Additionally, splenocytes derived from animals treated with the combination of Ad5-D24 and TIL killed autologous tumor cells more efficiently than monotherapy-derived splenocytes, suggesting that systemic antitumor immunity was induced. For the first time, TIL of the Syrian hamster have been cultured, characterized and used therapeutically together with oncolytic adenovirus for enhancing the efficacy of TIL therapy. Our results support human translation of oncolytic adenovirus as an enabling technology for adoptive T-cell therapy of solid tumors.
Abbreviations
Ad | = | adenovirus |
ANOVA | = | analysis of variance |
APC | = | allophycocyanin |
CAR | = | chimeric antigen receptor |
CTLA-4 | = | cytotoxic T-lymphocyte-associated protein 4 |
E/T | = | effector-to-target |
FBS | = | fetal bovine serum |
HEPES | = | 4-(2-Hydroxyethyl)piperazine-1-ethanesulfonic acid |
IFNγ | = | interferon gamma |
IFNα2 | = | interferon α-2 |
IL-2 | = | interleukin-2 |
IL-15 | = | interleukin-15 |
MHC | = | major histocompatibility complex |
MTS | = | 3-(4,5-dimethylthiazol-2-yl)-5-(3-carboxymethoxyphenyl)-2-(4-sulfophenyl)-2H-tetrazolium |
NK | = | natural killer |
OVA | = | ovalbumin |
PBS | = | phosphate buffered saline |
PE | = | phycoerythrin |
RANTES | = | regulated on activation, normal T-cell expressed and secreted |
REP | = | rapid expansion protocol |
SE | = | standard error |
TAA | = | tumor-associated antigen |
TCC | = | American Type Culture Collection |
TIL | = | tumor-infiltrating lymphocyte |
TNFα | = | tumor necrosis factor α |
VP | = | viral particle. |
Introduction
Adoptive transfer of ex vivo expanded TIL has shown promising results in murine models of melanoma,Citation1 and in humans.Citation2 However, not all patients benefit from the treatment and significant toxicity accompanies the high doses of intravenous IL-2 and preconditioning lymphodepletion that have been considered a prerequisite to support the T-cell graft.Citation3 Novel approaches for improving the efficacy and safety of adoptive transfer regimens are thus needed. Of note, a safe technology allowing the omission of pre- and post-conditioning, without reduction in efficacy, would be attractive.
Oncolytic viruses are genetically modified viruses that can infect and lyse tumor cells while leaving normal cell unharmed.Citation4 Preclinical and clinical studies have revealed that in addition to a direct cytopathic effect, oncolytic viruses induce the release of tumor-associated antigens (TAAs) upon infection of tumor cells and can thereby promote antitumor immune responses.Citation5 Oncolytic adenoviruses in particular possess a good safety profile and can be effectively combined with conventional cancer treatments.Citation6 Not all oncolytic viruses are alike with regard to their effects on adaptive immunity but adenovirus might be especially attractive in this regard.Citation7
Unlike most rodent species, the Syrian hamster supports productive replication of human adenovirus.Citation8 Several implantable tumor cell lines, with wide histological range, have been identified and utilized in cancer gene therapy studies with oncolytic adenovirus vectors in this fully immunocompetent model.Citation9,10 Yet, immunological studies of Syrian hamster tumors are scarce, owing to general lack of hamster-specific reagents. With regard to TIL therapy, the features and therapeutic potential of TIL in hamsters are unknown.
Standard human protocols for generating sufficient numbers of TIL for adoptive transfer comprise lengthy and labor-intensive in vitro expansion steps and immunological screening assays to identify tumor-reactive cultures.Citation11 In contrast, the use of “young” unselected TIL have simplified the production of large-scale numbers of lymphocytes that demonstrate comparable antitumor activity to standard TIL.Citation12,13 Young TIL also display a favorable differentiation status with higher expression of costimulatory molecules and longer telomeres.Citation14 Furthermore, recent clinical studies support the use of young unselected TIL in adoptive transfer trials.Citation15,16 Therefore, young TIL may represent a preferred option for therapy.
Here, we describe the use of minimally cultured TIL of the Syrian hamster as a novel platform for adoptive immunotherapy studies. Hamster TIL antitumor CD8+ T-cell activity was dependent on the tumor model, as validated by flow-cytometric cell labeling and negating of CD8+ cytolytic activity using blocking anti-mouse major histocompatibility complex (MHC)-I antibody. Synergistic antitumor efficacy in vivo was achieved when unselected bulk TIL (young TIL), generated in 10 d from tumor fragments cultured in high-dose human IL-2, were administered in combination with intratumoral injections of oncolytic adenovirus Ad5-D24. The Syrian hamster TIL models and the immunological methods described here will facilitate functional studies on hamster immune cells in the future. Our data strongly supports investigation of adenovirus-enhanced TIL therapy in human cancer patients.
Results
CD4+ to CD8+ ratio in Syrian hamster tumors
For immunophenotypic studies of Syrian hamster tumors, subcutaneously implanted tumors (representing various tumor types) were collected and analyzed by flow cytometry. CD4 and CD8 staining of single-cell suspensions revealed a tumor type-dependent pattern of lymphocyte infiltration (). HapT1 (pancreas), RPMI 1846 (melanoma), HaK (kidney) and PC1 (pancreas) tumors contained CD8+ lymphocytes ranging from 0.08% to 0.93%, while DDT1-MF2 (leiomyosarcoma) and HMAM5 (breast) did not contain detectable levels of CD8. CD4+ lymphocytes were observed in all tumor types and ranged from 0.17% to 3.25%.
Figure 1. Immunophenotyping of Syrian hamster tumors. Percentage of CD4+ (black bar) and CD8+ (gray bar) cells in various Syrian hamster tumors processed into single-cell suspensions, stained with cross-reactive antibodies and analyzed by flow cytometry (50,000 events). Data representative of four tumors. Error bars, SE.
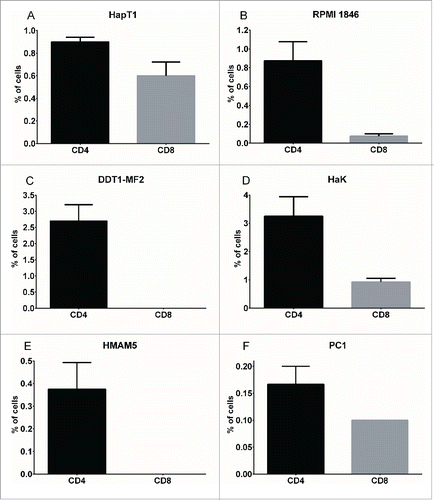
CD4+ lymphocytes predominate over CD8+ in hamster TIL cultures
To obtain TIL, we cultured tumor fragments in high-dose recombinant human IL-2 for 10 d. With all tumor types studied, TIL outgrowth was visible by Day 5 of culture. On Days 5 and 10, CD4/CD8 FACS was performed on individual TIL cultures (i.e. TIL/fragment/well) and on a pooled sample (). Interestingly, in all tumor types studied, CD4+ cells predominated over CD8+ cells to varying degrees. RPMI 1846 and DDT1-MF2 TIL consisted of 40% CD4+ cells while the CD8+ cells constituted 13.5% and 0.2% of total, respectively. The portion of CD4+ cells in HapT1, HaK, HMAM5 and PC1 cultures reached 12–24%, with CD8+ lymphocytes being observed mainly in HapT1 (9.6%) and possibly in HMAM5 (0.2%). It is noteworthy that the presence of natural killer (NK) cells, a likely component of IL-2-stimulated TIL cultures, could not be determined due to the lack of cross-reactive antibodies against Syrian hamster NK cells.
Figure 2. Lymphocyte composition of TIL cultures. Tumor fragments were cultured in recombinant human IL-2 (6,000 IU/mL) on 24-well plates. On Day 5, when TIL outgrowth became visible, the tumor fragment was removed and remaining TIL were analyzed for CD4+ and CD8+ by flow cytometry. On Day 10, individual wells with TIL outgrowth was combined and the pooled sample was analyzed for CD4+ and CD8+. Data representative of four fragments. Error bars, SE.
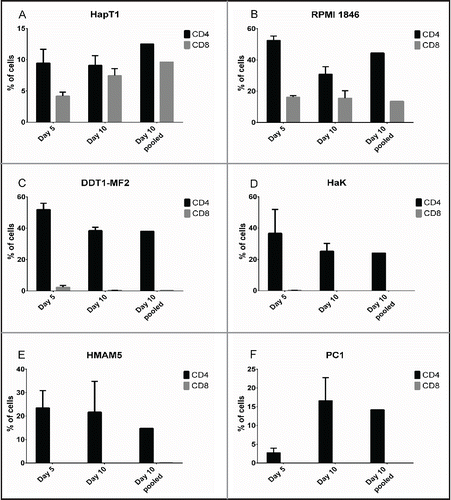
HapT1 and RPMI 1846 TIL exhibit tumor-specific cytolytic activity
The cell killing ability of Syrian hamster TIL was studied in E/T assays where autologous tumor cells, non-related hamster tumor cells or human cells were co-cultured with effector TIL (). HapT1 TIL efficiently killed autologous cells (HapT1), but not human cells (A549) or other hamster cells (DDT1-MF2), even at low E/T ratios (). RPMI 1846 TIL exhibited a similar pattern of tumor-specific lysis (). TIL from DDT1-MF2 and HaK killed target cells non-specifically as A549 and other hamster cells were also lysed at the higher E/T ratios (10:1 and 20:1) (). HMAM5 and PC1 TIL did not effectively kill either autologous tumor cells or other cells ().
Effect of MHC class I blocking on the cytolytic activity of TIL
The TIL that exhibited specific lytic activity in E/T assays (HapT1 and RPMI 1846) were selected for further analyses. HapT1 and RPMI 1846 target tumor cells were pre-incubated with a cross-reactive anti-MHC-I antibody before the addition of TIL (). In the presence of the antibody the cytolytic activity of RPMI 1846 TIL was abrogated, indicating that cytotoxic CD8+ T cells are responsible for the observed cytolytic activity (anti-MHCI vs. no inhibition, p = 0.01) (). For HapT1, MHC I blocking only partially inhibited cell killing (68% vs. 60% viability between blocked and non-blocked target cells, respectively; p = 0.02) (). HapT1 cells were also confirmed to express MHC Class II (Fig. S1), suggesting that cytotoxicity of HapT1 TIL could be mediated by CD4+ T cells.
Figure 4. Effect of MHC Class I blocking on cytolytic activity of TIL. (a) RPMI 1846 and (b) HapT1 target cells were pre-incubated for 2 h with anti-MHC Class I antibody (50 µg/mL), isotype control (50 µg/mL) or left untreated (no inhibition) before adding TIL. Target cell viability was determined 24 h later by MTS. Error bars, SE. *p < 0.05, ns = not significant.
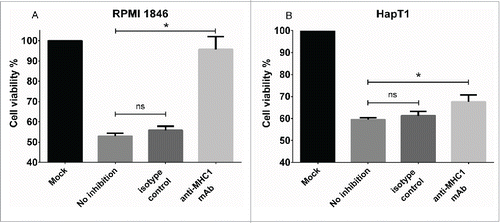
TIL and oncolytic adenovirus combination results in additive tumor cell killing in vitro
For the assessment of in vitro cell killing effects of TIL combined with oncolytic adenovirus, HapT1 cells were infected with Ad5-D24 at 100 VP/cell for 3 d before addition of HapT1 TIL (2:1 E/T ratio) (). When compared with control cells, single agents decreased cell viability to 58% (Ad5-D24) and 70% (TIL). The combination decreased cell viability to 24% (combination vs. Ad5-D24, p <0.0001; combination vs. TIL, p < 0.0001). We have previously shown that RPMI 1846 cells are not permissive to human adenovirus replication and therefore RPMI 1846 TIL were not studied in combination with Ad5-D24.Citation17
Ad5-D24 enhances the antitumor efficacy of adoptive TIL transfer
The antitumor activity of TIL and oncolytic adenovirus combination was studied in hamsters bearing established HapT1 tumors. Intratumoral injection of 1.5 × 106 HapT1 TIL and Ad5-D24 (1 × 107 VP/tumor) together were able to reduce tumor size significantly compared with the single agents (combination vs. TIL, p = 0.009; combination vs. Ad5-D24, p = 0.007; combination vs. mock, p = 0.046) (). The treatment regimen consisted of two virus injections given on Days 1 and 8 with one local administration of TIL on Day 2.
Figure 6. Combination treatment of established HapT1 tumors with TIL and Ad5-D24. Subcutaneous HapT1 tumors were allowed to develop on both flanks of Syrian hamsters (5 × 106 cells per flank) before reaching 6 mm in diameter. On Days 1 and 8, Ad5-D24 (1 × 107 VP/tumor) was injected intratumorally. On Day 2, HapT1 TIL (1.5 × 106 TIL/tumor) were administered intratumorally. Error bars, SE. *p < 0.05, **p < 0.01.
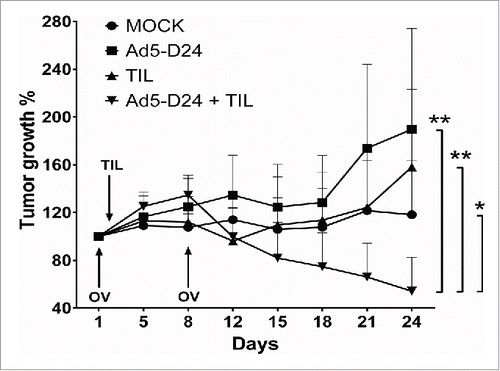
Combination therapy-derived splenocytes show increased cell killing capability
Tumors collected at the in vivo experiment end-point (Day 24) were analyzed for CD4+ and CD8+ T cells with flow cytometry. An increase in CD8+ lymphocytes was observed in the combination-treated tumors over TIL-treated tumors with a p value approaching significance (p = 0.05) (). No significant differences were seen in CD4+ between treatment groups (). Finally, splenocytes collected from end-point animals were co-cultured with HapT1 target cells. Interestingly, splenocytes from combination-treated animals killed HapT1 cells more efficiently than the splenocytes from mock-treated or monotherapy-treated animals (combination vs. mock, p <0.0001; combination vs. Ad5-D24, p = 0.0015; combination vs. TIL, p = 0.0018) ().
Figure 7. T-cell infiltration and splenocyte cell killing activity following treatment with TIL and Ad5-D24. (a) CD4+ and (b) CD8+ T cells were analyzed from Day 24 tumors by flow cytometry. (c) Splenocytes (collected from hamsters sacrificed on Day 24) were co-cultured with HapT1 cells at the effector-to-target ratio of 50:1. Target cell viability was determined 24 h later by MTS. Error bars, SE. **p < 0.01, ****p < 0.0001.
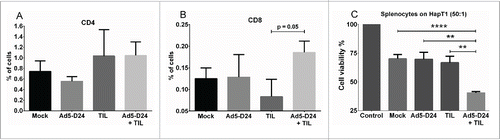
Discussion
Adoptive transfer of TIL represents a promising approach for the treatment of advanced cancer. However, the approach has not been successfully used beyond melanoma, and even in this disease the therapy only works when combined with highly toxic pre- and post-conditioning regimens. We hypothesize that the anti-immunosuppressive effects of adenoviruses could be a key feature in enabling the full potential of adoptive cell therapies.Citation18-20 However, assessing these questions has been plagued by the lack of a model system where the oncolytic potential of adenoviruses would be present in the context of an immunocompetent animal, taking into account that human adenoviruses do not produce new virions in mouse tissues, even if the genome does replicate.Citation10 Since the danger signaling relevant for anti-immunosuppression depends on oncolysis,Citation21 the lack thereof renders murine models suboptimal at best.
Tumors of various histological origin from the Syrian hamster were immunophenotyped with currently available cross-reactive antibodies. A common feature between the different tumor types was the preponderance of CD4+ TIL over CD8+ TIL. However, the specific subtypes of these CD4+ lymphocytes remain unknown given the shortage of antibodies against key molecules. Since CD4+ T cells can obtain “helper” or “regulator” functions depending on the cytokine milieu,Citation22,23 further investigations (pending hamster-specific reagents) could be performed to elucidate the phenotypic and functional characteristics of tumor-associated CD4+ T cells of the Syrian hamster. Some tumor types (such as HaK, HMAM5 and PC1 in hamsters) with notable CD4+ infiltration in the total absence of CD8+ TIL suggests that these CD4+ cells might consist mainly of T regulatory cells (Tregs), which have been reported to inhibit CD8+ T-cell infiltration into tumors.Citation24 On the other hand, the CD4+ cells in other tumor types (such as HapT1 and RPMI 1846) might in fact represent T helper cells (Th) and thus participate in the activation of CD8+ CTLs.Citation25 Importantly, as bulk TIL were used in the adoptive transfer experiments instead of CD8+ enriched cells, the potential immunostimulatory role of CD4+ lymphocytes cannot be discarded.
MHC-blocking experiments revealed that TIL cultured from RPMI 1846 tumors exhibited MHC-restricted cytotoxicity, indicative of cytotoxic CD8+ T-cell presence. The cytolytic activity of HapT1 TIL was only partially inhibited by MHC-blocking, suggesting that the HapT1 TIL population contained NK cells in addition to CD8+ and CD4+ T-cells. However, E/T assays performed with HapT1 TIL showed highly specific cell killing, contradicting the presence or tumor cell-killing activity of NK cells. Intriguingly, some tumor-reactive CD4+ T cells can develop cytotoxic activityCitation26 and many tumors express functional MHC-II.Citation27 We hypothesized that CD4+ T cells may have acquired this function in the HapT1 TIL population but not in RPMI 1846 TIL, which killed autologous tumor cells in a completely MHC-I-restricted manner. Indeed, we found that HapT1 cells express MHC Class II molecules, indicating that the highly specific cytotoxicity of HapT1 TIL that was (seemingly counter-intuitively) not abrogated by MHC-blocking can be explained by the presence of cytotoxic CD4+ T cells.
The TIL generated from RPMI 1846 melanoma tumors initially seemed to represent the most appealing option for adoptive transfer studies together with Ad5-D24. RPMI 1846 TIL grew more robustly in hIL-2 than other TIL and had the highest percentage of CD8+ lymphocytes that were also responsible for cytotoxicity. However, as reported before, these melanoma cells do not support adenovirus replicationCitation17 and were therefore excluded from combination studies. Yet, our data on RPMI 1846 TIL may be of interest to other investigators and potentially useful for immunotherapeutic approaches involving melanoma-derived TIL from a species other than mouse or human.
Ad5-D24 and HapT1 TIL acted additively in tumor cell killing assays in vitro. Previous research has shown that oncolytic adenoviruses can be successfully combined with more conventional cancer treatments i.e., chemotherapyCitation28 and radiotherapy.Citation29 Further studies are needed to elucidate the underlying mechanism behind the increased efficacy of the combination of TIL and oncolytic adenovirus in vitro. Superior tumor cell killing with the combination of CAR T-cells and Ad5-D24 was recently reported to be associated with accelerated caspase pathways in human tumor cells.Citation30 However, upregulation of death receptors or costimulatory molecules on infected tumor cells (rendering tumor cells more sensitive to lymphocyte-mediated killing) was not observed. Speculatively, these mechanisms may play a role in hamster tumor cells, warranting further investigation of the in vitro setting, if the appropriate reagents can be generated.
To study the in vivo efficacy of the combination treatment, TIL expanded in hIL-2 for 10 d were injected locally into established HapT1 tumors with or without adenovirus treatment. These TIL comprised 15% CD4+ cells and 10% CD8+ cells. In comparison, human TIL preparations exhibit highly variable CD4/CD8 T-cell ratios even between different fragments from the same tumor.Citation14 The remaining cells could not be characterized, but MHC-blocking experiments suggest at least the presence of NK cells and/or cytotoxic CD4+ T cells. Our TIL culture protocol did not include the rapid-expansion phase (REP) typically used in adoptive transfer trials.Citation31 NK cells expand alongside T cells in initial TIL cultures with IL-2, but are subsequently lost when anti-CD3 stimulation is introduced during the REP.Citation32 Due to lack of cross-reactive anti-CD3 antibody, the presence and/or expansion of NK cells in the hamster TIL cultures is rather likely. The role of NK cells in TIL products is unclear, but could prove valuable as shown in murine B16 melanoma, where the depletion of NK cells and CD8+ T cells together (but not either alone) abrogated the therapeutic efficacy of high-dose IL-2 and CTLA-4 blockade.Citation33 Similarly, the importance of CD4+ T cells in TIL products remains poorly understood since some patients have responded well to TIL treatments dominated by CD4+ T cells.Citation32 Preclinically, co-treatment with tumor-specific CD4+ and CD8+ T cells resulted in synergistic efficacy with indications that the CD4+ T cells reduced CD8+ T-cell exhaustion.Citation34 Furthermore, while the REP allows large numerical expansion of TIL it affects the survival and proliferative capacity of T cells after transfer, which is linked to decrease in telomere length and the loss of cell-surface CD28 expression.Citation35 Taken together, the heterogeneous “bulk” TIL product used for adoptive transfer into hamsters might represent the ideal TIL population.
It remains unclear whether the borderline significant p value (0.05) observed in CD8+ cells between TIL vs. combination tumors after treatment has true biological significance, i.e. increased tumor-infiltration or local expansion of CD8+ lymphocytes. Specifically, it is not known if increases in CD8+ are necessary or useful for efficacy. Mouse studies performed in our group do not necessarily support these hypotheses in the context of combination therapy. Instead, other mechanisms such as the activation status of T cells are suggested and discussed below.Citation36 In contrast to mouse studies, the available human evidence suggest that accumulation of T cells does correlate with efficacy endpoints.Citation37 Further, for most tumor types there is a large body of data indicating that the concentration of T cells in tumors (TIL) correlates with favorable outcome.Citation38-40
In addition to antitumor efficacy in vivo, increased tumor cell killing activity of combination treatment-derived splenocytes ex vivo suggest the presence of active antitumor T cells on a systemic level. Previously, we have shown that adenovirus improved the efficacy of adoptive T-cell transfer in mice by increasing the number of IFNγ-expressing CD8+ T cells as well as inducing endogenous CD8+ T cells against the TAA TRP-2 and gp100.Citation36 Unfortunately, these hypotheses cannot be studied in the hamster model due to lack of hamster-specific (or acceptably cross-reactive) reagents for the aforementioned markers. In addition, the actual mechanism-of-action might differ from mouse studies due to active oncolysis of hamster tumor cells, a scenario that cannot be studied in murine models but is much closer to the clinical situation in human patients.
The Syrian hamster model we describe has several advantages related to adenovirotherapy and adoptive cell transfer. First, Syrian hamsters are permissive for human adenovirus replication as opposed to mice,Citation8 enabling the safety and efficacy evaluation of adenovirus vectors in a model that more accurately mimicks the setting in human cancer patients. Secondly, immunodeficient mouse models lack stromal cells that affect tumor growth and response to therapy.Citation41 Currently, these aspects need to be dissected by using several mouse models. In this regard, the hamster model reported here represents a significant improvement.
Future studies could explore oncolytic adenoviruses armed with transgenes that support the transferred T cells in situ, e.g. cytokines and chemokines. Recently, this approach was used in a preclinical immunodeficient model of neuroblastoma with CAR T-cells and adenovirus coding for RANTES and IL-15.Citation30 Other candidates might include IL-2, TNFα, IFNα2 and IFNγ.Citation42 Of note, many human cytokines are biologically active in Syrian hamsters,Citation43,44 highlighting the value of this immunocompetent rodent model for future research. With regard to weaknesses of the model, key laboratory reagents are lacking and the growth of tumors over 2 weeks do not recapitulate the complexity which develops over a decade or more in human patients. Additionally, the route of administration of TIL in our study (intratumoral) was not typical of current TIL protocols that often employ intravenous administration, although also intratumoralCitation45 and intracavitaryCitation46 have been used. Unfortunately, since a stimulatory hamster anti-CD3 antibody is lacking, a true REP growth step could not be performed, which limited the available options to local injection. Moreover, the lack of a tail vain in hamsters complicates intravenous injection in this animal. The low efficacy of single-agent therapies observed in the in vivo experiment could be explained by the absence of concomitant pre- and post-conditioning treatments (TIL alone group), which are prerequisite for efficacy in humans,Citation3 and the low virus dose (Ad5-D24 alone group). Also, the virus used here was not armed with a transgene. It is generally thought that armed oncolytic viruses are more potent than unarmed viruses and therefore the former are typically employed in clinical development.Citation9,18,21,43
In conclusion, we have shown that oncolytic adenovirus can be used to improve the efficacy of adoptive TIL transfer. Also, this is the first time TIL of the Syrian hamster have been cultured, characterized and used therapeutically. Our model fulfills the key requirement for an effective preclinical combination therapy platform: adenoviral replication comparable to humans in a fully immunocompetent environment. These results support the further development of oncolytic adenoviruses as an enabling technology for adoptive T-cell therapies including but not limited to TIL therapy.
Materials and methods
Tumor cell lines and oncolytic virus
The following Syrian hamster tumor cell lines were used: HapT1 (courtesy of Dr Hernandez-Alcoceba, Pamplona, Spain); HMAM5 (courtesy of Dr Michael Mathis, Louisiana State University, LA, USA); DDT1-MF2, PC1, HaK (courtesy of Prof. William Wold, St. Louis, MO, USA); RPMI 1846 (American Type Culture Collection, ATCC). B16.OVA mouse melanoma cells were kindly gifted by Prof. Richard Vile (Mayo Clinic, Rochester, MN, USA). A549 (human lung adenocarcinoma) was purchased from ATCC. All cell lines were cultured in recommended conditions. Ad5-D24 has been described previously.Citation47
TIL culture
5–6-week-old Syrian hamsters (Mesocricetus auratus) were purchased from Harlan Laboratories. Subcutaneously implanted tumors were excised when they reached approximately 1 cm in size. Tumors were cut into fragments of 1–3 mm3 and cultured in individual wells of a 24-well plate (2 mL/well). Culture medium consisted of RPMI-1640 supplemented with 10% fetal bovine serum (FBS), 1% penicillin/streptomycin, 1% L-glutamine, 15 mM HEPES, 50 µM 2-mercaptoethanol, 1 mM Na-pyruvate and 6,000 IU/mL human IL-2 (hIL-2) (PeproTech). Half of the medium was renewed 5 d after culture initiation and every 2 d after that. On Day 10 of culture, wells with visible TIL growth were collected and pooled for E/T assays or adoptive transfer.
Flow cytometric analyses
Single-cell suspensions of excised tumors (Day 0) or TIL cultures (Days 5 and 10) were stained with the following cross-reactive antibodies: anti-rat CD8b PE (clone 341, eBioscience)Citation48 and anti-mouse CD4 APC (clone GK1.5, eBioscience)Citation48 for 30 min, fixed in 4% paraformaldehyde, and analyzed with BD Accuri C6 (BD Biosciences) collecting at least 50,000 events per sample. HapT1 cells were incubated with FITC-conjugated anti-mouse/rat MHC Class II antibody (I-Ek; clone 14-4-4 S, eBioscience)Citation49 for 1 h and fixed in 4% paraformaldehyde for 5 min, and analyzed on BD Accuri C6.
Co-culture and blocking experiments
E/T assays were performed by seeding 96-well plates with autologous tumor cells, other hamster tumor cells or human A549 cells (1 × 104 target cells/well). Effector TIL were added at different E/T ratios and cell viability was determined 24 h later by MTS assay (Promega). In combination experiments with TIL and Ad5-D24, target tumor cells were infected with 100 viral particles/cell (VP/cell) and TIL (2:1 E/T ratio) were added 3 d later. Cell viability was determined 24 h after addition of TIL. In blocking experiments, target cells on 96-wells plates were pre-incubated with 50 µg/mL of anti-MHC-I monoclonal antibody (clone 2G5, Novus Biologicals) or isotype control (mouse IgG2b, Novus Biologicals) for 2 h at 37°C before adding effector cells and determining cell viability 24 h later. The 2G5 clone is reported to cross-react with Syrian hamster MHC-ICitation50 and to block MHC-I in functional assays.Citation51 The blocking capability was confirmed by pre-incubating B16.OVA cells with the antibody (or isotype control) before adding OVA-specific CD8+-enriched OT-I T-cells (1:1 E/T ratio) and determining cell viability as above (Fig. S2).
Adoptive TIL transfer and virus treatments
Hamsters bearing established HapT1 tumors (five animals per group) were treated with intratumoral injection of 1 × 107 viral particles of Ad5-D24 on days 1 and 8 in 50 µL of PBS. HapT1 TIL were administered on day 2 by intratumoral injection in 50 µL of plain RPMI (1.5 × 106 TIL per tumor). All treatments were performed under isoflurane anesthesia. On day 24, all animals were killed and selected organs were collected for biological analyses. All animal protocols were reviewed and approved by the Experimental Animal Committee of the University of Helsinki and the Provincial Government of Southern Finland.
Statistical analyses
Statistics were performed with GraphPad Prism 6 (GraphPad Software Inc.). Student's t-test and repeated measures two-way ANOVA were used; p values of <0.05 were considered significant.
Disclosure of potential conflicts of interest
A.H. is shareholder in Targovax AS. A.H. is employee and shareholder in TILT Biotherapeutics Ltd. M.S. and S.P. are employees of TILT Biotherapeutics Ltd.
KONI_A_1136046_s02.zip
Download Zip (54.1 KB)Acknowledgments
The authors thank Susanna Grönberg-Vähä-Koskela and Minna Oksanen for expert assistance.
References
- Rosenberg SA, Spiess P, Lafreniere R. A new approach to the adoptive immunotherapy of cancer with tumor-infiltrating lymphocytes. Science 1986; 233:1318-21; PMID:3489291; http://dx.doi.org/10.1126/science.3489291
- Hinrichs CS, Rosenberg SA. Exploiting the curative potential of adoptive T-cell therapy for cancer. Immunol Rev 2014; 257:56-71; PMID:24329789; http://dx.doi.org/10.1111/imr.12132
- Rosenberg SA. IL-2: The first effective immunotherapy for human cancer. J Immunol 2014; 192:5451-8; PMID:24907378; http://dx.doi.org/10.4049/jimmunol.1490019
- Jiang H, Gomez-Manzano C, Lang FF, Alemany R, Fueyo J. Oncolytic adenovirus: Preclinical and clinical studies in patients with human malignant gliomas. Curr Gene Ther 2009; 9:422-7; PMID:19860656; http://dx.doi.org/10.2174/156652309789753356
- Pol J, Bloy N, Obrist F, Eggermont A, Galon J, Cremer I, Erbs P, Limacher JM, Preville X, Zitvogel L et al. Trial watch: Oncolytic viruses for cancer therapy. Oncoimmunology 2014; 3:e28694; PMID:25097804; http://dx.doi.org/10.4161/onci.28694
- Bressy C, Benihoud K. Association of oncolytic adenoviruses with chemotherapies: An overview and future directions. Biochem Pharmacol 2014; 90:97-106; PMID:24832861; http://dx.doi.org/10.1016/j.bcp.2014.05.003
- Zamarin D, Pesonen S. Replication-competent viruses as cancer immunotherapeutics: Emerging clinical data. Hum Gene Ther 2015; 26:538-49; PMID:26176173; http://dx.doi.org/10.1089/hum.2015.055
- Thomas MA, Spencer JF, La Regina MC, Dhar D, Tollefson AE, Toth K, Wold WS. Syrian hamster as a permissive immunocompetent animal model for the study of oncolytic adenovirus vectors. Cancer Res 2006; 66:1270-6; PMID:16452178; http://dx.doi.org/10.1158/0008-5472.CAN-05-3497
- Cerullo V, Pesonen S, Diaconu I, Escutenaire S, Arstila PT, Ugolini M, Nokisalmi P, Raki M, Laasonen L, Sarkioja M et al. Oncolytic adenovirus coding for granulocyte macrophage colony-stimulating factor induces antitumoral immunity in cancer patients. Cancer Res 2010; 70:4297-309]; PMID:20484030; http://dx.doi.org/10.1158/0008-5472.CAN-09-3567
- Wold WS, Toth K. Chapter three–syrian hamster as an animal model to study oncolytic adenoviruses and to evaluate the efficacy of antiviral compounds. Adv Cancer Res 2012; 115:69-92; PMID:23021242; http://dx.doi.org/10.1016/B978-0-12-398342-8.00003-3
- Besser MJ, Shapira-Frommer R, Treves AJ, Zippel D, Itzhaki O, Hershkovitz L, Levy D, Kubi A, Hovav E, Chermoshniuk N et al. Clinical responses in a phase II study using adoptive transfer of short-term cultured tumor infiltration lymphocytes in metastatic melanoma patients. Clin Cancer Res 2010; 16:2646-55; PMID:20406835; http://dx.doi.org/10.1158/1078-0432.CCR-10-0041
- Tran KQ, Zhou J, Durflinger KH, Langhan MM, Shelton TE, Wunderlich JR, Robbins PF, Rosenberg SA, Dudley ME. Minimally cultured tumor-infiltrating lymphocytes display optimal characteristics for adoptive cell therapy. J Immunother 2008; 31:742-51; PMID:18779745; http://dx.doi.org/10.1097/CJI.0b013e31818403d5
- Besser MJ, Shapira-Frommer R, Treves AJ, Zippel D, Itzhaki O, Schallmach E, Kubi A, Shalmon B, Hardan I, Catane R et al. Minimally cultured or selected autologous tumor-infiltrating lymphocytes after a lympho-depleting chemotherapy regimen in metastatic melanoma patients. J Immunother 2009; 32:415-23; PMID:19342963; http://dx.doi.org/10.1097/CJI.0b013e31819c8bda
- Donia M, Junker N, Ellebaek E, Andersen MH, Straten PT, Svane IM. Characterization and comparison of “standard” and “young” tumor infiltrating lymphocytes for adoptive cell therapy at a danish translational research institution. Scand J Immunol 2011; 75:157-167; PMID:21955245; http://dx.doi.org/10.1111/j.1365-3083.2011.02640.x
- Itzhaki O, Hovav E, Ziporen Y, Levy D, Kubi A, Zikich D, Hershkovitz L, Treves AJ, Shalmon B, Zippel D et al. Establishment and large-scale expansion of minimally cultured “young” tumor infiltrating lymphocytes for adoptive transfer therapy. J Immunother 2011; 34:212-20; PMID:21304398; http://dx.doi.org/10.1097/CJI.0b013e318209c94c
- Dudley ME, Gross CA, Somerville RP, Hong Y, Schaub NP, Rosati SF, White DE, Nathan D, Restifo NP, Steinberg SM et al. Randomized selection design trial evaluating CD8+-enriched versus unselected tumor-infiltrating lymphocytes for adoptive cell therapy for patients with melanoma. J Clin Oncol 2013; 31:2152-9; PMID:23650429; http://dx.doi.org/10.1200/JCO.2012.46.6441
- Bramante S, Kaufmann JK, Veckman V, Liikanen I, Nettelbeck DM, Hemminki O, Vassilev L, Cerullo V, Oksanen M, Heiskanen R et al. Treatment of melanoma with a serotype 5/3 chimeric oncolytic adenovirus coding for GM-CSF: Results in vitro, in rodents and in humans. Int J Cancer 2015; 137:1775-83; PMID:25821063; http://dx.doi.org/10.1002/ijc.29536
- Diaconu I, Cerullo V, Hirvinen ML, Escutenaire S, Ugolini M, Pesonen SK, Bramante S, Parviainen S, Kanerva A, Loskog AS et al. Immune response is an important aspect of the antitumor effect produced by a CD40L-encoding oncolytic adenovirus. Cancer Res 2012; 72:2327-38; PMID:22396493; http://dx.doi.org/10.1158/0008-5472.CAN-11-2975
- Kanerva A, Nokisalmi P, Diaconu I, Koski A, Cerullo V, Liikanen I, Tahtinen S, Oksanen M, Heiskanen R, Pesonen S et al. Antiviral and antitumor T-cell immunity in patients treated with GM-CSF-coding oncolytic adenovirus. Clin Cancer Res 2013; 19:2734-44; PMID:23493351; http://dx.doi.org/10.1158/1078-0432.CCR-12-2546
- Hemminki O, Parviainen S, Juhila J, Turkki R, Linder N, Lundin J, Kankainen M, Ristimaki A, Koski A, Liikanen I et al. Immunological data from cancer patients treated with Ad5/3-E2F-Delta24-GMCSF suggests utility for tumor immunotherapy. Oncotarget 2015; 6:4467-81; PMID:25714011; http://dx.doi.org/10.18632/oncotarget.2901
- Cerullo V, Diaconu I, Romano V, Hirvinen M, Ugolini M, Escutenaire S, Holm SL, Kipar A, Kanerva A, Hemminki A. An oncolytic adenovirus enhanced for toll-like receptor 9 stimulation increases antitumor immune responses and tumor clearance. Mol Ther 2012; 20:2076-86; PMID:22828500; http://dx.doi.org/10.1038/mt.2012.137
- Zhu J, Yamane H, Paul WE. Differentiation of effector CD4 T cell populations (*). Annu Rev Immunol 2010; 28:445-89; PMID:20192806; http://dx.doi.org/10.1146/annurev-immunol-030409-101212
- Geginat J, Paroni M, Maglie S, Alfen JS, Kastirr I, Gruarin P, De Simone M, Pagani M, Abrignani S. Plasticity of human CD4 T cell subsets. Front Immunol 2014; 5:630; PMID:25566245; http://dx.doi.org/10.3389/fimmu.2014.00630
- Nishikawa H, Sakaguchi S. Regulatory T cells in cancer immunotherapy. Curr Opin Immunol 2014; 27:1-7; PMID:24413387; http://dx.doi.org/10.1016/j.coi.2013.12.005
- Muranski P, Restifo NP. Adoptive immunotherapy of cancer using CD4(+) T cells. Curr Opin Immunol 2009; 21:200-8; PMID:19285848; http://dx.doi.org/10.1016/j.coi.2009.02.004
- Quezada SA, Simpson TR, Peggs KS, Merghoub T, Vider J, Fan X, Blasberg R, Yagita H, Muranski P, Antony PA et al. Tumor-reactive CD4(+) T cells develop cytotoxic activity and eradicate large established melanoma after transfer into lymphopenic hosts. J Exp Med 2010; 207:637-50 PMID:20156971; http://dx.doi.org/10.1084/jem.20091918
- Matsuzaki J, Tsuji T, Luescher I, Old LJ, Shrikant P, Gnjatic S, Odunsi K. Nonclassical antigen-processing pathways are required for MHC class II-restricted direct tumor recognition by NY-ESO-1-specific CD4(+) T cells. Cancer Immunol Res 2014; 2:341-50; PMID:24764581; http://dx.doi.org/10.1158/2326-6066.CIR-13-0138
- Siurala M, Bramante S, Vassilev L, Hirvinen M, Parviainen S, Tahtinen S, Guse K, Cerullo V, Kanerva A, Kipar A et al. Oncolytic adenovirus and doxorubicin-based chemotherapy results in synergistic antitumor activity against soft-tissue sarcoma. Int J Cancer 2015; 136:945-54; PMID:24975392; http://dx.doi.org/10.1002/ijc.29048
- Rajecki M, af Hallstrom T, Hakkarainen T, Nokisalmi P, Hautaniemi S, Nieminen AI, Tenhunen M, Rantanen V, Desmond RA, Chen DT et al. Mre11 inhibition by oncolytic adenovirus associates with autophagy and underlies synergy with ionizing radiation. Int J Cancer 2009; 125:2441-9; PMID:19672857; http://dx.doi.org/10.1002/ijc.24608
- Nishio N, Diaconu I, Liu H, Cerullo V, Caruana I, Hoyos V, Bouchier-Hayes L, Savoldo B, Dotti G. Armed oncolytic virus enhances immune functions of chimeric antigen receptor-modified T cells in solid tumors. Cancer Res 2014; 74:5195-205; PMID:25060519; http://dx.doi.org/10.1158/0008-5472.CAN-14-0697
- Dudley ME, Wunderlich JR, Shelton TE, Even J, Rosenberg SA. Generation of tumor-infiltrating lymphocyte cultures for use in adoptive transfer therapy for melanoma patients. J Immunother 2003; 26:332-42; PMID:12843795; http://dx.doi.org/10.1097/00002371-200307000-00005
- Wu R, Forget MA, Chacon J, Bernatchez C, Haymaker C, Chen JQ, Hwu P, Radvanyi LG. Adoptive T-cell therapy using autologous tumor-infiltrating lymphocytes for metastatic melanoma: Current status and future outlook. Cancer J 2012; 18:160-75; PMID:22453018; http://dx.doi.org/10.1097/PPO.0b013e31824d4465
- Kohlhapp FJ, Broucek JR, Hughes T, Huelsmann EJ, Lusciks J, Zayas JP, Dolubizno H, Fleetwood VA, Grin A, Hill GE et al. NK cells and CD8+ T cells cooperate to improve therapeutic responses in melanoma treated with interleukin-2 (IL-2) and CTLA-4 blockade. J Immunother Cancer 2015; 3:18, 015-0063-3. eCollection 2015; PMID:25992289; http://dx.doi.org/10.1186/s40425-015-0063-3
- Church SE, Jensen SM, Antony PA, Restifo NP, Fox BA. Tumor-specific CD4+ T cells maintain effector and memory tumor-specific CD8+ T cells. Eur J Immunol 2014; 44:69-79; PMID:24114780; http://dx.doi.org/10.1002/eji.201343718
- Li Y, Liu S, Hernandez J, Vence L, Hwu P, Radvanyi L. MART-1-specific melanoma tumor-infiltrating lymphocytes maintaining CD28 expression have improved survival and expansion capability following antigenic restimulation in vitro. J Immunol 2010; 184:452-65; PMID:19949105; http://dx.doi.org/10.4049/jimmunol.0901101
- Tahtinen S, Gronberg-Vaha-Koskela S, Lumen D, Merisalo-Soikkeli M, Siurala M, Airaksinen AJ, Vaha-Koskela M, Hemminki A. Adenovirus improves the efficacy of adoptive T-cell therapy by recruiting immune cells to and promoting their activity at the tumor. Cancer Immunol Res 2015; 3:915-25; PMID:25977260; http://dx.doi.org/10.1158/2326-6066.CIR-14-0220-T
- Vassilev L, Ranki T, Joensuu T, Jager E, Karbach J, Wahle C, Partanen K, Kairemo K, Alanko T, Turkki R et al. Repeated intratumoral administration of ONCOS-102 leads to systemic antitumor CD8 T-cell response and robust cellular and transcriptional immune activation at tumor site in a patient with ovarian cancer. Oncoimmunology 2015; 4:e1017702; PMID:26140248; http://dx.doi.org/10.1080/2162402X.2015.1017702
- Kawai O, Ishii G, Kubota K, Murata Y, Naito Y, Mizuno T, Aokage K, Saijo N, Nishiwaki Y, Gemma A et al. Predominant infiltration of macrophages and CD8(+) T cells in cancer nests is a significant predictor of survival in stage IV nonsmall cell lung cancer. Cancer 2008; 113:1387-95; PMID:18671239 http://dx.doi.org/10.1002/cncr.23712
- Pages F, Kirilovsky A, Mlecnik B, Asslaber M, Tosolini M, Bindea G, Lagorce C, Wind P, Marliot F, Bruneval P et al. In situ cytotoxic and memory T cells predict outcome in patients with early-stage colorectal cancer. J Clin Oncol 2009; 27:5944-51; PMID:19858404; http://dx.doi.org/10.1200/JCO.2008.19.6147
- Mina M, Boldrini R, Citti A, Romania P, D'Alicandro V, De Ioris M, Castellano A, Furlanello C, Locatelli F, Fruci D. Tumor-infiltrating T lymphocytes improve clinical outcome of therapy-resistant neuroblastoma. Oncoimmunology 2015; 4:e1019981; PMID:26405592; http://dx.doi.org/10.1080/2162402X.2015.1019981
- Maykel J, Liu JH, Li H, Shultz LD, Greiner DL, Houghton J. NOD-scidIl2rg (tm1Wjl) and NOD-Rag1 (null) Il2rg (tm1Wjl) : A model for stromal cell-tumor cell interaction for human colon cancer. Dig Dis Sci 2014; 59:1169-79; PMID:24798995; http://dx.doi.org/10.1007/s10620-014-3168-5
- Tahtinen S, Kaikkonen S, Merisalo-Soikkeli M, Gronberg-Vaha-Koskela S, Kanerva A, Parviainen S, Vaha-Koskela M, Hemminki A. Favorable alteration of tumor microenvironment by immunomodulatory cytokines for efficient T-cell therapy in solid tumors. PLoS One 2015; 10:e0131242; PMID:26107883; http://dx.doi.org/10.1371/journal.pone.0131242
- Koski A, Kangasniemi L, Escutenaire S, Pesonen S, Cerullo V, Diaconu I, Nokisalmi P, Raki M, Rajecki M, Guse K et al. Treatment of cancer patients with a serotype 5/3 chimeric oncolytic adenovirus expressing GMCSF. Mol Ther 2010; 18:1874-84; PMID:20664527; http://dx.doi.org/10.1038/mt.2010.161
- Gowen BB, Judge JW, Wong MH, Jung KH, Aylsworth CF, Melby PC, Rosenberg B, Morrey JD. Immunoprophylaxis of punta toro virus (phlebovirus, bunyaviridae) infection in hamsters with recombinant eimeria profilin-like antigen. Int Immunopharmacol 2008; 8:1089-94; PMID:18550012; http://dx.doi.org/10.1016/j.intimp.2008.03.019
- Adusumilli PS, Cherkassky L, Villena-Vargas J, Colovos C, Servais E, Plotkin J, Jones DR, Sadelain M. Regional delivery of mesothelin-targeted CAR T cell therapy generates potent and long-lasting CD4-dependent tumor immunity. Sci Transl Med 2014; 6:261ra151; PMID:25378643; http://dx.doi.org/10.1126/scitranslmed.3010162
- Freedman RS, Edwards CL, Kavanagh JJ, Kudelka AP, Katz RL, Carrasco CH, Atkinson EN, Scott W, Tomasovic B, Templin S. Intraperitoneal adoptive immunotherapy of ovarian carcinoma with tumor-infiltrating lymphocytes and low-dose recombinant interleukin-2: A pilot trial. J Immunother Emphasis Tumor Immunol 1994; 16:198-210; PMID:7834119; http://dx.doi.org/10.1097/00002371-199410000-00004
- Kanerva A, Zinn KR, Chaudhuri TR, Lam JT, Suzuki K, Uil TG, Hakkarainen T, Bauerschmitz GJ, Wang M, Liu B et al. Enhanced therapeutic efficacy for ovarian cancer with a serotype 3 receptor-targeted oncolytic adenovirus. Mol Ther 2003; 8:449-58; PMID:12946318; http://dx.doi.org/10.1016/S1525-0016(03)00200-4
- Hammerbeck CD, Hooper JW. T cells are not required for pathogenesis in the syrian hamster model of hantavirus pulmonary syndrome. J Virol 2011; 85:9929-44; PMID:21775442; http://dx.doi.org/10.1128/JVI.05356-11
- Ying B, Toth K, Spencer JF, Aurora R, Wold WS. Transcriptome sequencing and development of an expression microarray platform for liver infection in adenovirus type 5-infected syrian golden hamsters. Virology 2015; 485:305-12; PMID:26319212; http://dx.doi.org/10.1016/j.virol.2015.07.024
- Lobigs M, Mullbacher A, Blanden RV, Hammerling GJ, Momburg F. Antigen presentation in syrian hamster cells: Substrate selectivity of TAP controlled by polymorphic residues in TAP1 and differential requirements for loading of H2 class I molecules. Immunogenetics 1999; 49:931-41; PMID:10501835; http://dx.doi.org/10.1007/s002510050576
- Claesson MH, Endel B, Ulrik J, Pedersen LO, Skov S, Buus S. Antibodies directed against monomorphic and evolutionary conserved self epitopes may be generated in ‘knock-out’ mice. development of monoclonal antibodies directed against monomorphic MHC class I determinants. Scand J Immunol 1994; 40:257-64PMID:7519360; http://dx.doi.org/10.1111/j.1365-3083.1994.tb03459.x