ABSTRACT
Genome-wide association studies (GWAS) associated Family with sequence similarity 13, member A (FAM13A) with non-small cell lung cancer (NSCLC) occurrence. Here, we found increased numbers of FAM13A protein expressing cells in the tumoral region of lung tissues from a cohort of patients with NSCLC. Moreover, FAM13A inversely correlated with CTLA4 but directly correlated with HIF1α levels in the control region of these patients. Consistently, FAM13A RhoGAP was found to be associated with T cell effector molecules like HIF1α and Tbet and was downregulated in immunosuppressive CD4+CD25+Foxp3+CTLA4+ T cells. TGFβ, a tumor suppressor factor, as well as siRNA to FAM13A, suppressed both isoforms of FAM13A and inhibited tumor cell proliferation. RNA-Seq analysis confirmed this finding. Moreover, siRNA to FAM13A induced TGFβ levels. Finally, in experimental tumor cell migration, FAM13A was induced and TGFβ accelerated this process by inducing cell migration, HIF1α, and the FAM13A RhoGAP isoform. Furthermore, siRNA to FAM13A inhibited tumor cell proliferation and induced cell migration without affecting HIF1α. In conclusion, FAM13A is involved in tumor cell proliferation and downstream of TGFβ and HIF1α, FAM13A RhoGAP is associated with Th1 gene expression and lung tumor cell migration. These findings identify FAM13A as key regulator of NSCLC growth and progression.
Introduction
Lung cancer has the highest death rate of all cancer in the industrialized world.Citation1 Besides cigarette smoking, different other risk factors comprising gender and specific genetic traits are thought to contribute to lung cancer.Citation2
There are different approaches for treating lung cancer: surgery, chemotherapy, and/or radiation. Although nowadays there are more targeted therapies, the 5-year survival rate is still as low as 5–15%.Citation3
In the last years, genome-wide association studies (GWAS) identified some variant of the Family with sequence similarity 13, member A (FAM13A) gene to be strongly associated with chronic obstructive pulmonary disease (COPD)Citation4 and lung cancer,Citation5 especially in non-small cell lung cancer (NSCLC). NSCLC has three histologic subtypes: first the lung adenocarcinoma (ADC), which is responsible for 30 to 40% of NSCLC cases; then the lung squamous cell carcinoma (SCC), which has been associated to cigarette smoking;Citation6 and at last the large cell carcinoma (LCC).Citation7
Two FAM13A splice variants have been identified in humans, called FAM13A isoform 1 (v2) and FAM13A isoform 2 (v1). The FAM13A isoform 1 contains a Ras homologous GTPase-activating protein (RhoGAP) domain that is important for the regulation of cell proliferation and survival. The FAM13A isoform 2 (v1) does not contain the RhoGAP domain, indicating a distinct function with respect to the regulation of cellular pathways. In addition to FAM13A isoforms 1 and 2, about 23 FAM13A transcripts have been predicted whose biochemical and biological function is not explored so far. However, little is known about the role of FAM13A in tumor development, except for the existence of the RhoGAP domain in the isoform 1 of FAM13A, which is an important switch component of the cellular pathways controlling cell cycle and proliferation. The other known isoforms of FAM13A do not contain such a domain.Citation8,9
RhoGAP domains have the function to accelerate the dephosphorylation of GTP into GDP. Growth factors like epidermal growth factor (EGF), bind their membrane receptors, leading to the phosphorylation of GDP into GTP and therefore inducing the activation of the Rho protein. Then, the Rho protein connects to and activates other effector proteins involved in many cellular functions like cytoskeletal reorganization, polarity, and adhesion. The Rho protein also controls the proliferation and survival of the cell, which is of great relevance especially in tumor cell growth,Citation9 and indicates the importance of the regulation of this protein by the RhoGAP domains.
However, in tumor cells this modulating mechanism of the RhoGAP domains can be deranged and therefore fail to regulate the inhibition of the Rho proteins.Citation8,9
Recent results also suggest the possibility that FAM13A is involved in the WNT pathway, an important part of the regulation of adult tissue homeostasis. A dysregulation of the WNT pathway, however, causes severe consequences like tumourigenesis, which also points out the potential importance of the FAM13A in lung tumors.Citation10
In this study, we investigated the expression and function of FAM13A in tumor cells as well as in T cells to better understand the signaling pathways of these proliferating cells and eventually discover new targets for lung cancer therapy.
Results
Increased number of cells expressing FAM13A in the tumor region of patients with NSCLC as compared to the control region of the lung
To start to investigate the role of FAM13A in NSCLC, we first performed immuno-histochemical staining by using anti-FAM13A antibodies in histological lung tissue arrays obtained from lung tissue of our cohort of NSCLC patients with either ADC or SCC (; ). In addition, we immunostained histological tissue arrays of healthy control lung tissues (HC) to compare the FAM13A protein expression in the absence of lung cancer (; ). A semi-quantitative immunohistochemical evaluation of these histo-arrays indicated increased FAM13A positive cells in the lung tumor region in ADC and SCC as compared to their control areas and to the healthy controls (). We also confirmed these results with confocal fluorescence microscopy by staining one patient's slide with anti-FAM13A antibodies followed by an amplification system with a red fluorochrome and by DAPI blue counterstaining of the nuclei (). In conclusion, increased numbers of FAM13A+ cells could be confirmed in the tumor region and specifically in tumor cells of patients with NSCLC tumor.
Figure 1. Increased number of cells expressing FAM13A in the tumor region of patients with NSCLC. (A) Histological arrays obtained from the control (CTR) and tumoral region (TU) of lung tissue of our NSCLC patient cohort with adenocarcinoma (ADC) and squamous cell carcinoma (SCC) subtypes as well as lung tissue array from control patients (HC). (B) Immunohistochemistry (IHC) of FAM13A on lung tissue array: control (40x CTR) and tumoral (40x, 63x TU) area of patients with ADC or SCC as well as lung tissue from control patients (40x HC). Staining with anti-FAM13A antibody is shown in brown, negative controls (63x neg CTR) of control and tumoral area are depicted in the lower panel. Diagrams show semi-quantitative analysis of the immunohistochemical staining of ADC and SCC lung section samples comparing tumoral and control area of tumor patients and control area of healthy control patients (N (ADCcontrol) = 10; N (ADCtumoral) = 10; N (SCCcontrol) = 9; N (SCCtumoral) = 9; N (HC) = 10). (C) Confocal microscopic images of lung sections after immunofluorescence of the control (63x CTR) and tumoral (63x TU) area taken from the lung of a patient with SCC and the respective negative control (63x neg. CTR). Staining with anti-FAM13A antibody is shown in red and nuclei are counterstained in blue (DAPI). Data are shown as mean values ± s.e.m. using Student's two-tailed t-test *p = 0.05; **p = 0.01, ***p = 0.001.
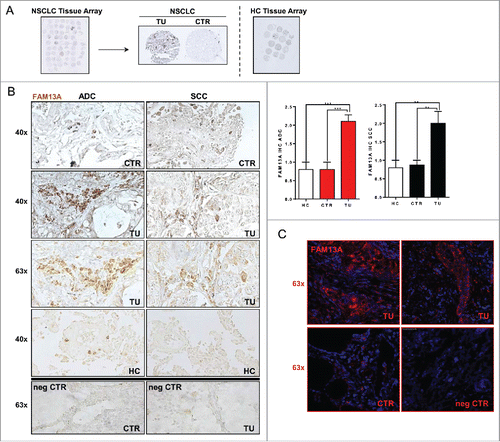
Table 1. Clinical data of the NSCLC patient cohort analyzed in this study.
Table 2. Clinical data of the cohort of control subjects analyzed in this study.
Increased FAM13A protein isoforms in the tumoral region of patients with NSCLC
To analyze the distribution of the FAM13A isoforms in our NSCLC patient cohort, we extracted proteins from the tumoral and control region of the lung tissue obtained after surgery. The anti-FAM13A antibody we used in this study, binds a C-terminal region that is present in both the FAM13A isoform 1 and 2 (). Here, we detected the FAM13A isoform 1 containing the RhoGAP domain with a molecular weight of about 117 kDa () in the tumor region of four out of seven ADC patients (MP-16, MP-22, MP-26, MP-28), while the FAM13A isoform 2 with a molecular weight of about 80 kDa () was present in the tumor region of five out of seven ADC patients (MP-16, MP-22, MP-23, MP-26, MP-28). Interestingly, we detected a third and a fourth FAM13A protein band with a molecular weight of about 40 kDa and 50 kDa, respectively () which could be related to additional, not yet described FAM13A isoforms. Regarding patients that suffer from SCC, neither the FAM13A isoform 1 nor the isoform 2 could be observed and we only detected the smaller FAM13A isoforms (). We finally found that the FAM13A total protein level, quantified by enclosing all FAM13A isoforms, is upregulated in the tumor as compared to the control area in both ADC and SCC (). In addition, in the ADC lung tumor subtype, we found generally higher FAM13A total protein levels as compared to the SCC subtype.
Figure 2. Increased FAM13A protein isoforms in the tumoral region of patients with NSCLC. (A) Schematic illustration of the Rho protein pathway and function of the RhoGAP domain of FAM13A. (B) Protein structure of FAM13A isoform 1 (v2) containing the RhoGAP domain (aa39-326, blue) with a total molecular weight of 117 kDa and of FAM13A isoform 2 (v1) with a molecular weight of 80 kDa. Binding sites of the anti-FAM13A antibody used in this study are depicted (aa910-971, yellow). (C) Western Blot analysis using anti-FAM13A antibody against total lung proteins isolated from the control and the tumoral area of patients with ADC and SCC (N (ADCcontrol) = 7; N (ADCtumoral) = 7; N (SCCcontrol) = 3; N (SCCtumoral) = 3). Red numbers indicate different FAM13A isoforms. (D) Western blot quantification of FAM13A total protein level, quantified by enclosing all FAM13A isoforms. Data are indicated as mean values ± s.e.m. using Student's two-tailed t-test *p = 0.05; **p = 0.01, ***p = 0.001.
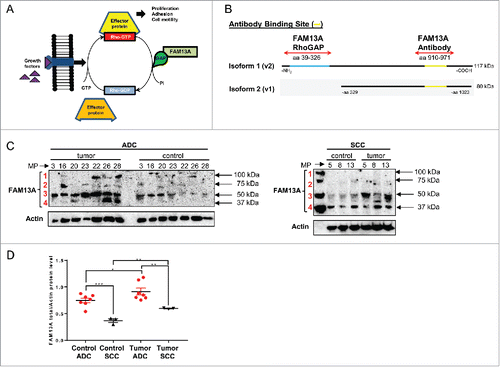
FAM13A RhoGAP is associated with T effector cells expressing Tbet and HIF1α but downregulated in CD4+CD25+Foxp3+CTLA4+ regulatory T cells
To investigate the expression of the FAM13A isoforms 1 and 2 in the CD4+CD25+ regulatory T cells and CD4+CD25− effector T cells, we designed two different primer pairs (). The first primer pair binds only the RhoGAP domain of the FAM13A isoform 1 at exon 2 to exon 3, whereas the second binds a region that is present in both isoforms. We next purified and sorted out CD4+CD25+ regulatory T cells and CD4+CD25− effector T cells from peripheral blood mononuclear cells (PBMC) of four healthy volunteers by using PE-labeled CD25 antibodies and anti-PE magnetic beads by depleting different cell populations (). CD25, the α chain of the IL-2 receptor, is an important surface molecule of T regulatory cells.Citation11 To determine the purity of the isolated cells, we performed FACS analysis which showed that about 85% of the CD4+CD25+ T cells expressed Foxp3 and were thus characterized as T regulatory cells (). Here, we found that FAM13A RhoGAP was consistently downregulated in CD4+CD25+Foxp3+ T regulatory cells and upregulated in CD4+CD25− T effector cells expressing Tbet (). Hypoxia induced factor 1 α (HIF1α) is induced in T cells during active proliferation when the cells use the glycolysis to generate energy.Citation12 HIF1α is also known to inhibit the development of regulatory T cells by degrading Foxp3.Citation13 Consistently, we found increased HIF1α in the effector CD4+CD25− T cells and a decrease in T regulatory cells where Foxp3 and other suppressive markers like CTLA4 were upregulated (). In conclusion, T regulatory cells purified from peripheral blood express Foxp3 and CTLA4 and inhibit FAM13A RhoGAP as well as HIF1α, two factors involved in T cell proliferation.
Figure 3. T regulatory cells purified from peripheral blood mononuclear cells express Foxp3 and inhibit FAM13A RhoGAP as well as HIF1α. (A) Binding sites of the primers used to analyze the expression of FAM13A isoforms 1 and 2. The first primer pair binds only the RhoGAP domain of the FAM13A isoform 1, whereas the second primer pair binds to a region that is present in both isoforms. (B) Experimental design of T regulatory cell isolation from healthy volunteers using PE labeled CD25 antibodies and anti-PE magnetic beads. (C) Purity of CD4+CD25+T cells (left panel) and CD4+CD25+Foxp3+ T cells (right panel) isolated using PE-labeled CD25 antibodies and anti-PE magnetic beads. (D) Quantitative real-time PCR analysis of FAM13ARHOGAP, TBX21, HIF1A, FOXP3, and CTLA4 in CD25− T effector cells (CD25−, N = 4) in comparison to CD25+ T regulatory cells (TREG, N = 4). (E) Correlation between CTLA4 mRNA expression and FAM13A protein level in the control region (CTR, N = 7) and tumoral region (TU, N = 9) of patients with NSCLC. (F) Correlation between FAM13A and HIF1A mRNA expression in the control region (CTR, N = 27) and tumoral region (TU, N = 24) of patients with NSCLC. Data are shown as mean values ± s.e.m. using Student's two-tailed t-test *p = 0.05; **p = 0.01, ***p = 0.001.
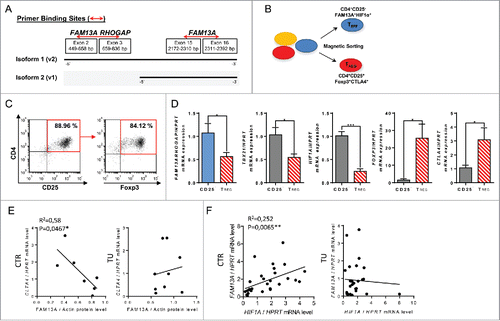
CTLA4 is a target of current immunotherapy for NSCLC because it is known to negatively regulate T cell receptor signaling.Citation14 As we mapped the presence of FAM13A with effector T cell function in healthy T cells, we reasoned that FAM13A could inversely correlate with molecules known to block T cell activation like CTLA4. Similarly, to the results in PBMCs isolated from healthy donors, we found an inverse correlation between FAM13A and CTLA4 expression in the lung control region of patients with NSCLC (). By contrast, in the presence of lung tumor cells, this correlation was lost (). In general, the function of FAM13A in tumor cells as well as in immune cells looks like to be linked to cell proliferation. Furthermore, FAM13A RhoGAP appears to be associated with CD4+CD25− effector T cells expressing Tbet together with HIF1α.
FAM13A positively correlated with HIF1α in the control region of the lung of patients with NSCLC
In developing lung tumor, the surrounding cells are often exposed to low oxygen levels and adapt by upregulating HIF1α to switch to glycolysis to gain energy.Citation15,16 We thus, next asked whether HIF1α expression in the tumor and in the surrounding lung tissue would correlate with FAM13A. Here, we found a positive correlation between HIF1α and FAM13A mRNA expression in the control lung region of patients with NSCLC (). In agreement with our results in CD4+CD25− T cells purified from PBMCs this data suggests the presence of increased FAM13A in antitumor CD4+CD25− effector T cells in the deoxygenated control area around the growing tumor. However, in the tumor region itself, we did not find any correlation (). In conclusion, FAM13A together with HIF1α seems to be associated with antitumor effector T cells in the control area surrounding the growing tumor.
Treatment with TGFβ reduces FAM13A, FAM13A RhoGAP, and HIF1A and induces programmed tumor cell death (apoptosis) in the lung adenocarcinoma cell line A549
TGFβ can act both as tumor suppressor and tumor promoter. In early stage cancers, it has been shown to inhibit tumor cell growth and proliferation as well as to induce apoptosis, whereas in advanced cancers TGFβ contributes to disease progression by the induction of cellular migration and metastasis.Citation17 Thus, we started to analyze if TGFβ influences FAM13A and its RhoGAP domain that has been described to be important for cancer cell proliferation and survival, as well. FACS analysis of untreated and TGFβ-treated A549 cells revealed a significant induction of early (Annexin V+/PI-) and late (Annexin V+/PI+) tumor cell apoptosis as well as a reduced number of living A549 cells ( and ). No downregulation of AKT, an important gene for cellular proliferation () or HIF1α () was detected after 3 ng/mL TGFβ treatment. Furthermore, TGFβ inhibited both the FAM13A isoform 1 containing the RhoGAP domain and the FAM13A isoform 2 (). By Western blot analysis, using the antibody that recognizes an epitope which is present in both isoforms as described in , we could verify a decrease of the FAM13A isoform 1 and 2 in cells treated with TGFβ in comparison to the unstimulated ones (). In addition, after 48 h incubation, we detected a third protein band with a molecular weight of about 250 kDa in the unstimulated condition that was inhibited by TGFβ, too. Again this could be another FAM13A isoform that is currently unknown. To determine the intracellular localization of FAM13A by immunohistochemistry with anti-FAM13A antibodies, cytospins with A549 cells were used. Here, a perinuclear and cytoplasmic localization of FAM13A could be mapped by confocal microscopy (). In this experiment, we could also confirm a decrease of FAM13A in cells treated with TGFβ in comparison to unstimulated cells (). In conclusion, TGFβ induced apoptosis in A549 cells and was associated with a FAM13A and FAM13A RhoGAP domain downregulation at RNA as well as at protein levels. To further analyze the effect of TGFβ on A549 lung ADC cell proliferation, we next performed a 96 h proliferation assay by using the cytoplasmic dye CFSE that is distributed to the daughter cells upon division. In accordance to this method, the proliferation is visible with a shift of the cell peak on the left-hand side and a decrease of fluorescence. Here, we demonstrated that increasing concentration of TGFβ (from 3 to 30 ng/mL) resulted in a decrease of A549 cell proliferation (). In conclusion, we found that 30 ng/mL of TGFβ had a higher suppressive effect on A549 cell proliferation as compare with 3 ng/mL.
Figure 4. Treatment with TGFβ reduces FAM13A mRNA levels and induces programmed tumor cell death in A549 cells. (A) Annexin V/PI FACS analysis of untreated A549 cells (UN, N = 3) in comparison to cells treated with 3 ng/mL of TGFβ (+3 ng TGFβ, N = 3). (B) Cell count of untreated A549 cells (UN, N = 3) in comparison to A549 cells treated with 3 ng/mL TGFβ (+3 ng TGFβ, N = 3). (C–E) Quantitative real-time PCR analysis of AKT (C), HIF1A (D), FAM13A, and FAM13ARHOGAP (E) mRNA expression in untreated (N (UN) = 3) and with 3 ng/mL TGFβ (+ 3 ng TGFβ = 3) treated A549 cells. (F) Western Blot analysis using anti-FAM13A antibody in untreated and with 30 ng/mL TGFβ treated A549 cells for 24 h and 48 h (left panel). Diagrams show the quantification of FAM13A isoforms. (G) Detailed view of fluorescence staining of A549 (+ 3 ng TGFβ) cytospins (63x) using anti-FAM13A antibody (red) and DAPI (blue) to counterstain the nuclei. (H) IHC of A549 cytospins (x40) left untreated (UN) or treated with 3 ng/mL TGFβ using anti-FAM13A antibody (brown). Negative controls (neg CTR) treated with PBS are shown on the right-hand side image. (I) CFSE proliferation assay of A549 cultured for 96 h in the presence of medium (unstimulated: black lane), 3 ng/mL of TGFβ (red lane) or 30 ng/mL of TGFβ (blue lane). Data are shown as mean values ± s.e.m. using Student's two-tailed t-test *p = 0.05; **p = 0.01, ***p = 0.001.
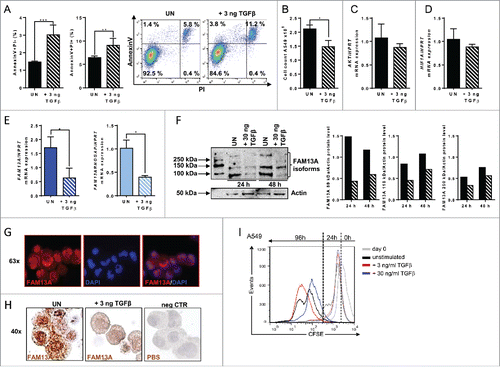
To look for further genes associated with FAM13A downregulation after TGFβ treatment in A549 cells, we performed RNA sequencing (RNA-Seq) by using Illumina technology ( and ). Here, we increased the doses of TGFβ treatment to 30 ng/mL and found that many genes involved in tumor cell proliferation including FAM13A and HIF1α were downregulated, whereas tumor suppressor genes and genes linked to cell migration were upregulated by TGFβ (Table S1). Consistently, Ki-67 a cell proliferation-associated marker,Citation18 was found downregulated in TGFβ-treated A549 lung tumor cells confirming its importance for the inhibition of cellular proliferation (). To validate the gene array data, we performed real-time PCR and confirmed the downregulation of FAM13A but not of FAM13A RhoGAP () indicating that higher doses of TGFβ do not result in downregulation of FAM13A RhoGAP but only FAM13A. Under these conditions also HIF1α and AKT were not significantly downregulated by TGFβ ().
Figure 5. Treatment with TGFβ decreases A549 cell proliferation alongside an upregulation of cellular migration genes. (A, B) RNA sequencing analysis (RNA-Seq) of RNA isolated from A549 cells treated with 30 ng/mL TGFβ (+ 30 ng TGFβ, N = 3) or left untreated (UN, N = 3). The log2 fold change of the RNA expression of significantly altered genes is shown as a heatmap (A) and as a bar chart (B), (red: upregulation; blue: downregulation). Selected genes belong to different classes: tumor suppressor and apoptosis (green), cell migration (pink), tumor promoter (blue) and proliferation (yellow). (C) Ki-67 proliferation FACS analysis of untreated (UN, N = 3) or with 30 ng/mL TGFβ (+ 30 ng TGFβ, N = 3) treated A549 cells. (D) Quantitative real-time PCR analysis of FAM13A, FAM13ARHOGAP, HIF1A, and AKT mRNA expression in untreated (UN, N = 3) and with 30 ng/mL TGFβ (+ 30 ng TGFβ, N = 3) treated A549 cells. Data are shown as mean values ± s.e.m. using Student's two-tailed t-test *p = 0.05; **p = 0.01, ***p = 0.001.
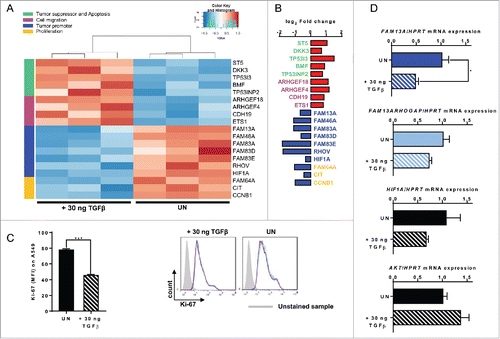
Targeted inhibition of FAM13A via siRNA in lung tumor cells: the role of FAM13A in controlling tumor cell proliferation and survival
To further analyze the role of FAM13A in human lung cancer cells, we silenced FAM13A in the human lung ADC cell line A549 via siRNA. Here, we found a specific downregulation of both the FAM13A isoform 1 containing the RhoGAP domain and the FAM13A isoform 2 at RNA level. By contrast, HIF1α was not found to be regulated upon siRNA treatment (). To visualize the success of the siRNA transfection green fluorescent protein (GFP) expressing vector was co-transfected (green) (). Moreover, we also performed RNA-Seq analysis by using Illumina technology () and found that many genes involved in tumor cell proliferation including FAM13A were downregulated, whereas tumor suppressor genes and genes linked to cell migration were upregulated (Table S2), similar to the results we obtained after the stimulation with TGFβ (). The specific knockdown of FAM13A and its RhoGAP domain reduced the proportion of total cells () as well as their proliferation as shown by decreased Ki67+ A549 cells further confirming the important role of FAM13A in tumor cell proliferation (). In conclusion, since HIF1α was not found downregulated after silencing both isoforms of FAM13A, we concluded that HIF1α is a factor downstream of TGFβ but upstream of FAM13A. Furthermore, we discovered that also FAM13A inhibited TGFβ () and TGFβ function via inhibition of TGFβRII (). In conclusion, we discovered an auto-regulative loop enclosing TGFβ and FAM13A.
Figure 6. Targeted inhibition of FAM13A in A549 cells via siRNA. (A) Quantitative real-time PCR analysis of FAM13A, FAM13AROHGAP, and HIF1A mRNA expression in untreated A549 cells (UN, N = 3) and in A549 cells transfected with non-targeting siRNA (siNT, N = 3) or FAM13A siRNA (siFAM13A, N = 3). Lower panel: detailed view of fluorescence staining of A549 transfected with siGFP (green) and counterstained with DAPI (blue) (63x). (B, C) RNA sequencing analysis (RNA-Seq) of RNA isolated from A549 cells transfected with FAM13A siRNA (siFAM13A, N = 3) or left untreated (UN, N = 3). The log2 fold change of the RNA expression of significantly altered genes is shown as a heatmap (B) and as a bar chart (C), (red: upregulation; blue: downregulation). Selected genes belong to different classes: tumor suppressor and apoptosis (green), cell migration (pink), tumor promoter (blue) and proliferation (yellow). (D) Cell count of untreated A549 cells (UN, N = 3) and A549 cells transfected with non-targeting siRNA (siNT, N = 3) or FAM13A siRNA (siFAM13A, N = 3). (E) Ki-67 proliferation FACS analysis of untreated A549 cells (UN, N = 3), A549 cells transfected with non-targeting siRNA (siNT, N = 3) or FAM13A siRNA (siFAM13A, N = 3). (F, G) Quantitative real-time PCR analysis of TGFB (F) and TGFBRII (G) mRNA expression in untreated A549 cells (UN, N = 3) and A549 cells transfected with non-targeting siRNA (siNT, N = 3) or FAM13A siRNA (siFAM13A, N = 3). Data are shown as mean values ± s.e.m. using Student's two-tailed t-test *p = 0.05; **p = 0.01, ***p = 0.001.
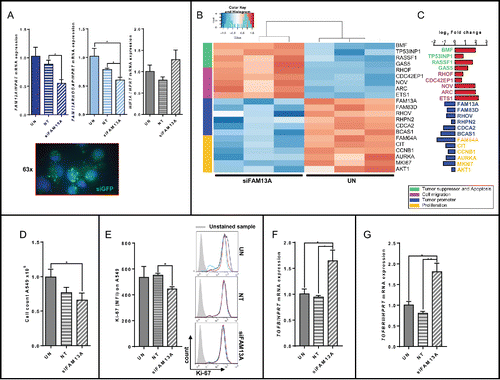
Increased FAM13A expression after scratching during cellular regeneration and migration of the lung adenocarcinoma cells A549: Regulatory role of TGFβ
As we found high levels of FAM13A protein in tumor cells and because of the importance of the RhoGAP domain for the tumor cell proliferation and survival as well as cytoskeletal reorganization and migration,Citation9 we started a series of scratch experiments with the human ADC cell line A549. A549 cell layer grown to confluence were scratched and observed for 48 h while cells were growing around the scratch (). We also isolated the mRNA from these A549 cells at different time points after the scratch and measured FAM13A mRNA levels. Soon after the scratch, both the FAM13A isoform 1 and FAM13A isoform 2 mRNA levels increased as the cells started to migrate during wound healing, thus to repair (). TGFβ is a potent inducer of growth arrest and apoptosis at early-stage of tumor development but has been also described to become a tumor-promoting factor in advanced cancers, especially by the induction of cellular migration and metastasis.Citation19 Therefore, we also analyzed the effect of TGFβ on cellular migration in the healing scratch and on FAM13A expression under these circumstances. Here, we found that TGFβ promotes cellular migration and accelerates wound repair (). Furthermore, 6 h, 12 h, and 24 h after scratching, TGFβ inhibited only the expression of the FAM13A isoform 2 but not the expression of the FAM13A isoform 1 containing the RhoGAP domain (). Moreover, and consistent with the role of TGFβ in tumor, we noticed that HIF1α was induced by TGFβ 12 h after the scratching (). Similarly to HIF1α also EGFR and ETS1 were induced at 12 h after scratching (). By contrast, TGFβ-induced FAM13A RhoGAP followed a sustained increase for 48 h as AKT did (). In conclusion, TGFβ induced tumor cell growth by activating EGFR and downstream FAM13A RhoGAP domain, AKT and ETS1 which results in cellular migration and growth. This cell growth induced hypoxia, thus activating HIF1α which also counteracts cell death.
Figure 7. Increased FAM13A expression during cellular migration of A549 cells around a cell wound: role of TGFβ. (A) Scratched A549 cell layer. Photos were taken 0, 6, 12, 24, and 48 h after the scratch to detect cellular migration. Upper panels show untreated (UN, N = 3) A549 cells, lower panels A549 cells treated with 3 ng/mL TGFβ (+ 3 ng TGFβ, N = 3). The scratch is highlighted in blue. (B) Relative migration rate in percent of scratched A549 cells left untreated or treated with 3 ng/mL TGFβ. (C) Cell count of scratched A549 cells left untreated (UN, N = 3) or treated with 3 ng/mL TGFβ (+ 3 ng TGFβ, N = 3). (D–I) Quantitative real-time PCR analysis of FAM13A (D), FAM13ARHOGAP (E), HIF1A (F), AKT (G), EGFR (H), and ETS1 (I) in scratched A549 cells left untreated (UN, N = 3) or treated with 3 ng/mL TGFβ (+ 3 ng TGFβ, N = 3). Data are shown as mean values ± s.e.m. using Student's two-tailed t-test *p = 0.05; **p = 0.01, ***p = 0.001.
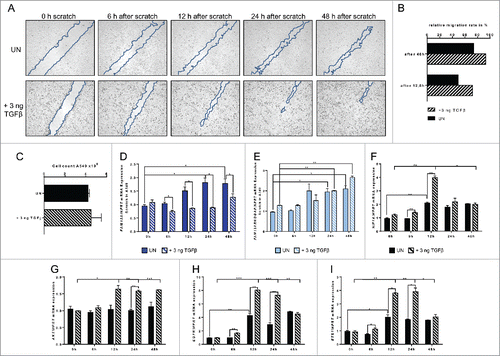
Effect of targeted inhibition of FAM13A via siRNA on cellular regeneration and migration of the lung adenocarcinoma cells A549
We next wanted to examine the effect of targeted inhibition of FAM13A via siRNA in the same environment. So, we transfected A549 cells with either non-targeting siRNA or siRNA against FAM13A, and repeated the scratch experiment (). In accordance to the RNA sequencing analysis and with the upregulation of genes that are important for cellular migration, we saw a movement of the cells into the scratched area 48 h after scratching. However, in the presence of FAM13A siRNA (), the cell density as well the cell number was reduced (). SiRNA to FAM13A resulted in a downregulation of FAM13A isoform 2 as well as FAM13A RhoGAP (). Thus, in contrast to the TGFβ scratch experiment, where we observed a progressive increase of the RhoGAP mRNA levels, the downregulation of FAM13A RhoGAP induced by siRNA treatment led to a decreased cell count after transfection (). Moreover, siRNA to FAM13A did not induce HIF1α (), indicating a reduced cell growth as compare with TGFβ treatment and confirming that HIF1α is upstream of FAM13A.
Figure 8. Effect of targeted inhibition of FAM13A via siRNA on cellular migration of A549 cells around a cell wound. (A) Scratched A549 cell layer after transfection with FAM13A siRNA (siFAM13A). Photos were taken 0, 6, 12, 24, and 48 h after the scratch to detect cellular migration. The scratch is highlighted in blue. (B) Relative migration rate in percent of scratched A549 cells left untreated (UN, N = 3) or transfected with FAM13A siRNA (siFAM13A, N = 3). (C) Cell count of scratched A549 cells left untreated (UN, N = 3) or transfected with FAM13A siRNA (siFAM13A, N = 3). (D–J) Quantitative real-time PCR analysis of FAM13A (D), FAM13ARHOGAP (E), HIF1A (F), AKT (G), EGFR (H), TGFB (I), and TGFBRII (J) in scratched A549 cells left untreated (UN, N = 3) or transfected with either NT (N = 3) or FAM13A siRNA (siFAM13A, N = 3). Data are shown as mean values ± s.e.m. using Student's two-tailed t-test *p = 0.05; **p = 0.01, ***p = 0.001.
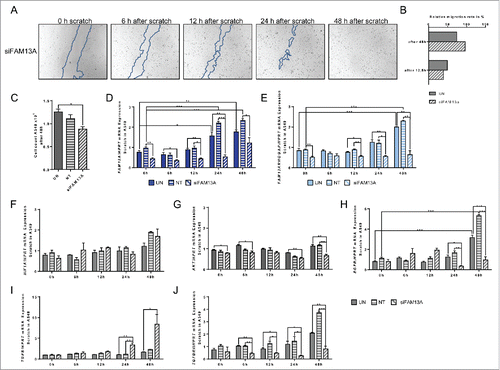
The lower cell growth after siRNA to FAM13A was also confirmed by downregulation of cell growth receptors like EGFR () and TGFβRII () as well as AKT () although TGFβ was induced (). Thus, FAM13A seems to induce cellular migration via Rho-dependent pathways and emerges as an important regulator in metastasis in lung ADC cells.
Discussion
The function of FAM13A as well as the pathways by which this gene and its RhoGAP domain influence the tumor and lymphatic cell life is largely unknown. In this paper, we mainly focused on the role of FAM13A in NSCLC proliferation and migration as well as its distribution in effector and regulatory T cells.
In the tumoral area of the lung of our patient cohort with ADC and SCC, we found increased FAM13A protein levels. Moreover, the FAM13A RhoGAP protein domain was mainly expressed in patients suffering from ADC. In the control area of the lung of these patients as well as in the lungs of healthy controls only a few cells were stained by anti-FAM13A antibodies.
Regulatory T cells play a crucial role in the immune escape of cancer cells Citation20 and we recently have shown that T regulatory cell markers are localized in the tumoral region of the lung of these NSCLC patients.Citation21 Thus, we next asked if FAM13A, which is upregulated in NSCLC tumor cells, might be important for the T regulatory cell development. In purified CD4+CD25+ Treg cells, we found a significant induction of regulatory T cell markers like Foxp3 and CTLA4 but a downregulation of FAM13ARHOGAP mRNA levels. Furthermore, the FAM13A RhoGAP domain was found increased in CD4+CD25− T effector cells together with HIF1α and the Th1 signature transcription factor Tbet. Therefore, we concluded that the FAM13A RhoGAP domain might be crucial for the development and survival of antitumor effector Th1 cells in a hypoxic environment.
The importance of CTLA4 as immunosuppressive therapeutical target in NSCLC patients has been recently evaluated.Citation22 Importantly, we found that FAM13A inversely correlates with CTLA4 but positively correlates with HIF1α in the control area around the tumor of NSCLC patients. As immune cells like activated T cells are limited at low oxygen level in the growing tumor area, they adapt by upregulating HIF1α in order to switch to anaerobic glycolysis to gain energy and to promote effector cell proliferation as well as the production of pro-inflammatory cytokines.Citation16,23 Therefore, the positive correlation between FAM13A and HIF1α in the control region of NSCLC patients support our hypothesis of the importance of FAM13A for the development of effector antitumor Th1 cells in adjacent tumor regions. In fact, we have previously reported increased Tbet expression in the peritumoral region of these patients.Citation21 Thus, FAM13A could play protective anti-tumoral roles in T cells in the control area surrounding the growing tumor. However, further studies are needed to clarify if FAM13A is a direct target of HIF1α. In conclusion, we found increased FAM13ARHOGAP mRNA levels in effector CD4+CD25−HIF1α+Tbet+ T cells. In contrast, decreased FAM13ARHOGAP mRNA levels were found in regulatory CD4+CD25+Foxp3+ T cells which inhibit the antitumor immune responses. Therefore, the RhoGAP domain seems to play a role in proliferation and migration of anti-tumoral T effector cells, which are suppressed by CTLA4+FOXP3+T regulatory cells in the control area of lung cancer patients. Additional direct supporting evidence is still needed to fully demonstrate this. This could also be in agreement with two GWA studies reported variants at the 4q22 locus, within the FAM13A gene associated with reduced risk of COPD. Sequence analysis of this gene indicated the presence of the RhoGAP in exon 2–5 in FAM13A thus indicating the possible tumor suppressive function of FAM13A via its RhoGAP domain. Moreover, a recent study proposed that augmented RhoGAP activity conferred by this FAM13A variants may be relevant in reducing the risk of both COPD and lung cancer.Citation4,5 Further studies need to be done to better understand the role of FAM13A in lung cancer as well as in COPD.
TGFβ, which we showed is able to inhibit FAM13A and its RhoGAP domain in A549 cells, plays also an important role in the development of T regulatory cells.Citation24 Therefore, the downregulation of FAM13ARhoGAP domain might be an important aspect of the development of T regulatory cells in the tumoral region of these patients. Further experiments are needed to verify this thesis.
In addition, TGFβ plays an important role in tumor cell life.
To confirm the thesis that FAM13A is connected to tumor cell proliferation, and is inversely correlated with TGFβ, we treated lung ADC A549 cell cultures with TGFβ. In our experimental setting, TGFβ upregulated early and late apoptosis in A549 cells at lower doses (3 ng/mL) and reduced A549 cell numbers as well as proliferation in comparison to untreated controls at higher doses (30 ng/mL). In accordance to this finding, even after the lower doses of TGFβ treatment, we saw a decrease of not only FAM13A but also the FAM13ARhoGAP domain at RNA as well as protein level, indicating a connection between TGFβ and FAM13A, especially its RhoGAP domain, and cellular survival. By increasing the doses of TGFβ, we saw an increased suppressive effect on A549 by CFSE analysis. This is also supported by RNA-Seq analysis of A549 cells treated with increased doses of TGFβ. Here, we found an upregulation of tumor suppressor and apoptosis genes as ST5, DKK3, TP53I3, BMF, and TP53INP2 as well as of genes connected to cellular migration as ARHGEF4, ARHGEF18, CDH19, and ETS1 (Table S1). Conversely, we saw that higher doses of TGFβ led to a downregulation of only FAM13A but not its RhoGAP domain. Similar to FAM13A other tumor promoter genes as HIF1α, RHOV, FAM83A-D, and FAM46A as well as genes which are important for cellular proliferation as FAM64A, CIT, and CCNB1 were downregulated after TGFβ treatment (Table S1). This supports the thesis that FAM13A and its RhoGAP domain being possible tumor suppressor genes and implicates their importance for suppression of tumor cellular proliferation.
To further examine the role of FAM13A in human lung cancer cells, we transfected A549 cells with FAM13A siRNA. Here, we observed a decrease in cell number and proliferation after the downregulation of FAM13A and its RhoGAP domain upon siRNA treatment, in comparison to cells treated with non-targeting siRNA. As consequence, there was a decrease in cell numbers and proliferation proved via Ki-67 FACS analysis. However, HIF1α was not found downregulated as after TGFβ treatment, indicating that HIF1α is downstream of TGFβ but upstream of FAM13A.
In order to identify further cellular changes after the siRNA treatment, we performed RNA-Seq analysis. In accordance with the TGFβ treatment, there was an upregulation of tumor suppressor genes as BMF, TP53INP1, RASSF1, and GAS5 and genes correlated with cell migration like as RHOF, CDC42EP1, NOV, ARC, and ETS1 (Table S2). In contrast, we saw a downregulation of tumor promoter genes as FAM83D, RHOV, RHPN2, CDCA2, and BCAS1, as well as genes which are important for cellular proliferation, for example, FAM64A, CIT, CCNB1, AURKA, MKI67, and AKT1 (Table S2). These results confirm our conclusions about the cellular function of FAM13A and its RhoGAP domain.
To analyze the role of FAM13A in cancer cell metastasis, we performed a series of cell scratch experiments. Both TGFβ and the knockdown of FAM13A via siRNA accelerated cell migration of A549 cells in this experimental setting. In addition, whereas TGFβ beside apoptosis induced also A549 cell proliferation during wound healing, as demonstrated by transient induction of HIF1α, EFGR, and AKT, FAM13A siRNA knockdown inhibited cell proliferation during migration as indicated by lower A549 cell numbers, decreased AKT, EGFR, and TGFβRII.Citation25,26 In conclusion, the knockdown of FAM13A RhoGAP domain by siRNA promotes the activity of Rho proteins thus resulting in random cell migration while the reduced cancer cell growth could be explained by the inhibition of the EGFR-AKT pathway.Citation25,27,28
TGFβ is known to act as tumor-suppressor in benign cells by inducing apoptosis or inhibit tumor cell proliferation as we also confirmed in this study. However, in advanced cancers TGFβ begins to actively contribute to tumor progression and has been shown to induce cellular migration and metastasis.Citation17,26 After TGFβ treatment, we found that A549 cells migrated faster and expressed a reduced amount of FAM13A mRNA as compare with the unstimulated control. In contrast, we found increased FAM13ARHOGAP mRNA levels as the cells started to migrate entirely unaffected by TGFβ treatment. In this setting, the migration of A549 cells seems to be induced by Rho-independent pathways. Moreover, migration of A549 cells could be promoted by the RAS/ERK signaling pathway, as we found here increased ETS1 expression. In fact, this pathway is known to induce ETS1.Citation29 In addition, we expected a decrease in A549 cell number because of the TGFβ treatment. Against these expectations, FAM13ARhoGAP seems to counteract the effect of TGFβ with respect to cellular proliferation and therefore results in constant cell numbers during migration. In confirmation of this, we found a transient increase of HIF1α 12 h after scratching and TGFβ treatment. In conclusion, knockdown of the FAM13A RhoGAP domain during migration seems to inhibit A549 cell proliferation. For tumoral metastasis driven by TGFβ, it is important to maintain a high proliferation even during cell migration. Therefore, to accelerate cell migration of cancer cells, TGFβ seems to only inhibit FAM13A while the FAM13A RhoGAP domain is still increasing to ensure constant cellular proliferation.
Even though the exact mechanism how FAM13A influences the cell cycle remains unclear, our studies suggest that especially the RhoGAP domain of FAM13A is important for cellular proliferation and survival and thus might be the next potential target for an anti-proliferative lung tumor therapy in order to arrest cell cycle as well as to inhibit cellular migration and thus invasion associated with metastasis.
Material and methods
Human subjects and study population
This study was performed at the Friedrich-Alexander-Universität Erlangen-Nürnberg, Germany and was approved by the ethics review board of the University of Erlangen (Re-No: 56_12B; DRKS-ID: DRKS00005376). Thirty-six patients, who were suffering from NSCLC, underwent surgery and gave their approval to being enrolled in this study. Patients' confidentiality was maintained.
The diagnosis of lung cancer was based on pathological confirmation. The histological types of lung cancer were classified according to the classification of the World Health Organization (WHO), formulated in 2004. The staging of lung cancer was based on the Cancer TNM Staging Manual, formulated by the International Association for the Study of Lung Cancer (IASLC) in 2010.
Tissue samples were taken from the tumor area (TU: solid tumor tissue) as well as from the tumor-free control area (CTR: at least 5 cm away from the solid tumor) of the surgically removed lung material.
Immunohistochemistry (IHC) of FAM13A on paraffin-embedded lung tissue sections
For dewaxing the paraffin-embedded slides with human lung cancer samples, arrays were heated at 72°C for 30 min, washed with roti-histol (Roth, 6640.4, Karlsruhe, Germany) two times for 10 min, with ethanol for 9 min (3 min each at 100%, 95%, and 70% EtOH concentration) and at last with purified water for 1 min. For antigen retrieval, the slides were heated in an autoclave (5 min at 120°C) and cooled down for 20 min in TrisEDTA buffer. After washing with purified water, slides were surrounded with a hydrophobic barrier using a barrier pen (Dako, Hamburg, Germany). Afterwards Dual endogenous enzyme block (Dako, K4065, Hamburg, Germany) was applied for 10 min at room temperature and slides were washed again with washing buffer (Dako, S3006, Hamburg Germany) for 5 min, followed by the incubation with the primary antibody to FAM13A 1:1000 (Sigma-Aldrich, HPA038109, Munich, Germany) for 2 h. Then, the slides were washed again with washing buffer for 5 min, incubated with labeled Polymer-HPR (Dako, K4065, Hamburg, Germany) for 30 min and washed with washing buffer for 5 min. Next, the tissue sections were treated with DAB chromogen solution (Dako, K4065, Hamburg Germany) for 10 min, then carefully rinsed with purified water and washed with hemalaun solution (Roth, T865.2, Karlsruhe, Germany) for 5 min. Afterwards slides were rinsed in water for 1 min, washed in purified water for 5 min and treated with ethanol for 9 min (3 min each at 70%, 95%, and 100% EtOH concentration). Aftewards, samples were incubated with roti-histol (Roth, 6640.4, Karlsruhe, Germany) for 20 min and were covered with coverslips using Entellan (Merck, HX255673, Darmstadt, Germany). Negative controls were not treated with a primary antibody; the other steps remain the same.
For confocal microscopy, after dewaxing and heating in an autoclave, slides were incubated with normal goat serum blocking solution 1:10 (Vector, S-1000, California) for 2 h at room temperature, then washed for 9 min in PBS and incubated with Streptavidin (Vector, SP-2002, California) for 15 min. After washing, the slides were incubated another 15 min with Biotin (Vector, SP-2002, California) and washed again. Now, the primary antibody FAM13A 1:1000 (Sigma-Aldrich, HPA038109, Munich, Germany) was added. After 2 h, slides were washed and incubated with the second antibody biotinylated goat anti-rabbit 1:300 (Vector, BA-1000, California) for 30 min. Then, the slides were washed again, the last antibody DyLight 549 1:200 (Dianova, Hamburg, Germany) was added for 30 min and after another washing step slides were covered with coverslips using Vectashield Mounting Medium and DAPI (Vector, H-1200, California).
Protein extraction and Western blot analysis
Lung tissue samples from patient collective described above were lysed with RIPA Buffer (Thermo Fisher Scientific, Waltham, MA, USA) and inhibitor cocktail (Complete Mini EDTA-free, Roche Diagnostics, Mannheim, Germany) followed by homogenization using SpeedMill PLUS (Analytik Jena, Jena, Germany) in innuSPEED lysis Tube P (Analytik Jena, Jena Germany). After further extraction by centrifugation the protein concentration was measured using Bradford Assay (Protein Assay Dye Reagent Concentration, Bio-Rad, Munich, Germany), followed by protein denaturation (95°C, 5 min) in reducing loading buffer (Carl Roth, Karlsruhe, Germany). A 10% SDS-PAGE (PAGEr Ex Gels, Lonza, Rockland, USA) was performed with 50 µg of protein at 106 V, 150 mA for 1 h. Proteins were then transferred to a 0.45 µm nitrocellulose membrane (GE Healthcare, Munich, Germany) at 19 V, 200 mA for 1 h. After washing and blocking of the membrane for 1 h at room temperature, the primary antibody against FAM13A 1:200 (HPA038109, Sigma-Aldrich, Munich, Germany) was applied and incubated over night at 4°C. Next day, the membrane was washed and incubated with the HRP-conjugated secondary antibody 1:1000 (rabbit IgG, GE Healthcare, Munich, Germany) solved in blocking buffer, for 1 h at room temperature. As control the primary antibody against Aktin 1:250 (sc-1616, Santa Cruz) and a HPR-conjugated secondary antibody against goat IgG 1:1000 (Lab Vectors, California, USA) were used. After final washing steps, detection was performed using the Western Bright Quantum Western Blotting detection kit (Biozym Scientific, Oldendorf, Germany). For the visualization of the western blot results the Fluor Chem FC2 (Biozym Scientific, Oldendorf, Germany) detection system was used.
CD4+CD25+ T cell isolation from PBMCs
PBMCs were isolated from volunteer's blood using Lymphoprep (Axis-Shield PoC AS) under the Ethic vote number 4556 at the Friedrich-Alexander-Universität Erlangen-Nürnberg, Germany.
Afterwards 10×106 cells were stained with 5 µL of PE labeled CD25 antibodies, enriched with anti-PE magnetic beads (Miltenyi Biotec) following the manufacturer's protocol and stained again with CD4-FITC and CD45RA-APC. Then, the cells were sorted using the FACS Aria II Special Order System (BD Biosciences), followed by the restimulation of 10 × 104 sorted T regulatory cells in L929 medium with L929 cells containing 100 ng/mL OKT3 and 100 ng/mL anti-CD28 antibody with 300 U/mL IL-2 (Proleukin S, Novartis). Cells were incubated at 37°C and 5% CO2 for 72 h, and after renewal of the L929 medium with 300 U/mL IL-2 again incubated for another 48 h. Then, 25 × 104 T regulatory cells were incubated in 4 mL L929 medium with 40 × 104 L929 cells containing 100 ng/mL anti-CD28 and OKT3 with 300 U/mL IL-2 for 48 h on six-well plates, and, after the renewal of 2 mL medium, again incubated for another 48 h. Afterwards the cells starved for 72 h in L929 medium with 300 U/mL IL-2 without feeder cells and OKT3 and anti-CD28, then they were re-stimulated by anti-CD3/CD28 beads (Life Technologies/Invitrogen) for 24 h. RNA was isolated from the cells to determine the mRNA levels of FAM13A, FAM13ARHOGAP, AKT, TBX21, FOXP3, and HIF1A.
Quantitative real-time PCR (qPCR)
Total RNA was extracted from frozen tissue samples or from cell-suspension samples, using peqGold RNA Pure (Peqlab, Erlangen, Germany) according to the manufacturer´s instructions. 1 µg of the resulting RNA was reverse-transcribed into cDNA via the RevertAid™ First Strand cDNA Synthesis Kit (Fermentas, St. Leon-Rot, Germany) according to manufacturer´s protocol. Each qPCR reaction mix contained 15 ng of cDNA, 300 nM transcript-specific forward and reverse primer and 2x SoFast™ EvaGreen® Supermix (BIO-RAD, Munich, Germany) in a total volume of 20 µL. QPCR primers were purchased from Eurofins-MWG-Operon, Ebersberg, Germany. The primer sequences are given for HPRT (fw: 5′-TGA CAC TGG CAA AAC AAT GCA-3′; rev: 5′-GGT CCT TTT CAC CAG CAA GCT-3′), FAM13A (fw: 5′-ATG ATG ATG AGC AAG AGG ACC T-3′; rev: 5′-CGG ATT GGC TGC TTT GTC ACT-3′), FAM13ARHOGAP (fw: 5′-GGGAGCTGCCTGACAGTCT-3′; rev 5′-GAGGAGGCAGTAGTGGGTGT-3′); HIF1A (fw: 5′-ATT TTG GCA GCA ACG ACA CA-3′; rev: 5′-CGT TTC AGC GGT GGG TAA TG-3′), AKT (fw: 5′-CAC ACC ACC TGA CCA AGA TGA-3′; rev: 5′-CCT CCA AGC TAT CGT CCA-3′); EGFR (fw: 5′-CTTCTGGAGGGTGAGCCAAG-3′; rev: 5′-GCGATGGACGGGATCTTAGG-3′), ETS1 (fw: 5′-TGG AGT CAA CCC AGC CTA TC-3′; rev: 5′-TCT GCA AGG TGT CTG TCT GG-3′), TGFB (fw: 5′-CAC GTG GAG CTG TAC CAG AA-3′; rev: 5′-GAA CCC GTT GAT GTC CAC TT-3′), TGFBRII (fw: 5′-TTT TCC ACC TGT GAC AAC CA-3′; rev: 5′-GGA GAA GCA GCA TCT TCC AG-3′), FOXP3 (fw: 5′-AAC AGC ACA TTC CCA GAG TTC CT-3′; rev: 5′-CAT TGA GTG TCC GCT GCT TCT-3′), CTLA4 (fw: 5′-CTC TGG ATC CTT GCA GCA GT-3′; rev: 5′-GCC TCA GCT CTT GGA AAT TG-3′), TBX21 (fw: 5′- CAGAATGCCGAGATTACTCAG-3′; rev: 5′- GGTTGGGTAGGAGAGGAGAG-3′). Reactions were performed for 50 cycles with an initial activation for 2 min at 98°C, denaturation for 5 min at 95°C and hybridization and elongation for 10 min at 60°C. QPCR reactions were performed using the CFX-96 Real-Time PCR Detection System (BIO-RAD, Munich, Germany), and analyzed via the CFX Manager Software. The relative expression level of specific transcripts was calculated with respect to the internal standard (HPRT). Expression levels were normalized to control lung tissues using CT calculation. For comparison of the expression of different genes, efficiency-corrected calculation of relative gene expression, normalized with HPRT, was performed using the relative standard curve method.
A549 cell culture and FACS apoptosis assay
For cell death analysis, 1×105 A549 cells were incubated in 48-well-plates for 48 h in 1 mL medium (Gibco F-12 Nut Mix, Thermo Fisher Scientific, Erlangen) supplemented with 10% FCS, 1% Penicillin+Streptomycin and 1% L-Glutamine, either unstimulated or treated with 3 ng/mL of TGFβ (PeproTech, Hamburg). Cells were incubated at 37°C, 5% CO2 and 96% RH. Apoptosis assay was performed by staining the cells with Annexin V (AnnV) (BD Bioscience, Heidelberg, Germany) and Propidium Iodide (PI) (BD Bioscience, Heidelberg, Germany) according to the manufacturer's protocol followed by flow cytometry analysis. Furthermore, RNA was extracted from the respective cells to perform qPCR.
In addition, RNA-Seq was performed to identify TGFβ driven gene expression in lung tumor cells. For this purpose, 3 × 105 A549 cells were cultured in six-well-plates for 24 h in 3 mL medium (Gibco F-12 Nut Mix, Thermo Fisher Scientific, Erlangen) supplemented with 0,4% FCS, 1% Penicillin+Streptomycin and 1% L-Glutamin, either unstimulated or treated with 30 ng/mL of TGFβ (PeproTech, Hamburg). Cells were incubated at 37°C, 5% CO2 and 96% RH. After RNA isolation, RNA-Seq of the respective probes was performed in collaboration with the Institute of Human Genetics, Friedrich-Alexander-Universität Erlangen-Nürnber.
A549 proliferation assay
To determine the proliferation of A549 ADC cells in response to TGFβ, 1×105 cells were cultured for 4 d (96 h) in medium (Gibco F-12 Nut Mix, Thermo Fisher Scientific, Erlangen) supplemented with 10% FCS, 1% Penicillin+Streptomycin and 1% L-Glutamine after staining with CFSE (5 µM) in PBS with 0.1% BSA on day 0. The tumor cells were stimulated with 3 and 30 ng/mL recombinant TGFβ (PeproTech, Hamburg). On day 0, 1, and 4, the proliferation was measured by flow cytometry (FACSCaliburTM, BD Biosciences, Heidelberg).
Immunohistochemistry (IHC) of FAM13A on cytospins
Cytospins were prepared using 1×105 A549 ADC cells in 100 µL medium (Gibco F-12 Nut Mix, Thermo Fisher Scientific, Erlangen) supplemented with 10% FCS, 1% Penicillin+Streptomycin and 1% L-Glutamine and fixed with −20°C acetone. The cytospins were surrounded with a hydrophobic barrier using a barrier pen (Dako, Hamburg, Germany). Afterwards, Dual endogenous enzyme block (Dako, K4065, Hamburg, Germany) was applied on the slides for 10 min and after washing for 5 min the primary antibody FAM13A 1:1000 (Sigma-Aldrich, HPA038109, Munich, Germany) was added for 2 h. Next, the cytospins were washed and incubated with labeled Polymer-HPR (Dako, K4065, Hamburg, Germany) for 30 min. After another washing step, slides were treated with DAB chromogen solution (Dako, K4065, Hamburg Germany) for 10 min, rinsed with purified water, and washed for 5 min in hemalaun solution (Roth, T865.2, Karlsruhe, Germany). Afterwards, the cytospins were washed with purified water, with ethanol (3 min each at 70%, 95%, and 100% EtOH concentration), stayed for 10 min in roti-histol (Roth, 6640.4, Karlsruhe, Germany) and were covered with coverslips using Entellan (Merck, HX255673, Darmstadt, Germany). Negative controls were not treated with a primary antibody; the other steps remain the same.
For fluorescence: After preparing the cytospins, the cells were surrounded with a hydrophobic barrier, then cells were incubated in Paraformaldehyd (Roth, 0335.1, Karlsruhe, Germany) for 10 min, then washed with PBS and TritonX-100 (Roth, 3051.3, Karlsruhe, Germany) was added for 4 min. Followed by another washing step, the slides were treated with blocking buffer bovine serum albumin and Tween20 (Roth, 9127.1, Karlsruhe, Germany) for 40 min, washed again and incubated with the primary antibody FAM13A 1:150 (Sigma-Aldrich, HPA038109, Munich, Germany). After 2 h, the cytospins were washed followed by a treatment with the second antibody biotinylated goat α rabbit 1:300 (Vector, BA-1000, California) for 30 min and washed again. Now Cy3 Streptavidin 1:200 (Jackson ImmunoResearch, 016–160–084, Pennsylvania) was added for 1 h, followed by washing and the coverage of the slides with coverslips using Dako Mounting Medium Fluorescence and DAPI 1:1000 (Life Technologies, S3023, California). Negative controls were not treated with a primary antibody; the other steps remain the same.
Determination of proliferating A549 cells using Ki-67 staining
Cells were harvested as described above and pelleted by centrifugation. To each pellet 500 µL of cold 75% ethanol were added dropwise while vortexing and incubated for at least 48 h at −20°C. Fixed cells were then washed twice (1,000 rpm, 10 min, 4°C) with 3 mL wash buffer containing PBS, 1% FCS and 0,09% NaN3 and resuspended in 100 µL wash buffer. For Ki-67 staining, 20 µL of FITC mouse anti-Ki-67 antibody (BD PharMingen, Heidelberg) were added to the cell suspension, mixed gently and incubated at room temperature for 25 min in the dark. The reaction was stopped by adding 2 mL of wash buffer, followed by centrifugation and re-suspension in 250 µL wash buffer. After adding 5 µL of PI Staining Solution, (BD PharMingen, Heidelberg) the samples were analyzed by flow cytometry (FACSCaliburTM, BD Biosciences, Heidelberg).
Transfection of A549 cells
For siRNA-mediated knockdown of FAM13A, 3 × 105 A549 cells were cultured overnight in six-well plates in 2 mL antibiotic-free medium (Gibco F-12 Nut Mix, Thermo Fisher Scientific, Erlangen) supplemented with 10% FCS and 1% L-Glutamine. Afterwards, cells were transfected with 25 nM FAM13A siRNA (GGAGAACUCUUAGAAAGAAUU, GE Dharmacon) in combination with 6 µL DharmaFECT Transfection reagent 1 (GE Dharmacon) according to the manufacturer's instructions. Transfected A549 cells were incubated for 24 h followed by RNA isolation and subsequent analysis of FAM13A as well as FAM13ARHOGAP knockdown via qPCR. As controls we used siGFP (GCAAGCUGACCCUGAAGUUCUU, GE Dharmacon) as well as non-targeting siRNA.
RNA sequencing (RNA-Seq) analysis
To identify TGFβ and FAM13A driven gene expression in lung tumor cells, RNA-Seq of the respective probes was performed in collaboration with the Institute of Human Genetics, Friedrich-Alexander-Universität Erlangen-Nürnber.
After RNA extraction using the RNeasy Micro Kit (QIAGEN, Redwood City, CA), the quality of RNA was analyzed using a 2100 Bioanalyzer system (Agilent Technologies). Barcoded RNA-sequencing libraries were prepared from 100 ng total RNA using the Ovation Human RNA-Seq system for small amounts of RNA, according to the manufacturer's instructions (Nugen, San Carlos, CA). For selective depletion of rRNAs, the insert-dependent adaptor cleavage method from Nugen was used prior to cDNA synthesis. To control sources of variability during sample and data processing, a common set of external spike-in RNA controls, developed by the external RNA controls consortium (ERCC), was used (Ambion).
Libraries were subjected to single-end sequencing (101 bp) on a HighSeq-2500 platform (Illumina, San Diego, CA). Reads mapping to, e.g., rRNAs, tRNAs, snRNAs, and interspersed repeats were first filtered out by performing alignment against a manually curated filter reference file using bwa-mem v. 0.7.8-r455 Citation30 and keeping only unmapped reads. Subsequently, these reads were mapped against the hg19 reference genome using the STAR aligner v. 2.4.0j,Citation31 and a STAR genome directory created by supplying an Ensembl gtf annotation file (genebuild 2013–09) for hg19. Read counts per gene were obtained using featureCounts program v. 1.4.6-p2 Citation32 and the Ensembl gtf annotation file.
Following analyses were performed using R version 3.2.1.Citation33 In particular, differential expression analysis was performed with the DESeq2 package v.1.8.1.Citation34 Genes were considered differentially expressed if their Benjamini-Hochberg adjusted p-value was less than 0.01. Heatmaps of regularized log-transformed data for selected differentially expressed genes were obtained relying on the package gplots v. 2.17.0 Citation35 and using gene values standardized across samples.
Migration/scratch assay
For the analysis of A549 cell migration, 3 × 106 cells were seeded into cell-culture petri dishes (60 × 15 mm, Greiner Bio-One) in 4 mL medium (Gibco F-12 Nut Mix, Thermo Fisher Scientific, Erlangen) supplemented with 10% FCS, 1% Penicillin+Streptomycin and 1% L-Glutamin and grown to 100% confluence. Cells were incubated at 37°C, 5% CO2 and 96% RH. By scratching the cell monolayer with a micropipette tip, wounds were created followed by detection of cell migration of either unstimulated A549 cells, A549 cells treated with 3 ng/mL TGFβ (PeproTech, Hamburg), or transfected with non-targeting or FAM13A siRNA as described above. Scratched A549 cell layer was monitored over a period of 48 h using JuLI™ Br live cell movie analyzer (NanoEnTek). The extent of healing was calculated by using a computer program ImageJ and was defined as the difference between the original (100%) and the resulting cell-free wound area expressed in percentage.
Statistical analysis
Differences were evaluated for significance (*p = 0.05; **p = 0.01, ***p = 0.001) by the Student's two-tailed t-test for independent events (Excel, PC). For RNA-Seq, we used adjusted p-value <0.01. Data are given as mean values ± SEM.
Graphs were created with GraphPad Prism, Windows.
Disclosure of potential conflicts of interest
No potential conflicts of interest were disclosed.
KONI_A_1256526_s02.docx
Download MS Word (51.2 KB)References
- Ferlay J, Shin HR, Bray F, Forman D, Mathers C, Parkin DM. Estimates of worldwide burden of cancer in 2008: GLOBOCAN 2008. Int J Cancer 2010; 127:2893-917; PMID:21351269; http://dx.doi.org/10.1002/ijc.25516
- Mitsudomi T. Molecular epidemiology of lung cancer and geographic variations with special reference to EGFR mutations. Transl Lung Cancer Res 2014; 3:205-11; PMID:25806302; http://dx.doi.org/10.3978/j.issn.2218-6751.2014.08.04
- Molina JR, Yang P, Cassivi SD, Schild SE, Adjei AA. Non-small cell lung cancer: epidemiology, risk factors, treatment, and survivorship. Mayo Clin Proc 2008; 83:584-94; PMID:18452692; http://dx.doi.org/10.1016/S0025-6196(11)60735-0
- Cho MH, Boutaoui N, Klanderman BJ, Sylvia JS, Ziniti JP, Hersh CP, DeMeo DL, Hunninghake GM, Litonjua AA, Sparrow D et al. Variants in FAM13A are associated with chronic obstructive pulmonary disease. Nat Genet 2010; 42:200-2; PMID:20173748; http://dx.doi.org/10.1038/ng.535
- Young RP, Hopkins RJ, Hay BA, Whittington CF, Epton MJ, Gamble GD. FAM13A locus in COPD is independently associated with lung cancer - evidence of a molecular genetic link between COPD and lung cancer. Appl Clin Genet 2011; 4:1-10; PMID:23776362; http://dx.doi.org/10.2147/TACG.S15758
- Kenfield SA, Wei EK, Stampfer MJ, Rosner BA, Colditz GA. Comparison of aspects of smoking among the four histological types of lung cancer. Tob Control 2008; 17:198-204; PMID:18390646; http://dx.doi.org/10.1136/tc.2007.022582
- Travis WD, Travis LB, Devesa SS. Lung cancer. Cancer 1995; 75:191-202; PMID:8000996; http://dx.doi.org/10.1002/1097-0142(19950101)75:1+%3c191::AID-CNCR2820751307%3e3.0.CO;2-Y
- Corvol H, Hodges CA, Drumm ML, Guillot L. Moving beyond genetics: is FAM13A a major biological contributor in lung physiology and chronic lung diseases? J Med Genet 2014; 51:646-9; PMID:25163686; http://dx.doi.org/10.1136/jmedgenet-2014-102525
- Malliri A, Collard JG. Role of Rho-family proteins in cell adhesion and cancer. Curr Opin Cell Biol 2003; 15:583-9; PMID:14519393; http://dx.doi.org/10.1016/S0955-0674(03)00098-X
- Jin Z, Chung JW, Mei W, Strack S, He C, Lau GW, Yang J. Regulation of nuclear-cytoplasmic shuttling and function of Family with sequence similarity 13, member A (Fam13a), by B56-containing PP2As and Akt. Mol Biol Cell 2015; 26:1160-73; PMID:25609086; http://dx.doi.org/10.1091/mbc.E14-08-1276
- Chen W, Konkel JE. Development of thymic Foxp3(+) regulatory T cells: TGF-beta matters. Eur J Immunol 2015; 45:958-65; PMID:25684698; http://dx.doi.org/10.1002/eji.201444999
- Gaber T, Strehl C, Sawitzki B, Hoff P, Buttgereit F. Cellular energy metabolism in T-lymphocytes. Int Rev Immunol 2015; 34:34-49; PMID:25259409; http://dx.doi.org/10.3109/08830185.2014.956358
- Dang EV, Barbi J, Yang HY, Jinasena D, Yu H, Zheng Y, Bordman Z, Fu J, Kim Y, Yen HR et al. Control of T(H)17/T(reg) balance by hypoxia-inducible factor 1. Cell 2011; 146:772-84; PMID:21871655; http://dx.doi.org/10.1016/j.cell.2011.07.033
- Buchbinder EI, Desai A. CTLA-4 and PD-1 pathways: similarities, differences, and implications of their inhibition. Am J Clin Oncol 2016; 39:98-106; PMID:26558876; http://dx.doi.org/10.1097/COC.0000000000000239
- Doedens AL, Phan AT, Stradner MH, Fujimoto JK, Nguyen JV, Yang E, Johnson RS, Goldrath AW. Hypoxia-inducible factors enhance the effector responses of CD8(+) T cells to persistent antigen. Nat Immunol 2013; 14:1173-82; PMID:24076634; http://dx.doi.org/10.1038/ni.2714
- Buck MD, O'Sullivan D, Pearce EL. T cell metabolism drives immunity. J Exp Med 2015; 212:1345-60; PMID:26261266; http://dx.doi.org/10.1084/jem.20151159
- Principe DR, Doll JA, Bauer J, Jung B, Munshi HG, Bartholin L, Pasche B, Lee C, Grippo PJ. TGF-β: duality of function between tumor prevention and carcinogenesis. J Natl Cancer Inst 2014; 106:djt369; PMID:24511106; http://dx.doi.org/10.1093/jnci/djt369
- Scholzen T, Gerdes J. The Ki-67 protein: from the known and the unknown. J Cell Physiol 2000; 182:311-22; PMID:10653597; http://dx.doi.org/10.1002/(SICI)1097-4652(200003)182:3%3c311::AID-JCP1%3e3.0.CO;2-9
- Massague J. TGFbeta in cancer. Cell 2008; 134:215-30; PMID:18662538; http://dx.doi.org/10.1016/j.cell.2008.07.001
- Wolf D, Sopper S, Pircher A, Gastl G, Wolf AM. Treg(s) in cancer: friends or foe? J Cell Physiol 2015; 230:2598-605; PMID:25913194; http://dx.doi.org/10.1002/jcp.25016
- Andreev K, Trufa ID, Siegemund R, Rieker R, Hartmann A, Schmidt J, Sirbu H, Finotto S. Impaired T-bet-pSTAT1alpha and perforin-mediated immune responses in the tumoral region of lung adenocarcinoma. Br J Cancer 2015; 113:902-13; PMID:26348446; http://dx.doi.org/10.1038/bjc.2015.255
- Garon EB. Current perspectives in immunotherapy for non-small cell lung cancer. Semin Oncol 2015; 42(Suppl 2):S11-8; PMID:26477470; http://dx.doi.org/10.1053/j.seminoncol.2015.09.019
- Cramer T, Yamanishi Y, Clausen BE, Förster I, Pawlinski R, Mackman N, Haase VH, Jaenisch R, Corr M, Nizet V et al. HIF-1α is essential for myeloid cell-mediated inflammation. Cell 2003; 112:645-57; PMID:12628185; http://dx.doi.org/10.1016/S0092-8674(03)00154-5
- Zhang L, Yi H, Xia XP, Zhao Y. Transforming growth factor-beta: an important role in CD4+CD25+ regulatory T cells and immune tolerance. Autoimmunity 2006; 39:269-76; PMID:16891215; http://dx.doi.org/10.1080/08916930600753903
- Henson ES, Gibson SB. Surviving cell death through epidermal growth factor (EGF) signal transduction pathways: implications for cancer therapy. Cell Signalling 2006; 18:2089-97; 16815674; http://dx.doi.org/10.1016/j.cellsig.2006.05.015
- Bhowmick NA, Ghiassi M, Bakin A, Aakre M, Lundquist CA, Engel ME, Arteaga CL, Moses HL. Transforming growth factor-β1 mediates epithelial to mesenchymal transdifferentiation through a RhoA-dependent mechanism. Mol Biol Cell 2001; 12:27-36; PMID:11160820; http://dx.doi.org/10.1091/mbc.12.1.27
- Krakhmal N, Zavyalova M, Denisov E, Vtorushin S, Perelmuter V. Cancer invasion: patterns and mechanisms. Acta Naturae 2015; 7:17-28; PMID:26085941
- Clark AG, Vignjevic DM. Modes of cancer cell invasion and the role of the microenvironment. Curr Opin Cell Biol 2015; 36:13-22; PMID:26183445; http://dx.doi.org/10.1016/j.ceb.2015.06.004
- Plotnik JP, Budka JA, Ferris MW, Hollenhorst PC. ETS1 is a genome-wide effector of RAS/ERK signaling in epithelial cells. Nucleic Acids Res 2014; 42:11928-40; PMID:25294825; http://dx.doi.org/10.1093/nar/gku929
- Hess Michelini R, Doedens AL, Goldrath AW, Hedrick SM. Differentiation of CD8 memory T cells depends on Foxo1. J Exp Med 2013; 210:1189-200; PMID:23712431; http://dx.doi.org/10.1084/jem.20130392
- Dobin A, Davis CA, Schlesinger F, Drenkow J, Zaleski C, Jha S, Batut P, Chaisson M, Gingeras TR. STAR: ultrafast universal RNA-seq aligner. Bioinformatics 2013; 29:15-21; PMID:23104886; http://dx.doi.org/10.1093/bioinformatics/bts635
- Liao Y, Smyth GK, Shi W. featureCounts: an efficient general purpose program for assigning sequence reads to genomic features. Bioinformatics 2014; 30:923-30; PMID:24227677; http://dx.doi.org/10.1093/bioinformatics/btt656
- Team RDC. R: A language and environment for statistical computing. R Foundation for Statistical Computing, Vienna, Austria 2011.
- Love MI, Huber W, Anders S. Moderated estimation of fold change and dispersion for RNA-seq data with DESeq2. Genome Biol 2014; 15:550; PMID:25516281; http://dx.doi.org/10.1186/s13059-014-0550-8
- Warnes GR, Bolker B, Bonebakker L, Gentleman R, Huber W, Liaw A et al. gplots: various R programming tools for plotting data. R package version 2.17.0 2015.