ABSTRACT
The lungs are ubiquitous receptacles of metastases originating from various bodily tumors. Although osteopontin (SPP1) has been associated with tumor dissemination, the role of its isoforms in lung-directed metastasis is incompletely understood. We employed syngeneic mouse models of spontaneous and induced lung-targeted metastasis in C57BL/6 mice competent and deficient in both Spp1 alleles. Tumor-derived osteopontin expression was modulated using either stable anti-Spp1 RNA interference, or forced overexpression of intracellular and secreted Spp1 isoforms. Identified osteopontin's downstream partners were validated using lung adenocarcinoma cells conditionally lacking the Trp53 gene and Ccr2-deficient mice. We determined that host-derived osteopontin was dispensable for pulmonary colonization by different tumor types. Oppositely, tumor-originated intracellular osteopontin promoted tumor cell survival by preventing tumor-related protein 53-mediated apoptosis, while the secretory osteopontin functioned in a paracrine mode to accelerate lung metastasis by enhancing tumor-derived C–C-motif chemokine ligand 2 signaling to cognate host receptors. As new ways to target osteopontin signaling are becoming available, the cytokine may constitute an important therapeutic target against pulmonary involvement by cancers of other organs.
Introduction
Pulmonary metastasis is frequent in patients suffering from various tumors, especially adenocarcinomas of the lungs, breast, and colon, and is a major cause of cancer-related death.Citation1-3 Systemic tumor dissemination involves complex interactions between tumor and host cells during the intravasation of tumor cells into blood and/or lymphatic vessels at the primary tumor site, their spread to target organs, and their survival and extravasation at distant sites.Citation4 These processes are dictated by genetic and epigenetic alterations of tumor cells that cross-talk to the host microenvironment to facilitate intercellular communication, immune evasion, and sustained proliferation, and such traits of metastasis present lucrative targets for therapy.Citation5
Osteopontin (also known as secreted phosphoprotein 1, SPP1), an adhesive glycophosphoprotein widely expressed in tissues, body fluids, and cells under normal conditions, regulates physiologic processes such as development, differentiation, inflammation, and wound healing.Citation6-9 In addition, SPP1 is highly expressed in the microenvironment of various tumors, both by malignant and host cells.Citation10-12 Osteopontin has been linked with enhanced tumor progression and metastasis by modulating cell–cell interactions, by activating signal transduction pathways, by altering gene expression, and by promoting tumor cell survival.Citation13-20 Interestingly, the diverse effects of SPP1 vary between cell types and target organs of metastasis, depending on which signaling pathways are activated and which isoforms of the protein are expressed.Citation16,21,22 Despite significant efforts, a comprehensive understanding of the functions of cancer- and host-originated SPP1 isoforms during pulmonary metastasis is elusive.
In this study, we asked whether host- or tumor-derived, intracellular (iSPP1) or secreted (sSPP1) SPP1 isoforms promote pulmonary dissemination of different tumor types and searched for the specific mechanisms of their involvement in this phenomenon. To address these questions, we combined several novel approaches: (i) syngeneic mouse models of both spontaneous and induced pulmonary metastasis on a background of full immunocompetence, (ii) tumor cell lines of different tissues of origin featuring different SPP1expression patterns, (iii) SPP1 silencing, (iv) engineering and overexpression of eukaryotic vectors encoding different SPP1 isoforms, (v) conditionally Trp53-deleted primary lung adenocarcinoma cells, and (vi) Ccr2 gene-deficient mice. We identified distinct roles for intracellular and secreted SPP1 isoforms expressed by tumor cells during pulmonary metastatic colonization. The former prolonged tumor cell survival in a cell-autonomous fashion and the latter enhanced metastasis in vivo via paracrine signaling to host cells.
Results
Characterization of mouse osteopontin transcript protein products
Since there is no definite way in literature to distinguish between the protein products of the two main murine Spp1 transcripts, Spp1is2 and Spp1is4, due to their similar molecular weight,Citation23 we initially aimed to characterize and correlate the main murine Spp1 transcripts with their corresponding proteins. For this, we transfected HEK293T cells with bicistronic vectors encoding murine Spp1 isoforms in-frame with GFP or GFP alone. The generation of these vectors is described in detail in the Supplementary Information. Transfection of HEK293T cells with Spp1is2.GFP resulted in expression of a single protein of an apparent mass of 68 KDa. Transfection of HEK293T cells with Spp1is4.GFP resulted in both the above-mentioned 68 KDa band and an additional band that consistently appeared at 50 KDa (). These results positioned the molecular mass of the Spp1is2 and Spp1is4 transcripts' protein products at approximately 41 KDa, and at 41 and 23 KDa, respectively, since GFP separates at 27 KDa (). The selective appearance of the 23 KDa protein upon overexpression of Spp1is4 was deemed to result from cleavage specific only for this transcript's protein product; the resulting 23 KDa fragment, a product of post-translational modifications, has been reported to be the secreted protein product.Citation24 From these studies and the literature we concluded that translation of Spp1is2 results in a 41 KDa cytoplasmic protein, hereafter called intracellular SPP1 (iSPP1), whereas translation of Spp1is4 in both an unprocessed iSPP1 isoform and a processed, secretory SPP1 isoform (sSPP1).
Figure 1. Characterization of osteopontin transcript protein products and impact of host-expressed SPP1 on lung metastasis. (A) Immunoblots probed with anti-SPP1 and anti-GFP antibodies of whole cell protein extracts of HEK293T cells (293T) transfected with no vector or with bicistronic vectors encoding Spp1is2 or Spp1is4 in-frame with GFP or GFP alone. Note the correspondence of the Spp1is2 transcript to the iSPP1.GFP fusion protein that runs at ∼68 KDa and of the Spp1is4 transcript to the sSPP1.GFP fusion protein that runs at ∼50 KDa, the GFP protein at ∼27 KDa, as well as the ∼40 KDa band appearing only after Spp1is4 transfection, which likely represents a proteolytic fragment of osteopontin. (B) Representative images of immunostaining of the main cell types and anatomic compartments of the naïve C57BL/6 mouse lung (n = 5) for endogenous SPP1 (brown), counterstained with hematoxylin (blue). (C, D) Primary tumor growth rate and number and total volume of lung metastases of Spp1-competent (Spp1+/+) and Spp1-deficient (Spp1−/−) mice after (C) s.c. injection (n = 5–7/group) or (D) i.v injection (n = 6–15/group) of B16F10, LLC, or MC38 cells. Note that only mice that received s.c LLC cells developed spontaneous lung metastases. Data are expressed as mean ± SD ns and *p > 0.05 and p < 0.05, respectively, for comparison between Spp1+/+ and Spp1−/− mice by two-way ANOVA with Bonferroni post-tests (dot plots) or unpaired Student's t-test (bar graphs).
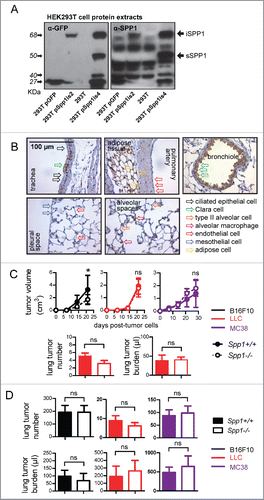
Host osteopontin expression and its impact on lung metastasis
We next characterized osteopontin expression in naïve mouse lungs and blood, the anatomic compartments where lung metastasis is initiated. Osteopontin immunoreactivity was evident in non-ciliated airway epithelial (Clara or club) cells, as well as alveolar macrophages, but not in ciliated airway and alveolar epithelial, mesothelial, and pulmonary endothelial cells ( and S1A, S1B). Osteopontin protein was also present in the bloodstream of Spp1 gene-competent (Spp1+/+) and of single-allele Spp1 gene-deficient (Spp1+/−), but not of homozygote Spp1 knockout (Spp1−/−) C57BL/6 mice (Fig. S1C.Citation9 Immunoblotting of whole lung protein extracts from Spp1+/+ and Spp1−/− mice and from HEK293T human embryonic kidney cells, known to produce iSPP1 and sSPP1,Citation25 revealed predominant expression of processed sSPP1 in the lungs (Fig. S1D). Unexpectedly, host-derived osteopontin did not impact spontaneous and forced pulmonary metastasis. To test this, Spp1+/+ and Spp1−/− mice received three different origin cancer cell lines derived from C57BL/6 mice: B16F10 skin melanoma, Lewis lung carcinoma (LLC), and MC38 colon adenocarcinoma. Tumor cell injections were done both subcutaneously (s.c.), and intravenously (i.v.) in separate cohorts of mice. As shown in , Spp1+/+ and Spp1−/− mice with s.c. LLC or MC38 cells displayed similar flank tumor growth rates, while Spp1−/− mice with s.c. B16F10 cells exhibited decreased primary tumor growth compared with Spp1+/+ mice. Importantly, only LLC cells could spontaneously metastasize to the lungs, with Spp1+/+ and Spp1−/− mice displaying equal number and size of lung lesions, as determined by both gross lung tumor counting and morphometry of randomly sampled lung sections. Citation26,27 Similar results were obtained after i.v. delivery of tumor cells. All cancer cell lines colonized the lungs upon tail vein injection, with Spp1+/+ and Spp1−/− mice developing similar number and size of lung lesions ( and S1E). These results suggested that host-originated osteopontin is not cardinal for pulmonary metastasis.
Differential SPP1 isoform expression by tumor cells
We next investigated tumor cells as a source of osteopontin. For this, B16F10, LLC, and MC38 cells were assessed for expression of osteopontin transcripts and protein by reverse transcriptase (RT)-PCR, immunoblotting, immunocytochemistry, and ELISA (). In parallel to parental cells, daughter cells stably overexpressing eukaryotic expression vectors encoding murine Spp1is2 and Spp1is4, as well as random control (shC) or anti-Spp1 (shSpp1) shRNA were generated. We determined that B16F10 cells express minimal amounts of both osteopontin transcripts and their corresponding proteins, that LLC cells express similar amounts of both transcripts, and that MC38 cells predominantly express Spp1is4 and processed sSPP1. In addition, we validated our daughter cell lines with modulated osteopontin isoform expression.
Figure 2. SPP1 isoform expression by C57BL/6 tumor cell lines and their role in cancer cell survival. (A–D) Parental B16F10, LLC, and MC38 cells, B16F10 cells stably expressing Spp1is2 and Spp1is4 constructs (p), and LLC and MC38 cells stably expressing anti-Spp1 (shSpp1) or random control (shC) shRNA were assessed for Spp1 mRNA by RT-PCR of total cellular RNA (A),SPP1 protein by immunoblotting of whole cells extracts (B), ELISA of cellular supernatants (C) and SPP1 cellular immunostaining (D). Inlays: isotype controls. Note amplicons of Spp1is2 and Spp1is4 at 933 and 885 bp, respectively, and the corresponding electrophoretic bands of iSPP1 and sSPP1 at 41 and 23 KDa (A and B). Note also that sSPP1 displays an apparent molecular mass that is smaller than anticipated by Spp1is4 sequence due to post-translational modification (cleavage) (B). Finally, note that only sSPP1 was detected in media conditioned for 24 h by 105 live cells (n = 5/group) (C). (E–G) Parental and SPP1-modulated tumor cells described above were assessed for cellular proliferation by MTT reduction (E) and for apoptosis by flow cytometric determination of annexin V and 7AAD staining (F) and caspase 3 and 8 immunoreactivity by immunocytochemistry (G). Data are expressed as mean ± SD of one representative of three experiments performed both in the presence or absence of 10% FBS. ns, **, and ***p > 0.05, p < 0.01, and p < 0.001, respectively, for the indicated comparisons by two-way (dot plots) or one-way (bar graphs) ANOVA with Bonferroni post-tests.
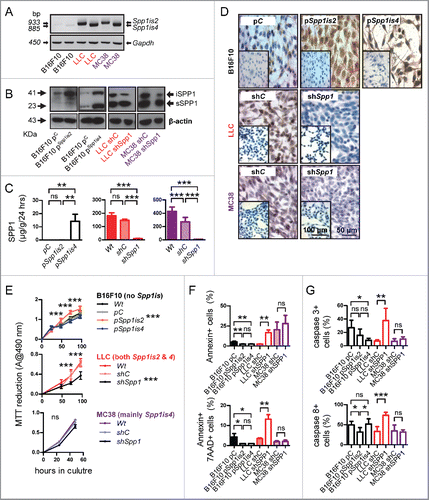
iSPP1 as a cell-autonomous tumor cell survival signal that requires wild-type TRP53
We next determined the proliferation and apoptosis rates of our parental and Spp1-modulated cell lines in vitro using MTT assay, labeling with annexin V and 7-aminoactinomycin D (7AAD), as well as immunostaining for caspases 3 and 8 ( and S2). We observed that overexpression of Spp1is2 in B16F10 cells that are osteopontin-deficient resulted in enhanced cell proliferation and diminished apoptotic indices, whereas overexpression of Spp1is4 in these cells did not impact cell proliferation. Moreover, silencing of Spp1 in LLC cells (competent in both osteopontin isoforms) significantly inhibited cell proliferation, augmented apoptosis, and induced caspases 3 and 8, while the same intervention in MC38 cells (predominantly competent in sSPP1) had no effect on these parameters. These results indicated that iSPP1 (encoded by Spp1is2) promotes tumor cell survival in a cell-autonomous fashion. We next subjected parental and Spp1-silenced LLC and MC38 cells to analysis of global gene expression. Interestingly, Spp1 silencing affected chemokine and cytokine signaling pathways in both cell types, but selectively perturbed the tumor-related protein 53 (TRP53) signaling pathway in LLC cells (Fig. S3A). In specific, Spp1 silencing in LLC cells induced key TRP53 pathway components that lead to activation of effector caspases 3 and 8, while the same intervention in MC38 cells suppressed several TRP53 pathway components (Fig. S3B). TRP53 immunostaining revealed that LLC cells showed minimal TRP53 immunoreactivity that was enhanced upon Spp1 silencing, whereas MC38 cells displayed high TRP53 expression that was not affected by Spp1 shRNA (Fig. S4A). This pattern indicated a Trp53 mutation in MC38 cells that results in increased stability of non-functional TRP53 protein. Indeed, Sanger sequencing and immunofluorescence confirmed a heterozygous Trp53R178P point mutation and ubiquitous nuclear non-labile TRP53 protein in these cells (Figs. S4B and C). These findings suggested that cell-autonomous pro-survival effects of osteopontin in tumor cells may be mediated by wild-type TRP53. To definitely test this in an isogenic cellular system, we derived a lung adenocarcinoma cell line from conditionally Trp53-deleted mice [Trp53f/f;Citation28]. For this, Trp53f/f mice (C57BL/6 strain) received 10 weekly intraperitoneal injections of the lung carcinogen urethane (1 g/Kg), as described elsewhere,Citation29,30 and were sacrificed after 10 months, followed by long-term lung tumor culture in vitro. The resulting cell line (C57BL/6 Urethane-induced Lung Adenocarcinoma, CULA) was tumorigenic and spontaneously metastatic to the lungs when implanted s.c. in C57BL/6 mice, and was efficiently recombined in vitro using a Cre recombinase plasmid,Citation31 yielding isogenic tumor cells with or without functional Trp53 alleles (). Using this system combined with Spp1 shRNA, we determined that osteopontin silencing decreased proliferation and increased apoptosis of parental CULA cells with functional Trp53 alleles, while this effect was lost upon Trp53 deletion (), confirming a role for wild-type TRP53 in the cell-autonomous pro-survival effects of iSPP1.
Figure 3. A murine lung adenocarcinoma cell line conditional for Trp53 alleles reveals that Trp53 is required for the pro-survival effects of intracellular SPP1. (A) Strategy for derivation of C57BL/6 Urethane-induced Lung Adenocarcinoma cells (CULA) from mice carrying loxP sites on either side of both Trp53 alleles (Trp53f/f). Mice (n = 5) were initiated on 10 weekly intraperitoneal urethane injections (1 gr/kg) 6 weeks after birth and were sacrificed 10 months after the first injection. Lungs were harvested under sterile conditions, tumors (n = 10) were enucleated, minced, and cultured separately. One clone was established after 4 weeks and was passaged more than 100 times over 2 y. (B) Representative stereoscopic image of lungs from Trp53f/f C57BL/6 mouse treated as described under (A) featuring lung tumors (arrows). (C, D) Representative images of immunostaining of lung tissue from Trp53f/f C57BL/6 mouse treated as described under (A) for PCNA, shows minimal nuclear immunoreactivity in airway epithelium (arrows in C) in contrast to the significant proportion of immunoreactive cells (arrows) in urethane-induced tumors (dashed outline in D). (E) Phase contrast image of Trp53f/f CULA cells in culture at passage 40. Note the spindle-shaped tumor cells. (F–I) C57BL/6 mice (n = 9) received 0.5×106 Trp53f/f CULA cells s.c. and were sacrificed after 8 weeks. Representative images of flank tumor [dashed outline; (F)], spontaneous lung metastases [arrows; (G)], lung section with lung metastases [(H); arrows], and of lung section with lung metastasis showing several mitoses per high-power field [arrows; (I)]. (J) RT-PCR of Trp53f/f CULA cells stably expressing vectors encoding a random sequence (pC) or CRE recombinase (pCre). Successful deletion of floxed Trp53 alleles is confirmed by the 270 bp product. (K) Immunoblots of whole cell protein extracts of Trp53f/f CULA cells stably expressing vectors encoding a random sequence (pC) or CRE recombinase (pCre) and random control (shC) or Spp1-specific shRNA (shSpp1) showing efficient combined modulation of both TRP53 and SPP1 protein expression in this system. (L, M) CULA cells featuring conditional Trp53 alleles (Trp53f/f) were stably transfected as described in (K) were assessed for cellular proliferation by MTT reduction (L) and for apoptosis by flow cytometric determination of annexin V and 7AAD staining (M). Data are expressed as mean ± SD (n = 5/data-point) of one representative of three experiments performed both in the presence or absence of 10% FBS. ns, *, **, and ***p > 0.05, p < 0.05, p < 0.01, and p < 0.001, respectively, for the indicated comparisons by two-way (dot plots) or one-way (bar graphs) ANOVA with Bonferroni post-tests.
![Figure 3. A murine lung adenocarcinoma cell line conditional for Trp53 alleles reveals that Trp53 is required for the pro-survival effects of intracellular SPP1. (A) Strategy for derivation of C57BL/6 Urethane-induced Lung Adenocarcinoma cells (CULA) from mice carrying loxP sites on either side of both Trp53 alleles (Trp53f/f). Mice (n = 5) were initiated on 10 weekly intraperitoneal urethane injections (1 gr/kg) 6 weeks after birth and were sacrificed 10 months after the first injection. Lungs were harvested under sterile conditions, tumors (n = 10) were enucleated, minced, and cultured separately. One clone was established after 4 weeks and was passaged more than 100 times over 2 y. (B) Representative stereoscopic image of lungs from Trp53f/f C57BL/6 mouse treated as described under (A) featuring lung tumors (arrows). (C, D) Representative images of immunostaining of lung tissue from Trp53f/f C57BL/6 mouse treated as described under (A) for PCNA, shows minimal nuclear immunoreactivity in airway epithelium (arrows in C) in contrast to the significant proportion of immunoreactive cells (arrows) in urethane-induced tumors (dashed outline in D). (E) Phase contrast image of Trp53f/f CULA cells in culture at passage 40. Note the spindle-shaped tumor cells. (F–I) C57BL/6 mice (n = 9) received 0.5×106 Trp53f/f CULA cells s.c. and were sacrificed after 8 weeks. Representative images of flank tumor [dashed outline; (F)], spontaneous lung metastases [arrows; (G)], lung section with lung metastases [(H); arrows], and of lung section with lung metastasis showing several mitoses per high-power field [arrows; (I)]. (J) RT-PCR of Trp53f/f CULA cells stably expressing vectors encoding a random sequence (pC) or CRE recombinase (pCre). Successful deletion of floxed Trp53 alleles is confirmed by the 270 bp product. (K) Immunoblots of whole cell protein extracts of Trp53f/f CULA cells stably expressing vectors encoding a random sequence (pC) or CRE recombinase (pCre) and random control (shC) or Spp1-specific shRNA (shSpp1) showing efficient combined modulation of both TRP53 and SPP1 protein expression in this system. (L, M) CULA cells featuring conditional Trp53 alleles (Trp53f/f) were stably transfected as described in (K) were assessed for cellular proliferation by MTT reduction (L) and for apoptosis by flow cytometric determination of annexin V and 7AAD staining (M). Data are expressed as mean ± SD (n = 5/data-point) of one representative of three experiments performed both in the presence or absence of 10% FBS. ns, *, **, and ***p > 0.05, p < 0.05, p < 0.01, and p < 0.001, respectively, for the indicated comparisons by two-way (dot plots) or one-way (bar graphs) ANOVA with Bonferroni post-tests.](/cms/asset/65548a2c-42da-4df0-a46c-e280a1c930fd/koni_a_1256528_f0003_oc.gif)
Tumor-derived sSPP1 as a driver of lung metastasis that signals via CCL2 to host cells
We next injected B16F10, LLC, and MC38 parental and modulated daughter cells s.c. or i.v. to C57BL/6 mice and assessed their potential for lung metastasis. Overexpression of osteopontin isoforms in B16F10 cells and silencing of osteopontin in MC38 cells had no apparent effects on flank tumor growth, but silencing of SPP1 in LLC cells resulted in decreased tumor growth, reduced spontaneous lung metastases, and prolonged survival of mice with s.c. tumors (). Moreover, overexpression of both osteopontin isoforms in naturally osteopontin-deficient B16F10 cells enhanced i.v. lung metastasis, with Spp1is4 (encoding sSPP1) exhibiting greater effects (). In addition, Spp1 silencing abrogated lung metastasis and prolonged the survival of C57BL/6 mice after i.v. delivery of LLC or MC38 cells (). These results indicated marked pro-metastatic effects of tumor-derived sSPP1. We next analyzed microarrays from parental and Spp1-silenced LLC and MC38 cells for individual transcripts and identified Ccl7 and Ccl2 (encoding C–C-motif chemokine ligands 2 and 7, CCL2 and CCL7, respectively) as the top mRNAs downregulated in both cells by shSpp1 ( and S5A; Tables S3 and S4). This was confirmed by qPCR, which showed that (i) tumor cell Ccl2 expression predominated over that of Ccl7; (ii) Spp1is4 overexpression in B16F10 cells augmented Ccl2 and Ccl7 expression; and (iii) shSpp1 significantly inhibited Ccl2, but not Ccl7, expression in LLC and MC38 cells (). These data were confirmed at protein level (Fig. S5B), identified CCL2 as a possible accomplice of sSPP1, and prompted us to evaluate the expression of Ccl2 and its receptor Ccr2 in the hosts and tumor cells of our models. In addition to bone marrow and liver, the lungs expressed significant levels of Ccr2, while Ccl2 was exclusively expressed by LLC and MC38 cells (). To test whether CCL2 signaling by tumor cells to host CCR2 receptors mediates the pro-metastatic effects of sSPP1, we injected MC38 cells (predominantly expressing sSPP1), Spp1-silenced MC38 cells, B16F10 cells (iSPP1 and sSPP1-deficient), and B16F10 cells overexpressing either Spp1is2 or Spp1is4 into the bloodstream of Ccr2-deficient and control mice bred on a pure C57BL/6 strain.Citation32 These experiments showed that host CCR2-competence is important during lung metastasis of sSPP1-competent cells but dispensable for pulmonary colonization by sSPP1-deficient tumor cells, and confirmed that tumor-derived sSPP1 functions via signaling to host CCR2 receptors ( and S6).
Figure 4. Tumor-derived SPP1 in spontaneous lung metastasis. Primary tumor growth rate (A–C), survival (D), and number (E), total volume (F), and representative images (G) of lung metastases of C57BL/6 mice (n = 6–16/group) after s.c. injection of parental and SPP1-modulated tumor cells described in . Note that only mice that received LLC cells developed spontaneous lung metastases. Shown are mean ± SD (all graphs except D) or Kaplan–Meier survival estimates (D). ns and ***p > 0.05 and p < 0.001, respectively, for the indicated comparisons by two-way ANOVA with Bonferroni post-tests (dot plots), unpaired Student's t-test (bar graphs), or log-rank test (D).
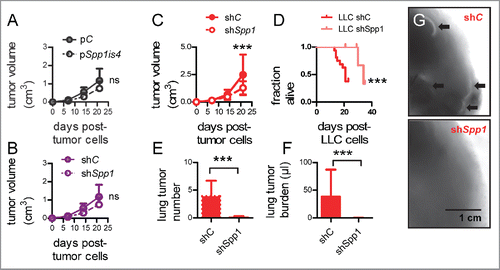
Figure 5. Tumor-derived SPP1 in induced lung metastasis. (A, B) Number and total volume of lung metastases (A) and survival (B) of C57BL/6 mice (n = 5–24/group) after i.v. injection of parental and SPP1-modulated tumor cells described in . (C) Representative microscopic images of lung sections stained with hematoxylin and eosin and stereoscopic images of lungs. Shown are mean ± SD (A) or Kaplan–Meier survival estimates (B). ns, *, **, and ***p > 0.05, p < 0.05, p < 0.01, and p < 0.001, respectively, for the indicated comparisons by one-way ANOVA with Bonferroni post-tests [(A), B16F10 cells], unpaired Student's t-test [(A), LLC and MC38 cells], or log-rank test (B).
![Figure 5. Tumor-derived SPP1 in induced lung metastasis. (A, B) Number and total volume of lung metastases (A) and survival (B) of C57BL/6 mice (n = 5–24/group) after i.v. injection of parental and SPP1-modulated tumor cells described in Fig. 2. (C) Representative microscopic images of lung sections stained with hematoxylin and eosin and stereoscopic images of lungs. Shown are mean ± SD (A) or Kaplan–Meier survival estimates (B). ns, *, **, and ***p > 0.05, p < 0.05, p < 0.01, and p < 0.001, respectively, for the indicated comparisons by one-way ANOVA with Bonferroni post-tests [(A), B16F10 cells], unpaired Student's t-test [(A), LLC and MC38 cells], or log-rank test (B).](/cms/asset/caa24533-dc65-4aa9-9040-bae994030ede/koni_a_1256528_f0005_oc.gif)
Figure 6. A requirement for CCL2 in the pro-metastatic effects of sSPP1. (A) Schematic of differential global gene expression by microarray of LLC and MC38 cells stably expressing anti-Spp1-specific (shSpp1) or random control (shC) shRNA. Listed are the top-10 common SPP1-dependent transcripts by order of magnitude. (B) qPCR of total cellular RNA of parental and SPP1-modulated tumor cells described in for Ccl2 and Ccl7 relative to Gusb mRNA (n = 3/data-point). Shown is one representative of three experiments. (C) qPCR of total cellular and tissue RNA of parental tumor cells used in this study and of common target organs of metastasis for Ccl2 and Ccr2 relative to Gusb mRNA (n = 5/group). (D, E) Number of lung metastases of C57BL/6 mice competent (Ccr2+/+) or deficient (Ccr2−/−) in both Ccr2 alleles after i.v. injection of parental and SPP1-modulated MC38 (D) and B16F10 (E) cells described in (n = 4–9/group). Data are expressed as mean ± SD ns, *, **, and ***p > 0.05, p < 0.05, p < 0.01, and p < 0.001, respectively, for the indicated comparisons (or comparison to brain in C) by one-way ANOVA with Bonferroni post-tests (B-D) or unpaired Student's t-test (E).
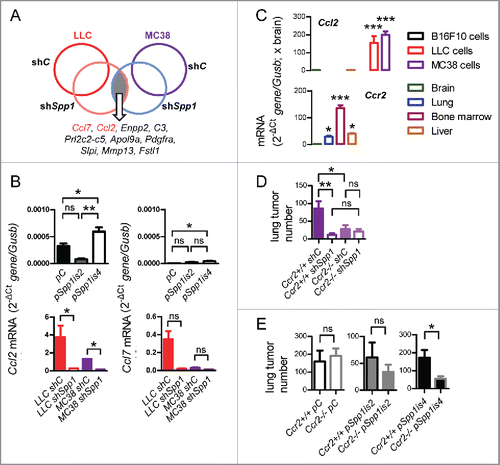
Discussion
We studied the role of osteopontin isoforms in lung metastasis by mapping their expression by tumor and host cells and by using hosts and tumor cells that feature loss or gain of their expression. Syngeneic, immunocompetent mouse models of pulmonary metastasis of both s.c. and i.v. cancer cells were employed to capture intact tumor–host interactions. We describe the distinct functions of tumor-derived osteopontin isoforms, including the in vitro pro-survival effects of iSPP1 and the in vivo pro-metastatic effects of sSPP1, and pin wild-type TRP53 and CCL2 as their respective signaling partners. Our results can be translated to strategies of osteopontin targeting against metastasis and are important on several counts.
First, tumor-derived osteopontin is corroborated as a culprit of lung metastasis of various tumor types, in line with published work.Citation15,33 Both in our and other studies, osteopontin effects were delivered during late-stage metastasis, since similar results were obtained from s.c. and i.v. models.Citation15,33 Hence, the cytokine is likely dispensable for tumor dissemination from the primary site, but is required for circulating tumor cell engraftment in the lungs. Since circulating tumor cells are ubiquitous in cancer patients with minimal disease and most cancer patients will already have metastasis at the time of diagnosis,Citation4,5 mediators of end-organ colonization such as osteopontin are marked targets for therapy. Thus, our findings bear potential clinical implications, as several approaches to inhibit osteopontin signaling are in development.Citation34,35 Our negative results from osteopontin-deficient mice are different from findings from breast cancer and malignant pleural effusion (MPE) models where host osteopontin was important.Citation15,16 However, the different results can be reconciled and convey mechanistic implications: The different models employed in these studies feature divergent anatomic and cellular compartmentalization of osteopontin expression and different osteopontin expression patterns by tumor cells.Citation36 Since osteopontin impacts late-stage metastasis, the local levels of tumor- and host-delivered osteopontin in the incipient metastatic niche are likely critical. Interestingly, Balb/c mice features high serum osteopontin levels compared with C57BL/6 mice,Citation37 and 4T1 cells secrete less osteopontin compared with LLC and MC38 cells (1 vs. 20–40 μg/mL/106 cells/24 h, respectively), likely rendering host osteopontin important in 4T1 cell metastasis,Citation15 but not in our hands. Similarly, we measured elevated levels of host osteopontin in MPE Citation16 as compared with the bloodstream in our study (300 vs. 70 ng/mL, respectively), making host osteopontin important in MPE,Citation16 but not in the work presented here. In our hands, the pulmonary endothelium did not express osteopontin and the bulk of local osteopontin was delivered by metastatic tumor cells, rendering tumor-expressed osteopontin pivotal for lung colonization. These observations likely explain the role of host osteopontin in the support of tumor growth when B16F10 cells were delivered s.c., since these cells do not express the cytokine and likely depend on host-derived osteopontin.
Second, we define the distinct functions of osteopontin isoforms during pulmonary metastasis. As previously reported on the distinct adhesive properties of murine osteopontin that result from cell specific post-translational modifications,Citation38 we show that iSPP1 and sSPP1 are differentially expressed by tumor cells, with the former preventing TRP53 stabilization and apoptosis, and the latter signaling to host CCR2 receptors via CCL2 to promote lung homing. Our results corroborate previous work on the inhibition of apoptosis Citation39 and the involvement of TRP53 in iSPP1-dependent anti-apoptotic signaling Citation40 and show for the first time that the pro-survival effects of osteopontin in cancer cells are conditional on wild-type TRP53. Our findings imply that targeting of human iSPP1 in clinical trials may be selectively effective against TRP53 wild-type tumors and provide a rationale for stratification of patients by TRP53 status. This notion is of special importance for neoplasms where osteopontin biology is cardinal and TRP53 mutations frequent, such as malignant pleural mesothelioma.Citation16,41-43
Third, sSPP1 is identified as the key osteopontin isoform that promotes lung metastasis across tumor types. To this end, overexpression of the corresponding transcript Spp1is4 exerted a far stronger pro-metastatic impact on naturally osteopontin-deficient B16F10 cells, compared with the transcript Spp1is2 that corresponds to iSPP1. These data explain the results of previous studies implicating osteopontin in tumor cells, as well as its receptor CD44 on host cells, in metastasis to other sites.Citation15,44,45 Furthermore, our results bear clinical implications for selective osteopontin isoform targeting, since selective sSPP1 inhibition can be accomplished using protein and RNA-based methods.Citation35
Furthermore, sSPP1 is identified to exert its pro-metastatic function by regulating tumor-to-host CCL2/CCR2 signaling. Our data reconcile the results of previous studies on breast cancer,Citation15,46 and help explain the mononuclear chemoattractant function of tumor-originated osteopontin observed by others. For the first time, murine osteopontin functions during in vivo metastasis are linked with CCL2 signaling, a known druggable trait of thoracic tumor dissemination.Citation47-50 CCL2 can, in turn, enhance osteopontin signaling, since it augments the expression of the osteopontin receptor avb3 integrin.Citation51,52 Both molecules activate pathways such as FAK/Akt, MAPK, JAK2-Stat5, p38MAPK, and NF-κB in endothelial cells, thereby promoting adhesion, extravasation, and immune evasion of circulating tumor cells.Citation18-21 Importantly, CCL2 provides a direct explanation for the pro-metastatic effects of sSPP1, since CCR2 signaling in pulmonary endothelial cells directly promotes circulating tumor cell extravasation in the lungs.Citation52 In addition, the findings bear implications for future targeting of sSPP1 against metastasis in terms of combined sSPP1/CCL2 inhibition, pre-treatment screens for sSPP1/CCL2 expression, and CCL2 blockade against sSPP1-expressing tumors.Citation48,50,53
In conclusion, we comprehensively mapped the expression patterns, the distinct functions, and the key downstream signaling partners of intracellular and secretory osteopontin isoforms during pulmonary metastasis. We provide proof-of-concept evidence that can be useful for the design of future preclinical and clinical studies aimed at targeting this metastatic trait.
Materials and methods
Detailed Supplementary Materials and Methods can be found with this article online.
Mice
C57BL/6 (#000664), B6.129S6-Spp1tm1Blh/J (#004936), B6.129P2-Trp53tm1Brn/J (#008462), and B6.129S4-Ccr2tm1Ifc/J (#004999) mice obtained from Jackson Laboratories (Bar Harbor, ME) were bred in the University of Patras Center for Animal Models of Disease. Experiments were designed and approved a priori by the local Veterinary Administration of the Prefecture of Western Greece (approvals # 3741/16.11.2010, 60291/3035/19.03.2012, and 118018/578/30.04.2014), and were conducted according to Directive 2010/63/EU. Male and female experimental mice and littermate controls were sex-, weight (20–25 g)-, and age (6–12 week)-matched (for n see Table S4).
Cells and constructs
LLC and B16F10 cells were from the National Cancer Institute Tumor Repository (Frederick, MD); MC38 cells were a gift from Dr Timothy S. Blackwell [Vanderbilt University, Nashville, TN;Citation53; and HEK293Τ cells were from the American Type Culture Collection (Manassas, VA). Cell lines were cultured in DMEM and tested biannually for identity and stability by short tandem repeats, Sanger sequencing for driver mutations, and microarray. Anti-Spp1 shRNA (16) and SPP1 isoform constructs are described in the online SI.
Metastasis models
For development of flank tumors and spontaneous lung metastases, 106 LLC, 106 MC38, or 0.25×106 B16F10 cells were injected s.c. For induction of forced lung metastases, 0.25 × 106 LLC, 0.25 × 106 MC38, or 0.15 × 106 B16F10 cells were injected into the tail vein. These numbers were tittered in preliminary experiments to yield 100% tumor take. Mice were sacrificed 30 d after s.c. or 14 d after i.v. tumor cells for metastasis analyses, or when moribund for survival analyses. Lungs were fixed in 10% neutral buffered formalin overnight and the number of lung metastasis was evaluated by two blinded investigators (I. G. and G. T. S.) and averaged. Lung volume was measured by saline immersion, and lungs were embedded in paraffin, randomly sampled by cutting 4 μm-thick lung sections (n = 10/lung), mounted on glass slides, and stained with H&E. Lung tumor burden was determined by point counting of the ratio of the area occupied by metastases versus the lung area and by extrapolating the average ratio per mouse to total lung volume, as described elsewhere.Citation27
Primary mouse lung adenocarcinoma cells
Trp53f/f mice (C57BL/6 strain) received 10 consecutive weekly intraperitoneal injections of the pulmonary carcinogen urethane (1 g/kg) starting at 6 weeks of age and were sacrificed 10 months later. Select lung tumors (n = 10) were isolated under sterile conditions for culture and the remaining lungs were fixed for histology. Lung tumor cells were cultured for more than 100 passages over 2 y to successfully yield one tumor cell line (CULA).
Statistics
Data are expressed as mean ± SD. Differences in means between two or multiple groups were examined by two-tailed Student's t-test or one-way ANOVA with Bonferoni post-tests, as appropriate, since data were distributed normally. Two-way ANOVA with Bonferoni post-tests was employed for analyses of cell and tumor growth. Survival proportions were examined by Kaplan–Meier analysis and log-rank test. All p values are two-tailed and were considered significant when < 0.05. All statistics and plots were done using Prism v5.0 (GraphPad, La Jolla, CA).
Disclosure of potential conflicts of interest
No potential conflicts of interest were disclosed.
Author contributions
I. G. performed most of the experiments; I. L., V. P., T. A., N.S., and M.S performed several in vitro assays and provided critical intellectual input; N. I. K. analyzed the microarray data and performed Quantitative Real-time PCR; I. G. and G. T. S. designed the experiments, analyzed the data, and wrote the paper; G. T. S. conceived the idea and supervised the study, and is the guarantor of the study's integrity. All authors reviewed and concur with the submitted manuscript.
Accession numbers
All plasmids were deposited with Addgene and their ID's are given in the text. Microarray data were deposited at GEO database (http://www.ncbi.nlm.nih.gov/geo/; Accession ID: GSE79831.
KONI_A_1256528_supplementary_data.pdf
Download PDF (1.2 MB)Funding
This work was supported by European Research Council 2010 Starting Independent Investigator and 2015 Proof of Concept Grants (260524 and 679345, respectively, to G.T.S.). The authors thank the University of Patras Center for Animal Models of Disease. The authors report no conflict of interest.
References
- Hess KR, Varadhachary GR, Taylor SH, Wei W, Raber MN, Lenzi R, Abbruzzese JL. Metastatic patterns in adenocarcinoma. Cancer 2006; 106:1624-33; PMID:16518827; http://dx.doi.org/10.1002/cncr.21778
- Disibio G, French SW. Metastatic patterns of cancers: results from a large autopsy study. Arch Pathol Lab Med 2008; 132:931-9; PMID:18517275; http://dx.doi.org/10.1043/1543-2165(2008)132[931:MPOCRF]2.0.CO;2
- Sethi N, Kang Y. Unravelling the complexity of metastasis - molecular understanding and targeted therapies. Nat Rev Cancer 2011; 11:735-48; PMID:21941285; http://dx.doi.org/10.1038/nrc3125
- Nguyen DX, Bos PD, Massague J. Metastasis: from dissemination to organ-specific colonization. Nat Rev Cancer 2009; 9:274-84; PMID:19308067; http://dx.doi.org/10.1038/nrc2622
- Vanharanta S, Massague J. Origins of metastatic traits. Cancer Cell 2013; 24:410-21; PMID:24135279; http://dx.doi.org/10.1016/j.ccr.2013.09.007
- Ashkar S, Weber GF, Panoutsakopoulou V, Sanchirico ME, Jansson M, Zawaideh S, Rittling SR, Denhardt DT, Glimcher MJ, Cantor H. Eta-1 (osteopontin): an early component of type-1 (cell-mediated) immunity. Science 2000; 287:860-4; PMID:10657301; http://dx.doi.org/10.1126/science.287.5454.860
- Zheng W, Li R, Pan H, He D, Xu R, Guo TB, Guo Y, Zhang JZ. Role of osteopontin in induction of monocyte chemoattractant protein 1 and macrophage inflammatory protein 1beta through the NF-kappaB and MAPK pathways in rheumatoid arthritis. Arthritis Rheum 2009; 60:1957-65; PMID:19565503; http://dx.doi.org/10.1002/art.24625
- Li G, Oparil S, Kelpke SS, Chen YF, Thompson JA. Fibroblast growth factor receptor-1 signaling induces osteopontin expression and vascular smooth muscle cell-dependent adventitial fibroblast migration in vitro. Circulation 2002; 106:854-9; PMID:12176960; http://dx.doi.org/10.1161/01.CIR.0000024113.26985.CC
- Liaw L, Birk DE, Ballas CB, Whitsitt JS, Davidson JM, Hogan BL. Altered wound healing in mice lacking a functional osteopontin gene (spp1). J Clin Invest 1998; 101:1468-78; PMID:9525990; http://dx.doi.org/10.1172/JCI2131
- Bramwell VH, Tuck AB, Chapman JA, Anborgh PH, Postenka CO, Al-Katib W, Shepherd LE, Han L, Wilson CF, Pritchard KI et al. Assessment of osteopontin in early breast cancer: correlative study in a randomised clinical trial. Breast Cancer Res 2014; 16:R8; PMID:24451146; http://dx.doi.org/10.1186/bcr3600
- Fedarko NS, Jain A, Karadag A, Van Eman MR, Fisher LW. Elevated serum bone sialoprotein and osteopontin in colon, breast, prostate, and lung cancer. Clin Cancer Res 2001; 7:4060-6; PMID:11751502
- Agrawal D, Chen T, Irby R, Quackenbush J, Chambers AF, Szabo M, Cantor A, Coppola D, Yeatman TJ. Osteopontin identified as lead marker of colon cancer progression, using pooled sample expression profiling. J Natl Cancer Inst 2002; 94:513-21; PMID:11929952; http://dx.doi.org/10.1093/jnci/94.7.513
- Khodavirdi AC, Song Z, Yang S, Zhong C, Wang S, Wu H, Pritchard C, Nelson PS, Roy-Burman P. Increased expression of osteopontin contributes to the progression of prostate cancer. Cancer Res 2006; 66:883-8; PMID:16424021; http://dx.doi.org/10.1158/0008-5472.CAN-05-2816
- Yin M, Soikkeli J, Jahkola T, Virolainen S, Saksela O, Holtta E. Osteopontin promotes the invasive growth of melanoma cells by activating integrin alphavbeta3 and down-regulating tetraspanin CD9. Am J Pathol 2014; 184:842-58; PMID:24412090; http://dx.doi.org/10.1016/j.ajpath.2013.11.020
- Sangaletti S, Tripodo C, Sandri S, Torselli I, Vitali C, Ratti C, Botti L, Burocchi A, Porcasi R, Tomirotti A et al. Osteopontin shapes immunosuppression in the metastatic niche. Cancer Res 2014; 74:4706-19; PMID:25035397; http://dx.doi.org/10.1158/0008-5472.CAN-13-3334
- Psallidas I, Stathopoulos GT, Maniatis NA, Magkouta S, Moschos C, Karabela SP, Kollintza A, Simoes DC, Kardara M, Vassiliou S et al. Secreted phosphoprotein-1 directly provokes vascular leakage to foster malignant pleural effusion. Oncogene 2013; 32:528-35; PMID:22370646; http://dx.doi.org/10.1038/onc.2012.57
- Bellahcene A, Castronovo V, Ogbureke KU, Fisher LW, Fedarko NS. Small integrin-binding ligand N-linked glycoproteins (SIBLINGs): multifunctional proteins in cancer. Nat Rev Cancer 2008; 8:212-26; PMID:18292776; http://dx.doi.org/10.1038/nrc2345
- Lin YH, Yang-Yen HF. The osteopontin-CD44 survival signal involves activation of the phosphatidylinositol 3-kinase/Akt signaling pathway. J Biol Chem 2001; 276:46024-30; PMID:11590166; http://dx.doi.org/10.1074/jbc.M105132200
- Philip S, Bulbule A, Kundu GC. Osteopontin stimulates tumor growth and activation of promatrix metalloproteinase-2 through nuclear factor-kappa B-mediated induction of membrane type 1 matrix metalloproteinase in murine melanoma cells. J Biol Chem 2001; 276:44926-35; PMID:11564733; http://dx.doi.org/10.1074/jbc.M103334200
- Das R, Mahabeleshwar GH, Kundu GC. Osteopontin induces AP-1-mediated secretion of urokinase-type plasminogen activator through c-Src-dependent epidermal growth factor receptor transactivation in breast cancer cells. J Biol Chem 2004; 279:11051-64; PMID:14704150; http://dx.doi.org/10.1074/jbc.M310256200
- Crawford HC, Matrisian LM, Liaw L. Distinct roles of osteopontin in host defense activity and tumor survival during squamous cell carcinoma progression in vivo. Cancer Res 1998; 58:5206-15; PMID:9823334
- Bourassa B, Monaghan S, Rittling SR. Impaired anti-tumor cytotoxicity of macrophages from osteopontin-deficient mice. Cell Immunol 2004; 227:1-11; PMID:15051510; http://dx.doi.org/10.1016/j.cellimm.2004.01.001
- Shinohara ML, Kim HJ, Kim JH, Garcia VA, Cantor H. Alternative translation of osteopontin generates intracellular and secreted isoforms that mediate distinct biological activities in dendritic cells. Proc Natl Acad Sci U S A 2008; 105:7235-9; PMID:18480255; http://dx.doi.org/10.1073/pnas.0802301105
- Sodek J, Ganss B, McKee MD. Osteopontin. Crit Rev Oral Biol Med 2000; 11:279-303; PMID:11021631; http://dx.doi.org/10.1177/10454411000110030101
- Mirza M, Shaughnessy E, Hurley JK, Vanpatten KA, Pestano GA, He B, Weber GF. Osteopontin-c is a selective marker of breast cancer. Int J Cancer 2008; 122:889-97; PMID:17960616; http://dx.doi.org/10.1002/ijc.23204
- Zaynagetdinov R, Sherrill TP, Gleaves LA, McLoed AG, Saxon JA, Habermann AC, Connelly L, Dulek D, Peebles RS Jr, Fingleton B et al. Interleukin-5 facilitates lung metastasis by modulating the immune microenvironment. Cancer Res 2015; 75:1624-34; PMID:25691457; http://dx.doi.org/10.1158/0008-5472.CAN-14-2379
- Hsia CC, Hyde DM, Ochs M, Weibel ER. An official research policy statement of the American Thoracic Society/European Respiratory Society: standards for quantitative assessment of lung structure. Am J Respir Crit Care Med 2010; 181:394-418; PMID:20130146; http://dx.doi.org/10.1164/rccm.200809-1522ST
- Meylan E, Dooley AL, Feldser DM, Shen L, Turk E, Ouyang C, Jacks T. Requirement for NF-kappaB signalling in a mouse model of lung adenocarcinoma. Nature 2009; 462:104-7; PMID:19847165; http://dx.doi.org/10.1038/nature08462
- Stathopoulos GT, Sherrill TP, Cheng DS, Scoggins RM, Han W, Polosukhin VV, Connelly L, Yull FE, Fingleton B, Blackwell TS. Epithelial NF-kappaB activation promotes urethane-induced lung carcinogenesis. Proc Natl Acad Sci U S A 2007; 104:18514-9; PMID:18000061; http://dx.doi.org/10.1073/pnas.0705316104
- Doris K, Karabela SP, Kairi CA, Simoes DC, Roussos C, Zakynthinos SG, Kalomenidis I, Blackwell TS, Stathopoulos GT. Allergic inflammation does not impact chemical-induced carcinogenesis in the lungs of mice. Respir Res 2010; 11:118; PMID:20796309; http://dx.doi.org/10.1186/1465-9921-11-118
- Tashiro F, Niwa H, Miyazaki J. Constructing adenoviral vectors by using the circular form of the adenoviral genome cloned in a cosmid and the Cre-loxP recombination system. Hum Gene Ther 1999; 10:1845-52; PMID:10446924; http://dx.doi.org/10.1089/10430349950017527
- Boring L, Gosling J, Chensue SW, Kunkel SL, Farese RV, Jr., Broxmeyer HE, Charo IF. Impaired monocyte migration and reduced type 1 (Th1) cytokine responses in C-C chemokine receptor 2 knockout mice. J Clin Invest 1997; 100:2552-61; PMID:9366570; http://dx.doi.org/10.1172/JCI119798
- Kumar V, Behera R, Lohite K, Karnik S, Kundu GC. p38 kinase is crucial for osteopontin-induced furin expression that supports cervical cancer progression. Cancer Res 2010; 70:10381-91; PMID:20980434; http://dx.doi.org/10.1158/0008-5472.CAN-10-1470
- Mason CK, McFarlane S, Johnston PG, Crowe P, Erwin PJ, Domostoj MM, Campbell FC, Manaviazar S, Hale KJ, El-Tanani M. Agelastatin A: a novel inhibitor of osteopontin-mediated adhesion, invasion, and colony formation. Mol Cancer Ther 2008; 7:548-58; PMID:18347142; http://dx.doi.org/10.1158/1535-7163.MCT-07-2251
- Bandopadhyay M, Bulbule A, Butti R, Chakraborty G, Ghorpade P, Ghosh P, Gorain M, Kale S, Kumar D, Kumar S et al. Osteopontin as a therapeutic target for cancer. Expert Opin Ther Targets 2014; 18:883-95; PMID:24899149; http://dx.doi.org/10.1517/14728222.2014.925447
- Kazanecki CC, Uzwiak DJ, Denhardt DT. Control of osteopontin signaling and function by post-translational phosphorylation and protein folding. J Cell Biochem 2007; 102:912-24; PMID:17910028; http://dx.doi.org/10.1002/jcb.21558
- Schoensiegel F, Bekeredjian R, Schrewe A, Weichenhan D, Frey N, Katus HA, Ivandic BT. Atrial natriuretic peptide and osteopontin are useful markers of cardiac disorders in mice. Comp Med 2007; 57:546-53; PMID:18246866
- Christensen B, Kazanecki CC, Petersen TE, Rittling SR, Denhardt DT, Sorensen ES. Cell type-specific post-translational modifications of mouse osteopontin are associated with different adhesive properties. J Biol Chem 2007; 282:19463-72; PMID:17500062; http://dx.doi.org/10.1074/jbc.M703055200
- Courter D, Cao H, Kwok S, Kong C, Banh A, Kuo P, Bouley DM, Vice C, Brustugun OT, Denko NC et al. The RGD domain of human osteopontin promotes tumor growth and metastasis through activation of survival pathways. PLoS One 2010; 5:e9633; PMID:20224789; http://dx.doi.org/10.1371/journal.pone.0009633
- Pass HI, Lott D, Lonardo F, Harbut M, Liu Z, Tang N, Carbone M, Webb C, Wali A. Asbestos exposure, pleural mesothelioma, and serum osteopontin levels. N Engl J Med 2005; 353:1564-73; PMID:16221779; http://dx.doi.org/10.1056/NEJMoa051185
- Bueno R, Stawiski EW, Goldstein LD, Durinck S, De Rienzo A, Modrusan Z, Gnad F, Nguyen TT, Jaiswal BS, Chirieac LR et al. Comprehensive genomic analysis of malignant pleural mesothelioma identifies recurrent mutations, gene fusions and splicing alterations. Nat Genet 2016; 48:407-16; PMID:26928227; http://dx.doi.org/10.1038/ng.3520
- Testa JR, Cheung M, Pei J, Below JE, Tan Y, Sementino E, Cox NJ, Dogan AU, Pass HI, Trusa S et al. Germline BAP1 mutations predispose to malignant mesothelioma. Nat Genet 2011; 43:1022-5; PMID:21874000; http://dx.doi.org/10.1038/ng.912
- Gunthert U, Hofmann M, Rudy W, Reber S, Zoller M, Haussmann I, Matzku S, Wenzel A, Ponta H, Herrlich P. A new variant of glycoprotein CD44 confers metastatic potential to rat carcinoma cells. Cell 1991; 65:13-24; PMID:1707342; http://dx.doi.org/10.1016/0092-8674(91)90403-L
- Sun J, Feng A, Chen S, Zhang Y, Xie Q, Yang M, Shao Q, Liu J, Yang Q, Kong B et al. Osteopontin splice variants expressed by breast tumors regulate monocyte activation via MCP-1 and TGF-beta1. Cell Mol Immunol 2013; 10:176-82; PMID:23416968; http://dx.doi.org/10.1038/cmi.2012.67
- Sun SJ, Wu CC, Sheu GT, Chang HY, Chen MY, Lin YY, Chuang CY, Hsu SL, Chang JT. Integrin β3 and CD44 levels determine the effects of the OPN-a splicing variant on lung cancer cell growth. Oncotarget 2016; PMID:27487131; http://dx.doi.org/10.18632/oncotarget.10865; [Epub ahead of print].
- Marazioti A, Kairi CA, Spella M, Giannou AD, Magkouta S, Giopanou I, Papaleonidopoulos V, Kalomenidis I, Snyder LA, Kardamakis D et al. Beneficial impact of CCL2 and CCL12 neutralization on experimental malignant pleural effusion. PLoS One 2013; 8:e71207; PMID:23967166; http://dx.doi.org/10.1371/journal.pone.0071207
- Stathopoulos GT, Psallidas I, Moustaki A, Moschos C, Kollintza A, Karabela S, Porfyridis I, Vassiliou S, Karatza M, Zhou Z et al. A central role for tumor-derived monocyte chemoattractant protein-1 in malignant pleural effusion. J Natl Cancer Inst 2008; 100:1464-76; PMID:18840818; http://dx.doi.org/10.1093/jnci/djn325
- Zhang J, Lu Y, Pienta KJ. Multiple roles of chemokine (C-C motif) ligand 2 in promoting prostate cancer growth. J Natl Cancer Inst 2010; 102:522-8; PMID:20233997; http://dx.doi.org/10.1093/jnci/djq044
- Qian BZ, Li J, Zhang H, Kitamura T, Zhang J, Campion LR, Kaiser EA, Snyder LA, Pollard JW. CCL2 recruits inflammatory monocytes to facilitate breast-tumour metastasis. Nature 2011; 475:222-5; PMID:21654748; http://dx.doi.org/10.1038/nature10138
- Lin TH, Liu HH, Tsai TH, Chen CC, Hsieh TF, Lee SS, Lee YJ, Chen WC, Tang CH. CCL2 increases alphavbeta3 integrin expression and subsequently promotes prostate cancer migration. Biochim Biophys Acta 2013; 1830:4917-27; PMID:23845726; http://dx.doi.org/10.1016/j.bbagen.2013.06.033
- Liaw L, Skinner MP, Raines EW, Ross R, Cheresh DA, Schwartz SM, Giachelli CM. The adhesive and migratory effects of osteopontin are mediated via distinct cell surface integrins. Role of alpha v beta 3 in smooth muscle cell migration to osteopontin in vitro. J Clin Invest 1995; 95:713-24; PMID:7532190; http://dx.doi.org/10.1172/JCI117718
- Wolf MJ, Hoos A, Bauer J, Boettcher S, Knust M, Weber A, Simonavicius N, Schneider C, Lang M, Stürzl M et al. Endothelial CCR2 signaling induced by colon carcinoma cells enables extravasation via the JAK2-Stat5 and p38MAPK pathway. Cancer Cell 2012; 22:91-105; PMID:22789541; http://dx.doi.org/10.1016/j.ccr.2012.05.023
- Stathopoulos GT, Sherrill TP, Karabela SP, Goleniewska K, Kalomenidis I, Roussos C, Fingleton B, Yull FE, Peebles RS Jr, Blackwell TS. Host-derived interleukin-5 promotes adenocarcinoma-induced malignant pleural effusion. Am J Respir Crit Care Med 2010; 182:1273-81; PMID:20595227; http://dx.doi.org/10.1164/rccm.201001-0001OC