ABSTRACT
Soft-tissue sarcomas (STS) are rare malignancies that account for 1% of adult cancers and comprise more than 50 entities. Current therapeutic options for advanced-stage STS are limited. Immune checkpoint inhibitors targeting the PD-1/PD-L1 signaling axis are being explored as new treatment modality in STS; however, the determinants of response to these agents are largely unknown. Using the sarcoma data set of The Cancer Genome Altas (TCGA) and an independent cohort of untreated high-grade STS, we analyzed DNA copy number status and mRNA expression of PD-L1 in a total of 335 STS cases. Copy number gains (CNG) were detected in 54 TCGA cases (21.1%), of which 21 (8.2%) harbored focal PD-L1 CNG and that were most prevalent in myxofibrosarcoma (35%) and undifferentiated pleomorphic sarcoma (34%). In the untreated high-grade STS cohort, we detected CNG in six cases (7.6%). Analysis of co-amplified genes identified a 5.6-Mb core region comprising 27 genes, including JAK2. Patients with PD-L1 CNG had higher PD-L1 expression compared with STS without CNG (fold change, 1.8; p = 0.02), an effect that was most pronounced in the setting of focal PD-L1 CNG (fold change, 3.0; p = 0.0027). STS with PD-L1 CNG showed a significantly higher mutational load compared with tumors with a diploid PD-L1 locus (median number of mutated genes; 58 vs. 40; p = 3.6E-06), and PD-L1 CNG were associated with inferior survival (HR = 1.82; p = 0.025). In contrast, T-cell infiltrates quantified by mRNA expression of CD3Z were associated with improved survival (HR = 0.88; p = 0.024) and consequently influenced the prognostic power of PD-L1 CNG, with low CD3Z levels conferring poor survival in cases with PD-L1 CNG (HR = 1.8; p = 0.049). These data demonstrate that PD-L1 GNG and elevated expression of PD-L1 occur in a substantial proportion of STS, have prognostic impact that is modulated by T-cell infiltrates, and thus warrant investigation as response predictors for immune checkpoint inhibition.
Introduction
Soft-tissue sarcomas (STS) are a heterogeneous group of rare tumors comprising more than 50 different entities.Citation1-2,3 STS may be curable when presenting as localized disease; however, local recurrence and metastatic spread are frequent despite multimodal therapy. In patients with advanced-stage STS, conventional chemotherapy is generally administered with palliative intent, as reflected by a median survival after development of distant metastases of 12 months, and associated with substantial toxicity.Citation4,5 With the notable exception of gastrointestinal stromal tumors,Citation6,7 these data emphasize the urgent need for new therapies beyond palliation and disease control.
Pioneering work by Rosenberg and colleagues demonstrated that the immune system can be harnessed for cancer therapy. Specifically, administration of interleukin-2, either alone or in combination with tumor-infiltrating lymphocytes expanded ex vivo and chemotherapy, resulted in objective responses and even long-term survival in subsets of patients with melanoma and renal cell carcinoma.Citation8-10 However, treatment-related toxicity limited the widespread use of these regimens. Underscoring the potential of immune-mediated therapy, Robbins et al. observed partial responses in four of six patients with metastatic synovial sarcoma (SS) treated with T-cells harboring a T-cell receptor that was genetically modified to detect the NY-ESO-1 antigen.Citation11
With an increasingly better understanding of the immune system and its interactions with tumor cells, monoclonal antibodies targeting immune checkpoints involved in regulating T-cell activation were added to the arsenal of therapeutic options. For example, there is evidence from multiple clinical trials that blockade of the PD-1/PD-L1 axis can lead to durable responses in a considerable proportion of patients with advanced-stage melanoma, non-small cell lung cancer, renal cell carcinoma, bladder cancer, head and neck cancer, and Hodgkin lymphoma.Citation12 In contrast to these insights, the role of immune checkpoint inhibition in STS is largely unexplored. However, in a recent phase 2 trial of the anti-PD-1 antibody pembrolizumab, partial response or disease stabilization were observed in 19% and 40% of 40 STS patients, respectively, indicating that further investigation of this approach is warranted.Citation13
To select patients who potentially benefit from checkpoint blockade, PD-L1 expression levels on tumor cells and/or immune cells, as measured by immunohistochemistry, were introduced as predictive biomarker, although their predictive value remains controversial.Citation14,15 This prompted several investigations of PD-L1 expression across different tumor types, including STS. For example, Kim et al. reported that PD-L1 and PD-1 expression were associated with poor overall and progression-free survival in patients with leiomyosarcoma (LMS), SS, and undifferentiated pleomorphic sarcoma (UPS).Citation16 Subsequent studies corroborated the expression of PD-L1 in different STS subtypes and confirmed, in part, the association with outcome parameters.Citation17-19
A potential molecular mechanism underlying aberrant PD-L1 expression are acquired DNA copy number gains (CNG) comprising the PD-L1 locus, which have been observed in several tumor typesCitation20-23 and are being used as response predictor in clinical trials of PD-1/PD-L1 antagonists in Hodgkin lymphoma.Citation24
In contrast to epithelial and lymphoid malignancies, the prevalence of PD-L1 CNG and their association with PD-L1 expression and clinical outcome among the major STS subtypes are currently unknown. To address these issues, we interrogated the sarcoma data set of The Cancer Genome Altas (TCGA) and an independent in-house cohort of untreated high-grade STS for changes in PD-L1 copy number and mRNA expression and correlated the results with overall survival. Furthermore, we delineated the genes that are co-amplified with PD-L1 in STS and searched for an association between PD-L1 alterations and overall mutational load.
Material and methods
Analysis of the TCGA sarcoma cohort
Analysis of PD-L1 (CD274) was performed using copy number, gene expression, mutation, and clinical data from the TCGA cohort of 256 STS cases, which comprises six STS subtypes. Specifically, we analyzed dedifferentiated liposarcoma (DDLS, 58 cases), leiomysarcoma (LMS, 103 cases), malignant peripheral nerve sheath tumors (MPNST, 9 cases), myxofibrosarcoma (MFS, 23 cases), SS (10 cases), undifferentiated pleomorphic sarcoma (UPS, 50 cases), desmoid tumors (2 cases), and STS not otherwise specified (1 case). In line with TCGA publication guidelines, we included only data sets that are publicly available without restrictions (http://cancergenome.nih.gov/).
Data retrieval, data analysis, and graphics generation were performed using the statistical programming language R.Citation25 Putative copy number alterations (GISTIC), mRNA expression data (RNA Seq V2 RSEM), and clinical data, including overall and disease-free survival were retrieved from the Cancer Genomic Data Server (http://www.cbioportal.org/public-portal) using the R package cgdsr.Citation26
Classification of copy number alterations as focal, arm, or chromosome-scale was based on genomic information retrieved from the R annotation package org.Hs.eg.db.Citation27 CNG were classified as arm alterations if, 90% or more genes on the chromosome arm were amplified. CNG were classified as chromosome alterations if, 90% or more genes on the chromosome were amplified. Differences in gene expression were assessed using fold changes, and significance was assessed by Welch's t-test.
Somatic mutation data (level 2) from STS cases were retrieved from the TCGA Data Portal (https://tcga-data.nci.nih.gov/tcga/dataAccessMatrix.htm). To assess mutational load, the number of genes carrying at least one nonsynonymous mutation in the coding region was computed for each tumor. The significance of the difference in mutational load between tumors with and without PD-L1 CNG was computed using the Wilcoxon test. Univariate survival analysis was performed using the Kaplan–Meier method and proportional hazards modeling as implemented by the functions survfit and coxph of the R package survival.Citation28 Significance of hazard ratios (HR) was assessed using the log-rank test.
RNA sequencing data of immune genes were put to log2 scale and centered to mean = 0 and scaled to variance = 1. Data were visualized using the function heatmap from the R package stats. Hierarchical clustering was performed using Pearson correlations as similarity measure and the average linkage method to calculate distances between clusters. The CD4/CD8 ratio was calculated as 2×CD4/(CD8A+CD8B). Analysis of overall survival was performed using continuous data of mRNA expression. HR refer to a doubling of gene expression. Significance was assessed using the log-rank test. A combined analysis of immune gene expression and PD-L1 copy number status was performed by stratifying patients in four subgroups defined by dichotomized CD3Z (CD247) mRNA expression (cutoff, median) and presence or absence of PD-L1 CNG.
Analysis of the untreated high-grade STS cohort
The second cohort comprised samples from 79 patients with untreated high-grade STS (UPS, LMS, SS, MPNST, DDLS, MFS, myxoid liposarcoma (MLS), and pleomorphic liposarcoma (PLS)) that were collected at the Institute of Pathology, Heidelberg University Hospital, Heidelberg, Germany, between 1989 and 2008. Tumors were categorized according to current World Health Organization criteria,Citation1 and grading was performed using the Fédération Nationale des Centers de Lutte Contre le Cancer system.Citation29 Cases of MLS, SS, and DDLS were also verified by detection of the FUS-DDIT3 and SS18-SSX fusion genes and MDM2 amplification, respectively.
Array-based comparative genomic hybridization (aCGH) was performed as described previously.Citation30 In brief, 1.5 µg tumor or gender-matched control DNA were labeled with the Genomic DNA ULS Labeling Kit (Agilent Technologies, Waldbronn, Germany). Microarray processing, including hybridization, washing, and scanning steps, was performed according to the manufacturer's recommendations (Agilent Technologies). Data extraction was done using Feature Extraction Software v10 (Agilent Technologies). The R package DNAcopy was used for normalization of profiles, and outliers were smoothed. The R package CGHcall was used for calling of copy number profiles. Gene expression profiling was performed using HumanHT-12 v3 Expression BeadChip technology (Illumina, San Diego, USA) as described previously,Citation31 and the data set is available at NCBI's Gene Expression Omnibus (http://www.ncbi.nlm.nih.gov/geo; accession number GSE52392). The study was approved by the Ethics Committee of the Medical Faculty Heidelberg (ethics votes 206/2005 and 207/2005). Differences in PD-L1 mRNA expression were assessed using fold changes and p-values from Welch's t-test, and correlation analysis of PD-L1 protein expression and PD-L1 CNG was performed by assessing the overlap between positive cases using Fisher's exact test.
Fluoresence in situ hybridization (FISH) using a commercially available dual-color probe for the PD-L1 (CD274) gene (Zytovision; Germany, #Z-2179–200) was performed on whole slides according to the manufacturer's instructions. Four-micrometer-thick sections were cut, mounted on SuperFrost slides, and deparaffinized. Immunhistochemical staining was performed on formalin-fixed and paraffin-embedded (FFPE) whole-tissue slides using a BenchMark ULTRA device (Ventana Medical Systems, Tucson, AZ, USA) according to quality-controlled standard procedures. After antigen retrieval, a monoclonal anti-rabbit antibody (Ventana/Roche, clone: SP263, conc.: 1.61 µg/mL; prediluted) was used and 3,3′ diaminobenzidine (DAB) served as chromogen. According to the manufacturer, membraneous and/or cytoplasmic staining patterns can be observed. Detailed characteristics of the antibody were recently published by Rebelatto et al.Citation32
Results
Landscape of PD-L1 copy number gains
We detected PD-L1 CNG in 54 of 256 cases (21.1%) from the TCGA sarcoma cohort. Of these, 21 (8.2%) harbored focal PD-L1 CNG. As shown in , the frequency of PD-L1 CNG varied among the different STS subtypes and was highest in MFS (35%) and UPS (34%), followed by DDLS (21%), LMS (16%), MPNST (11%), and SS (10%). In an independent cohort of 79 patients with primary untreated high-grade STS, we detected focal PD-L1 CNG in six tumors (7.6%; ), including SS, UPS, LMS, and DDLS. No PD-L1 CNG were observed in MFS, PLS, MLS, and MPNST. For six cases, we validated PD-L1 CNG and associated protein expression by FISH and immunohistochemistry, respectively. Three representative cases are shown in .
Figure 1. PD-L1 CNG in STS. (A) Percentages of focal gains, 9p gains, and chromosome 9 gains in different STS subtypes in the TCGA cohort. (B) Percentages of PD-L1 CNG in the independent high-grade sarcoma cohort. (C) Overview of PD-L1 co-amplified genes on chromosome 9p. A core region including 27 genes (length, 5.6 Mb; inset) was co-amplified in more than 80% of STS with focal PD-L1 CNG (TCGA cohort).
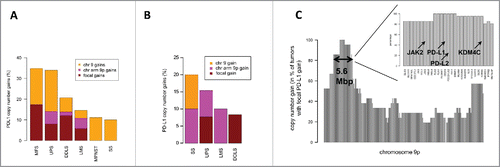
Figure 2. Immunohistochemical and FISH analysis of PD-L1 in 48 cases of the high-grade STS cohort. (A) Immunhistochemical staining and FISH for PD-L1. (1) PD-L1 staining of lung adenocarcinoma serving as a positive control. Note the membranous and cytoplasmic staining of tumor cells as well as membranous staining of inflammatory cells. (2) PD-L1 staining of tonsillar tissue illustrates membranous staining of lymphocytes and antigen presenting cells. PD-L1 CNG and protein expression in STS cases without (3) and with (5, and 7) CNG as detected by aCGH. Higher magnifications are presented aside (4, 6, and 8). A case of UPS (3, and 4) with balanced PD-L1 copy number on aCGH analysis showing absent PD-L1 protein expression. Representative pictures showing membranous staining of PD-L1 in a sample of DDLS (5, and 6) and UPS (7, and 8). Insets illustrates results of FISH analyses demonstrating PD-L1 copy number status (PD-L1/CEN9 dual-color FISH probe; green, PD-L1; red, CEN9; blue, DNA). Scale bars, 50 µm. (B) Pie chart showing the association of PD-L1 CNG, PD-L1 staining of tumors cells and PD-L1 staining of immune cells.
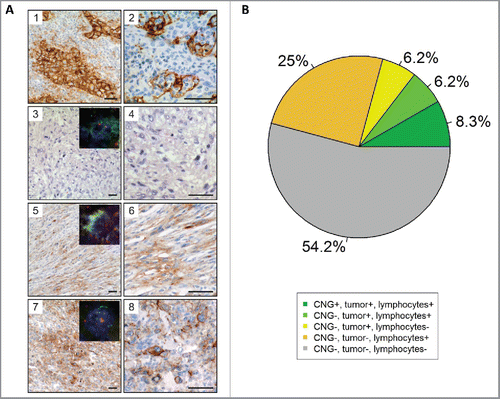
In the TCGA sarcoma cohort, analysis of co-amplified genes located on chromosome arm 9p identified a 5.6-Mb core region comprising 27 genes (). In addition to PD-L1, this region included PD-L2, encoding the second PD1 ligand, and JAK2, encoding a cytoplasmic tyrosine kinase.
PD-L1 mRNA and protein expression
In the TCGA data set (), PD-L1 expression was significantly higher in MFS compared with DDLS (fold change = 2.2; p = 0.036) and SS (fold change = 12.2; p = 0.00012). Likewise, PD-L1 expression was significantly higher in LMS compared with DDLS (fold change = 1.5; p = 0.039) and SS (fold change = 8.6; p = 0.00047). PD-L1 expression in SS was significantly lower compared with each of the other subtypes. In the independent cohort of primary untreated high-grade STS (), PD-L1 expression was highest in LMS and UPS, whereas PD-L1 expression was significantly lower (p < 0.05) in MLS compared with all other subtypes except MPNST. As observed in the TCGA data set, SS showed significantly lower PD-L1 expression compared with LMS (p = 0.0012), UPS (p = 0.013), and MFS (p = 0.025). To verify these results at the protein level, we performed PD-L1 immunhistochemistry in 48 cases (60.1% of the untreated high-grade STS cohort) for which the same FFPE tissue block was available that was used for aCGH (, for details see Table S1). We detected PD-L1-positive tumor cells in 10 (20.8%) and PD-L1-positive lymphocytes in 19 (39.6%) of 48 tumors. Seven tumors were PD-L1-positive for both tumor cells and immune cells, resulting in a significant positive correlation between tumor cell and immune cell PD-L1 protein expression (p = 0.036). While the correlation between PD-L1 protein expression of tumor cells and PD-L1 mRNA expression, as determined by microarray analysis of bulk tissues, was not significant (fold change = 1.1; p = 0.63), we noted a borderline significant correlation between PD-L1 protein expression in immune cells and PD-L1 mRNA levels (fold change = 1.2; p = 0.075).
Figure 3. PD-L1 mRNA expression in the TCGA cohort (A) and in the independent cohort of high-grade STS (B). In the TCGA cohort, sarcomas with PD-L1 CNG showed higher PD-L1 expression than STS without PD-L1 CNG (fold change, 1.8; p = 0.02). PD-L1 expression was significantly higher in MFS compared with DDLS (fold change, 2.2; p = 0.036) and SS (fold change, 12.2; p = 0.00012). Furthermore, PD-L1 expression in LMS was significantly higher than in DDLS (fold change, 1.5; p = 0.039) and SS (fold change, 8.6; p = 0.00047). In the high-grade STS cohort, LMS and UPS cases showed highest PD-L1 expression levels, whereas PD-L1 expression was significantly lower (p < 0.05) in MLS compared with all other subtypes except MPNST.
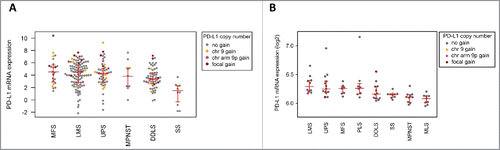
Association between PD-L1 CNG, mRNA expression, and mutational load
In the TCGA cohort, samples with PD-L1 CNG were characterized by higher PD-L1 expression compared with samples without PD-L1 CNG (fold change = 1.8; p = 0.02). As expected, the effect of focal PD-L1 CNG on PD-L1 expression was even more pronounced (fold change = 3.0; p = 0.0027), particularly in LMS (fold change = 3.1; p = 0.04), and UPS (fold change = 4.9; p = 0.055). The median number of mutated genes () was highest in MFS (64), followed by MPNST (56), UPS (53), LMS (46), DDLS (42), and SS (36). Samples with PD-L1 CNG showed a significantly higher mutational load compared with samples without PD-L1 amplification (median number of mutated genes: 58 vs. 40; p = 3.6E-06). This was especially pronounced in the subgroup of DDLS (55 vs. 34; p = 0.0020). Among the 48 cases from the untreated high-grade STS cohort that were immunostained for PD-L1 (Table S1), detection of PD-L1-positive tumor cells correlated significantly with PD-L1 CNG (p = 0.0011). Of the 10 tumors with PD-L1 immunoreactivity in tumor cells, four showed PD-L1 CNG. The remaining 38 cases showed no PD-L1 CNG and were negative for PD-L1 protein expression.
Figure 4. Analysis of mutational load in STS (TCGA cohort). (A) The median number of mutated genes was highest in MFS, followed by MPNST, UPS, LMS, DDLS, and SS. (B) Sarcomas with PD-L1 CNG and PD-L1 copy number losses showed a significantly higher mutational load compared with tumors without PD-L1 CNG.
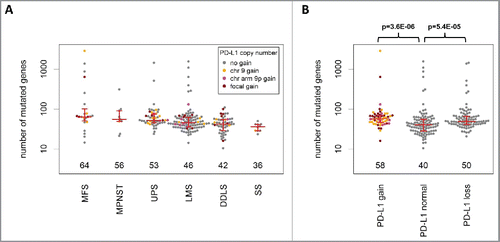
Prognostic impact of PD-L1 copy number gains
PD-L1 CNG were associated with significantly inferior overall survival across the entire TCGA sarcoma cohort (HR = 1.82; p = 0.025; ) and in the subgroup of LMS (HR = 2.84; p = 0.013; ). Separate analysis of focal, chromosome 9p, and chromosome 9 alterations revealed that only chromosome 9p gains were significantly associated with poorer outcome in the entire TCGA sarcoma cohort (HR = 5.12; p = 2.2E-05; ) and in LMS (HR = 6.01; p = 0.00053; ).
Figure 5. Prognostic impact of PD-L1 CNG in STS (TCGA cohort). PD-L1 CNG were associated with shortened overall survival in (A) the entire TCGA cohort (162 patients, 56 events) and (B) in the LMS subcohort 63 patients, 22 events). Chromosome 9p gains were associated with shortened overall survival (C) in the entire TCGA cohort (162 patients, 56 events) and (D) in the LMS subcohort (63 patients, 22 events).
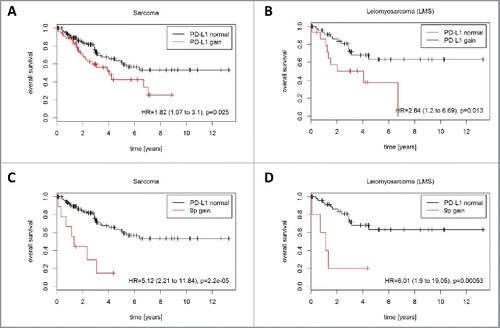
Expression of immune genes in the tumor microenvironment
To explore the role of immune cells, we investigated the expression of T-cell- (CD3, CD4, CD8) and macrophage (CD4, CD68) markers as well as PD-1 and PD-L1 transcript levels in the TCGA cohort ( and ). CD3 chains (CD3D, CD3E, CD3G, and CD3Z (CD247) were strongly co-expressed with CD8 (CD8A, CD8B) as well as with PD-1 (R > 0.75 for all pairwise Pearson correlations), whereas the expression of macrophage markers only partly overlapped with that of T-cell-specific genes. PD-L1 showed a distinctly different expression pattern compared with the other immune genes (R < 0.55 for all pairwise Pearson correlations). The described expression patterns were observed in the entire TCGA cohort and specifically in LMS.
Figure 6. Expression and prognostic impact of immune genes in STS (TCGA cohort). (A) Analysis of co-expression patterns and correlation of mRNA expression with overall survival in all STS. (B) Analysis of co-expression patterns and correlation of mRNA expression with overall survival in LMS. (C) Inferior prognosis of CD3Z low/PD-L1 CNG sarcomas. (D) Inferior prognosis of CD3Z low/PD-L1 CNG LMS.
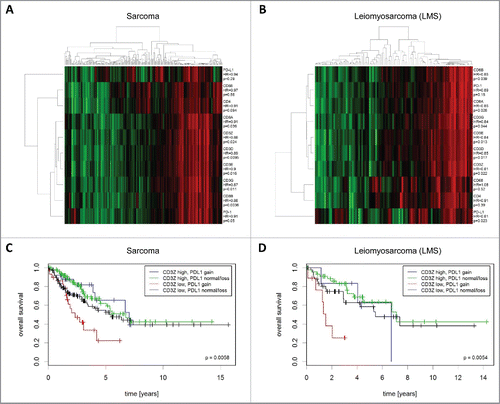
Prognostic impact of immune genes
The mRNA levels of the different T-cell-related genes were positively correlated with overall survival both in the entire TCGA cohort and specifically in LMS. For example, the HR per doubling of mRNA expression of CD3D were 0.89 (confidence interval [CI]: 0.82–0.97; p = 0.0095) in the entire cohort and 0.85 (CI: 0.74–0.97; p = 0.017) in LMS. There was no significant correlation between CD4 or CD68 expression and prognosis in the entire cohort or in LMS. The CD4/CD8 ratio was not prognostic in the entire cohort, but associated with significantly inferior outcome in LMS (HR = 1.34; CI, 1.09–1.65; p = 0.0053).
Prognostic impact of PD-L1 CNG and CD3Z levels
Finally, we sought to investigate the combined impact of immune gene expression and PD-L1 copy number status on prognosis. To this end, we stratified patients from the TCGA cohort by combining CD3Z mRNA expression and PD-L1 copy number status. This analysis demonstrated that low CD3Z expression in combination with PD-L1 CNG was a negative prognostic factor ( and ). Specifically, the outcome of tumors with PD-L1 CNG and low CD3Z levels was inferior compared with that of CD3Z low/PD-L1 normal/loss (HR = 1.8; p = 0.049), CD3Z high/PD-L1 normal/loss (HR = 3.08; p = 0.00019), or CD3Z high/PD-L1 CNG tumors (HR = 3.29; p = 0.0098). Similar associations of these two markers with prognosis were found in the subgroup of patients with LMS (HR = 2.92 (p = 0.024), HR = 6.8 (p = 0.0001), and HR = 6.16 (p = 0.057)).
Discussion
We here report the first analysis of PD-L1 CNG across major STS subtypes and analyzed associations with PD-L1 expression levels, mutational load, immune infiltrates, as well as survival. We observed a significant correlation of PD-L1 CNG with increased PD-L1 expression across all STS subtypes and for UPS and LMS in particular. These data are in line with our previous analysis of 22 major cancer types,Citation23 and in the light of recent trial data of patients with relapsed or refractory Hodgkin lymphoma,Citation24 it is reasonable to argue that PD-L1 CNG could also be predictive of response toward immune checkpoint blockade in STS. In line with this notion, a recent study from our group identified a patient with metastastic cancer of unknown primary and PD-L1 amplification, who achieved a durable response to PD-1 blockade.Citation33 As the predictive power of PD-L1 expression varies considerably due to a multitude of reasons, including diagnostic assays, antibody clones, and tumor types,Citation34,35 these data collectively open up new avenues for identifying adjunct parameters that may improve response prediction.
In keeping with a recent report from Roemer et al. on the prognostic significance of PD-L1 and PD-L2 alterations in classical Hodgkin lymphoma,Citation36 we found that chromosome 9p gains were associated with poor survival in the entire set of STS patients investigated and particularly in patients with LMS. These observations underscore the biological relevance of 9p amplification and, in particular, focal amplification of PD-L1 and framing genes,Citation23,32 which likely confer oncogenic properties by faciliating evasion of 9p/PD-L1-amplified cancer cells from attack by the immune system.
We also identified 27 genes framing PD-L1 that are co-amplified in more than 80% of STS cases with PD-L1 CNG. Hence, it is likely that other genes beyond PD-L1 contribute to a specific tumor biology that confers a dismal prognosis in patients with 9p-amplified STS. Importantly, some of these putative “co-drivers” might represent therapeutic targets. For instance, a recent report by Balko et al.Citation37 showed that triple-negative breast cancers harboring 9p24 amplifications are dependent on JAK2 signaling. KDM4C, encoding a lysine demethylase, was recently reported to facilitate leukemic transformation, and combined inhibition of PRMT1 and KDM4C abrogated aberrant transcriptional programs mediating leukemia development in mice.Citation38 In addition, KDM4C appears to be implicated in regulating chromosome segregation, thereby influencing genomic instability.Citation39 Conversely, the chromosome 9p gains observed here might also be the result of a more general mechanism of genetic instability surgical in STS.
The varying incidence of PD-L1 CNG between the TCGA data set and the high-grade STS cohort may be attributed to differences in the respective patient populations. For example, the histologic subtypes most commonly associated with PD-L1 CNG (UPS, DDLS, and LMS) account for 82% and 44% of cases in the TCGA cohort and in the high-grade STS cohort, respectively. Furthermore, the high-grade STS cohort is exclusively composed of untreated high-grade sarcomas, whereas the TCGA cohort also includes lower-grade tumors and possibly pre-treated cases. Of particular clinical relevance, immunohistochemistry analysis of 48 cases from the untreated high-grade STS cohort revealed a significant association between PD-L1 CNG and protein expression. As expected, we also observed cases without CNG that express PD-L1, reflecting other mechanisms of PD-L1 upregulation described in the literature.Citation15 In this context, it is important to note that we cannot exclude some bias in the immunohistochemical results as the untreated high-grade STS cases had been selected for high tumor cell content and particularly low immune cell levels to facilitate optimal aCGH analysis.Citation31 Additionally, it is well known that different antibody clones yield different staining results that may influence immunohistochemistry-based expression analysis, and PD-L1 levels may show spatial intratumoral heterogeneity,Citation40,41 a point that we addressed by using whole-tissue slides instead of a tissue microarray.
Mutational load has gained considerable attention as surrogate marker for neoantigens that stimulate the immune system, and several tumor types with particularly high mutational burden have been identified.Citation42,43 Several clinical studies showed that assessment of mutational load can be used to predict response to immunotherapies in a variety of solid tumors, including microsatellite instability-driven colorectal cancer, melanoma, non-small cell lung cancer, and possibly viral antigen-negative Merkel cell carcinoma.Citation44-49 While we noted statistically significant differences in mutational load among STS subtypes, with SS showing fewer mutations and MFS exhibiting a relatively high mutational burden, our analysis suggests that the number of non-synonymous mutations per se might not be an ideal predictor of response to immune checkpoint inhibition, as the range of mutational loads across subtypes is rather narrow and only few cases harbored 100 or more mutated genes. However, as some STS subtypes in the TCGA data set, e.g. MPNST and SS, are represented by very few cases, the data might be too preliminary to draw definite conclusions. The same notion might apply to the prevalence of PD-L1 CNG in MPNST, SS, and MFS, where only 9, 10, and 23 cases were available.
In the TCGA cohort, we found T-cell infiltrates in STS to correlate with improved survival, a phenomenon that has been observed in several tumor entities.Citation50,51 Interestingly, T-cell infiltrates also appear to modify the prognostic value of PD-L1 CNG in STS, a finding that is in keeping with the concept that both the levels and composition of immune cell infiltrates are key components in the multifaceted interaction between the immune system and cancer cells, which shapes the biological behavior of the tumor.Citation52,53 Immune infiltrates have also been reported to predict therapy response, which raises the intriguing question whether STS cases with a “hot” microenvironment may respond differently to immune checkpoint blockade compared with “cold” tumors.
Our analysis might be of particular interest in light of preliminary data from SARC028, a phase II trial investigating the efficacy and safety of pembrolizumab in advanced STS,Citation13 which demonstrated that a substantial proportion of patients with UPS, LMS, SS, and liposarcoma either responded or had stable disease. Considering that current therapeutic options for advanced STS are limited,Citation5 these results suggest that immune checkpoint inhibitors might be a viable therapeutic option in a relevant fraction of STS patients.Citation54-56 However, an important challenge will be to identify patients most likely to benefit from PD-1/PD-L1 blockade. Our results suggest that studies such as SARC028 might be ideally suited to assess the predictive value of PD-L1 CNG and the composition of immune infiltrates in STS, a tempting question that we could not answer by our analysis as therapy data for patients included in the TCGA sarcoma cohort were not available and immune checkpoint inhibition was no standard of care at initiation of the TCGA study.
In summary, together with the encouraging data on the efficacy of the PD-1 blocker pembrolizumab in advanced STS,Citation13 our results support further exploration of the predictive power of PD-L1 CNG, mutational load, and immune cell infiltrates in STS to broaden the therapeutic armamentarium for this challenging group of diseases.
Disclosure of potential conflicts of interest
No potential conflicts of interest were disclosed.
KONI_A_1279777_s02.docx
Download MS Word (22.2 KB)Funding
This study was funded by the German Cancer Consortium (DKTK).
References
- Fletcher CD, Hogendoorn P, Mertens F, Bridge J. WHO Classification of Tumours of Soft Tissue and Bone. 4th ed. Lyon, France: IARC Press, 2013
- Brooks JS, Lee S. Contemporary diagnostics: sarcoma pathology update. J Surg Oncol 2015; 111:513-9; PMID:25689920; http://dx.doi.org/10.1002/jso.23853
- Ducimetière F, Lurkin A, Ranchère-Vince D, Decouvelaere AV, Péoc'h M, Istier L, Chalabreysse P, Muller C, Alberti L, Bringuier PP et al. Incidence of sarcoma histotypes and molecular subtypes in a prospective epidemiological study with central pathology review and molecular testing. PLoS One 2011; 6:e20294; PMID:21826194; http://dx.doi.org/10.1371/journal.pone.0020294
- Karavasilis V, Seddon BM, Ashley S, Al-Muderis O, Fisher C, Judson I. Significant clinical benefit of first-line palliative chemotherapy in advanced soft-tissue sarcoma: retrospective analysis and identification of prognostic factors in 488 patients. Cancer 2008; 112:1585-91; PMID:18278813; http://dx.doi.org/10.1002/cncr.23332
- Linch M, Miah AB, Thway K, Judson IR, Benson C. Systemic treatment of soft-tissue sarcoma-gold standard and novel therapies. Nat Rev Clin Oncol 2014; 11:187-202; PMID:24642677; http://dx.doi.org/10.1038/nrclinonc.2014.26
- Verweij J, Casali PG, Zalcberg J, LeCesne A, Reichardt P, Blay JY, Issels R, van Oosterom A, Hogendoorn PC, Van Glabbeke M et al. Progression-free survival in gastrointestinal stromal tumours with high-dose imatinib: randomised trial. Lancet 2004; 364:1127-34; PMID:15451219; http://dx.doi.org/10.1016/S0140-6736(04)17098-0
- Blanke CD, Rankin C, Demetri GD, Ryan CW, von Mehren M, Benjamin RS, Raymond AK, Bramwell VH, Baker LH, Maki RG et al. Phase III randomized, intergroup trial assessing imatinib mesylate at two dose levels in patients with unresectable or metastatic gastrointestinal stromal tumors expressing the kit receptor tyrosine kinase: S0033. J Clin Oncol 2008;26:626-32; PMID:18235122; http://dx.doi.org/10.1200/JCO.2007.13.4452
- Rosenberg SA, Lotze MT, Yang JC, Aebersold PM, Linehan WM, Seipp CA, White DE. Experience with the use of high-dose interleukin-2 in the treatment of 652 cancer patients. Ann Surg 1989; 210:474-84; PMID:2679456; http://dx.doi.org/10.1097/00000658-198910000-00008
- Rosenberg SA. IL-2: the first effective immunotherapy for human cancer. J Immunol 2014; 192:5451-8; PMID:24907378; http://dx.doi.org/10.4049/jimmunol.1490019
- Rosenberg SA, Restifo NP. Adoptive cell transfer as personalized immunotherapy for human cancer. Science 2015; 348:62-8; PMID:25838374; http://dx.doi.org/10.1126/science.aaa4967
- Robbins PF, Morgan RA, Feldman SA, Yang JC, Sherry RM, Dudley ME, Wunderlich JR, Nahvi AV, Helman LJ, Mackall CL et al. Tumor regression in patients with metastatic synovial cell sarcoma and melanoma using genetically engineered lymphocytes reactive with NY-ESO-1. J Clin Oncol 2011; 29:917-24; PMID:21282551; http://dx.doi.org/10.1200/JCO.2010.32.2537
- Lipson EJ, Forde PM, Hammers HJ, Emens LA, Taube JM, Topalian SL. Antagonists of PD-1 and PD-L1 in Cancer Treatment. Semin Oncol 2015;42:587-600; PMID:26320063; http://dx.doi.org/10.1053/j.seminoncol.2015.05.013
- Tawbi H. Safety and efficacy of PD-1 blockade using pembrolizumab in patients with advanced soft tissue (STS) and bone sarcomas (BS): Results of SARC028—A multicenter phase II study. Presented at ASCO 2016, Chicago, Abstract# 11006
- Topalian SL, Hodi FS, Brahmer JR, Gettinger SN, Smith DC, McDermott DF, Powderly JD, Carvajal RD, Sosman JA, Atkins MB et al. Safety, activity, and immune correlates of anti-PD-1 antibody in cancer. N Engl J Med 2012; 366:2443-54; PMID:22658127; http://dx.doi.org/10.1056/NEJMoa1200690
- Topalian SL, Taube JM, Anders RA, Pardoll DM. Mechanism-driven biomarkers to guide immune checkpoint blockade in cancer therapy. Nat Rev Cancer 2016; 16:275-87; PMID:27079802; http://dx.doi.org/10.1038/nrc.2016.36
- Kim JR, Moon YJ, Kwon KS, Bae JS, Wagle S, Kim KM, Park HS, Lee H, Moon WS, Chung MJ et al. Tumor infiltrating PD1-positive lymphocytes and the expression of PD-L1 predict poor prognosis of soft tissue sarcomas. PLoS One 2013; 8:e82870; PMID:24349382; http://dx.doi.org/10.1371/journal.pone.0082870
- D'Angelo SP, Shoushtari AN, Agaram NP, Kuk D, Qin LX, Carvajal RD, Dickson MA, Gounder M, Keohan ML, Schwartz GK et al. Prevalence of tumor-infiltrating lymphocytes and PD-L1 expression in the soft tissue sarcoma microenvironment. Hum Pathol 2015; 46:357-65; PMID:25540867; http://dx.doi.org/10.1016/j.humpath.2014.11.001
- Raj S, Bui M, Gonzales R, Letson D, Antonia SJ. Impact of PDL1 expression on clinical outcomes in subtypes of sarcoma. Ann Oncol 2014; 25 (suppl 4):iv498; http://doi.org/10.1093/annonc/mdu354.10
- Movva S, Wen W, Chen W, Millis SZ, Gatalica Z, Reddy S, von Mehren M, Van Tine BA. Multi-platform profiling of over 2000 sarcomas: identification of biomarkers and novel therapeutic targets. Oncotarget 2015; 6:12234-47; PMID:25906748; http://dx.doi.org/10.18632/oncotarget.3498
- Inoue Y, Yoshimura K, Mori K, Kurabe N, Kahyo T, Mori H, Kawase A, Tanahashi M, Ogawa H, Inui N et al. Clinical significance of PD-L1 and PD-L2 copy number gains in non-small-cell lung cancer. Oncotarget 2016; 7:32113-28; PMID:27050074; http://dx.doi.org/10.18632/oncotarget.8528.
- Van Roosbroeck K, Ferreiro JF, Tousseyn T, van der Krogt JA, Michaux L, Pienkowska-Grela B, Theate I, De Paepe P, Dierickx D, Doyen C et al. Genomic alterations of the JAK2 and PDL loci occur in a broad spectrum of lymphoid malignancies. Genes Chromosomes Cancer 2016; 55:428-41; PMID:26850007; http://dx.doi.org/10.1002/gcc.22345
- Barrett MT, Anderson KS, Lenkiewicz E, Andreozzi M, Cunliffe HE, Klassen CL, Dueck AC, McCullough AE, Reddy SK, Ramanathan RK et al. Genomic amplification of 9p24.1 targeting JAK2, PD-L1, and PD-L2 is enriched in high-risk triple negative breast cancer. Oncotarget 2015; 6:26483-93; PMID:26317899; http://dx.doi.org/10.18632/oncotarget.4494
- Budczies J, Bockmayr M, Denkert C, Klauschen F, Gröschel S, Darb-Esfahani S, Pfarr N, Leichsenring J, Onozato ML, Lennerz JK et al. Pan-cancer analysis of copy number changes in programmed death-ligand 1 (PD-L1, CD274) – associations with gene expression, mutational load, and survival. Genes Chromosomes Cancer 2016; 55:626-39; PMID:27106868; http://dx.doi.org/10.1002/gcc.22365
- Ansell SM, Lesokhin AM, Borrello I, Halwani A, Scott EC, Gutierrez M, Schuster SJ, Millenson MM, Cattry D, Freeman GJ et al. PD-1 blockade with nivolumab in relapsed or refractory Hodgkin's lymphoma. N Engl J Med 2015; 372:311-9; PMID:25482239; http://dx.doi.org/10.1056/NEJMoa1411087
- R Core Team. 2013. R: A language and environment for statistical computing. R Foundation for Statistical Computing, Vienna, Austria. URL: http://www.R-project.org/
- Jacobson A. R-Based API for Accessing the MSKCC Cancer Genomics Data Server. R package version 2015; 1.2.5. http://CRAN.R-project.org/package
- Carlson M. Genome wide annotation for Human, primarily based on mapping using Entrez Gene identifiers. R package version 2015; 3.0.0
- Therneau TM, Grambsch PM. Modeling Survival Data: Extending the Cox Model. USA: Springer, 2000.
- Coindre JM. Grading of soft tissue sarcomas: review and update. Arch Pathol Lab Med 2006; 130:1448-53; PMID:17090186
- Chudasama P, Renner M, Straub M, Mughal SS, Hutter B, Kosaloglu Z, Schweßinger R, Scheffler M, Alldinger I, Schimmack S et al. Targeting fibroblast growth factor receptor 1 for treatment of soft-tissue sarcoma. Clin Cancer Res 2016; pii: clincanres.0860.2016; PMID:27535980; http://dx.doi.org/10.1158/1078-0432.CCR-16-0860 [Epub ahead of print]
- Renner M, Wolf T, Meyer H, Hartmann W, Penzel R, Ulrich A, Lehner B, Hovestadt V, Czwan E, Egerer G et al. Integrative DNA methylation and gene expression analysis in high-grade soft tissue sarcomas. Genome Biol 2013; 14:r137; PMID:24345474; http://dx.doi.org/10.1186/gb-2013-14-12-r137
- Rebelatto MC, Midha A, Mistry A, Sabalos C, Schechter N, Li X, Jin X, Steele KE, Robbins PB, Blake-Haskins JA et al. Development of a programmed cell death ligand-1 immunohistochemical assay validated for analysis of non-small cell lung cancer and head and neck squamous cell carcinoma. Diagn Pathol 2016; 11:95; PMID:27717372; http://dx.doi.org/10.1186/s13000-016-0545-8
- Gröschel S, Bommer M, Hutter B, Budczies J, Bonekamp D, Heining C, Horak P, Fröhlich M, Uhrig S, Hübschmann D et al. Integration of genomics and histology revises diagnosis and enables effective therapy of refractory cancer of unknown primary with PDL1 amplification. Cold Spring Harb Mol Case Stud 2016; 2:a001180; PMID:27900363; http://dx.doi.org/10.1101/mcs.a001180
- McLaughlin J, Han G, Schalper KA, Carvajal-Hausdorf D, Pelekanou V, Rehman J, Velcheti V, Herbst R, LoRusso P, Rimm DL. Quantitative assessment of the heterogeneity of PD-L1 expression in non-small-cell lung cancer. JAMA Oncol 2016; 2:46-54; PMID:26562159; http://dx.doi.org/10.1001/jamaoncol.2015.3638
- Patel SP, Kurzrock R. PD-L1 Expression as a predictive biomarker in cancer immunotherapy. Mol Cancer Ther 2015; 14:847-56; PMID:25695955; http://dx.doi.org/10.1158/1535-7163.MCT-14-0983
- Roemer MG, Advani RH, Ligon AH, Natkunam Y, Redd RA, Homer H, Connelly CF, Sun HH, Daadi SE, Freeman GJ et al. PD-L1 and PD-L2 genetic alterations define classical hodgkin lymphoma and predict outcome. J Clin Oncol 2016; 34(23):2690-7; PMID:27069084; http://dx.doi.org/10.1200/JCO.2016.66.4482
- Balko JM, Schwarz LJ, Luo N, Estrada MV, Giltnane JM, Dávila-González D, Wang K, Sánchez V, Dean PT, Combs SE et al. Triple-negative breast cancers with amplification of JAK2 at the 9p24 locus demonstrate JAK2-specific dependence. Sci Transl Med 2016; 8:334ra53; PMID:27075627; http://dx.doi.org/10.1126/scitranslmed.aad3001
- Cheung N, Fung TK, Zeisig BB, Holmes K, Rane JK, Mowen KA, Finn MG, Lenhard B, Chan LC, So CW. Targeting aberrant epigenetic networks mediated by PRMT1 and KDM4C in acute myeloid leukemia. Cancer Cell 2016; 29:32-48; PMID:26766589; http://dx.doi.org/10.1016/j.ccell.2015.12.007
- Kupershmit I, Khoury-Haddad H, Awwad SW, Guttmann-Raviv N, Ayoub N. KDM4C (GASC1) lysine demethylase is associated with mitotic chromatin andregulates chromosome segregation during mitosis. Nucleic Acids Res 2014; 42:6168-82; PMID:24728997; http://dx.doi.org/10.1093/nar/gku253
- McLaughlin J, Han G, Schalper KA, Carvajal-Hausdorf D, Pelekanou V, Rehman J, Velcheti V, Herbst R, LoRusso P, Rimm DL. Quantitative assessment of the heterogeneity of PD-L1 expression in non-small-cell lung cancer. JAMA Oncol 2016; 2:46-54; PMID:26562159; http://dx.doi.org/10.1001/jamaoncol.2015.3638
- Scheel AH, Dietel M, Heukamp LC, Jöhrens K, Kirchner T, Reu S, Rüschoff J, Schildhaus HU, Schirmacher P, Tiemann M et al. Harmonized PD-L1 immunohistochemistry for pulmonary squamous-cell and adenocarcinomas. Mod Pathol 2016; 29:1165-72; PMID:27389313; http://dx.doi.org/10.1038/modpathol.2016.117
- Chabanon RM, Pedrero M, Lefebvre C, Marabelle A, Soria JC, Postel-Vinay S. Mutational landscape and sensitivity to immune checkpoint blockers. Clin Cancer Res 2016; 22:4309-21; PMID:27390348; http://dx.doi.org/10.1158/1078-0432.CCR-16-0903
- Budczies J, Bockmayr M, Denkert C, Klauschen F, Lennerz JK, Györffy B, Dietel M, Loibl S, Weichert W, Stenzinger A. Classical pathology and mutational load of breast cancer – integration of two worlds. J Pathol Clin Res 2015; 1:225-38; PMID:27499907; http://dx.doi.org/10.1002/cjp2.25
- Snyder A, Makarov V, Merghoub T, Yuan J, Zaretsky JM, Desrichard A, Walsh LA, Postow MA, Wong P, Ho TS et al. Genetic basis for clinical response to CTLA-4 blockade in melanoma. N Engl J Med 2014; 371:2189-99; PMID:25409260; http://dx.doi.org/10.1056/NEJMoa1406498
- Van Allen EM, Miao D, Schilling B, Shukla SA, Blank C, Zimmer L, Sucker A, Hillen U, Geukes Foppen MH, Goldinger SM et al. Genomic correlates of response to CTLA-4 blockade in metastatic melanoma. Science 2015; 350:207-1; PMID:26359337; http://dx.doi.org/10.1126/science.aad0095
- McGranahan N, Furness AJ, Rosenthal R, Ramskov S, Lyngaa R, Saini SK, Jamal-Hanjani M, Wilson GA, Birkbak NJ, Hiley CT et al. Clonal neoantigens elicit T cell immunoreactivity and sensitivity to immune checkpoint blockade. Science 2016; 351:1463-9; PMID:26940869; http://dx.doi.org/10.1126/science.aaf1490
- Le DT, Uram JN, Wang H, Bartlett BR, Kemberling H, Eyring AD, Skora AD, Luber BS, Azad NS, Laheru D et al. PD-1 Blockade in tumors with mismatch-repair deficiency. N Engl J Med 2015; 372:2509-20; PMID:26028255; http://dx.doi.org/10.1056/NEJMoa1500596
- Rizvi NA, Hellmann MD, Snyder A, Kvistborg P, Makarov V, Havel JJ, Lee W, Yuan J, Wong P, Ho TS et al. Cancer immunology. Mutational landscape determines sensitivity to PD-1 blockade in non-small cell lung cancer. Science 2015; 348:124-8; PMID:25765070; http://dx.doi.org/10.1126/science.aaa1348
- Nghiem PT, Bhatia S, Lipson EJ, Kudchadkar RR, Miller NJ, Annamalai L, Berry S, Chartash EK, Daud A, Fling SP et al. PD-1 blockade with pembrolizumab in advanced merkel-cell carcinoma. N Engl J Med 2016; 374:2542-52; PMID:27093365; http://dx.doi.org/10.1056/NEJMoa1603702
- Savas P, Salgado R, Denkert C, Sotiriou C, Darcy PK, Smyth MJ, Loi S. Clinical relevance of host immunity in breast cancer: from TILs to the clinic. Nat Rev Clin Oncol 2016; 13:228-41; PMID:26667975; http://dx.doi.org/10.1038/nrclinonc.2015.215
- Fridman WH, Galon J, Pagès F, Tartour E, Sautès-Fridman C, Kroemer G. Prognostic and predictive impact of intra- and peritumoral immune infiltrates. Cancer Res 2011; 71:5601-5; PMID:21846822; http://dx.doi.org/10.1158/0008-5472.CAN-11-1316
- Galon J, Pagès F, Marincola FM, Angell HK, Thurin M, Lugli A, Zlobec I, Berger A, Bifulco C, Botti G et al. Cancer classification using the Immunoscore: a worldwide task force. J Transl Med 2012; 10:205; PMID:23034130; http://dx.doi.org/10.1186/1479-5876-10-205
- Wargo JA, Reddy SM, Reuben A, Sharma P. Monitoring immune responses in the tumor microenvironment. Curr Opin Immunol 2016; 41:23-31; PMID:27240055; http://dx.doi.org/10.1016/j.coi.2016.05.006
- Burgess M, Tawbi H. Immunotherapeutic approaches to sarcoma. Curr Treat Options Oncol 2015; 16:26; PMID:25975445; http://dx.doi.org/10.1007/s11864-015-0345-5
- Burgess M, Gorantla V, Weiss K, Tawbi H. Immunotherapy in sarcoma: future horizons. Curr Oncol Rep 2015; 17:52; PMID:26423769; http://dx.doi.org/10.1007/s11912-015-0476-7
- Lee A, Huang P, DeMatteo RP, Pollack SM. Immunotherapy for soft tissue sarcoma: tomorrow is only a day away. Am Soc Clin Oncol Educ Book 2016; 35:281-90; PMID:27249707; http://dx.doi.org/10.14694/EDBK_157439