ABSTRACT
The expression “immunogenic cell death” (ICD) refers to a functionally unique form of cell death that facilitates (instead of suppressing) a T cell-dependent immune response specific for dead cell-derived antigens. ICD critically relies on the activation of adaptive responses in dying cells, culminating with the exposure or secretion of immunostimulatory molecules commonly referred to as “damage-associated molecular patterns”. Only a few agents can elicit bona fide ICD, including some clinically established chemotherapeutics such as doxorubicin, epirubicin, idarubicin, mitoxantrone, bleomycin, bortezomib, cyclophosphamide and oxaliplatin. In this Trial Watch, we discuss recent progress on the development of ICD-inducing chemotherapeutic regimens, focusing on studies that evaluate clinical efficacy in conjunction with immunological biomarkers.
Introduction
The possibility that cancer cells might undergo an immunogenic form of regulated cell death (RCD) in response to selected stimuli has been proposed for the first time more than 10 years ago.Citation1 Since then, considerable efforts have been dedicated to the elucidation of the molecular and cellular mechanisms underlying immunogenic cell death (ICD), defined as a functionally distinct form of regulated cell death that facilitates (instead of suppressing) an adaptive immune response specific for dead cell-derived antigens.Citation1–7 Based on the available data, it is clear that ICD can facilitate T cell responses against a wide-spectrum of differentiation, over-expressed, and mutated tumor-associated antigens (TAAs).Citation1–4,8–13 However, the predominance of a fraction of TAA-specific T cells in driving ICD-based immunity might be regulated by: (1) the spatiotemporal expression patterns of specific TAAs within a tumor,Citation14–20 (2) the overall coverage of various TAAs by central or peripheral tolerance,Citation21–23 (3) the overall avidity of the T cell receptor (TCR) for specific TAA,Citation24–32 and (4) the general cellular and metabolic health of effector or memory T cell fractions.Citation33–38 Operationally, two experimental procedures have been established over the years to identify bona fide ICD inducers in vivo. First, bona fide ICD inducers display elevated efficacy against malignant cells growing in immunocompetent hosts, but are largely ineffective when the same tumors are established in immunocompromised animals.Citation39–43 Second, cancer cells succumbing to bona fide ICD in vitro are able to vaccinate syngeneic immunocompetent hosts against a subsequent challenge with living cancer cells of the same type.Citation1,3,39,44 Although the former approach (therapeutic setting) is rather convenient, it is intrinsically unable to discriminate between bona fide ICD inducers and molecules that exert other on-target immunostimulatory effects or drive off-target immunostimulation.Citation45–47 Thus, the only gold-standard approach to identify immunogenic instances of cell death relies on the latter approach (vaccination setting).Citation39 Since vaccination tests can only be performed with murine cancer cells and immunocompetent syngeneic hosts, however, surrogate approaches have been developed.Citation3,39,48 On the one side, surrogate biomarkers of ICD can be measured in (human and murine) cancer cells responding to putative ICD inducers.Citation2–4,48–51 On the other side, malignant cells succumbing to a putative ICD inducer can be fed to dendritic cells (DCs),Citation2,44,52–55 followed by (1) phagocytosis assaysCitation56–62; (2) assessment of activation markers on the DC surface (e.g., CD80, CD86, MHC Class II) and functional markers in conditioned media (e.g., interleukin-6 or IL6, IL1β, IL12p70)Citation54,63–72; or (3) functional cross-priming assays with syngeneic lymphocytes.Citation73–81 Although none of these assays (alone or in combination) can reliably predict the ability of a specific intervention to cause ICD, the use of surrogate approaches is highly convenient for screening purposes or when studies are focused on the human model.Citation2,3,44,48,82
A number of mechanisms regulate the capacity of a particular agent to drive bona fide ICD and the ability of the host to perceive such an instance of cell death as immunogenic, and hence respond with potentially curative TAA-specific adaptive immunity.Citation3 At the level of cancer cells, ICD depends upon the timely emission of a constellation of immunomodulatory damage-associated molecular patterns (DAMPs).Citation40,83 In the case of chemotherapy-induced ICD, these include (but may not be limited to): (1) surface-exposed endoplasmic reticulum (ER) chaperones including calreticulin (CALR)Citation84–86; (2) extracellular ATP;Citation87–91 (3) extracellular high mobility group box 1 (HMGB1)Citation13,92; (4) extracellular annexin A1 (ANXA1)Citation55; (5) secreted type I interferon;Citation93–96 and (6) extracellular nucleic acids.Citation97 That said, ICD triggered by stimuli other than chemotherapy (e.g., radiation therapy, photodynamic therapy) is not necessarily associated with the same DAMPs.Citation3,40,98,99 Moreover, new DAMPs underlying the immunogenicity of specific instances of RCD are continuously being uncovered (see below).
When emitted in a proper spatiotemporal pattern, in combination with TAAs, and in the context of a tumor milieu amenable to immune intervention,Citation83,100–104 these DAMPs can efficiently recruit antigen-presenting cells including DCs to the tumor bed, facilitate the engulfment of dying cells or their corpses (along with their TAA-laden cargo) in the context of immunostimulatory signals (which promote DC maturation).Citation13,41,44,93,99 Mature DCs acquire therefore the ability to cross-present processed TAAs to CD4+ and CD8+ T cells along with suitable co-stimulation, thereby resulting in engagement of TAA-specific immunity.Citation105–110 Accordingly, RCD can no longer be perceived as immunogenic when: (1) the intracellular stress responses regulating the emission of ICD-associated DAMPs are pharmacologically or genetically ablated in cancer cells; or (2) when the molecular machinery dedicated to DAMP detection is inhibited or ablated.Citation13,44,84,91,93,97 Moreover, ICD-driven immunity can no longer operate in the presence of general immunological defects,Citation111 such as (1) an intrinsically low antigenicity of cancer cells, owing to low levels of TAAs or downregulation of MHC Class I moleculesCitation112–119; (2) an increased immunological tolerance of the host, secondary to increased amounts of immunosuppressive cytokines,Citation120–128 or inhibitors of chemotaxis,Citation129–134 increased tumor infiltration by immunosuppressive cell populations,Citation135–145 or robust immune checkpoint activation;Citation126,130,136–138,146 (3) a reduced persistence of TAA-specific CD8+ memory T cells due to peripheral tolerance;Citation21,23,144,147–149 and (4) an intrinsically elevated resistance of cancer cells to lysis by immune effectors.Citation136,147,150,151 Additional details about ICD-associated signaling pathways and resistance mechanisms can be found in various publications from us and others.Citation2–4,7,40,152–155
Of note, only a limited number of cell death inducers can elicit bona fide ICD, and this capacity cannot be predicted on the basis of structural or functional similarities. Thus, while cisplatin and oxaliplatin both induce RCD at least in part by forming inter- and intra-strand DNA adducts,Citation156 only the latter induces ICD.Citation157 Similarly, even though both melphalan and cyclophosphamide efficiently kill cancer cells by operating as DNA alkylating agents, only the latter drives ICD.Citation158 In both examples, the ability of a specific agent (e.g., oxaliplatin, cyclophosphamide) but not one of its alike (e.g., cisplatin, melphalan) to drive ICD can be explained by the differential activation of ER stress (and hence differential exposure of CALR in the course of RCD).Citation100,157–159 Well established ICD inducers include commonly employed anticancer chemotherapeutics such as: (1) doxorubicin, an anthracycline approved by the US Food and Drug Administration (FDA) for treating acute myeloid leukemia (AML), acute lymphoblastic leukemia (ALL), small cell lung carcinoma, breast carcinoma, neuroblastoma, lymphoma, thyroid carcinoma, soft tissue and bone sarcomas, multiple myeloma (MM), gastric cancer, ovarian carcinoma, transitional cell bladder carcinoma and Wilms tumor;Citation1,84,160–166 (2) epirubicin, an anthracycline licensed for use in breast carcinoma patients;Citation1,84,167,168 (3) idarubicin, an anthracycline generally employed against AML;Citation84,167–169 (4) mitoxantrone, an anthracenedione approved for the treatment of prostate carcinoma, AML, non-Hodgkin's lymphoma (NHL) and breast carcinoma;Citation1,84,167,168 (5) bleomycin, a glycopeptide antibiotic approved for the treatment of patients with NHL, testicular cancer, Hodgkin's lymphoma, penile cancer and squamous carcinomas of the cervix, head and neck or vulva;Citation167,168,170 (6) bortezomib, a proteasomal inhibitor approved for the therapy MM and mantle cell lymphoma (MCL);Citation171–181 (7) cyclophosphamide, a DNA-alkylating agent approved for use in patients with chronic myeloid leukemia (CML), AML, ALL, chronic lymphocytic leukemia, MM, ovarian carcinoma, breast carcinoma, mycosis fungoides, lymphoma, neuroblastoma, and retinoblastoma;Citation177,182–191 and (8) oxaliplatin, a platinum-derivative licensed for the therapy of advanced colorectal carcinoma in combination with 5-fluorouracil and folinic acid.Citation156,157,192–198 Moreover, there is some evidence that microtubule-targeting agents including taxanes and vinca alkaloids (which are commonly used for the treatment of multiple carcinomas) can stimulate ICD.Citation41,199
Along the lines of our Trial Watch series, here we discuss recent preclinical and clinical advances in the development of ICD-inducing chemotherapeutic regimens.Citation200 Several other interventions that trigger bona fide ICD, such as radiation therapy administered according to specific regimens,Citation94,201–203 high hydrostatic pressure,Citation3,4 oncolytic virotherapyCitation204–208 and photodynamic therapy,Citation44,86,98,99 are not discussed here in further detail.
Recent preclinical developments
A high amount of preclinical and/or translational studies on chemotherapy-induced ICD has been published since the latest Trial Watch dealing with this topic (April 2015).Citation50 Of such an abundant scientific production from us and others, we found of particular significance the following works.
We documented that anthracycline-induced ICD critically relies on the release of ANXA1 by cancer cells, driving the late-stage phases of DC chemotaxis in a formyl peptide receptor 1 (FPR1)-dependent manner.Citation55 Accordingly, a loss-of-function single-nucleotide polymorphism in FPR1 was found to be associated with poor prognosis in cohorts of breast and colorectal carcinoma patients undergoing anthracycline or oxaliplatin-based chemotherapy.Citation55 Moreover, we found that combining chemotherapy-induced ICD with short-term fasting or caloric restriction mimetics (CRMs)Citation90 like hydroxycitrate or spermidine, boosts the efficacy of mitoxantrone and oxaliplatin in mice, a therapeutic improvement that is accompanied by decreased tumor infiltration by immunosuppressive CD4+CD25+FOXP3+ regulatory T (TREG) cells.Citation209 Along similar lines, Di Biase et al. (from University of Southern California, Los Angeles, CA, USA) observed that the combination of doxorubicin with a fasting-mimicking diet strongly delays breast cancer and melanoma progression as it increases the amounts of tumor-infiltrating cytotoxic T lymphocytes (CTLs) while concomitantly decreasing the expression levels of the immunosuppressive enzyme heme oxygenase-1 (HMOX1; also known as HO1).Citation210 We also discovered that cancer cells undergoing mitoxantrone-induced ICD trigger a pathogen response-like chemokine (PARC) signature characterized by the co-release of C-X-C motif chemokine ligand 1 (CXCL1), C-C motif chemokine ligand 2 (CCL2) and C-X-C motif chemokine ligand 10 (CXCL10) (or homologues thereof), in thus far mimicking bacteria or virus infected cells.Citation97 Such a chemokine mixture is particularly efficient at recruiting neutrophils towards the dying cells (a process that appears to be evolutionarily conserved), paving the way to the CALR-dependent phagocytosis of dying cancer cells or corpses thereof, and the cytotoxic targeting of residual malignant cells.Citation97 We characterized a naturally-occurring preclinical model of cancer that exhibits intrinsic resistance against mitoxantrone-induced ICD in vivo secondary to a defect in CALR exposure,Citation199 and we documented that anthracyclines and oxaliplatin can trigger a necroptotic variant of ICDCitation211–215 in cancer cells expressing receptor interacting serine/threonine kinase 3 (RIP3K) and the pseudokinase mixed lineage kinase domain-like (MLKL).Citation215 Finally, we found that an engineered oncolytic vaccinia virusCitation216 can induce ICD-dependent antitumor immunity, which can be further potentiated by the co-administration of ICD-inducing chemotherapy or immune checkpoint blockers (ICBs),Citation216 and that pharmacological inhibition of signal transducer and activator of transcription 3 (STAT3)Citation217 signaling boosts the therapeutic efficacy of anthracyclines upon increased type I interferon (IFN) secretion.Citation217
Pfirschke and co-authors (from Massachusetts General Hospital Research Institute and Harvard Medical School, Boston, MA, USA) found that autochthonous tumors lacking tumor-infiltrating lymphocytes (TILs) can be sensitized to immunological rejection via a Toll-like receptor 4 (TLR4)-dependent mechanism when suitable ICD inducers like oxaliplatin or cyclophosphamide are combined with ICBs targeting programmed cell death 1 (PDCD1; best known as PD-1)Citation218 and/or cytotoxic T-lymphocyte associated protein 4 (CTLA4).Citation218 Similarly, Stewart and colleagues (from MedImmune Ltd, Cambridge, United Kingdom) characterized a novel antibody directed against CD274 (best known as PD-L1) (MEDI4736), specifically engineered to prevent antibody-dependent cell-mediated cytotoxicity (ADCC),Citation219 which exhibits potent antitumor activity when combined with oxaliplatin (only in immunocompetent mice).Citation219 In a multi-modal combinatorial study, Rios-Doria and collaborators (from MedImmune, Gaithersburg, MD, USA) documented that liposomal doxorubicin induces potent ICD-associated innate and adaptive immune responses, in vivo, and effectively controls tumor growth, especially in combination with ICBs against CTLA4, PD-1, or its main ligand PD-L1 or with immunostimulatory fusion proteins targeting TNF receptor superfamily, member 4 (TNFRSF4; best known as OX40) or TNF receptor superfamily member 18 (TNFRSF18; best known as GITR).Citation220 In a slightly different approach, Evans et al. (from Vaccinex, Inc., Rochester, NY, USA) reported that semaphorin 4D (SEMA4D) expression at the tumor invasive margin facilitates pro-tumorigenic inflammation, and that combining anti-SEMA4D monoclonal antibodies (mAbs) with ICBs or cyclophosphamide promotes immunological rejection in murine colorectal carcinoma models.Citation221 Finally, Blake et al. (from QIMR Berghofer Medical Research Institute, Herston, Australia) observed that inhibiting CD96 – a negative regulator of natural killer (NK) cell activityCitation222 – with ICBs or doxorubicin exerts superior antimetastatic effects in the lung.Citation223
Musahl et al. (from Max Planck Institute for Molecular Genetics, Berlin, Germany) observed that the long non-coding RNA, ncRNA-RB1, positively regulates overall CALR expression levels so that, following anthracyclines-induced ICD, ncRNA-RB1 supports surface-exposed CALR driven phagocytosis.Citation224 In a similar fashion, Colangelo and co-authors (from University of Sannio, Benevento, Italy) found that the microRNA miR-27a specifically suppresses the exposure of CALR by mitoxantrone and oxaliplatin in colorectal cancer cells by negatively affecting the intracellular CALR trafficking pathway.Citation225 In a study exploring novel combinatorial regimens, Lu and colleagues (from University of Alabama, Birmingham, AL, USA) documented that combining an inhibitor of thrombospondin 1 (THBS1; also known as TSP1) and transforming growth factor beta 1 (TFGB1) signaling, i.e., SRI31277, with bortezomib mediates superior antineoplastic effects as compared to either agents alone.Citation226 In a different combinatorial approach, Hsu and colleagues (from National Yang-Ming University, Taipei, Taiwan) revealed that pre-conditioning with low-dose doxorubicin or paclitaxel before adoptive cell transfer (ACT) significantly improves antitumor immunity upon inhibition of NF-kB-regulated immunosuppressive factors.Citation227 Similarly, Koo and collaborators (from Catholic University of Korea, Bucheon, Republic of Korea) found that X-shaped double-stranded oligodeoxynucleotide molecules (so called “X-DNA”) that bind TLR9228 greatly enhance the antitumor efficacy of doxorubicin against colitis-associated colorectal carcinoma, via a mechanism that depends on DCs and T cells.Citation229 Finally, Monk et al. (from University of Arizona College of Medicine-Phoenix, Phoenix, AZ, USA) documented that combining pegylated liposomal doxorubicin with a TLR8 agonist, i.e., motolimod, retards the growth of patient-derived ovarian carcinoma cells implanted in humanized mice.Citation230
In a study exploring resistance mechanisms, Buondonno et al. (from University of Torino, Torino, Italy) documented that treating osteosarcoma cells that overexpress ATP binding cassette subfamily B member 1 (ABCB1) – a plasma membrane transporter that actively extrudes several chemotherapeutics including doxorubicin – with mitochondria-targeted doxorubicin overcomes chemoresistance to restore ICD and associated immune responses in vivo.Citation231 Finally, Shalapour and co-authors (from University of California San Diego, San Diego, CA, USA) reported that B cells producing class A immunoglobulins (IgAs), IL10 and PD-L1 in a TGFB1-dependent fashion act as immunosuppressive plasmocytes and prevent oxaliplatin-driven tumor rejection in different murine models of prostate cancer.Citation232 Thounaojam and colleagues (from Meharry Medical College, Nashville, TN, USA) found that bortezomib suppresses the growth of various solid tumors by upregulating the expression of various components of the Notch signaling pathway in lymphoid tissues including CD8+ CTLs, resulting in increased cytotoxic functions.Citation219,233 Guillerey and collaborators (from QIMR Berghofer Medical Research Institute, Herston, Australia) observed that anti-myeloma immune response elicited by bortezomib or cyclophosphamide critically relies on CD226, which is crucial for the effector and cytotoxic functions of NK and CD8+ T cells.Citation234 Finally, Wong and collaborators (from National University of Singapore, Singapore) screened a library of chemotherapeutically active platinum derivatives and characterized a Pt(II) N-heterocyclic carbene complex as a putative inducer of ICD (pending in vivo validation), based on its capacity to trigger oxidative ER stress, CALR exposure, ATP secretion, and HMGB1 release.Citation235
Taken together, these findings exemplify the attention currently focused around the molecular and cellular mechanisms through which the death of cancer cells responding to some (but not all) chemotherapeutics initiates a therapeutically relevant tumor-specific immune response.
Completed clinical trials
Since the publication of the latest Trial Watch dealing with this topic (April 2015), multiple peer-reviewed articles documented the outcome of clinical trials evaluating the efficacy of bona fide ICD-inducing chemotherapeutics (i.e., doxorubicin, epirubicin, idarubicin, mitoxantrone, bortezomib, bleomycin, cyclophosphamide or oxaliplatin) along with ICD-associated immunological biomarkers.Citation236,237 These publications were acquired from PubMed (http://www.ncbi.nlm.nih.gov/pubmed), and the initial list was manually curated to ensure relevance for this Trial Watch.
Several clinical studies demonstrated the beneficial immunostimulatory effects of ICD induction by chemotherapy. Loi et al. (from Peter MacCallum Cancer Centre, Melbourne, Australia) documented that increased tumor infiltration by T cells is associated with improved prognosis in patients affected by triple-negative breast cancer (TNBC) with residual disease following neoadjuvant chemotherapy (consisting of doxorubicin, cyclophosphamide, and in some cases paclitaxel).Citation238 Cornelissen and collaborators (from Erasmus MC Cancer Institute, Rotterdam, Netherlands) reported that combining DC-based vaccines with metronomic cyclophosphamide (which efficiently reduces circulating TREG cells) resulted in radiographic tumor control (and increased overall survival) in 8 out of 10 patients with malignant pleural mesothelioma patients.Citation239 Schijns and colleagues (from Wageningen University, Wageningen, Netherlands) found that individuals with recurrent glioblastoma multiforme (GBM) receiving a vaccine composed of autologous antigens in combination with cyclophosphamide, experienced improved overall survival.Citation240 Klein and co-authors (from Ludwig Institute for Cancer Research, Heidelberg, Australia) reported that cyclophosphamide promoted TAA-specific CD4+ T cell responses driven by a peptide-based vaccine targeting cancer/testis antigen 1B (CTAG1B; best known as NY-ESO-1)Citation241 in patients with advanced melanoma, in the absence of TREG cell depletion.Citation238 Murahashi et al. (from Kyushu University, Fukuoka, Japan) evaluated a multipeptide-based vaccine combined with escalating doses of cyclophosphamide in patients with locally advanced, metastatic and/or recurrent gastrointestinal, lung or cervical cancer, achieving increased overall survival accompanied by increased TAA-specific T cells and peripheral TREG cell depletion.Citation242 Tanis and collaborators (from The Netherlands Cancer Institute, Amsterdam, Netherlands) found that patients with liver metastases from colorectal carcinoma obtain a survival benefit from the FOLFOX therapeutic regimen (consisting of folinic acid, fluorouracil and oxaliplatin), which is accompanied by increased amount of CTLs at invasive tumor margin, as well as with mast cell infiltration.Citation243 Collectively, these reports demonstrate that ICD-inducing chemotherapeutic regimens can prolong the survival of some cancer patients, and this often correlates with biomarkers of ongoing anticancer immunity.
Alongside, a few articles documented negative immunological consequences of chemotherapeutic regimens (at least potentially) triggering ICD, such as the R-CHOP regimen (consisting of rituximab, cyclophosphamide, doxorubicin, vincristine and prednisone). Specifically, Ito et al. (from Fujita Health University Hospital, Aichi, Japan) found that diffuse large B-cell lymphoma (DLBCL) patients receiving R-CHOP experienced immunosuppression for two or more years following treatment, mainly manifesting with marked decrease in circulating CD20+ B cells and CD4+ T cells.Citation244 Brown and collaborators (from University of Oxford, John Radcliffe Hospital, Oxford, UK) reported that patients with DLBCL receiving R-CHOP exhibited decreased levels of major histocompatibility complex, class II, DR alpha (HLA-DRA) on DLBCL cells, correlating with inferior overall survival.Citation245 On a relatively conciliatory note though, Wu and co-authors (from The Second Hospital of Lanzhou University, Lanzhou, China) found that the low circulating levels of monocytes and monocytic myeloid-derived suppressor cells (MDSCs) have profound positive prognostic impact on the overall survival of DLBCL patients treated with R-CHOP.Citation246 It is tempting to speculate, yet remains to be formally investigated, that the immunosuppressive effects of R-CHOP originate from prednisone.Citation247 It would therefore be interesting to see whether an “R-CHO” regimen deprived of prednisone might exert superior immunostimulatory and therapeutic effects.
Ongoing clinical trials
Official sources list no less than 58 clinical trials evaluating the clinical efficacy of bona fide ICD-inducing chemotherapeutic regimens in conjunction with relevant immune biomarkers initiated after April 2015 (since the publication of the latest Trial Watch dealing with this topic) (, and ). These studies were retrieved from the ClinicalTrials.gov database (http://www.clinicaltrials.gov/), and the initial list was manually curated to ensure relevance for this Trial Watch.
Figure 1. Current clinical trials testing immunogenic cell death (ICD)-inducing chemotherapies in oncological indications. A. Distribution by immunological biomarker (biomarkers directly relevant for ICD are in bold). B. Distribution by main chemotherapeutic agent. C. Distribution by oncological indication. D. Number of clinical trials currently testing ICD-inducing chemotherapeutic regimens in combination with immunotherapy. CIK, cytokine-induced killer; CSF1, colony stimulating factor 1; DC, dendritic cell; mAb, monoclonal antibody; MDSC, myeloid-derived suppressor cell; NK, natural killer; TAA, tumor-associated antigen; TLR, Toll-like receptor; TREG, regulatory T.
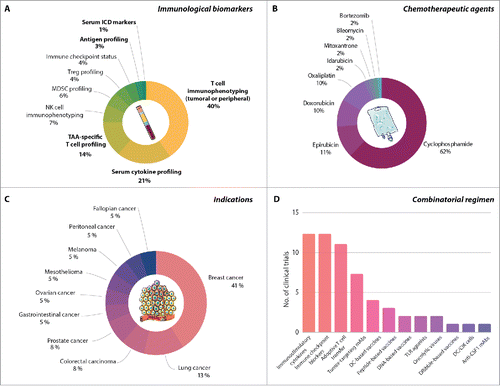
Table 1. Current clinical trials evaluating the therapeutic and immunological profile of ICD-inducing chemotherapeutic regimens.Footnote*
Table 2. Current clinical trials evaluating the therapeutic and immunological profile of cyclophosphamide-based chemotherapy.Footnote*
Our survey revealed that multiple ICD-relevant biomarkers are being investigated as immunological outcomes across recently initiated clinical trials based on ICD-inducing chemotherapeutic regimens (). These immunological biomarkers include: (1) parameters of broad T cell immunophenotyping, such as the so-called “Immunoscore”,Citation248–250 and the abundance of tumor-infiltrating or circulating CD3+, CD4+ or CD8+ T cells, amongst others;Citation251–253 (2) the circulating levels of multiple cytokines, such as interferon, gamma (IFNG; best known as IFN-γ),Citation254 IL6,Citation255 and tumor necrosis factor (TNF);Citation256–258 (3) the amounts of TAA-specific T cells (via tetramer assays);Citation259,260 (4) the tumor mutational load and/or the abundance of predicted neo-antigens (via genome or whole-exome sequencing);Citation115,138,261–265 and (5) the levels of circulating biomarkers of ICD (via proteomic or metabolomic assays).Citation49,266 Alongside, several immunological biomarkers with indirect relevance for ICD and its therapeutic outcomes are also being tested, including the abundance of circulating or tumor-infiltrating NK cells, MDSCs and TREG cells, as well as the expression levels and activation status of immune checkpoints.Citation48,261,267–273 In this setting, the profiling of TREG cells is being performed in most cases to estimate the ability of cyclophosphamide to specifically target these immunosuppressive cells.Citation274–276 It will be interesting to see whether the peripheral profile (in terms of abundance and activation status) of CD4+ and/or CD8+ T cells can serve as reliable biomarker for the immunological consequences of chemotherapy.Citation4,277 Preclinical and (mostly retrospective) clinical data advocate that tumor-infiltrating T cells provide superior prognostic and/or predictive information,Citation251,267,278 but the identification of a reliable circulating biomarker would be a major asset for immunomonitoring.
A majority of the clinical trials surveyed here aim at testing cyclophosphamide, epirubicin, doxorubicin or oxaliplatin, typically as on-label therapeutic interventions and often as part of gold standard chemotherapeutic regimen (, and ). In some cases, the ICD-inducing potential of the chemotherapeutic regimen of choice was cited as part of the rational to the study. However, we were unable to identify any clinical trial specifically comparing the ICD-inducing potential of these agents with a relevant non-immunogenic chemotherapeutic. In a majority of cases, cyclophosphamide is administered with the primary aim of inhibiting or depleting (systemic) TREG cells. However, considering the wide array of immunological biomarkers monitored in these clinical trials (), the ICD-inducing potential of cyclophosphamideCitation191 may also become apparent.
As for oncological indication, the studies we retrieved are currently enrolling patients with breast carcinoma (16 trials), lung cancer (in particular non-small cell lung carcinoma, NSCLC) (5 trials), colorectal carcinoma (3 trials), prostate cancer (3 trials), and other cancer types (31 trials) (, and ). In general, solid tumors (51 trials) are preferred to hematological malignancies (8 trials). Possibly, this reflects, (1) the high sensitivity of several hematological tumors to standard-of-care treatments, and/or (2) the fact that hematological malignancies often stem from components of the immune system, resulting in compromised immune functions and limited susceptibility to multiple forms of immunotherapy and immunogenic chemotherapy.Citation279,280 In multiple clinical studies, bona fide ICD-inducing chemotherapeutic regimens are combined with agents that elicit ICD per se, such as radiation therapy (2 trials), or considerably boost the immunogenicity of cancer cells, such as taxanes or zoledronic acid (10 trials). Finally, in a limited amount of trials, ICD inducers are combined with targeted anticancer agents, including the inhibitor of JAK kinases ruxolitinib (1 trial) and the inhibitor of mechanistic target of rapamycin kinase (MTOR) rapamycinCitation281,282 (also known as sirolimus, or its derivative everolimus) (2 trials) ( and ).
Fitting well within the current oncology landscape, a majority of clinical trials surveyed for this Trial Watch combine ICD-inducing chemotherapeutic regimens with bona fide immunotherapies (, and ). These include: (1) immunostimulatory cytokines such as granulocyte-macrophage colony stimulating factor (GM-CSF), IL2, IFN-α and IRX-2, a cell-free mixture of cytokines encompassing IL1, IL2, IL6, IL8, IL10, IL12, TNF, IFN-γ and colony stimulating factor 1 (CSF1, also called macrophage colony stimulating factor 1 or M-CSF); (2) ICBs such as the PD-1-targeting agents pembrolizumabCitation283 and SHR-1210;Citation284 and the PD-L1-targeting agents avelumab and MEDI4736;284 (3) the inhibitors of indoleamine 2,3-dioxygenase 1 (IDO1);Citation138,285 (4) adoptively transferred T cells, often CD19-specific chimeric antigen receptor (CAR)-expressing T cells or PD-1-deficient T cells;Citation286 (5) tumor-targeting mAbs, such as the CD20-specific agent rituximab,Citation287 the vascular endothelial growth factor (VEGF)-targeting mAb bevacizumab,Citation288 and various molecules targeting HER2;Citation289 (6) anticancer vaccines;Citation52,53,290,291 and (7) oncolytic virusesCitation266,292–295 (, and ).
Finally, most of the clinical trials we surveyed are ongoing (i.e., either actively recruiting or initiated/registered but not yet recruiting), with a few notable exceptions. NCT03050814 (a Phase 2 study testing oxaliplatin-based chemo(immuno)therapy in combination with PD-L1 blockade or bevacizumab in colorectal carcinoma patients) has been suspended pending further discussion with the US FDA, because an unspecified number of individuals died within 30 days of treatment. On a different note, NCT02419170 (a Phase I study testing DC-based vaccination plus cyclophosphamide in NSCLC patients) has been withdrawn prior to enrollment because the investigator manufacturing the vaccines left the concerned institution. Finally, NCT02461121 (a Phase III trial comparing cyclophosphamide-containing versus cyclophosphamide-free preconditioning in patients with acute myeloid leukemia allocated to transplantation) and NCT02655458 (a Phase I study involving cyclophosphamide-containing preconditioning in multiple myeloma patients) have been completed. However, to the best of our knowledge, the results of these studies have not been released yet.
Concluding remarks
A number of ICD-inducing chemotherapeutic regimens are currently approved by the US FDA or equivalent regulatory agencies worldwide for use in cancer patients. However, the widespread use of these treatments has been implemented mostly on empirical (rather than immunological) grounds.Citation200,296–300 Indeed, the possibility that chemotherapy and other forms of treatment (including radiotherapy and photodynamic therapy) might promote an immunogenic form of cancer cell death has been overlooked for several decades.Citation301–303 Thus, current anticancer drugs have been developed in immunodeficient preclinical models and in clinical trials devoid of any form of immunomonitoring, mainly most often aimed at identifying maximum tolerated doses (MTDs).Citation298,304 Nonetheless, a majority of currently available anticancer agents mediate on-target or off-target immunostimulatory effects, which strongly argues against an irrelevant role for the immune system in the therapeutic effects of these treatments.Citation305–308 Moreover, in various cases, chemotherapeutics applied through multiple treatment cycles may negatively affect the immune system, by causing lymphopenia or leucopenia, thereby further compromising antitumor immune responses.Citation44,53,54 One of the major challenges for the future will be to identify doses and administration schedules that mediate maximal immunostimulatory effects.Citation297,309,310 Accumulating evidence suggest indeed that metronomic chemotherapy and hypofractionated radiation (rather than chemotherapy at the MTD and high single-dose radiation) exerts superior immunostimulatory (and hence therapeutic, at least in some settings) effects.Citation311–314 Alongside, it will be important to devise highly efficient combinatorial regimens that harness not only the ability of some treatments to drive ICD, but also the off-target immunostimulatory effects of a variety of agents. We are convinced that conventional therapeutic regimens – if properly employed – are a very powerful and relatively economical tool to drive clinically relevant anticancer immune responses.
Abbreviations
ACT | = | adoptive cell transfer |
DAMP | = | damage-associated molecular pattern |
DC | = | dendritic cell |
ER | = | endoplasmic reticulum |
FDA | = | Food and Drug Administration |
GM-CSF | = | granulocyte-macrophage colony-stimulating factor |
ICB | = | immune checkpoint blocker |
ICD | = | immunogenic cell death |
IFN | = | interferon |
IL | = | interleukin |
mAb | = | monoclonal antibody |
MTD | = | maximum tolerated dose |
NSCLC | = | non-small cell lung carcinoma |
RCD | = | regulated cell death |
TAA | = | tumor-associated antigen |
TIL | = | tumor-infiltrating lymphocyte |
TLR | = | Toll-like receptor |
Acknowledgments
ADG is a recipient of FWO Postdoctoral (Renewal) Fellowship from FWO-Vlaanderen, Belgium and the POR award funds from KU Leuven. NR, SM, MLS are funded by European Union's Horizon 2020 research and innovation programme under the Marie Sklodowska-Curie grant (Agreement no. 642295 for NR and 675448 for SM/MLS). PA is supported by grants from FWO (G060713 N, G076617 N) and KU Leuven (C16/15/073). GK is supported by Ligue contre le Cancer (équipe labelisée); Agence National de la Recherche (ANR); Association pour la recherche sur le cancer (ARC); Cancéropôle Ile-de-France; AXA Chair for Longevity Research; Institut National du Cancer (INCa); Fondation Bettencourt-Schueller; Fondation de France; Fondation pour la Recherche Médicale (FRM); the European Commission (ArtForce); the European Research Council (ERC); the LabEx Immuno-Oncology; the SIRIC Stratified Oncology Cell DNA Repair and Tumor Immune Elimination (SOCRATE); the SIRIC Cancer Research and Personalized Medicine (CARPEM); and the Paris Alliance of Cancer Research Institutes (PACRI). LG is supported by WCMC (intramural funds) and Sotio a.c. (Prague, Czech Republic).
References
- Casares N, Pequignot MO, Tesniere A, Ghiringhelli F, Roux S, Chaput N, Schmitt E, Hamai A, Hervas-Stubbs S, Obeid M, et al. Caspase-dependent immunogenicity of doxorubicin-induced tumor cell death. J Exp Med. 2005;202:1691–701. doi:10.1084/jem.20050915.
- Kroemer G, Galluzzi L, Kepp O, Zitvogel L. Immunogenic cell death in cancer therapy. Annu Rev Immunol. 2013;31:51–72. doi:10.1146/annurev-immunol-032712-100008.
- Galluzzi L, Buque A, Kepp O, Zitvogel L, Kroemer G. Immunogenic cell death in cancer and infectious disease. Nat Rev Immunol. 2017;17:97–111. doi:10.1038/nri.2016.107 10.1038/nri.2017.48.
- Garg AD, Galluzzi L, Apetoh L, Baert T, Birge RB, Bravo-San Pedro JM, Breckpot K, Brough D, Chaurio R, Cirone M, et al. Molecular and Translational Classifications of DAMPs in Immunogenic Cell Death. Front Immunol. 2015;6:588. doi:10.3389/fimmu.2015.00588.
- Cicchelero L, Denies S, Devriendt B, de Rooster H, Sanders NN. Can dendritic cells improve whole cancer cell vaccines based on immunogenically killed cancer cells? OncoImmunology. 2015;4:e1048413. doi:10.1080/2162402X.2015.1048413.
- Garrido G, Rabasa A, Sanchez B, Lopez MV, Blanco R, Lopez A, Hernández DR, Pérez R, Fernández LE. Induction of immunogenic apoptosis by blockade of epidermal growth factor receptor activation with a specific antibody. J Immunol (Baltimore, Md: 1950). 2011;187:4954–66. doi:10.4049/jimmunol.1003477.
- Garg AD, Dudek-Peric AM, Romano E, Agostinis P. Immunogenic cell death. Int J Dev Biol. 2015;59:131–40. doi:10.1387/ijdb.150061pa.
- Paroli M, Bellati F, Videtta M, Focaccetti C, Mancone C, Donato T, Antonilli M, Perniola G, Accapezzato D, Napoletano C, et al. Discovery of chemotherapy-associated ovarian cancer antigens by interrogating memory T cells. Int J Cancer. 2014;134:1823–34. doi:10.1002/ijc.28515.
- Palombo F, Focaccetti C, Barnaba V. Therapeutic implications of immunogenic cell death in human cancer. Front Immunol. 2014;4:503. doi:10.3389/fimmu.2013.00503.
- Gameiro SR, Jammeh ML, Wattenberg MM, Tsang KY, Ferrone S, Hodge JW. Radiation-induced immunogenic modulation of tumor enhances antigen processing and calreticulin exposure, resulting in enhanced T-cell killing. Oncotarget. 2014;5:403–16. doi:10.18632/oncotarget.1719.
- Loi S, Pommey S, Haibe-Kains B, Beavis PA, Darcy PK, Smyth MJ, Stagg J. CD73 promotes anthracycline resistance and poor prognosis in triple negative breast cancer. Proc Natl Acad Sci U S A. 2013;110:11091–6. doi:10.1073/pnas.1222251110.
- Hodge JW, Garnett CT, Farsaci B, Palena C, Tsang KY, Ferrone S, Gameiro SR. Chemotherapy-induced immunogenic modulation of tumor cells enhances killing by cytotoxic T lymphocytes and is distinct from immunogenic cell death. Int J Cancer. 2013;133:624–36. doi:10.1002/ijc.28070.
- Apetoh L, Ghiringhelli F, Tesniere A, Obeid M, Ortiz C, Criollo A, Mignot G, Maiuri MC, Ullrich E, Saulnier P, et al. Toll-like receptor 4-dependent contribution of the immune system to anticancer chemotherapy and radiotherapy. Nat Med. 2007;13:1050–9. doi:10.1038/nm1622.
- Rizvi NA, Hellmann MD, Snyder A, Kvistborg P, Makarov V, Havel JJ, Lee W, Yuan J, Wong P, Ho TS, et al. Cancer immunology. Mutational landscape determines sensitivity to PD-1 blockade in non-small cell lung cancer. Science. 2015;348:124–8. doi:10.1126/science.aaa1348.
- Zingg D, Arenas-Ramirez N, Sahin D, Rosalia RA, Antunes AT, Haeusel J, Sommer L, Boyman O. The Histone Methyltransferase Ezh2 Controls Mechanisms of Adaptive Resistance to Tumor Immunotherapy. Cell Rep. 2017;20:854–67. doi:10.1016/j.celrep.2017.07.007.
- Turajlic S, Litchfield K, Xu H, Rosenthal R, McGranahan N, Reading JL, Wong YNS, Rowan A, Kanu N, Al Bakir M, et al. Insertion-and-deletion-derived tumour-specific neoantigens and the immunogenic phenotype: a pan-cancer analysis. Lancet Oncol. 2017;18:1009–21. doi:10.1016/S1470-2045(17)30516-8.
- Parakh S, Gan HK, Parslow AC, Burvenich IJG, Burgess AW, Scott AM. Evolution of anti-HER2 therapies for cancer treatment. Cancer Treat Rev. 2017;59:1–21. doi:10.1016/j.ctrv.2017.06.005.
- O'Rourke DM, Nasrallah MP, Desai A, Melenhorst JJ, Mansfield K, Morrissette JJD, Martinez-Lage M, Brem S, Maloney E, Shen A, et al. A single dose of peripherally infused EGFRvIII-directed CAR T cells mediates antigen loss and induces adaptive resistance in patients with recurrent glioblastoma. Sci Transl Med. 2017;9:eaaa0984. doi:10.1126/scitranslmed.aaa0984.
- Jimenez-Sanchez A, Memon D, Pourpe S, Veeraraghavan H, Li Y, Vargas HA, Gill MB, Park KJ, Zivanovic O, Konner J, et al. Heterogeneous Tumor-Immune Microenvironments among Differentially Growing Metastases in an Ovarian Cancer Patient. Cell. 2017;170:927–38 e20. doi:10.1016/j.cell.2017.07.025.
- Gubin MM, Zhang X, Schuster H, Caron E, Ward JP, Noguchi T, Ivanova Y, Hundal J, Arthur CD, Krebber WJ, et al. Checkpoint blockade cancer immunotherapy targets tumour-specific mutant antigens. Nature. 2014;515:577–81. doi:10.1038/nature13988.
- Ott PA, Hu Z, Keskin DB, Shukla SA, Sun J, Bozym DJ, Zhang W, Luoma A, Giobbie-Hurder A, Peter L, et al. An immunogenic personal neoantigen vaccine for patients with melanoma. Nature. 2017;547:217–21. doi:10.1038/nature22991.
- Leonard JD, Gilmore DC, Dileepan T, Nawrocka WI, Chao JL, Schoenbach MH, Jenkins MK, Adams EJ, Savage PA. Identification of natural regulatory T cell epitopes reveals convergence on a dominant autoantigen. Immunity. 2017;47:107–17 e8. doi:10.1016/j.immuni.2017.06.015.
- Kumai T, Fan A, Harabuchi Y, Celis E. Cancer immunotherapy: moving forward with peptide T cell vaccines. Curr Opin Immunol. 2017;47:57–63. doi:10.1016/j.coi.2017.07.003.
- Nakagawa H, Mizukoshi E, Kobayashi E, Tamai T, Hamana H, Ozawa T, Kishi H, Kitahara M, Yamashita T, Arai K, et al. Association Between High-Avidity T-Cell Receptors, Induced by alpha-Fetoprotein-Derived Peptides, and Anti-Tumor Effects in Patients With Hepatocellular Carcinoma. Gastroenterology. 2017;152:1395–406.e10. doi:10.1053/j.gastro.2017.02.001.
- Segal G, Prato S, Zehn D, Mintern JD, Villadangos JA. Target Density, Not Affinity or Avidity of Antigen Recognition, Determines Adoptive T Cell Therapy Outcomes in a Mouse Lymphoma Model. J Immunol (Baltimore, Md: 1950). 2016;196:3935–42. doi:10.4049/jimmunol.1502187.
- Jaigirdar A, Rosenberg SA, Parkhurst M. A High-avidity WT1-reactive T-Cell Receptor Mediates Recognition of Peptide and Processed Antigen but not Naturally Occurring WT1-positive Tumor Cells. J Immunol (Baltimore, Md: 1950). 2016;39:105–16.
- Zhao Q, Ahmed M, Tassev DV, Hasan A, Kuo TY, Guo HF, O'Reilly RJ, Cheung NK. Affinity maturation of T-cell receptor-like antibodies for Wilms tumor 1 peptide greatly enhances therapeutic potential. Leukemia. 2015;29:2238–47. doi:10.1038/leu.2015.125.
- Tassev DV, Hasan A, Kuo TY, Guo HF, O'Reilly RJ, Cheung NK, et al. Quantitative TCR:pMHC Dissociation Rate Assessment by NTAmers Reveals Antimelanoma T Cell Repertoires Enriched for High Functional Competence. Leukemia. 2015;195:356–66.
- Palmer DC, Guittard GC, Franco Z, Crompton JG, Eil RL, Patel SJ, Ji Y, Van Panhuys N, Klebanoff CA, Sukumar M, et al. Cish actively silences TCR signaling in CD8+ T cells to maintain tumor tolerance. J Exp Med. 2015;212:2095–113. doi:10.1084/jem.20150304.
- Nakatsugawa M, Yamashita Y, Ochi T, Tanaka S, Chamoto K, Guo T, Butler MO, Hirano N. Specific roles of each TCR hemichain in generating functional chain-centric TCR. J Immunol (Baltimore, Md: 1950). 2015;194:3487–500. doi:10.4049/jimmunol.1401717.
- Hebeisen M, Schmidt J, Guillaume P, Baumgaertner P, Speiser DE, Luescher I, Rufer N. Identification of Rare High-Avidity, Tumor-Reactive CD8+ T Cells by Monomeric TCR-Ligand Off-Rates Measurements on Living Cells. Cancer Res. 2015;75:1983–91. doi:10.1158/0008-5472.CAN-14-3516.
- Oren R, Hod-Marco M, Haus-Cohen M, Thomas S, Blat D, Duvshani N, Denkberg G, Elbaz Y, Benchetrit F, Eshhar Z, et al. Functional comparison of engineered T cells carrying a native TCR versus TCR-like antibody-based chimeric antigen receptors indicates affinity/avidity thresholds. J Immunol (Baltimore, Md: 1950). 2014;193:5733–43. doi:10.4049/jimmunol.1301769.
- Chen DS, Mellman I. Elements of cancer immunity and the cancer-immune set point. Nature. 2017;541:321–30. doi:10.1038/nature21349.
- Patsoukis N, Weaver JD, Strauss L, Herbel C, Seth P, Boussiotis VA. Immunometabolic Regulations Mediated by Coinhibitory Receptors and Their Impact on T Cell Immune Responses. European J Immunol. 2017;8:330.
- Cibrian D, Sanchez-Madrid F. CD69: from activation marker to metabolic gatekeeper. European J Immunol. 2017;47:946–53. doi:10.1002/eji.201646837.
- Gerritsen B, Pandit A. The memory of a killer T cell: models of CD8(+) T cell differentiation. Immunol Cell Biol. 2016;94:236–41. doi:10.1038/icb.2015.118.
- Dogra P, Ghoneim HE, Abdelsamed HA, Youngblood B. Generating long-lived CD8(+) T-cell memory: Insights from epigenetic programs. European J Immunol. 2016;46:1548–62. doi:10.1002/eji.201545550.
- Almeida L, Lochner M, Berod L, Sparwasser T. Metabolic pathways in T cell activation and lineage differentiation. Seminars in immunology. 2016;28:514–24. doi:10.1016/j.smim.2016.10.009.
- Kepp O, Senovilla L, Vitale I, Vacchelli E, Adjemian S, Agostinis P, Apetoh L, Aranda F, Barnaba V, Bloy N, et al. Consensus guidelines for the detection of immunogenic cell death. Oncoimmunology. 2014;3:e955691. doi:10.4161/21624011.2014.955691.
- Garg AD, Martin S, Golab J, Agostinis P. Danger signalling during cancer cell death: origins, plasticity and regulation. Cell Death Differ. 2014;21:26–38. doi:10.1038/cdd.2013.48.
- Senovilla L, Vitale I, Martins I, Tailler M, Pailleret C, Michaud M, Galluzzi L, Adjemian S, Kepp O, Niso-Santano M, et al. An immunosurveillance mechanism controls cancer cell ploidy. Science. 2012;337:1678–84. doi:10.1126/science.1224922.
- Vesely MD, Kershaw MH, Schreiber RD, Smyth MJ. Natural innate and adaptive immunity to cancer. Annu Rev Immunol. 2011;29:235–71. doi:10.1146/annurev-immunol-031210-101324.
- Schreiber RD, Old LJ, Smyth MJ. Cancer immunoediting: integrating immunity's roles in cancer suppression and promotion. Science. 2011;331:1565–70. doi:10.1126/science.1203486.
- Garg AD, Vandenberk L, Koks C, Verschuere T, Boon L, Van Gool SW, Agostinis P. Dendritic cell vaccines based on immunogenic cell death elicit danger signals and T cell-driven rejection of high-grade glioma. Sci Transl Med. 2016;8:328ra27. doi:10.1126/scitranslmed.aae0105.
- Galluzzi L, Zitvogel L, Kroemer G. Immunological Mechanisms Underneath the Efficacy of Cancer Therapy. Cancer Immunol Res. 2016;4:895–902. doi:10.1158/2326-6066.CIR-16-0197.
- Galluzzi L, Vacchelli E, Bravo-San Pedro JM, Buque A, Senovilla L, Baracco EE, Bloy N, Castoldi F, Abastado JP, Agostinis P, et al. Classification of current anticancer immunotherapies. Oncotarget. 2014;5:12472–508. doi:10.18632/oncotarget.2998.
- Galluzzi L, Bravo-San Pedro JM, Kepp O, Kroemer G. Regulated cell death and adaptive stress responses. Cell Mol Life Sci. 2016;73:2405–10. doi:10.1007/s00018-016-2209-y.
- Garg AD, De Ruysscher D, Agostinis P. Immunological metagene signatures derived from immunogenic cancer cell death associate with improved survival of patients with lung, breast or ovarian malignancies: A large-scale meta-analysis. Oncoimmunology. 2016;5:e1069938. doi:10.1080/2162402X.2015.1069938.
- Wittwer C, Boeck S, Heinemann V, Haas M, Stieber P, Nagel D, Holdenrieder S. Circulating nucleosomes and immunogenic cell death markers HMGB1, sRAGE and DNAse in patients with advanced pancreatic cancer undergoing chemotherapy. Int J Cancer. 2013;133:2619–30.
- Pol J, Vacchelli E, Aranda F, Castoldi F, Eggermont A, Cremer I, Sautès-Fridman C, Fucikova J, Galon J, Spisek R, et al. Trial Watch: Immunogenic cell death inducers for anticancer chemotherapy. Oncoimmunology. 2015;4:e1008866. doi:10.1080/2162402X.2015.1008866.
- Exner R, Sachet M, Arnold T, Zinn-Zinnenburg M, Michlmayr A, Dubsky P, Bartsch R, Steger G, Gnant M, Bergmann M, et al. Prognostic value of HMGB1 in early breast cancer patients under neoadjuvant chemotherapy. Cancer medicine. 2016;5:2350–8. doi:10.1002/cam4.827.
- Garg AD, Vara Perez M, Schaaf M, Agostinis P, Zitvogel L, Kroemer G, Galluzzi L. Trial watch: Dendritic cell-based anticancer immunotherapy. Oncoimmunology. 2017;6:e1328341. doi:10.1080/2162402X.2017.1328341.
- Garg AD, Coulie PG, Van den Eynde BJ, Agostinis P. Integrating Next-Generation Dendritic Cell Vaccines into the Current Cancer Immunotherapy Landscape. Trends Immunol. 2017;38:577–93. doi:10.1016/j.it.2017.05.006.
- Bol KF, Aarntzen EHJG, Hout FEMit, Schreibelt G, Creemers JHA, Lesterhuis WJ, et al. Favorable overall survival in stage III melanoma patients after adjuvant dendritic cell vaccination. OncoImmunology. 2016;5:e1057673. doi:10.1080/2162402X.2015.1057673.
- Vacchelli E, Ma Y, Baracco EE, Sistigu A, Enot DP, Pietrocola F, Yang H, Adjemian S, Chaba K, Semeraro M, et al. Chemotherapy-induced antitumor immunity requires formyl peptide receptor 1. Science. 2015;350:972–8. doi:10.1126/science.aad0779.
- Di Blasio S, Wortel IMN, van Bladel DAG, de Vries LE, Duiveman-de Boer T, Worah K, de Haas N, Buschow SI, de Vries IJ, Figdor CG, et al. Human CD1c+ DCs are critical cellular mediators of immune responses induced by immunogenic cell death. OncoImmunology. 2016;5:e1192739. doi:10.1080/2162402X.2016.1192739.
- Garg AD, Romano E, Rufo N, Agostinis P. Immunogenic versus tolerogenic phagocytosis during anticancer therapy: mechanisms and clinical translation. Cell Death Differ. 2016;23:938–51. doi:10.1038/cdd.2016.5.
- Lukacsi S, Nagy-Balo Z, Erdei A, Sandor N, Bajtay Z. The role of CR3 (CD11b/CD18) and CR4 (CD11c/CD18) in complement-mediated phagocytosis and podosome formation by human phagocytes. Immunol Lett. 2017;189:64–72. doi:10.1016/j.imlet.2017.05.014.
- Ligeon LA, Romao S, Munz C. Analysis of LC3-Associated Phagocytosis and Antigen Presentation. Methods Mol Biol. 2017;1519:145–68. doi:10.1007/978-1-4939-6581-6_10.
- Ohlsson SM, Pettersson A, Ohlsson S, Selga D, Bengtsson AA, Segelmark M, Hellmark T. Phagocytosis of apoptotic cells by macrophages in anti-neutrophil cytoplasmic antibody-associated systemic vasculitis. Clin Exp Immunol. 2012;170:47–56. doi:10.1111/j.1365-2249.2012.04633.x.
- Choi SC, Simhadri VR, Tian L, Gil-Krzewska A, Krzewski K, Borrego F, Coligan JE. Cutting edge: mouse CD300f (CMRF-35-like molecule-1) recognizes outer membrane-exposed phosphatidylserine and can promote phagocytosis. J Immunol (Baltimore, Md: 1950). 2011;187:3483–7. doi:10.4049/jimmunol.1101549.
- Ishimoto H, Yanagihara K, Araki N, Mukae H, Sakamoto N, Izumikawa K, Seki M, Miyazaki Y, Hirakata Y, Mizuta Y, et al. Single-cell observation of phagocytosis by human blood dendritic cells. Jpn J Infect Dis. 2008;61:294–7.
- Cornwall SMJ, Wikstrom M, Musk AW, Alvarez J, Nowak AK, Nelson DJ. Human mesothelioma induces defects in dendritic cell numbers and antigen-processing function which predict survival outcomes. OncoImmunology. 2016;5:e1082028. doi:10.1080/2162402X.2015.1082028.
- Boudewijns S, Koornstra RHT, Westdorp H, Schreibelt G, van den Eertwegh AJM, Geukes Foppen MH, Haanen JB, de Vries IJ, Figdor CG, Bol KF, et al. Ipilimumab administered to metastatic melanoma patients who progressed after dendritic cell vaccination. OncoImmunology. 2016;5:e1201625. doi:10.1080/2162402X.2016.1201625.
- Boudewijns S, Bol KF, Schreibelt G, Westdorp H, Textor JC, van Rossum MM, Scharenborg NM, de Boer AJ, van de Rakt MW, Pots JM, et al. Adjuvant dendritic cell vaccination induces tumor-specific immune responses in the majority of stage III melanoma patients. OncoImmunology. 2016;5:e1191732. doi:10.1080/2162402X.2016.1191732.
- Borch TH, Engell-Noerregaard L, Zeeberg Iversen T, Ellebaek E, Met Ö, Hansen M, Andersen MH, Thor Straten P, Svane IM. mRNA-transfected dendritic cell vaccine in combination with metronomic cyclophosphamide as treatment for patients with advanced malignant melanoma. OncoImmunology. 2016;5:e1207842. doi:10.1080/2162402X.2016.1207842.
- Besse B, Charrier M, Lapierre V, Dansin E, Lantz O, Planchard D, Le Chevalier T, Livartoski A, Barlesi F, Laplanche A, et al. Dendritic cell-derived exosomes as maintenance immunotherapy after first line chemotherapy in NSCLC. OncoImmunology. 2016;5:e1071008. doi:10.1080/2162402X.2015.1071008.
- Pyfferoen L, Brabants E, Everaert C, De Cabooter N, Heyns K, Deswarte K, Vanheerswynghels M, De Prijck S, Waegemans G, Dullaers M, et al. The transcriptome of lung tumor-infiltrating dendritic cells reveals a tumor-supporting phenotype and a microRNA signature with negative impact on clinical outcome. Oncoimmunology. 2017;6:e1253655. doi:10.1080/2162402X.2016.1253655.
- McDonnell AM, Cook A, Robinson BWS, Lake RA, Nowak AK. Serial immunomonitoring of cancer patients receiving combined antagonistic anti-CD40 and chemotherapy reveals consistent and cyclical modulation of T cell and dendritic cell parameters. BMC Cancer. 2017;17:417. doi:10.1186/s12885-017-3403-5.
- Chen L, Hasni MS, Jondal M, Yakimchuk K. Modification of anti-tumor immunity by tolerogenic dendritic cells. Autoimmunity. 2017;50:370–6. doi:10.1080/08916934.2017.1344837.
- Castiello L, Sabatino M, Ren J, Terabe M, Khuu H, Wood LV, Berzofsky JA, Stroncek DF. Expression of CD14, IL10, and Tolerogenic Signature in Dendritic Cells Inversely Correlate with Clinical and Immunologic Response to TARP Vaccination in Prostate Cancer Patients. Autoimmunity. 2017;23:3352–64.
- Van den Bergh J, Willemen Y, Lion E, Van Acker H, De Reu H, Anguille S, Goossens H, Berneman Z, Van Tendeloo V, Smits E. Transpresentation of interleukin-15 by IL-15/IL-15Ralpha mRNA-engineered human dendritic cells boosts antitumoral natural killer cell activity. Oncotarget. 2015;6:44123–33. doi:10.18632/oncotarget.6536.
- Ghinnagow R, De Meester J, Cruz LJ, Aspord C, Corgnac S, Macho-Fernandez E, Soulard D, Fontaine J, Chaperot L, Charles J, et al. Co-delivery of the NKT agonist alpha-galactosylceramide and tumor antigens to cross-priming dendritic cells breaks tolerance to self-antigens and promotes antitumor responses. Oncoimmunology. 2017;6:e1339855. doi:10.1080/2162402X.2017.1339855.
- Briseno CG, Haldar M, Kretzer NM, Wu X, Theisen DJ, Kc W, Durai V, Grajales-Reyes GE, Iwata A, Bagadia P, et al. Distinct Transcriptional Programs Control Cross-Priming in Classical and Monocyte-Derived Dendritic Cells. Cell Rep. 2016;15:2462–74. doi:10.1016/j.celrep.2016.05.025.
- Zhang Y, Chen G, Liu Z, Tian S, Zhang J, Carey CD, Murphy KM, Storkus WJ, Falo LD Jr, You Z. Genetic vaccines to potentiate the effective CD103+ dendritic cell-mediated cross-priming of antitumor immunity. J Immunol (Baltimore, Md: 1950). 2015;194:5937–47. doi:10.4049/jimmunol.1500089.
- Osmond TL, Farrand KJ, Painter GF, Ruedl C, Petersen TR, Hermans IF. Activated NKT Cells Can Condition Different Splenic Dendritic Cell Subsets To Respond More Effectively to TLR Engagement and Enhance Cross-Priming. J Immunol (Baltimore, Md: 1950). 2015;195:821–31. doi:10.4049/jimmunol.1401751.
- Leavy O. Cell death: Pathways for cross-priming. Nat Rev Immunol. 2015;15:725. doi:10.1038/nri3933.
- Katakam AK, Brightbill H, Franci C, Kung C, Nunez V, Jones C 3rd, Peng I, Jeet S, Wu LC, Mellman I, et al. Dendritic cells require NIK for CD40-dependent cross-priming of CD8+ T cells. Proc Natl Acad Sci U S A. 2015;112:14664–9. doi:10.1073/pnas.1520627112.
- Stephenson RM, Lim CM, Matthews M, Dietsch G, Hershberg R, Ferris RL. TLR8 stimulation enhances cetuximab-mediated natural killer cell lysis of head and neck cancer cells and dendritic cell cross-priming of EGFR-specific CD8+ T cells. Cancer Immunol Immunother. 2013;62:1347–57. doi:10.1007/s00262-013-1437-3.
- Gamrekelashvili J, Kapanadze T, Han M, Wissing J, Ma C, Jaensch L, Manns MP, Armstrong T, Jaffee E, White AO, et al. Peptidases released by necrotic cells control CD8+ T cell cross-priming. J Clin Invest. 2013;123:4755–68. doi:10.1172/JCI65698.
- Watson AM, Mylin LM, Thompson MM, Schell TD. Modification of a tumor antigen determinant to improve peptide/MHC stability is associated with increased immunogenicity and cross-priming a larger fraction of CD8+ T cells. J Immunol (Baltimore, Md: 1950). 2012;189:5549–60. doi:10.4049/jimmunol.1102221.
- Menger L, Vacchelli E, Adjemian S, Martins I, Ma Y, Shen S, Yamazaki T, Sukkurwala AQ, Michaud M, Mignot G, et al. Cardiac glycosides exert anticancer effects by inducing immunogenic cell death. Sci Transl Med. 2012;4:143ra99. doi:10.1126/scitranslmed.3003807.
- Hernandez C, Huebener P, Schwabe RF. Damage-associated molecular patterns in cancer: a double-edged sword. Oncogene. 2016;35:5931–41. doi:10.1038/onc.2016.104.
- Obeid M, Tesniere A, Ghiringhelli F, Fimia GM, Apetoh L, Perfettini JL, Castedo M, Mignot G, Panaretakis T, Casares N, et al. Calreticulin exposure dictates the immunogenicity of cancer cell death. Nat Med. 2007;13:54–61. doi:10.1038/nm1523.
- Gardai SJ, McPhillips KA, Frasch SC, Janssen WJ, Starefeldt A, Murphy-Ullrich JE, Bratton DL, Oldenborg PA, Michalak M, Henson PM. Cell-surface calreticulin initiates clearance of viable or apoptotic cells through trans-activation of LRP on the phagocyte. Cell. 2005;123:321–34. doi:10.1016/j.cell.2005.08.032.
- Garg AD, Elsen S, Krysko DV, Vandenabeele P, de Witte P, Agostinis P. Resistance to anticancer vaccination effect is controlled by a cancer cell-autonomous phenotype that disrupts immunogenic phagocytic removal. Oncotarget. 2015;6:26841–60. doi:10.18632/oncotarget.4754.
- Elliott MR, Chekeni FB, Trampont PC, Lazarowski ER, Kadl A, Walk SF, Park D, Woodson RI, Ostankovich M, Sharma P, et al. Nucleotides released by apoptotic cells act as a find-me signal to promote phagocytic clearance. Nature. 2009;461:282–6. doi:10.1038/nature08296.
- Chekeni FB, Elliott MR, Sandilos JK, Walk SF, Kinchen JM, Lazarowski ER, Armstrong AJ, Penuela S, Laird DW, Salvesen GS, et al. Pannexin 1 channels mediate ‘find-me’ signal release and membrane permeability during apoptosis. Nature. 2010;467:863–7. doi:10.1038/nature09413.
- Martins I, Wang Y, Michaud M, Ma Y, Sukkurwala AQ, Shen S, Kepp O, Métivier D, Galluzzi L, Perfettini JL, et al. Molecular mechanisms of ATP secretion during immunogenic cell death. Cell Death Differ. 2014;21:79–91. doi:10.1038/cdd.2013.75.
- Galluzzi L, Bravo-San Pedro JM, Demaria S, Formenti SC, Kroemer G. Activating autophagy to potentiate immunogenic chemotherapy and radiation therapy. Nat Rev Clin Oncol. 2017;14:247–58. doi:10.1038/nrclinonc.2016.183.
- Garg AD, Krysko DV, Vandenabeele P, Agostinis P. Extracellular ATP and P(2)X(7) receptor exert context-specific immunogenic effects after immunogenic cancer cell death. Cell Death Dis. 2016;7:e2097. doi:10.1038/cddis.2015.411.
- Scaffidi P, Misteli T, Bianchi ME. Release of chromatin protein HMGB1 by necrotic cells triggers inflammation. Nature. 2002;418:191–5. doi:10.1038/nature00858.
- Sistigu A, Yamazaki T, Vacchelli E, Chaba K, Enot DP, Adam J, Vitale I, Goubar A, Baracco EE, Remédios C, et al. Cancer cell-autonomous contribution of type I interferon signaling to the efficacy of chemotherapy. Nat Med. 2014;20:1301–9. doi:10.1038/nm.3708.
- Vanpouille-Box C, Alard A, Aryankalayil MJ, Sarfraz Y, Diamond JM, Schneider RJ, Inghirami G, Coleman CN, Formenti SC, Demaria S. DNA exonuclease Trex1 regulates radiotherapy-induced tumour immunogenicity. Nat Commun. 2017;8:15618. doi:10.1038/ncomms15618.
- Harding SM, Benci JL, Irianto J, Discher DE, Minn AJ, Greenberg RA. Mitotic progression following DNA damage enables pattern recognition within micronuclei. Nature. 2017;548:466–70. doi:10.1038/nature23470.
- Mackenzie KJ, Carroll P, Martin CA, Murina O, Fluteau A, Simpson DJ, Olova N, Sutcliffe H, Rainger JK, Leitch A, et al. cGAS surveillance of micronuclei links genome instability to innate immunity. Nature. 2017;548:461–5. doi:10.1038/nature23449.
- Garg AD, Vandenberk L, Fang S, Fasche T, Van Eygen S, Maes J, Van Woensel M, Koks C, Vanthillo N, Graf N, et al. Pathogen response-like recruitment and activation of neutrophils by sterile immunogenic dying cells drives neutrophil-mediated residual cell killing. Cell Death Differ. 2017;24:832–43. doi:10.1038/cdd.2017.15.
- Garg AD, Krysko DV, Verfaillie T, Kaczmarek A, Ferreira GB, Marysael T, Rubio N, Firczuk M, Mathieu C, Roebroek AJ, et al. A novel pathway combining calreticulin exposure and ATP secretion in immunogenic cancer cell death. EMBO J. 2012;31:1062–79. doi:10.1038/emboj.2011.497.
- Garg AD, Dudek AM, Ferreira GB, Verfaillie T, Vandenabeele P, Krysko DV, Mathieu C, Agostinis P. ROS-induced autophagy in cancer cells assists in evasion from determinants of immunogenic cell death. Autophagy. 2013;9:1292–307. doi:10.4161/auto.25399.
- Spranger S, Bao R, Gajewski TF. Melanoma-intrinsic beta-catenin signalling prevents anti-tumour immunity. Nature. 2015;523:231–5. doi:10.1038/nature14404.
- Venereau E, Ceriotti C, Bianchi ME. DAMPs from Cell Death to New Life. Front Immunol. 2015;6:422. doi:10.3389/fimmu.2015.00422.
- Li G, Tang D, Lotze MT. Menage a Trois in stress: DAMPs, redox and autophagy. Semin Cancer Biol. 2013;23:380–90. doi:10.1016/j.semcancer.2013.08.002.
- Hou W, Zhang Q, Yan Z, Chen R, Zeh Iii HJ, Kang R, Lotze MT, Tang D. Strange attractors: DAMPs and autophagy link tumor cell death and immunity. Cell Death Dis. 2013;4:e966. doi:10.1038/cddis.2013.493.
- He Y, Zha J, Wang Y, Liu W, Yang X, Yu P. Tissue Damage–Associated “Danger Signals” Influence T-cell Responses That Promote the Progression of Preneoplasia to Cancer. Cancer Res. 2013;73:629–39. doi:10.1158/0008-5472.CAN-12-2704.
- Willemen Y, Van den Bergh JM, Lion E, Anguille S, Roelandts VA, Van Acker HH, Heynderickx SD, Stein BM, Peeters M, Figdor CG, et al. Engineering monocyte-derived dendritic cells to secrete interferon-alpha enhances their ability to promote adaptive and innate anti-tumor immune effector functions. Cancer Immunol Immunother. 2015;64:831–42. doi:10.1007/s00262-015-1688-2.
- Kayser S, Bobeta C, Feucht J, Witte KE, Scheu A, Bulow HJ, Joachim S, Stevanović S, Schumm M, Rittig SM, et al. Rapid generation of NY-ESO-1-specific CD4+ THELPER1 cells for adoptive T-cell therapy. Oncoimmunology. 2015;4:e1002723. doi:10.1080/2162402X.2014.1002723.
- Poschke I, Mougiakakos D, Hansson J, Masucci GV, Kiessling R. Immature immunosuppressive CD14+HLA-DR-/low cells in melanoma patients are Stat3hi and overexpress CD80, CD83, and DC-sign. Cancer Res. 2010;70:4335–45. doi:10.1158/0008-5472.CAN-09-3767.
- Birkholz K, Schwenkert M, Kellner C, Gross S, Fey G, Schuler-Thurner B, Schuler G, Schaft N, Dörrie J. Targeting of DEC-205 on human dendritic cells results in efficient MHC class II-restricted antigen presentation. Blood. 2010;116:2277–85. doi:10.1182/blood-2010-02-268425.
- Nagahara K, Arikawa T, Oomizu S, Kontani K, Nobumoto A, Tateno H, Watanabe K, Niki T, Katoh S, Miyake M, et al. Galectin-9 increases Tim-3+ dendritic cells and CD8+ T cells and enhances antitumor immunity via galectin-9-Tim-3 interactions. J Immunol (Baltimore, Md: 1950). 2008;181:7660–9. doi:10.4049/jimmunol.181.11.7660.
- Keller AM, Schildknecht A, Xiao Y, van den Broek M, Borst J. Expression of costimulatory ligand CD70 on steady-state dendritic cells breaks CD8+ T cell tolerance and permits effective immunity. Immunity. 2008;29:934–46. doi:10.1016/j.immuni.2008.10.009.
- Sharma P, Hu-Lieskovan S, Wargo JA, Ribas A. Primary, Adaptive, and Acquired Resistance to Cancer Immunotherapy. Cell. 2017;168:707–23. doi:10.1016/j.cell.2017.01.017.
- Lampen MH, van Hall T. Strategies to counteract MHC-I defects in tumors. Curr Opin Immunol. 2011;23:293–8. doi:10.1016/j.coi.2010.12.005.
- Schmid DA, Irving MB, Posevitz V, Hebeisen M, Posevitz-Fejfar A, Sarria JC, Gomez-Eerland R, Thome M, Schumacher TN, Romero P, et al. Evidence for a TCR affinity threshold delimiting maximal CD8 T cell function. J Immunol (Baltimore, Md: 1950). 2010;184:4936–46. doi:10.4049/jimmunol.1000173.
- Meije CB, Swart GW, Lepoole C, Das PK, Van den Oord JJ. Antigenic profiles of individual-matched pairs of primary and melanoma metastases. Hum Pathol. 2009;40:1399–407. doi:10.1016/j.humpath.2008.11.018.
- Gubin MM, Artyomov MN, Mardis ER, Schreiber RD. Tumor neoantigens: building a framework for personalized cancer immunotherapy. J Clin Invest. 2015;125:3413–21. doi:10.1172/JCI80008.
- Zhang Y, Kurupati R, Liu L, Zhou XY, Zhang G, Hudaihed A, Filisio F, Giles-Davis W, Xu X, Karakousis GC, et al. Enhancing CD8+ T Cell Fatty Acid Catabolism within a Metabolically Challenging Tumor Microenvironment Increases the Efficacy of Melanoma Immunotherapy. Cancer Cell. 2017;32:377–91.e9. doi:10.1016/j.ccell.2017.08.004.
- Wennhold K, Thelen M, Schlosser HA, Haustein N, Reuter S, Garcia-Marquez M, Lechner A, Kobold S, Rataj F, Utermöhlen O, et al. Using Antigen-Specific B Cells to Combine Antibody and T Cell-Based Cancer Immunotherapy. Cancer Immunol Res. 2017;5:730–43. doi:10.1158/2326-6066.CIR-16-0236.
- Patel SJ, Sanjana NE, Kishton RJ, Eidizadeh A, Vodnala SK, Cam M, Gartner JJ, Jia L, Steinberg SM, Yamamoto TN, et al. Identification of essential genes for cancer immunotherapy. Nat Immunol. 2017;548:537–42.
- Araki K, Morita M, Bederman AG, Konieczny BT, Kissick HT, Sonenberg N, Ahmed R. Translation is actively regulated during the differentiation of CD8+ effector T cells. Nat Immunol. 2017;18:1046–57.
- Li T, Zhang Q, Jiang Y, Yu J, Hu Y, Mou T, Chen G, Li G. Gastric cancer cells inhibit natural killer cell proliferation and induce apoptosis via prostaglandin E2. Oncoimmunology. 2016;5:e1069936. doi:10.1080/2162402X.2015.1069936.
- Strannegard O, Thoren FB. Opposing effects of immunotherapy in melanoma using multisubtype interferon-alpha – can tumor immune escape after immunotherapy accelerate disease progression? Oncoimmunology. 2016;5:e1091147. doi:10.1080/2162402X.2015.1091147.
- Chung EY, Liu J, Homma Y, Zhang Y, Brendolan A, Saggese M, Han J, Silverstein R, Selleri L, Ma X. Interleukin-10 expression in macrophages during phagocytosis of apoptotic cells is mediated by homeodomain proteins Pbx1 and Prep-1. Immunity. 2007;27:952–64. doi:10.1016/j.immuni.2007.11.014.
- Fadok VA, Bratton DL, Konowal A, Freed PW, Westcott JY, Henson PM. Macrophages that have ingested apoptotic cells in vitro inhibit proinflammatory cytokine production through autocrine/paracrine mechanisms involving TGF-beta, PGE2, and PAF. J Clin Invest. 1998;101:890–8. doi:10.1172/JCI1112.
- Grivennikov SI, Greten FR, Karin M. Immunity, inflammation, and cancer. Cell. 2010;140:883–99. doi:10.1016/j.cell.2010.01.025.
- Wong HS, Chang CM, Liu X, Huang WC, Chang WC. Characterization of cytokinome landscape for clinical responses in human cancers. Oncoimmunology. 2016;5:e1214789. doi:10.1080/2162402X.2016.1214789.
- Rahbar A, Cederarv M, Wolmer-Solberg N, Tammik C, Stragliotto G, Peredo I, Fornara O, Xu X, Dzabic M, Taher C, et al. Enhanced neutrophil activity is associated with shorter time to tumor progression in glioblastoma patients. Oncoimmunology. 2016;5:e1075693. doi:10.1080/2162402X.2015.1075693.
- Yeku OO, Purdon TJ, Koneru M, Spriggs D, Brentjens RJ. Armored CAR T cells enhance antitumor efficacy and overcome the tumor microenvironment. Sci Rep. 2017;7:10541. doi:10.1038/s41598-017-10940-8.
- Haderk F, Schulz R, Iskar M, Cid LL, Worst T, Willmund KV, Schulz A, Warnken U, Seiler J, Benner A, et al. Tumor-derived exosomes modulate PD-L1 expression in monocytes. Sci Immunol. 2017;2:eaah5509. doi:10.1126/sciimmunol.aah5509.
- Spranger S, Dai D, Horton B, Gajewski TF. Tumor-Residing Batf3 Dendritic Cells Are Required for Effector T Cell Trafficking and Adoptive T Cell Therapy. Cancer Cell. 2017;31:711–23.e4. doi:10.1016/j.ccell.2017.04.003.
- Cottone L, Capobianco A, Gualteroni C, Monno A, Raccagni I, Valtorta S, Canu T, Di Tomaso T, Lombardo A, Esposito A, et al. Leukocytes recruited by tumor-derived HMGB1 sustain peritoneal carcinomatosis. Oncoimmunology. 2016;5:e1122860. doi:10.1080/2162402X.2015.1122860.
- Serra S, Horenstein AL, Vaisitti T, Brusa D, Rossi D, Laurenti L, D'Arena G, Coscia M, Tripodo C, Inghirami G, et al. CD73-generated extracellular adenosine in chronic lymphocytic leukemia creates local conditions counteracting drug-induced cell death. Blood. 2011;118:6141–52. doi:10.1182/blood-2011-08-374728.
- McDonald B, Pittman K, Menezes GB, Hirota SA, Slaba I, Waterhouse CC, Beck PL, Muruve DA, Kubes P. Intravascular danger signals guide neutrophils to sites of sterile inflammation. Science. 2010;330:362–6. doi:10.1126/science.1195491.
- Moser B, Wolf M, Walz A, Loetscher P. Chemokines: multiple levels of leukocyte migration control. Trends Immunol. 2004;25:75–84. doi:10.1016/j.it.2003.12.005.
- Taylor NA, Vick SC, Iglesia MD, Brickey WJ, Midkiff BR, McKinnon KP, Reisdorf S, Anders CK, Carey LA, Parker JS, et al. Treg depletion potentiates checkpoint inhibition in claudin-low breast cancer. J Clin Invest. 2017;127:3472–83. doi:10.1172/JCI90499.
- Lim TS, Chew V, Sieow JL, Goh S, Yeong JP, Soon AL, Ricciardi-Castagnoli P. PD-1 expression on dendritic cells suppresses CD8+ T cell function and antitumor immunity. Oncoimmunology. 2016;5:e1085146. doi:10.1080/2162402X.2015.1085146.
- Pardoll DM. The blockade of immune checkpoints in cancer immunotherapy. Nat Rev Cancer. 2012;12:252–64. doi:10.1038/nrc3239.
- Sharma P, Allison JP. The future of immune checkpoint therapy. Science. 2015;348:56–61. doi:10.1126/science.aaa8172.
- Garg AD, Vandenberk L, Van Woensel M, Belmans J, Schaaf M, Boon L, De Vleeschouwer S, Agostinis P. Preclinical efficacy of immune-checkpoint monotherapy does not recapitulate corresponding biomarkers-based clinical predictions in glioblastoma. Oncoimmunology. 2017;6:e1295903. doi:10.1080/2162402X.2017.1295903.
- Kuttke M, Sahin E, Pisoni J, Percig S, Vogel A, Kraemmer D, Hanzl L, Brunner JS, Paar H, Soukup K, et al. Myeloid PTEN deficiency impairs tumor-immune surveillance via immune-checkpoint inhibition. Oncoimmunology. 2016;5:e1164918. doi:10.1080/2162402X.2016.1164918.
- Wu MZ, Cheng WC, Chen SF, Nieh S, O'Connor C, Liu CL, Tsai WW, Wu CJ, Martin L, Lin YS, et al. miR-25/93 mediates hypoxia-induced immunosuppression by repressing cGAS. Nat Cell Biol. 2017;19(10):1286–96. doi:10.1038/ncb3615.
- Turtle CJ, Hay KA, Hanafi LA, Li D, Cherian S, Chen X, Wood B, Lozanski A, Byrd JC, Heimfeld S, et al. Durable Molecular Remissions in Chronic Lymphocytic Leukemia Treated With CD19-Specific Chimeric Antigen Receptor-Modified T Cells After Failure of Ibrutinib. Nat Cell Biol. 2017;35:3010–20.
- Manguso RT, Pope HW, Zimmer MD, Brown FD, Yates KB, Miller BC, Collins NB, Bi K, LaFleur MW, Juneja VR, et al. In vivo CRISPR screening identifies Ptpn2 as a cancer immunotherapy target. Sci Signal. 2017;547:413–8.
- Grinberg-Bleyer Y, Oh H, Desrichard A, Bhatt DM, Caron R, Chan TA, Schmid RM, Klein U, Hayden MS, Ghosh S, et al. NF-kappaB c-Rel Is Crucial for the Regulatory T Cell Immune Checkpoint in Cancer. Cell. 2017;170:1096–108.e13. doi:10.1016/j.cell.2017.08.004.
- Burr ML, Sparbier CE, Chan YC, Williamson JC, Woods K, Beavis PA, Lam EYN, Henderson MA, Bell CC, Stolzenburg S, et al. CMTM6 maintains the expression of PD-L1 and regulates anti-tumour immunity. Nature. 2017;549:101–5. doi:10.1038/nature23643.
- Budhu S, Schaer DA, Li Y, Toledo-Crow R, Panageas K, Yang X, Zhong H, Houghton AN, Silverstein SC, Merghoub T, et al. Blockade of surface-bound TGF-beta on regulatory T cells abrogates suppression of effector T cell function in the tumor microenvironment. Sci Signal. 2017;10:aak9702. doi:10.1126/scisignal.aak9702.
- Sharma P, Allison JP. Immune checkpoint targeting in cancer therapy: toward combination strategies with curative potential. Cell. 2015;161:205–14. doi:10.1016/j.cell.2015.03.030.
- Dunn GP, Bruce AT, Ikeda H, Old LJ, Schreiber RD. Cancer immunoediting: from immunosurveillance to tumor escape. Nat Immunol. 2002;3:991–8. doi:10.1038/ni1102-991.
- Henrickson SE, Perro M, Loughhead SM, Senman B, Stutte S, Quigley M, Alexe G, Iannacone M, Flynn MP, Omid S, et al. Antigen availability determines CD8(+) T cell-dendritic cell interaction kinetics and memory fate decisions. Immunity. 2013;39:496–507. doi:10.1016/j.immuni.2013.08.034.
- Nirschl CJ, Suarez-Farinas M, Izar B, Prakadan S, Dannenfelser R, Tirosh I, Liu Y, Zhu Q, Devi KSP, Carroll SL, et al. IFNgamma-Dependent Tissue-Immune Homeostasis Is Co-opted in the Tumor Microenvironment. Cell. 2017;170:127–41.e15. doi:10.1016/j.cell.2017.06.016.
- Griffith TS, Ferguson TA. Cell death in the maintenance and abrogation of tolerance: the five Ws of dying cells. Immunity. 2011;35:456–66. doi:10.1016/j.immuni.2011.08.011.
- Paul S, Kulkarni N, Shilpi, Lal G. Intratumoral natural killer cells show reduced effector and cytolytic properties and control the differentiation of effector Th1 cells. Oncoimmunology. 2016;5:e1235106. doi:10.1080/2162402X.2016.1235106.
- Showalter A, Limaye A, Oyer JL, Igarashi R, Kittipatarin C, Copik AJ, Khaled AR. Cytokines in immunogenic cell death: Applications for cancer immunotherapy. Cytokine. 2017;97:123–32. doi:10.1016/j.cyto.2017.05.024.
- Seelige R, Searles S, Bui JD. Mechanisms regulating immune surveillance of cellular stress in cancer. Cell Mol Life Sci. 2017. doi:10.1007/s00018-017-2597-7.
- Tsuchikawa T, Takeuchi S, Nakamura T, Shichinohe T, Hirano S. Clinical impact of chemotherapy to improve tumor microenvironment of pancreatic cancer. World J Gastrointest Oncol. 2016;8:786–92. doi:10.4251/wjgo.v8.i11.786.
- Terenzi A, Pirker C, Keppler BK, Berger W. Anticancer metal drugs and immunogenic cell death. Cell Mol Life Sci. 2016;165:71–9.
- Galluzzi L, Senovilla L, Vitale I, Michels J, Martins I, Kepp O, et al. Molecular mechanisms of cisplatin resistance. Oncogene. 2012;31:1869–83. doi:10.1038/onc.2011.384.
- Martins I, Kepp O, Schlemmer F, Adjemian S, Tailler M, Shen S, Michaud M, Menger L, Gdoura A, Tajeddine N, et al. Restoration of the immunogenicity of cisplatin-induced cancer cell death by endoplasmic reticulum stress. Oncogene. 2011;30:1147–58. doi:10.1038/onc.2010.500.
- Dudek-Peric AM, Ferreira GB, Muchowicz A, Wouters J, Prada N, Martin S, Kiviluoto S, Winiarska M, Boon L, Mathieu C, et al. Antitumor immunity triggered by melphalan is potentiated by melanoma cell surface-associated calreticulin. Cancer Res. 2015;75:1603–14. doi:10.1158/0008-5472.CAN-14-2089.
- Garg AD, Maes H, van Vliet AR, Agostinis P. Targeting the hallmarks of cancer with therapy-induced endoplasmic reticulum (ER) stress. Mol Cell Oncol. 2015;2:e975089. doi:10.4161/23723556.2014.975089.
- Berry V, Basson L, Bogart E, Mir O, Blay JY, Italiano A, Bertucci F, Chevreau C, Clisant-Delaine S, Liegl-Antzager B, et al. REGOSARC: Regorafenib versus placebo in doxorubicin-refractory soft-tissue sarcoma-A quality-adjusted time without symptoms of progression or toxicity analysis. Cancer. 2017;123:2294–302. doi:10.1002/cncr.30661.
- Tap WD, Jones RL, Van Tine BA, Chmielowski B, Elias AD, Adkins D, Agulnik M, Cooney MM, Livingston MB, Pennock G, et al. Olaratumab and doxorubicin versus doxorubicin alone for treatment of soft-tissue sarcoma: an open-label phase 1b and randomised phase 2 trial. Lancet. 2016;388:488–97. doi:10.1016/S0140-6736(16)30587-6.
- Orlowski RZ, Nagler A, Sonneveld P, Blade J, Hajek R, Spencer A, Robak T, Dmoszynska A, Horvath N, Spicka I, et al. Final overall survival results of a randomized trial comparing bortezomib plus pegylated liposomal doxorubicin with bortezomib alone in patients with relapsed or refractory multiple myeloma. Cancer. 2016;122:2050–6. doi:10.1002/cncr.30026.
- Choy E, Flamand Y, Balasubramanian S, Butrynski JE, Harmon DC, George S, Cote GM, Wagner AJ, Morgan JA, Sirisawad M, et al. Phase 1 study of oral abexinostat, a histone deacetylase inhibitor, in combination with doxorubicin in patients with metastatic sarcoma. Cancer. 2015;121:1223–30. doi:10.1002/cncr.29175.
- Prajapati HJ, Xing M, Spivey JR, Hanish SI, El-Rayes BF, Kauh JS, Chen Z, Kim HS. Survival, efficacy, and safety of small versus large doxorubicin drug-eluting beads TACE chemoembolization in patients with unresectable HCC. AJR Am J Roentgenol. 2014;203:W706–14. doi:10.2214/AJR.13.12308.
- Morris PG, Iyengar NM, Patil S, Chen C, Abbruzzi A, Lehman R, Steingart R, Oeffinger KC, Lin N, Moy B, et al. Long-term cardiac safety and outcomes of dose-dense doxorubicin and cyclophosphamide followed by paclitaxel and trastuzumab with and without lapatinib in patients with early breast cancer. Cancer. 2013;119:3943–51. doi:10.1002/cncr.28284.
- Lipshultz SE, Miller TL, Lipsitz SR, Neuberg DS, Dahlberg SE, Colan SD, Silverman LB, Henkel JM, Franco VI, Cushman LL, et al. Continuous Versus Bolus Infusion of Doxorubicin in Children With ALL: Long-term Cardiac Outcomes. Pediatrics. 2012;130:1003–11. doi:10.1542/peds.2012-0727.
- Sun F, Shi J, Geng C. Dexrazoxane improves cardiac autonomic function in epirubicin-treated breast cancer patients with type 2 diabetes. Medicine. 2016;95:e5228. doi:10.1097/MD.0000000000005228.
- Hemdan T, Johansson R, Jahnson S, Hellstrom P, Tasdemir I, Malmstrom PU. 5-Year outcome of a randomized prospective study comparing bacillus Calmette-Guerin with epirubicin and interferon-alpha2b in patients with T1 bladder cancer. J Urol. 2014;191:1244–9. doi:10.1016/j.juro.2013.11.005.
- Fucikova J, Kralikova P, Fialova A, Brtnicky T, Rob L, Bartunkova J, Spísek R. Human tumor cells killed by anthracyclines induce a tumor-specific immune response. Cancer Res. 2011;71:4821–33. doi:10.1158/0008-5472.CAN-11-0950.
- Bugaut H, Bruchard M, Berger H, Derangere V, Odoul L, Euvrard R, et al. Bleomycin exerts ambivalent antitumor immune effect by triggering both immunogenic cell death and proliferation of regulatory T cells. PLoS One. 2013;8:e65181. doi:10.1371/journal.pone.0065181.
- Attal M, Lauwers-Cances V, Hulin C, Leleu X, Caillot D, Escoffre M, Arnulf B, Macro M, Belhadj K, Garderet L, et al. Lenalidomide, Bortezomib, and Dexamethasone with Transplantation for Myeloma. N Engl J Med. 2017;376:1311–20. doi:10.1056/NEJMoa1611750.
- Palumbo A, Chanan-Khan A, Weisel K, Nooka AK, Masszi T, Beksac M, Spicka I, Hungria V, Munder M, Mateos MV, et al. Daratumumab, Bortezomib, and Dexamethasone for Multiple Myeloma. N Engl J Med. 2016;375:754–66. doi:10.1056/NEJMoa1606038.
- Kumar SK, LaPlant BR, Reeder CB, Roy V, Halvorson AE, Buadi F, Masszi T, Spencer A, Hájek R, Romeril K, et al. Randomized phase 2 trial of ixazomib and dexamethasone in relapsed multiple myeloma not refractory to bortezomib. Blood. 2016;128:2415–22. doi:10.1182/blood-2016-05-717769.
- Jakubowiak A, Offidani M, Pegourie B, De La Rubia J, Garderet L, Laribi K, Bosi A, Marasca R, Laubach J, Mohrbacher A, et al. Randomized phase 2 study: elotuzumab plus bortezomib/dexamethasone vs bortezomib/dexamethasone for relapsed/refractory MM. Blood. 2016;127:2833–40. doi:10.1182/blood-2016-01-694604.
- Chari A, Htut M, Zonder JA, Fay JW, Jakubowiak AJ, Levy JB, Lau K, Burt SM, Tunquist BJ, Hilder BW, et al. A phase 1 dose-escalation study of filanesib plus bortezomib and dexamethasone in patients with recurrent/refractory multiple myeloma. Cancer. 2016;122:3327–35. doi:10.1002/cncr.30174.
- Robak T, Huang H, Jin J, Zhu J, Liu T, Samoilova O, Pylypenko H, Verhoef G, Siritanaratkul N, Osmanov E, et al. Bortezomib-based therapy for newly diagnosed mantle-cell lymphoma. N Engl J Med. 2015;372:944–53. doi:10.1056/NEJMoa1412096.
- Offner F, Samoilova O, Osmanov E, Eom HS, Topp MS, Raposo J, Pavlov V, Ricci D, Chaturvedi S, Zhu E, et al. Frontline rituximab, cyclophosphamide, doxorubicin, and prednisone with bortezomib (VR-CAP) or vincristine (R-CHOP) for non-GCB DLBCL. Blood. 2015;126:1893–901. doi:10.1182/blood-2015-03-632430.
- Fenske TS, Shah NM, Kim KM, Saha S, Zhang C, Baim AE, Farnen JP, Onitilo AA, Blank JH, Ahuja H, et al. A phase 2 study of weekly temsirolimus and bortezomib for relapsed or refractory B-cell non-Hodgkin lymphoma: A Wisconsin Oncology Network study. Cancer. 2015;121:3465–71. doi:10.1002/cncr.29502.
- Spisek R, Charalambous A, Mazumder A, Vesole DH, Jagannath S, Dhodapkar MV. Bortezomib enhances dendritic cell (DC)-mediated induction of immunity to human myeloma via exposure of cell surface heat shock protein 90 on dying tumor cells: therapeutic implications. Blood. 2007;109:4839–45. doi:10.1182/blood-2006-10-054221.
- Demaria S, Santori FR, Ng B, Liebes L, Formenti SC, Vukmanovic S. Select forms of tumor cell apoptosis induce dendritic cell maturation. J Leukoc Biol. 2005;77:361–8. doi:10.1189/jlb.0804478.
- Cirone M, Di Renzo L, Lotti LV, Conte V, Trivedi P, Santarelli R, Gonnella R, Frati L, Faggioni A. Primary effusion lymphoma cell death induced by bortezomib and AG 490 activates dendritic cells through CD91. PLoS One. 2012;7:e31732. doi:10.1371/journal.pone.0031732.
- Baz RC, Martin TG, 3rd, Lin HY, Zhao X, Shain KH, Cho HJ, Wolf JL, Mahindra A, Chari A, Sullivan DM, et al. Randomized multicenter phase 2 study of pomalidomide, cyclophosphamide, and dexamethasone in relapsed refractory myeloma. Blood. 2016;127:2561–8. doi:10.1182/blood-2015-11-682518.
- Short NJ, Keating MJ, Wierda WG, Faderl S, Ferrajoli A, Estrov Z, Smith SC, O'Brien SM. Fludarabine, cyclophosphamide, and multiple-dose rituximab as frontline therapy for chronic lymphocytic leukemia. Cancer. 2015;121:3869–76. doi:10.1002/cncr.29605.
- Rossi D, Terzi-di-Bergamo L, De Paoli L, Cerri M, Ghilardi G, Chiarenza A, Bulian P, Visco C, Mauro FR, Morabito F, et al. Molecular prediction of durable remission after first-line fludarabine-cyclophosphamide-rituximab in chronic lymphocytic leukemia. Blood. 2015;126:1921–4. doi:10.1182/blood-2015-05-647925.
- Pagnoux C, Quemeneur T, Ninet J, Diot E, Kyndt X, de Wazieres B, Reny JL, Puéchal X, le Berruyer PY, Lidove O, et al. Treatment of systemic necrotizing vasculitides in patients aged sixty-five years or older: results of a multicenter, open-label, randomized controlled trial of corticosteroid and cyclophosphamide-based induction therapy. Arthritis Rheumatol (Hoboken, NJ). 2015;67:1117–27. doi:10.1002/art.39011.
- Kastritis E, Gavriatopoulou M, Kyrtsonis MC, Roussou M, Hadjiharissi E, Symeonidis A, Repoussis P, Michalis E, Delimpasi S, Tsatalas K, et al. Dexamethasone, rituximab, and cyclophosphamide as primary treatment of Waldenstrom macroglobulinemia: final analysis of a phase 2 study. Blood. 2015;126:1392–4. doi:10.1182/blood-2015-05-647420.
- Brown JR, O'Brien S, Kingsley CD, Eradat H, Pagel JM, Lymp J, Hirata J, Kipps TJ. Obinutuzumab plus fludarabine/cyclophosphamide or bendamustine in the initial therapy of CLL patients: the phase 1b GALTON trial. Blood. 2015;125:2779–85. doi:10.1182/blood-2014-12-613570.
- Geisler CH, van T' Veer MB, Jurlander J, Walewski J, Tjonnfjord G, Itala Remes M, Kimby E, Kozak T, Polliack A, Wu KL, et al. Frontline low-dose alemtuzumab with fludarabine and cyclophosphamide prolongs progression-free survival in high-risk CLL. Blood. 2014;123:3255–62. doi:10.1182/blood-2014-01-547737.
- Derosa L, Galli L, Orlandi P, Fioravanti A, Di Desidero T, Fontana A, Antonuzzo A, Biasco E, Farnesi A, Marconcini R, et al. Docetaxel plus oral metronomic cyclophosphamide: a phase II study with pharmacodynamic and pharmacogenetic analyses in castration-resistant prostate cancer patients. Cancer. 2014;120:3923–31. doi:10.1002/cncr.28953.
- Abrisqueta P, Villamor N, Terol MJ, Gonzalez-Barca E, Gonzalez M, Ferra C, Abella E, Delgado J, García-Marco JA, González Y, et al. Rituximab maintenance after first-line therapy with rituximab, fludarabine, cyclophosphamide, and mitoxantrone (R-FCM) for chronic lymphocytic leukemia. Blood. 2013;122:3951–9. doi:10.1182/blood-2013-05-502773.
- Schiavoni G, Sistigu A, Valentini M, Mattei F, Sestili P, Spadaro F, Sanchez M, Lorenzi S, D'Urso MT, Belardelli F, et al. Cyclophosphamide synergizes with type I interferons through systemic dendritic cell reactivation and induction of immunogenic tumor apoptosis. Cancer Res. 2011;71:768–78. doi:10.1158/0008-5472.CAN-10-2788.
- Gulhati P, Raghav K, Shroff RT, Varadhachary GR, Kopetz S, Javle M, Qiao W, Wang H, Morris J, Wolff RA, et al. Bevacizumab combined with capecitabine and oxaliplatin in patients with advanced adenocarcinoma of the small bowel or ampulla of vater: A single-center, open-label, phase 2 study. Cancer. 2017;123:1011–7. doi:10.1002/cncr.30445.
- Meulendijks D, de Groot JW, Los M, Boers JE, Beerepoot LV, Polee MB, Beeker A, Portielje JE, Goey SH, de Jong RS, et al. Bevacizumab combined with docetaxel, oxaliplatin, and capecitabine, followed by maintenance with capecitabine and bevacizumab, as first-line treatment of patients with advanced HER2-negative gastric cancer: A multicenter phase 2 study. Cancer. 2016;122:1434–43. doi:10.1002/cncr.29864.
- Leone F, Marino D, Cereda S, Filippi R, Belli C, Spadi R, Nasti G, Montano M, Amatu A, Aprile G, et al. Panitumumab in combination with gemcitabine and oxaliplatin does not prolong survival in wild-type KRAS advanced biliary tract cancer: A randomized phase 2 trial (Vecti-BIL study). Cancer. 2016;122:574–81. doi:10.1002/cncr.29778.
- O'Reilly EM, Perelshteyn A, Jarnagin WR, Schattner M, Gerdes H, Capanu M, Tang LH, LaValle J, Winston C, DeMatteo RP, et al. A single-arm, nonrandomized phase II trial of neoadjuvant gemcitabine and oxaliplatin in patients with resectable pancreas adenocarcinoma. Ann Surg. 2014;260:142–8. doi:10.1097/SLA.0000000000000251.
- Leone F, Artale S, Marino D, Cagnazzo C, Cascinu S, Pinto C, Fornarini G, Tampellini M, Di Fabio F, Sartore-Bianchi A, et al. Panitumumab in combination with infusional oxaliplatin and oral capecitabine for conversion therapy in patients with colon cancer and advanced liver metastases. The MetaPan study. Cancer. 2013;119:3429–35. doi:10.1002/cncr.28223.
- Kim EJ, Ben-Josef E, Herman JM, Bekaii-Saab T, Dawson LA, Griffith KA, Francis IR, Greenson JK, Simeone DM, Lawrence TS, et al. A multi-institutional phase 2 study of neoadjuvant gemcitabine and oxaliplatin with radiation therapy in patients with pancreatic cancer. Cancer. 2013;119:2692–700. doi:10.1002/cncr.28117.
- Kidwell KM, Yothers G, Ganz PA, Land SR, Ko CY, Cecchini RS, Kopec JA, Wolmark N. Long-term neurotoxicity effects of oxaliplatin added to fluorouracil and leucovorin as adjuvant therapy for colon cancer: results from National Surgical Adjuvant Breast and Bowel Project trials C-07 and LTS-01. Cancer. 2012;118:5614–22. doi:10.1002/cncr.27593.
- Garg AD, Elsen S, Krysko DV, Vandenabeele P, de Witte P, Agostinis P. Resistance to anticancer vaccination effect is controlled by a cancer cell-autonomous phenotype that disrupts immunogenic phagocytic removal. Oncotarget. 2015;6:26841–60. doi:10.18632/oncotarget.4754.
- Hanoteau A, Moser M. Chemotherapy and immunotherapy: A close interplay to fight cancer? Oncoimmunology. 2016;5:e1190061. doi:10.1080/2162402X.2016.1190061.
- Dewan MZ, Galloway AE, Kawashima N, Dewyngaert JK, Babb JS, Formenti SC, Demaria S. Fractionated but not single-dose radiotherapy induces an immune-mediated abscopal effect when combined with anti-CTLA-4 antibody. Clin Cancer Res. 2009;15:5379–88. doi:10.1158/1078-0432.CCR-09-0265.
- Bouquet F, Pal A, Pilones KA, Demaria S, Hann B, Akhurst RJ, Babb JS, Lonning SM, DeWyngaert JK, Formenti SC, et al. TGFbeta1 inhibition increases the radiosensitivity of breast cancer cells in vitro and promotes tumor control by radiation in vivo. Clin Cancer Res. 2011;17:6754–65. doi:10.1158/1078-0432.CCR-11-0544.
- Wennerberg E, Lhuillier C, Vanpouille-Box C, Pilones KA, Garcia-Martinez E, Rudqvist NP, Formenti SC, Demaria S. Barriers to Radiation-Induced In Situ Tumor Vaccination. Front Immunol. 2017;8:229. doi:10.3389/fimmu.2017.00229.
- Veinalde R, Grossardt C, Hartmann L, Bourgeois-Daigneault MC, Bell JC, Jager D, von Kalle C, Ungerechts G, Engeland CE. Oncolytic measles virus encoding interleukin-12 mediates potent antitumor effects through T cell activation. Oncoimmunology. 2017;6:e1285992. doi:10.1080/2162402X.2017.1285992.
- Hemminki O, Hemminki A. A century of oncolysis evolves into oncolytic immunotherapy. Oncoimmunology. 2016;5:e1074377. doi:10.1080/2162402X.2015.1074377.
- Clements DR, Kim Y, Gujar SA, Lee PW. All that glitters is not gold: the need to consider desirable and undesirable immune aspects of oncolytic virus therapy. Oncoimmunology. 2016;5:e1057674. doi:10.1080/2162402X.2015.1057674.
- Bramante S, Koski A, Liikanen I, Vassilev L, Oksanen M, Siurala M, Heiskanen R, Hakonen T, Joensuu T, Kanerva A, et al. Oncolytic virotherapy for treatment of breast cancer, including triple-negative breast cancer. Oncoimmunology. 2016;5:e1078057. doi:10.1080/2162402X.2015.1078057.
- Kloos A, Woller N, Gerardy-Schahn R, Kuhnel F. Retargeted oncolytic viruses provoke tumor-directed T-cell responses. Oncoimmunology. 2015;4:e1052933. doi:10.1080/2162402X.2015.1052933.
- Pietrocola F, Pol J, Vacchelli E, Rao S, Enot DP, Baracco EE, Levesque S, Castoldi F, Jacquelot N, Yamazaki T, et al. Caloric Restriction Mimetics Enhance Anticancer Immunosurveillance. Cancer Cell. 2016;30:147–60. doi:10.1016/j.ccell.2016.05.016.
- Di Biase S, Lee C, Brandhorst S, Manes B, Buono R, Cheng CW, Cacciottolo M, Martin-Montalvo A, de Cabo R, Wei M, et al. Fasting-Mimicking Diet Reduces HO-1 to Promote T Cell-Mediated Tumor Cytotoxicity. Cancer Cell. 2016;30:136–46. doi:10.1016/j.ccell.2016.06.005.
- Galluzzi L, Kepp O, Chan FK, Kroemer G. Necroptosis: Mechanisms and Relevance to Disease. Annu Rev Pathol. 2017;12:103–30. doi:10.1146/annurev-pathol-052016-100247.
- Pasparakis M, Vandenabeele P. Necroptosis and its role in inflammation. Nature. 2015;517:311–20. doi:10.1038/nature14191.
- Weinlich R, Oberst A, Beere HM, Green DR. Necroptosis in development, inflammation and disease. Nat Rev Mol Cell Biol. 2017;18:127–36. doi:10.1038/nrm.2016.149.
- Yatim N, Jusforgues-Saklani H, Orozco S, Schulz O, Barreira da Silva R, Reis e Sousa C, Green DR, Oberst A, Albert ML. RIPK1 and NF-kappaB signaling in dying cells determines cross-priming of CD8(+) T cells. Science. 2015;350:328–34. doi:10.1126/science.aad0395.
- Yang H, Ma Y, Chen G, Zhou H, Yamazaki T, Klein C, Pietrocola F, Vacchelli E, Souquere S, Sauvat A, et al. Contribution of RIP3 and MLKL to immunogenic cell death signaling in cancer chemotherapy. Oncoimmunology. 2016;5:e1149673. doi:10.1080/2162402X.2016.1149673.
- Fend L, Yamazaki T, Remy C, Fahrner C, Gantzer M, Nourtier V, Préville X, Quéméneur E, Kepp O, Adam J, et al. Immune Checkpoint Blockade, Immunogenic Chemotherapy or IFN-alpha Blockade Boost the Local and Abscopal Effects of Oncolytic Virotherapy. Cancer Res. 2017;77:4146–57. doi:10.1158/0008-5472.CAN-16-2165 10.1158/1538-7445.AM2017-4563.
- Yang H, Yamazaki T, Pietrocola F, Zhou H, Zitvogel L, Ma Y, Kroemer G. STAT3 Inhibition Enhances the Therapeutic Efficacy of Immunogenic Chemotherapy by Stimulating Type 1 Interferon Production by Cancer Cells. Cancer Res. 2015;75:3812–22. doi:10.1158/0008-5472.CAN-15-1122.
- Pfirschke C, Engblom C, Rickelt S, Cortez-Retamozo V, Garris C, Pucci F, Yamazaki T, Poirier-Colame V, Newton A, Redouane Y, et al. Immunogenic Chemotherapy Sensitizes Tumors to Checkpoint Blockade Therapy. Immunity. 2016;44:343–54. doi:10.1016/j.immuni.2015.11.024.
- Stewart R, Morrow M, Hammond SA, Mulgrew K, Marcus D, Poon E, Watkins A, Mullins S, Chodorge M, Andrews J, et al. Identification and Characterization of MEDI4736, an Antagonistic Anti-PD-L1 Monoclonal Antibody. Cancer Immunol Res. 2015;3:1052–62. doi:10.1158/2326-6066.CIR-14-0191.
- Rios-Doria J, Durham N, Wetzel L, Rothstein R, Chesebrough J, Holoweckyj N, Zhao W, Leow CC, Hollingsworth R. Doxil synergizes with cancer immunotherapies to enhance antitumor responses in syngeneic mouse models. Neoplasia. 2015;17:661–70. doi:10.1016/j.neo.2015.08.004.
- Evans EE, Jonason AS Jr, Bussler H, Torno S, Veeraraghavan J, Reilly C, Doherty MA, Seils J, Winter LA, Mallow C, et al. Antibody Blockade of Semaphorin 4D Promotes Immune Infiltration into Tumor and Enhances Response to Other Immunomodulatory Therapies. Cancer Immunol Res. 2015;3:689–701. doi:10.1158/2326-6066.CIR-14-0171.
- Lopez-Soto A, Gonzalez S, Smyth MJ, Galluzzi L. Control of Metastasis by NK Cells. Cancer Cell. 2017;32:135–54. doi:10.1016/j.ccell.2017.06.009.
- Blake SJ, Stannard K, Liu J, Allen S, Yong MC, Mittal D, Aguilera AR Miles JJ, Lutzky VP, de Andrade LF, et al. Suppression of Metastases Using a New Lymphocyte Checkpoint Target for Cancer Immunotherapy. Cancer Discov. 2016;6:446–59. doi:10.1158/2159-8290.CD-15-0944.
- Musahl AS, Huang X, Rusakiewicz S, Ntini E, Marsico A, Kroemer G, Kepp O, Ørom UA. A long non-coding RNA links calreticulin-mediated immunogenic cell removal to RB1 transcription. Oncogene. 2015;34:5046–54. doi:10.1038/onc.2014.424.
- Colangelo T, Polcaro G, Ziccardi P, Muccillo L, Galgani M, Pucci B, Milone MR, Budillon A, Santopaolo M, Mazzoccoli G, et al. The miR-27 a-calreticulin axis affects drug-induced immunogenic cell death in human colorectal cancer cells. Cell Death Dis. 2016;7:e2108. doi:10.1038/cddis.2016.29.
- Lu A, Pallero MA, Lei W, Hong H, Yang Y, Suto MJ, Murphy-Ullrich JE. Inhibition of Transforming Growth Factor-beta Activation Diminishes Tumor Progression and Osteolytic Bone Disease in Mouse Models of Multiple Myeloma. Am J Pathol. 2016;186:678–90. doi:10.1016/j.ajpath.2015.11.003.
- Hsu FT, Chen TC, Chuang HY, Chang YF, Hwang JJ. Enhancement of adoptive T cell transfer with single low dose pretreatment of doxorubicin or paclitaxel in mice. Oncotarget. 2015;6:44134–50. doi:10.18632/oncotarget.6628.
- Iribarren K, Bloy N, Buque A, Cremer I, Eggermont A, Fridman WH, Fucikova J, Galon J, Špíšek R, Zitvogel L, et al. Trial Watch: Immunostimulation with Toll-like receptor agonists in cancer therapy. Oncoimmunology. 2016;5:e1088631. doi:10.1080/2162402X.2015.1088631.
- Koo JE, Shin SW, Um SH, Lee JY. X-shaped DNA potentiates therapeutic efficacy in colitis-associated colon cancer through dual activation of TLR9 and inflammasomes. Mol Cancer. 2015;14:104. doi:10.1186/s12943-015-0369-2.
- Monk BJ, Facciabene A, Brady WE, Aghajanian CA, Fracasso PM, Walker JL, Lankes HA, Manjarrez KL, Danet-Desnoyers GH, Bell-McGuinn KM, et al. Integrative Development of a TLR8 Agonist for Ovarian Cancer Chemoimmunotherapy. Clin Cancer Res. 2017;23:1955–66. doi:10.1158/1078-0432.CCR-16-1453.
- Buondonno I, Gazzano E, Jean SR, Audrito V, Kopecka J, Fanelli M, Salaroglio IC, Costamagna C, Roato I, Mungo E, et al. Mitochondria-Targeted Doxorubicin: A New Therapeutic Strategy against Doxorubicin-Resistant Osteosarcoma. Mol Cancer Ther. 2016;15:2640–52. doi:10.1158/1535-7163.MCT-16-0048.
- Shalapour S, Font-Burgada J, Di Caro G, Zhong Z, Sanchez-Lopez E, Dhar D, Willimsky G, Ammirante M, Strasner A, Hansel DE, et al. Immunosuppressive plasma cells impede T-cell-dependent immunogenic chemotherapy. Nature. 2015;521:94–8. doi:10.1038/nature14395.
- Thounaojam MC, Dudimah DF, Pellom ST Jr, Uzhachenko RV, Carbone DP, Dikov MM, Shanker A. Bortezomib enhances expression of effector molecules in anti-tumor CD8+ T lymphocytes by promoting Notch-nuclear factor-kappaB crosstalk. Oncotarget. 2015;6:32439–55. doi:10.18632/oncotarget.5857.
- Guillerey C, Ferrari de Andrade L, Vuckovic S, Miles K, Ngiow SF, Yong MC, Teng MW, Colonna M, Ritchie DS, Chesi M, et al. Immunosurveillance and therapy of multiple myeloma are CD226 dependent. J Clin Invest. 2015;125:2077–89. doi:10.1172/JCI82646 10.1172/JCI77181.
- Wong DY, Ong WW, Ang WH. Induction of immunogenic cell death by chemotherapeutic platinum complexes. Angew Chem Int Ed Engl. 2015;54:6483–7. doi:10.1002/anie.201500934.
- Ladoire S, Enot D, Andre F, Zitvogel L, Kroemer G. Immunogenic cell death-related biomarkers: Impact on the survival of breast cancer patients after adjuvant chemotherapy. Oncoimmunology. 2016;5:e1082706. doi:10.1080/2162402X.2015.1082706.
- Goswami S, Sharma P. Genetic biomarker for cancer immunotherapy. Science. 2017;357:358. doi:10.1126/science.aao1894.
- Loi S, Dushyanthen S, Beavis PA, Salgado R, Denkert C, Savas P, Combs S, Rimm DL, Giltnane JM, Estrada MV, et al. RAS/MAPK activation is associated with reduced tumor-infiltrating lymphocytes in triple-negative breast cancer: therapeutic cooperation between MEK and PD-1/PD-L1 immune checkpoint inhibitors. Clin Cancer Res. 2016;22:1499–509. doi:10.1158/1078-0432.CCR-15-1125.
- Cornelissen R, Hegmans JP, Maat AP, Kaijen-Lambers ME, Bezemer K, Hendriks RW, Hoogsteden HC, Aerts JG. Extended Tumor Control after Dendritic Cell Vaccination with Low-Dose Cyclophosphamide as Adjuvant Treatment in Patients with Malignant Pleural Mesothelioma. Am J Respir Crit Care Med. 2016;193:1023–31. doi:10.1164/rccm.201508-1573OC.
- Schijns VE, Pretto C, Devillers L, Pierre D, Hofman FM, Chen TC, Mespouille P, Hantos P, Glorieux P, Bota DA, et al. First clinical results of a personalized immunotherapeutic vaccine against recurrent, incompletely resected, treatment-resistant glioblastoma multiforme (GBM) tumors, based on combined allo- and auto-immune tumor reactivity. Vaccine. 2015;33:2690–6. doi:10.1016/j.vaccine.2015.03.095.
- Klein O, Davis ID, McArthur GA, Chen L, Haydon A, Parente P, Dimopoulos N, Jackson H, Xiao K, Maraskovsky E, et al. Low-dose cyclophosphamide enhances antigen-specific CD4(+) T cell responses to NY-ESO-1/ISCOMATRIX vaccine in patients with advanced melanoma. Cancer Immunol Immunother. 2015;64:507–18. doi:10.1007/s00262-015-1656-x.
- Murahashi M, Hijikata Y, Yamada K, Tanaka Y, Kishimoto J, Inoue H, Marumoto T, Takahashi A, Okazaki T, Takeda K, et al. Phase I clinical trial of a five-peptide cancer vaccine combined with cyclophosphamide in advanced solid tumors. Clin Immunol. 2016;166–67:48–58. doi:10.1016/j.clim.2016.03.015.
- Tanis E, Julie C, Emile JF, Mauer M, Nordlinger B, Aust D, Roth A, Lutz MP, Gruenberger T, Wrba F, et al. Prognostic impact of immune response in resectable colorectal liver metastases treated by surgery alone or surgery with perioperative FOLFOX in the randomised EORTC study 40983. Eur J Cancer. 2015;51:2708–17. doi:10.1016/j.ejca.2015.08.014.
- Ito K, Okamoto M, Inaguma Y, Okamoto A, Ando M, Ando Y, Tsuge M, Tomono A, Kakumae Y, Hayashi T, et al. Influence of R-CHOP Therapy on Immune System Restoration in Patients with B-Cell Lymphoma. Oncology. 2016;91:302–10. doi:10.1159/000449251.
- Brown PJ, Wong KK, Felce SL, Lyne L, Spearman H, Soilleux EJ, Pedersen LM, Møller MB, Green TM, Gascoyne DM, et al. FOXP1 suppresses immune response signatures and MHC class II expression in activated B-cell-like diffuse large B-cell lymphomas. Leukemia. 2016;30:605–16. doi:10.1038/leu.2015.299.
- Wu C, Wu X, Liu X, Yang P, Xu J, Chai Y, Guo Q, Wang Z, Zhang L. Prognostic Significance of Monocytes and Monocytic Myeloid-Derived Suppressor Cells in Diffuse Large B-Cell Lymphoma Treated with R-CHOP. Cell Physiol Biochem. 2016;39:521–30. doi:10.1159/000445644.
- Nalesnik MA, Jaffe R, Starzl TE, Demetris AJ, Porter K, Burnham JA, Makowka L, Ho M, Locker J. The pathology of posttransplant lymphoproliferative disorders occurring in the setting of cyclosporine A-prednisone immunosuppression. Am J Pathol. 1988;133:173–92.
- Galon J, Mlecnik B, Bindea G, Angell HK, Berger A, Lagorce C, Lugli A, Zlobec I, Hartmann A, Bifulco C, et al. Towards the introduction of the ‘Immunoscore’ in the classification of malignant tumours. J Pathol. 2014;232:199–209. doi:10.1002/path.4287.
- Anitei MG, Zeitoun G, Mlecnik B, Marliot F, Haicheur N, Todosi AM, Kirilovsky A, Lagorce C, Bindea G, Ferariu D, et al. Prognostic and predictive values of the immunoscore in patients with rectal cancer. Clin Cancer Res. 2014;20:1891–9. doi:10.1158/1078-0432.CCR-13-2830.
- Angell H, Galon J. From the immune contexture to the Immunoscore: the role of prognostic and predictive immune markers in cancer. Curr Opin Immunol. 2013;25:261–7. doi:10.1016/j.coi.2013.03.004.
- Blank CU, Haanen JB, Ribas A, Schumacher TN. CANCER IMMUNOLOGY. The “cancer immunogram”. Science. 2016;352:658–60.
- Van Allen EM, Miao D, Schilling B, Shukla SA, Blank C, Zimmer L, Sucker A, Hillen U, Foppen MHG, Goldinger SM, et al. Genomic correlates of response to CTLA-4 blockade in metastatic melanoma. Science. 2015;350:207–11. doi:10.1126/science.aad0095.
- Coulie PG, Van den Eynde BJ, van der Bruggen P, Boon T. Tumour antigens recognized by T lymphocytes: at the core of cancer immunotherapy. Nat Rev Cancer. 2014;14:135–46. doi:10.1038/nrc3670.
- Aachoui Y, Kajiwara Y, Leaf IA, Mao D, Ting JP, Coers J, Aderem A, Buxbaum JD, Miao EA. Canonical Inflammasomes Drive IFN-gamma to Prime Caspase-11 in Defense against a Cytosol-Invasive Bacterium. Cell Host Microbe. 2015;18:320–32. doi:10.1016/j.chom.2015.07.016.
- Wang J, Cao Z, Zhang XM, Nakamura M, Sun M, Hartman J, Harris RA, Sun Y, Cao Y. Novel mechanism of macrophage-mediated metastasis revealed in a zebrafish model of tumor development. Cancer Res. 2015;75:306–15. doi:10.1158/0008-5472.CAN-14-2819.
- Jaco I, Annibaldi A, Lalaoui N, Wilson R, Tenev T, Laurien L, Kim C, Jamal K, Wicky John S, Liccardi G, et al. MK2 Phosphorylates RIPK1 to Prevent TNF-Induced Cell Death. Mol Cell. 2017;66:698–710 e5. doi:10.1016/j.molcel.2017.05.003.
- Lan P, Fan Y, Zhao Y, Lou X, Monsour HP, Zhang X, Choi Y, Dou Y, Ishii N, Ghobrial RM, et al. TNF superfamily receptor OX40 triggers invariant NKT cell pyroptosis and liver injury. J Clin Invest. 2017;127:2222–34. doi:10.1172/JCI91075.
- Ting AT, Bertrand MJ. More to Life than NF-kappaB in TNFR1 Signaling. Trends Immunol. 2016;37:535–45. doi:10.1016/j.it.2016.06.002.
- Johanns TM, Ward JP, Miller CA, Wilson C, Kobayashi DK, Bender D, Fu Y, Alexandrov A, Mardis ER, Artyomov MN, et al. Endogenous Neoantigen-Specific CD8 T Cells Identified in Two Glioblastoma Models Using a Cancer Immunogenomics Approach. Cancer Immunol Res. 2016;4:1007–15. doi:10.1158/2326-6066.CIR-16-0156.
- de Vries IJ, Lesterhuis WJ, Scharenborg NM, Engelen LP, Ruiter DJ, Gerritsen MJ, Croockewit S, Britten CM, Torensma R, Adema GJ, et al. Maturation of dendritic cells is a prerequisite for inducing immune responses in advanced melanoma patients. Clin Cancer Res. 2003;9:5091–100.
- Chabanon RM, Pedrero M, Lefebvre C, Marabelle A, Soria JC, Postel-Vinay S. Mutational Landscape and Sensitivity to Immune Checkpoint Blockers. Clin Cancer Res. 2016;22:4309–21. doi:10.1158/1078-0432.CCR-16-0903.
- Braun DA, Burke KP, Van Allen EM. Genomic Approaches to Understanding Response and Resistance to Immunotherapy. Clin Cancer Res. 2016;22:5642–50. doi:10.1158/1078-0432.CCR-16-0066.
- Gfeller D, Bassani-Sternberg M, Schmidt J, Luescher IF. Current tools for predicting cancer-specific T cell immunity. Oncoimmunology. 2016;5:e1177691. doi:10.1080/2162402X.2016.1177691.
- Schumacher TN, Schreiber RD. Neoantigens in cancer immunotherapy. Science. 2015;348:69–74. doi:10.1126/science.aaa4971.
- Yadav M, Jhunjhunwala S, Phung QT, Lupardus P, Tanguay J, Bumbaca S, Franci C, Cheung TK, Fritsche J, Weinschenk T, et al. Predicting immunogenic tumour mutations by combining mass spectrometry and exome sequencing. Nature. 2014;515:572–6. doi:10.1038/nature14001.
- Liikanen I, Koski A, Merisalo-Soikkeli M, Hemminki O, Oksanen M, Kairemo K, Joensuu T, Kanerva A, Hemminki A. Serum HMGB1 is a predictive and prognostic biomarker for oncolytic immunotherapy. Oncoimmunology. 2015;4:e989771. doi:10.4161/2162402X.2014.989771.
- Davoli T, Uno H, Wooten EC, Elledge SJ. Tumor aneuploidy correlates with markers of immune evasion and with reduced response to immunotherapy. Science. 2017;355:eaaf8399.
- Heine A, Held SAE, Schulte-Schrepping J, Wolff JFA, Klee K, Ulas T, Schmacke NA, Daecke SN, Riethausen K, Schultze JL, et al. Generation and functional characterization of MDSC-like cells. Oncoimmunology. 2017;6:e1295203. doi:10.1080/2162402X.2017.1295203.
- Gunaydin G, Kesikli SA, Guc D. Cancer associated fibroblasts have phenotypic and functional characteristics similar to the fibrocytes that represent a novel MDSC subset. Oncoimmunology. 2015;4:e1034918. doi:10.1080/2162402X.2015.1034918.
- Danelli L, Frossi B, Pucillo CE. Mast cell/MDSC a liaison immunosuppressive for tumor microenvironment. Oncoimmunology. 2015;4:e1001232. doi:10.1080/2162402X.2014.1001232.
- Ortner D, Tripp CH, Komenda K, Dubrac S, Zelger B, Hermann M, Doppler W, Tymoszuk PZ, Boon L, Clausen BE, et al. Langerhans cells and NK cells cooperate in the inhibition of chemical skin carcinogenesis. Oncoimmunology. 2017;6:e1260215. doi:10.1080/2162402X.2016.1260215.
- Semeraro M, Rusakiewicz S, Zitvogel L, Kroemer G. Natural killer cell mediated immunosurveillance of pediatric neuroblastoma. Oncoimmunology. 2015;4:e1042202. doi:10.1080/2162402X.2015.1042202.
- Vacchelli E, Semeraro M, Enot DP, Chaba K, Poirier Colame V, Dartigues P, Perier A, Villa I, Rusakiewicz S, Gronnier C, et al. Negative prognostic impact of regulatory T cell infiltration in surgically resected esophageal cancer post-radiochemotherapy. Oncotarget. 2015;6:20840–50. doi:10.18632/oncotarget.4428.
- Emadi A, Jones RJ, Brodsky RA. Cyclophosphamide and cancer: golden anniversary. Nat Rev Clin Oncol. 2009;6:638–47. doi:10.1038/nrclinonc.2009.146.
- Audia S, Nicolas A, Cathelin D, Larmonier N, Ferrand C, Foucher P, Fanton A, Bergoin E, Maynadie M, Arnould L, et al. Increase of CD4+ CD25+ regulatory T cells in the peripheral blood of patients with metastatic carcinoma: a Phase I clinical trial using cyclophosphamide and immunotherapy to eliminate CD4+ CD25+ T lymphocytes. Clin Exp Immunol. 2007;150:523–30. doi:10.1111/j.1365-2249.2007.03521.x.
- Ghiringhelli F, Menard C, Puig PE, Ladoire S, Roux S, Martin F, Solary E, Le Cesne A, Zitvogel L, Chauffert B. Metronomic cyclophosphamide regimen selectively depletes CD4+CD25+ regulatory T cells and restores T and NK effector functions in end stage cancer patients. Cancer Immunol Immunother. 2007;56:641–8. doi:10.1007/s00262-006-0225-8.
- Garg AD, Dudek-Peric AM, Agostinis P. Melphalan, Antimelanoma Immunity, and Inflammation–Response. Cancer Res. 2015;75:5400–1. doi:10.1158/0008-5472.CAN-15-2061.
- Hsu MS, Sedighim S, Wang T, Antonios JP, Everson RG, Tucker AM, Du L, Emerson R, Yusko E, Sanders C, et al. TCR Sequencing Can Identify and Track Glioma-Infiltrating T Cells after DC Vaccination. Cancer Immunol Res. 2016;4:412–8. doi:10.1158/2326-6066.CIR-15-0240.
- Lim WA, June CH. The Principles of Engineering Immune Cells to Treat Cancer. Cell. 2017;168:724–40. doi:10.1016/j.cell.2017.01.016.
- Topalian SL, Wolchok JD, Chan TA, Mellman I, Palucka K, Banchereau J, Rosenberg SA, Dane Wittrup K. Immunotherapy: The path to win the war on cancer? Cell. 2015;161:185–6. doi:10.1016/j.cell.2015.03.045.
- Galluzzi L, Baehrecke EH, Ballabio A, Boya P, Bravo-San Pedro JM, Cecconi F, Choi AM, Chu CT, Codogno P, Colombo MI, et al. Molecular definitions of autophagy and related processes. EMBO J. 2017;36:1811–36. doi:10.15252/embj.201796697.
- Galluzzi L, Bravo-San Pedro JM, Levine B, Green DR, Kroemer G. Pharmacological modulation of autophagy: therapeutic potential and persisting obstacles. Nat Rev Drug Discov. 2017;16:487–511. doi:10.1038/nrd.2017.22.
- Garon EB, Rizvi NA, Hui R, Leighl N, Balmanoukian AS, Eder JP, Patnaik A, Aggarwal C, Gubens M, Horn L, et al. Pembrolizumab for the treatment of non-small-cell lung cancer. N Engl J Med. 2015;372:2018–28. doi:10.1056/NEJMoa1501824.
- Kourie HR, Awada G, Awada AH. Learning from the “tsunami” of immune checkpoint inhibitors in 2015. Crit Rev Oncol Hematol. 2016;101:213–20. doi:10.1016/j.critrevonc.2016.03.017.
- Opitz CA, Litzenburger UM, Opitz U, Sahm F, Ochs K, Lutz C, Wick W, Platten M. The indoleamine-2,3-dioxygenase (IDO) inhibitor 1-methyl-D-tryptophan upregulates IDO1 in human cancer cells. PLoS One. 2011;6:e19823. doi:10.1371/journal.pone.0019823.
- Kakarla S, Gottschalk S. CAR T cells for solid tumors: armed and ready to go? Cancer J. 2014;20:151–5. doi:10.1097/PPO.0000000000000032.
- Zhao T, Ren H, Wang X, Liu P, Yan F, Jiang W, Li Y, Li J, Gribben JG, Jia L, et al. Rituximab-induced HMGB1 release is associated with inhibition of STAT3 activity in human diffuse large B-cell lymphoma. Oncotarget. 2015;6:27816–31. doi:10.18632/oncotarget.4816.
- Reardon DA, De Groot JF, Colman H, Jordan JT, Daras M, Clarke JL, Nghiemphu PL, Gaffey SC, Peters KB, et al. Safety of pembrolizumab in combination with bevacizumab in recurrent glioblastoma (rGBM). J Clin Oncol. 2016;34: URL: http://meetinglibrary.asco.org/content/163977-176.
- Shi Y, Fan X, Deng H, Brezski RJ, Rycyzyn M, Jordan RE, Strohl WR, Zou Q, Zhang N, An Z. Trastuzumab triggers phagocytic killing of high HER2 cancer cells in vitro and in vivo by interaction with Fcgamma receptors on macrophages. J Immunol (Baltimore, Md: 1950). 2015;194:4379–86. doi:10.4049/jimmunol.1402891.
- Schreibelt G, Bol KF, Westdorp H, Wimmers F, Aarntzen EH, Duiveman-de Boer T, van de Rakt MW, Scharenborg NM, de Boer AJ, Pots JM, et al. Effective Clinical Responses in Metastatic Melanoma Patients after Vaccination with Primary Myeloid Dendritic Cells. Clin Cancer Res. 2016;22:2155–66. doi:10.1158/1078-0432.CCR-15-2205.
- Delamarre L, Mellman I, Yadav M. Cancer immunotherapy. Neo approaches to cancer vaccines. Science. 2015;348:760–1.
- Hardcastle J, Mills L, Malo CS, Jin F, Kurokawa C, Geekiyanage H, Schroeder M, Sarkaria J, Johnson AJ, Galanis E. Immunovirotherapy with measles virus strains in combination with anti-PD-1 antibody blockade enhances antitumor activity in glioblastoma treatment. Neuro-Oncol. 2017;19:493–502.
- Vassilev L, Ranki T, Joensuu T, Jager E, Karbach J, Wahle C, Partanen K, Kairemo K, Alanko T, Turkki R, et al. Repeated intratumoral administration of ONCOS-102 leads to systemic antitumor CD8+ T-cell response and robust cellular and transcriptional immune activation at tumor site in a patient with ovarian cancer. Oncoimmunology. 2015;4:e1017702. doi:10.1080/2162402X.2015.1017702.
- Koks CA, Garg AD, Ehrhardt M, Riva M, Vandenberk L, Boon L, De Vleeschouwer S, Agostinis P, Graf N, Van Gool SW. Newcastle disease virotherapy induces long-term survival and tumor-specific immune memory in orthotopic glioma through the induction of immunogenic cell death. Int J Cancer. 2015;136:E313–25. doi:10.1002/ijc.29202.
- Zamarin D, Holmgaard RB, Subudhi SK, Park JS, Mansour M, Palese P, Merghoub T, Wolchok JD, Allison JP. Localized oncolytic virotherapy overcomes systemic tumor resistance to immune checkpoint blockade immunotherapy. Sci Transl Med. 2014;6:226ra32. doi:10.1126/scitranslmed.3008095.
- Zhou L, Xu L, Chen L, Fu Q, Liu Z, Chang Y, Lin Z, Xu J. Tumor-infiltrating neutrophils predict benefit from adjuvant chemotherapy in patients with muscle invasive bladder cancer. Oncoimmunology. 2017;6:e1293211. doi:10.1080/2162402X.2017.1293211.
- Zhang Y, Mei Q, Liu Y, Li X, Brock MV, Chen M, Dong L, Shi L, Wang Y, Guo M, et al. The safety, efficacy, and treatment outcomes of a combination of low-dose decitabine treatment in patients with recurrent ovarian cancer. Oncoimmunology. 2017;6:e1323619. doi:10.1080/2162402X.2017.1323619.
- Nikanjam M, Patel H, Kurzrock R. Dosing immunotherapy combinations: Analysis of 3,526 patients for toxicity and response patterns. Oncoimmunology. 2017;6:e1338997. doi:10.1080/2162402X.2017.1338997.
- Formenti SC, Golden EB, Goldberg JD, Li X, Taff J, Fenton-Kerimian MB, Chandrasekhar S, Demaria S, Novik Y. Results of a phase I-II study of adjuvant concurrent carboplatin and accelerated radiotherapy for triple negative breast cancer. Oncoimmunology. 2017;6:e1274479. doi:10.1080/2162402X.2016.1274479.
- Remark R, Lupo A, Alifano M, Biton J, Ouakrim H, Stefani A, Cremer I, Goc J, Régnard JF, Dieu-Nosjean MC, et al. Immune contexture and histological response after neoadjuvant chemotherapy predict clinical outcome of lung cancer patients. Oncoimmunology. 2016;5:e1255394. doi:10.1080/2162402X.2016.1255394.
- Lazzari C, Bulotta A, Ducceschi M, Vigano MG, Brioschi E, Corti F, Gianni L, Gregorc V. Historical Evolution of Second-Line Therapy in Non-Small Cell Lung Cancer. Front Med. 2017;4:4. doi:10.3389/fmed.2017.00004.
- Morano WF, Aggarwal A, Love P, Richard SD, Esquivel J, Bowne WB. Intraperitoneal immunotherapy: historical perspectives and modern therapy. Cancer Gene Ther. 2016;23:373–81. doi:10.1038/cgt.2016.49.
- Shekarian T, Valsesia-Wittmann S, Caux C, Marabelle A. Paradigm shift in oncology: targeting the immune system rather than cancer cells. Mutagenesis. 2015;30:205–11. doi:10.1093/mutage/geu073.
- Wu J, Waxman DJ. Metronomic cyclophosphamide eradicates large implanted GL261 gliomas by activating antitumor Cd8+ T-cell responses and immune memory. Oncoimmunology. 2015;4:e1005521. doi:10.1080/2162402X.2015.1005521.
- Kerbel RS, Shaked Y. The potential clinical promise of ‘multimodality’ metronomic chemotherapy revealed by preclinical studies of metastatic disease. Cancer Lett. 2017;400:293–304. doi:10.1016/j.canlet.2017.02.005.
- Chen YL, Chang MC, Cheng WF. Metronomic chemotherapy and immunotherapy in cancer treatment. Cancer Lett. 2017;400:282–92. doi:10.1016/j.canlet.2017.01.040.
- Weiss T, Weller M, Roth P. Immunological effects of chemotherapy and radiotherapy against brain tumors. Expert Rev Anticancer Ther. 2016;16:1087–94. doi:10.1080/14737140.2016.1229600.
- Cook AM, Lesterhuis WJ, Nowak AK, Lake RA. Chemotherapy and immunotherapy: mapping the road ahead. Curr Opin Immunol. 2016;39:23–9. doi:10.1016/j.coi.2015.12.003.
- Zhao XY, Zhao XS, Wang YT, Chen YH, Xu LP, Zhang XH, Han W, Chen H, Wang Y, Yan CH, et al. Prophylactic use of low-dose interleukin-2 and the clinical outcomes of hematopoietic stem cell transplantation: A randomized study. Oncoimmunology. 2016;5:e1250992. doi:10.1080/2162402X.2016.1250992.
- Garg AD, Agostinis P. Editorial: Immunogenic Cell Death in Cancer: From Benchside Research to Bedside Reality. Front Immunol. 2016;7:110. doi:10.3389/fimmu.2016.00110.
- Kikuchi M, Clump DA, Srivastava RM, Sun L, Zeng D, Diaz-Perez JA, Anderson CJ, Edwards WB, Ferris RL. Preclinical immunoPET/CT imaging using Zr-89-labeled anti-PD-L1 monoclonal antibody for assessing radiation-induced PD-L1 upregulation in head and neck cancer and melanoma. Oncoimmunology. 2017;6:e1329071. doi:10.1080/2162402X.2017.1329071.
- Dovedi SJ, Illidge TM. The antitumor immune response generated by fractionated radiation therapy may be limited by tumor cell adaptive resistance and can be circumvented by PD-L1 blockade. Oncoimmunology. 2015;4:e1016709. doi:10.1080/2162402X.2015.1016709.
- Borch TH, Engell-Noerregaard L, Zeeberg Iversen T, Ellebaek E, Met O, Hansen M, Andersen MH, Thor Straten P, Svane IM. mRNA-transfected dendritic cell vaccine in combination with metronomic cyclophosphamide as treatment for patients with advanced malignant melanoma. Oncoimmunology. 2016;5:e1207842. doi:10.1080/2162402X.2016.1207842.
- Berinstein NL, Karkada M, Oza AM, Odunsi K, Villella JA, Nemunaitis JJ, Morse MA, Pejovic T, Bentley J, Buyse M, et al. Survivin-targeted immunotherapy drives robust polyfunctional T cell generation and differentiation in advanced ovarian cancer patients. Oncoimmunology. 2015;4:e1026529. doi:10.1080/2162402X.2015.1026529.