ABSTRACT
As classical therapy method of advanced hepatocellular carcinoma (HCC) is not effective enough, HCC immunotherapy is a hot spot for research in recent years. Although in recent years, immune checkpoint inhibitors are focused in cancer therapy, vaccines and adoptive cell therapy (ACT), as traditional immunotherapy methods for HCC are still promising. We found that δ-Catenin might be a new tumor-associated antigen for HCC, for it could be upregulated as a stress associated protein under hypoxia and irradiation treatment. δ-Catenin peptide vaccines could inhibit the growth of subcutaneous hepatocellular tumors in vivo. According to our work, δ-Catenin peptide vaccines could stimulate the activation of cytotoxic T lymphocytes (CTLs) and enhance the infiltration of CD8+ T cells into tumors. Moreover, δ-Catenin peptide vaccines could enhance the secretion of IFN-γ and the killing of tumor cells by T cells. Mechanistically, δ-Catenin peptide vaccines, presented by antigen-presenting cells to T cells, could enhance the activation of T cells via MAPK/ERK signaling and the transcriptional factors Eomes and T-bet. Our research results indicate new potential peptide vaccines, which can be applied in clinical HCC therapy.
Introduction
Hepatocellular carcinoma (HCC) is one of the leading causes of cancer death.Citation1 For HCC at early stages, surgical therapies and radiofrequency ablation (RFA) can be applied. However, advanced HCC is hardly to surgery and usually resistant to chemotherapy or radiotherapy, resulting in a poor prognosis.Citation2 Therefore, researches on new therapy methods for HCC are still promising. HCC immunotherapy is a hot spot for research in recent years.
Tumor vaccines and adoptive cell therapy (ACT) are traditional immunotherapy for HCC.Citation3,Citation4 Apart from tumor whole lysates vaccines, peptide vaccines and DC vaccines are all included in tumor vaccines.Citation3,Citation5 Although DC vaccines and ACT show clinical benefits, the benefits are limited, because of the limited activation of tumor killing immune cells.Citation4,Citation6-Citation8 In recent years, immune checkpoint inhibitors, like anti-CTLA-4 and anti-PD-1 mAbs, are new immunotherapy methods for HCC.Citation2,Citation9 However, blockade of CTLA-4 and PD-1 can only enhance the activation of T cells non-specifically, for the activated T cells are not the subpopulations which respond to specific TAAs. Peptide vaccines are based on the amino acid sequences of tumor-associated antigens (TAAs), the vaccines can stimulate the activation of T cells which can recognize specific TAAs.Citation2,Citation5,Citation10 Peptide vaccines derived from hTERT, AFP and GPC3 have been applied to clinical trials, which can prolong the overall survival time of HCC patients.Citation2,Citation11,Citation12 However, only limited tumor-associated antigens (TAAs), hTERT, WT-1, AFP, GPC3, MAGE-A, SSX-2, NY-ESO-1 included, can be applied for peptide vaccines.Citation10-Citation14 Therefore, to find new TAAs for the choice of HCC immunotherapy might be meaningful.
δ-Catenin, coded by the gene CTNND2, has been reported to be overexpressed in various types of cancers, prostate, breast, lung and ovarian cancer included.Citation15-Citation18 It is a potential biomarker for cancer. CTNND2 is also overexpressed in HCC carcinoma tissues, compared with normal tissues.Citation19 We found that expression of CTNND2 increased when hepatoma cells underwent hypoxia or irradiation treatment. Thus, CTNND2 might be a gene regulating stress response and malignancy in HCC. We proposed the hypothesis that peptides derived from δ-Catenin can be TAAs.
We designed δ-Catenin peptide vaccines to treat mice with tumors formed by hepatoma cells. In cancer immunotherapy, tumor cells are major killed by CD8+IFN-γ+ cytotoxic T lymphocytes (CTLs) or nature killer cells (NKs). Helper T cells (Ths), like Th1, Th2 or Th17 can facilitate the killing functions. On the contrary, regulatory T cells (Tregs) can inhibit the killing functions.Citation20,Citation21 We found that δ-Catenin peptide vaccines could repress the growth of hepatocellular carcinoma via CD8+ IFN-γ+ T cell activation, which was through the ERK/MAPK signaling pathway. Our work indicates that δ-Catenin peptide vaccines immunization can potentially be a new therapy method for HCC therapy in the future.
Results
δ-Catenin may be a stress-induced factor and highly conserved for peptide vaccines design
Hypoxia is a stress factor for most solid tumors, HCC included.Citation22,Citation23 Hypoxia can induced the expression of HIF-1α (hypoxia inducible factor-1α) and its downstream target genes, resulting in tumor progression.Citation24 HIF-1α and its downstream genes have been focused as targets for tumor therapy.Citation24,Citation25 We found that the mRNA level of the gene Ctnnd2 increased when mice hepatoma cell line H22 was under hypoxia treatment (). Therefore, we came to the hypothesis that, δ-Catenin may be a stress-induced factor for cancer. Moreover, as mentioned above, Ctnnd2 coded δ-Catenin is a potential biomarker and therapy target for cancer.Citation15 We aimed to design peptide vaccines targeted δ-Catenin for HCC therapy. Irradiation is a conventional method for whole tumor cell lysates vaccines, for irradiation treatment may induce the expression of TAAs.Citation3,Citation26 We found that the expression of Ctnnd2 also increase when H22 was treated by 8 Gy ionization irradiation. Thus, peptide vaccines based on δ-Catenin amino acids sequence may be feasible. We designed two peptide vaccines designed based on mice δ-Catenin amino acids sequence in consideration of MHC-I binding activity and immunogenicity. The sequences of the two peptide vaccines are completely conserved in human and mice ().
Figure 1. δ-Catenin might be a potential target for designing cancer peptide vaccines. (A) H22 cells were treated by 2% O2 or 8 Gy ionization irradiation for 6 h. The mRNA levels of Ctnnd2 were examined by qRT-PCR. (B) The amino acid sequences of δ-Catenin were aligned with sequences of δ-Catenin peptide vaccines by Invitrogen AlignX.
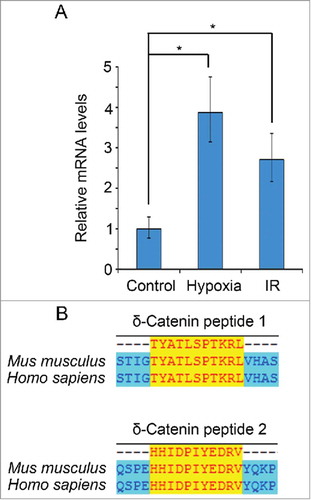
Treatment with δ-Catenin peptide vaccines inhibited the growth of HCC
Hepatoma cells, H22 and Hepa1-6, were injected into ICR mice and C57BL/6 mice subcutaneously to make tumors formation. Mice were immunized with δ-Catenin peptide vaccines subcutaneously once a week, for three times totally. The first time point of immunization was 24 h after tumor cells injection. Detailed information of the therapeutic models was in and . In ICR mice model, we found that H22 cells formed tumors with average volumes to about 2.5 cmCitation3 within 20 days. Peptide vaccine 1 and peptide vaccine 2 immunized separately could weakly inhibit tumor growth. However, combination immunization of peptide vaccine 1 and peptide vaccine 2 could significantly inhibit H22 tumor growth in ICR mice (). In C57 BL/6 mice model, Hepa1-6 cells formed tumors much slower than H22 cells. Hepa1-6 tumors grew to about 0.3 cmCitation3 within 20 days, but combination immunization of peptide vaccine 1 and peptide vaccine 2 could significantly inhibit Hepa1-6 tumor growth as well (). Mice were sacrificed at Day 24 to harvest tumors for further research. Tumor weight also decreased much after combination immunization of peptide vaccine 1 and peptide vaccine 2 ( and ). Photos of the tumors harvested were in and . Results above indicated that combination immunization of δ-Catenin peptide vaccine 1 and peptide vaccine 2 could make hepatoma regressed.
Figure 2. δ-Catenin peptide vaccines could inhibit the growth of subcutaneous tumors formed by liver cancer cells. (A-B) Experimental design of the therapeutic model for examining the functions of δ-Catenin peptide vaccines in vivo. (C) 5×105 H22 cells were injected subcutaneously into ICR mice. δ-Catenin peptide vaccine 1, peptide vaccine 2 or combined vaccines were immunized as shown in (A). Tumor sizes were measured and tumor volumes were calculated at the indicated time to draw the curves (*P < 0.05, **P < 0.01). (D) 5×105 Hepa1-6 cells were injected subcutaneously into C57 BL/6 mice. δ-Catenin peptide vaccine 1 and peptide vaccine 2 were combined to be immunized as shown in (B). Tumor sizes were measured and tumor volumes were calculated at the indicated time to draw the curves (*P < 0.05, **P < 0.01). (E) ICR mice were sacrificed at Day 24 as shown in (A). Tumor were harvested and tumor weights were measured (*P < 0.05, **P < 0.01). (F) C57 BL/6 mice were sacrificed at Day 24 as shown in (B). Tumor were harvested and tumor weights were measured (*P < 0.05, **P < 0.01). (G) ICR mice were sacrificed at Day 24 as shown in (A). Tumors harvested were shown. (H) C57 BL/6 mice were sacrificed at Day 24 as shown in (A). Tumors harvested were shown.
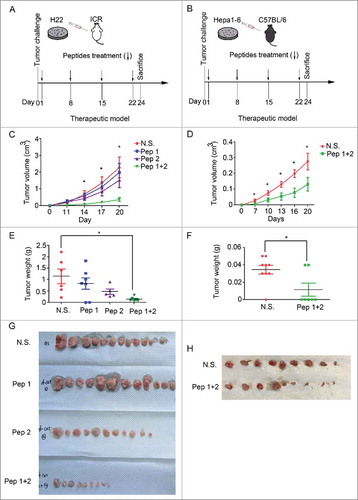
Immunization of δ-Catenin peptide vaccines resulted in increasing of CTLs in PB and tumor tissues
Tumor cells in vivo are majorly killed by CTLs or NKs. Ths can facilitate CTLs to improve the killing efficiency. Tregs and myeloid-derived suppressor cells (MDSCs), on the contrary, can inhibit the anti-tumor immunity response to enhance tumor growth and metastasis. Therefore, cancer immunotherapy aims to enhance the function of CTLs or inhibit the effects of Tregs or MDSCs.Citation20,Citation21 We found that the percentage of CD8+IFN-γ+ CTLs in peripheral blood increased when ICR tumor bearing mice were immunized with δ-Catenin peptide vaccines (). However, the percentage of CD8+IFN-γ+ CTLs in spleens was not changed (Sup 1 A-B). The same phenomena were seen in C57 BL/6 mice (, Sup 1 C-D). The concentration of IFN-γ secreted in tumor tissues was also increased when ICR tumor bearing mice were immunized with δ-Catenin peptide vaccines (). In C57 BL/6 tumor bearing mice, the concentration of IFN-γ secreted in tumor tissues was also increased if mice were immunized with δ-Catenin peptide vaccines (). In ICR and C57 BL/6 mice bearing mice, we could also find more CD8+ T cell infiltrated into tumor tissues when mice were immunized with δ-Catenin peptide vaccines (). What's more, when ICR tumor bearing mice were immunized with δ-Catenin peptide vaccines, the percentages of CD4+IFN-γ+ Th1 cells and CD4+CD25+FOXP3+ Treg cells in peripheral blood (Sup 2 A-B) and spleens (Sup 3 A-B) were not changed. Thus, immunization of δ-Catenin peptide vaccines mainly caused the increasing of CTLs to have the anti-tumor functions.
Figure 3. δ-Catenin peptide vaccines might stimulate the activation of CTLs in PB and the infiltration of CTLs in tumors to have the anti-tumor effect. (A-B) ICR mice were sacrificed at Day 24 as shown in . Peripheral blood was harvested. The percentages of CTLs were examined by flow cytometry. (C-D) C57 BL/6 mice were sacrificed at Day 24 as shown in . Peripheral blood was harvested. The percentages of CTLs were examined by flow cytometry. (E) ICR mice were sacrificed at Day 24 as shown in . Tumors were homogenized in PBS to be the lysates with a concentration of 10 mg/mL proteins. The concentration of IFN-γ in tumor lysates was measured by Elisa. (F) C57 BL/6 mice were sacrificed at Day 24 as shown in . Tumors were homogenized in PBS to be the lysates with a concentration of 10 mg/mL proteins. The concentration of IFN-γ in tumor lysates was measured by Elisa. (G-H) ICR mice were sacrificed at Day 24 as shown in . Tumors were harvested and the infiltration of CD8+ T cells was measured by immunofluorescence.
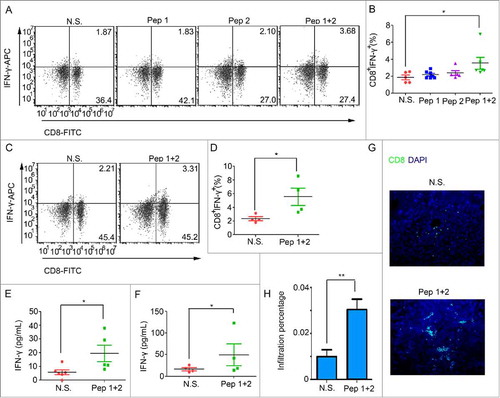
δ-Catenin peptide vaccines could stimulate the activation of CD8+ T cells to CTLs in vitro
T cell activation initiates with the TCR complex consisting of the variable αβ chains noncovalently associated with the non-polymorphic CD3 proteins.Citation27 We isolated lymphocytes from spleens and lymph nodes of normal ICR mice. Anti-CD3 antibody and IL-2 were added to non-specifically activate T cells in vitro. Anti-CD3 antibody could stimulate the proliferation of CD8+ T cells, but δ-Catenin peptide vaccines could not further enhance this process (Sup 4 A-B). Differently, δ-Catenin peptide vaccines could specific stimulate the increasing of CD8+IFN-γ+ CTLs but not non-activated CD8+ T cells in vitro (). Thus, δ-Catenin peptide vaccines specifically enhanced the activation of CD8+ T cells to CTLs, but did not enhance the proliferation of CD8+ T cells. In order to further confirm our results, we sorted CD3+ T cells and CD3− APCs from ICR lymphocytes to examine whether δ-Catenin peptides presented by APCs could activate CD8+IFN-γ+ CTLs. We found APCs non-pulsed with antigens could activate total CD8+ T cells. However, if APCs were pulsed with δ-Catenin peptides before co-cultured with T cells, the activation of CTLs increased (). The same results could be observed in lymphocytes form C57B/6 mice (, Sup 4C-D)). These results in vitro were consistent with the results in vivo.
Figure 4. δ-Catenin peptide vaccines could stimulate the activation of CTLs in vitro. (A-B) Lymphocytes from ICR mice were cultured in vitro with different treatment for 48 h. The percentages of CTLs were examined by flow cytometry. (C-D) CD3+ T cells and CD3− APCs from ICR mice were isolated by FACS. T cells and APCs were co-cultured with different treatment in vitro for 48 h. The percentages of CTLs were examined by flow cytometry. (E-F) Lymphocytes from C57 BL/6 mice were cultured in vitro with different treatment for 48 h. The percentages of CTLs were examined by flow cytometry. (G-H) CD3+ T cells and CD3− APCs from C57 BL/6 mice were isolated by FACS. T cells and APCs were co-cultured with different treatment in vitro for 48 h. The percentages of CTLs were examined by flow cytometry.
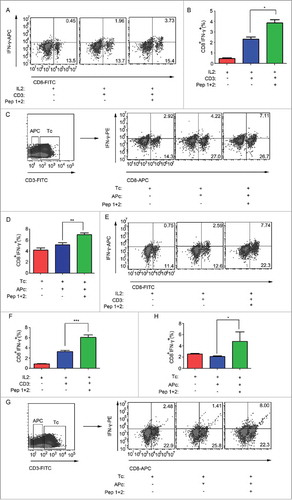
δ-Catenin peptide vaccines enhanced the killing of tumor cells by T cells in vitro
IFN-γ, granzyme B and perforin are usually secreted by CTLs to kill tumor cells to make tumors' regression.Citation21,Citation28 We found that δ-Catenin peptide vaccines could enhance the secretion of IFN-γ by lymphocytes in ICR mice (). We sorted CD3+ T cells and CD3− APCs from ICR mice lymphocytes. We found that if T cells were co-cultured with APCs pulsed by δ-Catenin peptides, the secretion of IFN-γ by T cells also increased (). Moreover, lymphocytes stimulated by δ-Catenin peptide vaccines had higher ability to kill H22 tumor cells (). The same results could be observed in lymphocytes form C57B/6 mice (). δ-Catenin peptide vaccines could enhance the secretion of IFN-γ by T cells from C57B/6 mice and enhance lymphocytes from C57B/6 mice to kill Hepa1-6 tumor cells.
Figure 5. δ-Catenin peptide vaccines could enhance the killing activity of T cells in vitro. (A) Lymphocytes from ICR mice were cultured in vitro with different treatment for 48 h. The concentration of IFN-γ in supernatant was measured by Elisa. (B) CD3+ T cells and CD3- APCs from ICR mice were isolated by FACS for 48 h. T cells and APCs were co-cultured with different treatment in vitro. The concentration of IFN-γ in supernatant was measured by Elisa. (C) Lymphocytes from ICR mice were cultured in vitro with different treatment for 48 h. Then lymphocytes were co-cultured with H22 cells as the ratio 1:1, 5:1, 10:1, 25:1 and 50:1 for 4 h. The cell killing efficiency of lymphocytes was measured by examining the relative LDH released levels in the supernatant. (D) Lymphocytes from C57 BL/6 mice were cultured in vitro with different treatment for 48 h. The concentration of IFN-γ in supernatant was measured by Elisa. (E) CD3+ T cells and CD3- APCs from C57 BL/6 mice were isolated by FACS for 48 h. T cells and APCs were co-cultured with different treatment in vitro. The concentration of IFN-γ in supernatant was measured by Elisa. (F) Lymphocytes from C57 BL/6 mice were cultured in vitro with different treatment for 48 h. Then lymphocytes were co-cultured with Hepa1-6 cells as the ratio 1:1, 5:1, 10:1, 25:1 and 50:1 for 4 h. The cell killing efficiency of lymphocytes was measured by examining the relative LDH released levels in the supernatant.
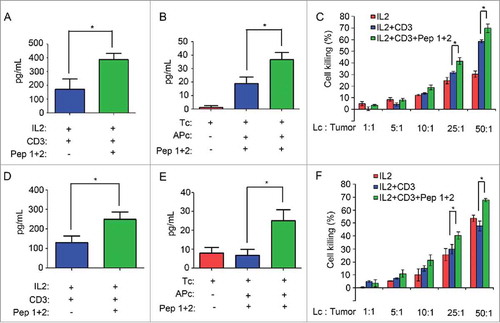
δ-Catenin peptide vaccines enhanced the activation of CTLs via ERK signaling
PI3K-Akt signaling and MAPK/ERK signaling have been reported play significant roles in the activation of CD8+ T cells.Citation29,Citation30 We found that in CD8+ T cells, anti-CD3 antibody could stimulate both Akt and ERK signaling. However, δ-Catenin peptides treatment could only stimulate ERK signaling to further activate the differentiation of CTLs both in CD8+ T cells from ICR and C57B/6 mice (, ). T-bet and eomesodermin are the key transcriptional factors for the differentiation of CD8+ T cells to effector or memory T cells.Citation31-Citation33 δ-Catenin peptides treatment could induce the upregulation of both T-bet and Eomes in CD8+ T cells from ICR mice (). However, the peptide vaccines could only upregulate Eomes in CD8+ T cells from C57B/6 mice (). According the results above, δ-Catenin peptides presented to CD8+ T cells, probably activate anti-tumor ability of immune system through activation of ERK signaling and the TF Eomes in CD8+ T cells.
Figure 6. δ-Catenin peptide vaccines enhanced the activation of CD8+ T cells via the activation of ERK signaling(A) Lymphocytes from ICR mice were cultured in vitro with different treatment for 1 h. Then the CD8+ T cells were isolated by FACS. (B) Cell lysates of CD8+ T cells from were indicated to immunoblotting. (C) Lymphocytes from ICR mice were cultured in vitro with different treatment for 4 h. Then the CD8+ T cells were isolated by FACS. The mRNA levels of T-bet were examined by qRT-PCR. (D) Lymphocytes from ICR mice were cultured in vitro with different treatment for 4 h. Then the CD8+ T cells were isolated by FACS. The mRNA levels of Eomes were examined by qRT-PCR. (E) Lymphocytes from C57 BL/6 mice were cultured in vitro with different treatment for 1 h. Then the CD8+ T cells were isolated by FACS. (F) Cell lysates of CD8+ T cells from were indicated to immunoblotting. (G) Lymphocytes from C57 BL/6 mice were cultured in vitro with different treatment for 4 h. Then the CD8+ T cells were isolated by FACS. The mRNA levels of T-bet were examined by qRT-PCR. (H) Lymphocytes from C57 BL/6 mice were cultured in vitro with different treatment for 4 h. Then the CD8+ T cells were isolated by FACS. The mRNA levels of Eomes were examined by qRT-PCR.
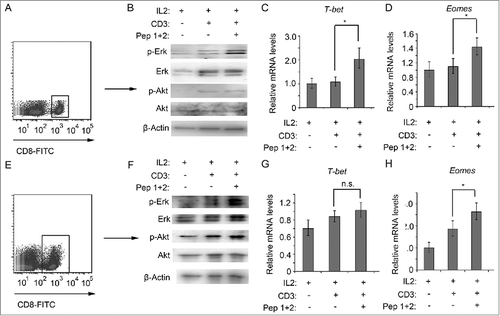
δ-Catenin peptide vaccines prolonged the survival time of mice after tumors were resected
Tumors formed by H22 cells could grow to 2–3 cm3 within 30 days. We tried to cut out the tumors at Day 7, however, the tumors relapsed with 7 days. We immunized the ICR mice with δ-Catenin peptide vaccines to research whether the vaccines could prolong the survival time of mice after relapse (). We found that mice immunized with δ-Catenin peptide vaccines could survive longer than control mice (). Therefore, δ-Catenin peptide vaccines perhaps both had therapy potentials for both newly diagnosed liver cancer patients but also relapsed patients.
Discussion
Hepatocellular carcinoma is one of the cancers with the poorest prognosis. HCC at late stages is usually resistant to chemotherapy or radiotherapy.Citation34 Exploring new adjuvant methods for HCC therapy, immunotherapy included, is a hot spot in recent years. Our research results indicate a new tumor-associated antigen, δ-Catenin, can be a HCC therapy target. δ-Catenin peptide vaccines immunization can reduce HCC tumor burdens in mice via the activation of CTLs, showing a promising therapy method for clinical application.
Tumor vaccines can trigger anti-tumor immune response with minimal toxicity.Citation3,Citation5,Citation35 Tumor vaccines immunization is one of active immunotherapy methods. Active immunotherapy is safe and cost-effective, compared with antibody drugs.Citation5,Citation36 However, tumor vaccines cannot be widely applied for clinical therapy due to its limited effects. Nowadays, researches for tumor vaccines are focused on developing personalized vaccines and finding neo-antigens as targets.Citation37 δ-Catenin has been reported to be a potential biomarker and therapy target for various cancers.Citation15 We find it may be also a neo-TAA for HCC. However, drugs or antibodies targeting δ-Catenin have not yet been developed. We firstly report the anti-tumor functions of peptide vaccines targeting δ-Catenin, which may be an economical and effective way to target δ-Catenin for HCC therapy.
According to our work, δ-Catenin peptide vaccines can stimulate the activation of CD8+ T cell to have the anti-tumor functions, but the vaccines have no effects on the Th1 cells and Treg cells. The immunity response induced by tumor vaccines may be complicated. Tumor vaccines majorly are presented by APCs to T cells, resulting in the activation of CTLs or Ths.Citation38 However, peptide vaccines have been reported to induce macrophages to infiltrate into tumors, which may contribute to tumor regression.Citation39 We also find δ-Catenin peptide vaccines have functions on the myeloid subpopulations. MDSCs have been known to promote cancer progression for years.Citation40 We also find that δ-Catenin peptide vaccines immunization make the percentages of granulocytic-MDSCs decreased in mice peripheral blood (data not shown). As the MDSC frequency is usually inversely correlated with T cell frequency in peripheral blood, we don't make too many efforts on this phenomenon.
Currently, the clinical application of tumor vaccines is limited, because of T cell exhaustion.Citation41,Citation42 Peptide vaccines can be recognized by CD8+ T cells to stimulate anti-tumor response. However, tumors can escape immune surveillance via T cell exhaustion or immunosuppressive cells.Citation43-Citation45 Immune checkpoint inhibitors, like anti-CTLA-4 or anti-PD-1 mAbs can rescue T cells from exhaustion. Therefore, tumor vaccines can be combined with immune checkpoint inhibitors to improve therapy effects.Citation46 We haven't checked the therapy effects of δ-Catenin peptide vaccines combined with anti-CTLA-4 or anti-PD-1 mAbs, which can be researched in the following projects.
Materials and methods
Antibodies and reagents
Antibodies were used in this study: anti-mouse-CD8a-FITC (eBioscience, 85-11-0081-82); anti-mouse-CD8-APC (eBioscience, 85-17-0081-81); anti-mouse-CD4-FITC (eBioscience, 85-11-0041-82); anti-mouse-CD4-APC (eBioscience, 85-17-0041-81); anti-mouse-CD25-FITC (eBioscience, 05-1595-02S); anti-mouse-IFN-γ-PE(eBioscience, 85-12-7311-82); anti-mouse-IFN-γ-APC(Biolegend,505810); anti-mouse-FOXP3-PE(eBioscience, 85-12-5773-80); anti-p-Erk (Cell Signaling Technology, 4370T); anti-Erk (Cell Signaling Technology, 4695T); anti-p-Akt (Cell Signaling Technology, 4060T); anti-Akt (Cell Signaling Technology, 9272S); anti-β-Actin (Sigma, A1978). Tris-HCl, NaCl and other chemicals were from Sigma.
Mice
ICR and C57 BL/6 mice used in this study were bred and maintained in a specific pathogen-free animal facility at Fujian Medical University. Mice are euthanized by cervical dislocation after anesthesia. All animal experiments were approved by the Animal Ethical Committee of Fujian Medical University.
Tumorigenesis
5 × 105 H22 or Hepa1-6 cells were injected subcutaneously into the abdomen of each ICR or C57 BL/6 mice to make the subcutaneous tumorigenesis mice model. Tumor sizes were measured by tumor length and width using clipper and then tumor volume was calculated using the formula V = (L × W × W)/2, where V is tumor volume, W is tumor width, L is tumor length.
Peptide vaccines
Peptide binding predictions were performed using IEDB (http://www.iedb.org/) and SYFPEITHI (http://www.syfpeithi.com/). Peptides were synthesized by ChinaPeptides.
Mice PB samples
Heparin anti-coagulated peripheral blood (PB) from mice tail veins was diluted 1:2 (vol:vol) in RPMI-1640 with 5%FBS. The diluted whole blood samples were stimulated by 25 ng/mL phorbol 12-myristate 13 acetate (PMA, Sigma, 79346) and 250 ng/mL ionomycin (Cell Signaling Technology, 9995) for 4 h in 37°C, 5% CO2. The samples were blocked by 10 mg/ml of brefeldin A (BFA, Cell Signaling Technology, 9972) for 2 h in 37°C, 5% CO2. After RBCs (red blood cells) were lysed by lysing solution for 5 min at room temperature, the white blood cells (WBCs) were washed with PBS. Then WBCs were subjected to immunostaining for flow cytometry.
Mice spleen samples
Spleens from mice were grinded in RPMI-1640 with 5%FBS to be single cells suspension. After RBCs were lysed, the lymphocytes suspension were stimulated by 25 ng/mL PMA and 250 ng/mL ionomycin for 4 h in 37°C, 5% CO2. Then The samples were blocked by 10 μg/ml of brefeldin A for 2 h in 37°C, 5% CO2, before subjected to immunostaining for flow cytometry.
Culture and activation of T cells in vitro
Mice spleens and lymph node were harvested to be grinded in RPMI-1640 with 5% FBS to be single cells suspension. After RBCs were lysed, the lymphocytes were resuspended in complete RPMI-1640 at 106/mL. 20 U/ml interleukin-2 (IL2, PeproTech, 200-02), 5 ug/mL anti-CD3 antibody and 100 ug/mL peptide vaccines were added as grouped.
CFSE-labeled lymphocyte proliferation
Lymphocytes were resuspended in 1 mL PBS with 2.5 uM CFSE. Lymphocytes dyed in CFSE solution were vortex in 37°C water bath for 10 min.Cells were washed with complete RPMI-1640 culture medium for twice. After cultured with cytokines stimulation for 48 h, lymphocytes were subjected to flow cytometry.
CTL killing assay in vitro
T cells activated by cytokines and antigens were harvested as effective cells. H22 and Hepa1-6 tumor cells were harvested as targeted cells. T cells and tumor cells were co-cultured as the ratio 1:1, 5:1, 10:1, 25:1 and 50:1 in 96-well plates for 4 h in 37°C, 5% CO2. Supernatant was collected for LDH release assay. LDH Release Assay Kit was from Beyotime.
Expansion of antigen-specific T cells
Mice spleens and lymph node were harvested to be grinded in RPMI-1640 with 5%FBS to be single cells suspension. After RBCs were lysed, the lymphocytes were resuspended in PBS with 2% FBS at 106/mL. After stained by anti-CD3-FITC antibody for 20 min at 4°C, the lymphocytes suspension was subjected to fluorescence-activated cell sorting (FACS, BD Biosciences) for CD3+ T cells and CD3− antigen-presenting cells (APCs). APCs were inactivated by 25 ug/mL Mitomycin C after pulsed with 100 ug/mL peptide vaccines or PBS for 30 min at 37°C. Then T cells and APCs were co-cultured as the ratio 5:1 in complete RPMI-1640 medium with 20 U/ml IL-2 and 5 ug/mL anti-CD3.
Immunostaining for flow cytometry
Lymphocytes samples were resuspeneded in PBS with 2% FBS. The surface molecules CD4, CD8 and CD25 were stained with antibodies for 30 min at 4°C. Then the lymphocytes were fixed with 4% paraformaldehyde (PFA) for 20 min at room temperature. IFN-γ and FOXP3 were stained in permeabilization buffer (0.2% saponin and 2% FBS in PBS) for 1 h at 4°C.
Flow cytometry
Flow cytometric analysis was performed using BD FACS C6 Flow Cytometer. The results were analyzed by the software FlowJo 7.6.1.
Immunofluorescence for tissues
The tissues are embedded in paraffin to be cut into 3 μm tissue sections. Tissue sections are dewaxed with xylene. Then sections were rehydrated with 100% −95% −75% alcohol gradients. After antigens were repaired in 0.01 M citrate buffer (Ph = 6.0) at 95°C for 15 min, the sections were stained with CD8-FITC antibody and DAPI overnight at 4°C. Then sections were mounted with anti-quencher (Beyotime, P0128).
Elisa assay
Tumor tissues were homogenized in PBS to be samples. All samples originated from tumors were quantified to the concentration 10 mg/mL by BCA assay. The BCA kit was from Byeotime (P0009). Samples from tumors or supernatant form T cell cultures were subjected to IFN-γ elisa assay (R&D, DY485-05).
Quantitative real-time PCR
Total cell RNA was isolated with TRIzol (Invitrogen), and cDNA was synthesized with Revertra Ace (Promega, Madison, USA). Real-time PCR was performed with an ABI QuantStudio 5 system. The expression level of genes was measured using the comparative Ct method. Expression values were normalized to β-Actin expression. The primer sequences were: T-bet: Forward: 5′-AACCGCTTATATGTCCACCCA-3′; Reverse: 5′-CTTGTTGTTGGTGAGCTTTAGC-3′. Eomes: Forward: 5′-GCGCATGTTTCCTTTCTTGAG-3′; Reverse: 5′-GGTCGGCCAGAACCACTTC-3′. β-Actin: Forward: 5′-ACCAACTGGGACGATATGGAGAAGA-3′; Reverse: 5′-TACGACCAGAGGCATACAGGGACAA-3′.
Immunoblotting
Cells were lysed with TNE buffer (10 mM Tris-HCl, 150 mM NaCl, 1 mM EDTA, 0.5% NP40, pH = 7.5). For immunoblotting assay, cell lysates were mixed with 4 × loading buffer (40 mM Tris-HCl, 200 mM DTT, 4% SDS, 40% Glycerol, 0.032% Bromophenol Blue, pH = 8.0). The samples were run with 4% stacking gel and 10% separating gels. Then proteins on the gels were transferred to nitrocellulose filter membranes for antibodies incubated. The membranes' exposure was done with thermo Pierce ECL and FluorChem E (ProteinSample).
Statistical analysis
The student's t-test, one-way anova test, wilcox rank sum test, log-rank test were used. P < 0.05 were considered statistically significant.
2018ONCOIMM0004R-s02.docx
Download MS Word (428 KB)Acknowledgment
We thank Felix Wu for the excellent and patient assistance.
Additional information
Funding
References
- Bruix J, Gores GJ, Mazzaferro V. Hepatocellular carcinoma: clinical frontiers and perspectives. Gut. 2014;63:844–55. PMID:24531850.
- Prieto J, Melero I, Sangro B. Immunological landscape and immunotherapy of hepatocellular carcinoma. Nat Rev Gastroenterol Hepatol. 2015;12:681–700. PMID:26484443.
- Chiang CL, Coukos G, Kandalaft LE. Whole Tumor Antigen Vaccines: Where Are We? Vaccines (Basel). 2015;3:344–72. PMID:26343191.
- Ma Y, Xu YC, Tang L, Zhang Z, Wang J, Wang HX. Cytokine-induced killer (CIK) cell therapy for patients with hepatocellular carcinoma: efficacy and safety. Exp Hematol Oncol. 2012;1:11. PMID:23210562.
- Parmiani G, Russo V, Maccalli C, Parolini D, Rizzo N, Maio M. Peptide-based vaccines for cancer therapy. Human vaccines & immunotherapeutics. 2014;10:3175–8.
- El Ansary M, Mogawer S, Elhamid SA, Alwakil S, Aboelkasem F, Sabaawy HE, Abdelhalim O. Immunotherapy by autologous dendritic cell vaccine in patients with advanced HCC. J Cancer Res Clin Oncol. 2013;139:39–48. PMID:22886490.
- Butterfield LH, Ribas A, Dissette VB, Lee Y, Yang JQ, De la Rocha P, Duran SD, Hernandez J, Seja E, Potter DM, et al. A phase I/II trial testing immunization of hepatocellular carcinoma patients with dendritic cells pulsed with four alpha-fetoprotein peptides. Clin Cancer Res. 2006;12:2817–25. PMID:16675576.
- Tada F, Abe M, Hirooka M, Ikeda Y, Hiasa Y, Lee Y, Jung NC, Lee WB, Lee HS, Bae YS, et al. Phase I/II study of immunotherapy using tumor antigen-pulsed dendritic cells in patients with hepatocellular carcinoma. Int J Oncol. 2012;41:1601–9. PMID:22971679.
- Chen Y, Ramjiawan RR, Reiberger T, Ng MR, Hato T, Huang Y, Ochiai H, Kitahara S, Unan EC, Reddy TP, et al. CXCR4 inhibition in tumor microenvironment facilitates anti-programmed death receptor-1 immunotherapy in sorafenib-treated hepatocellular carcinoma in mice. Hepatology. 2015;61:1591–602. PMID:25529917.
- Tagliamonte M, Petrizzo A, Tornesello ML, Ciliberto G, Buonaguro FM, Buonaguro L. Combinatorial immunotherapy strategies for hepatocellular carcinoma. Curr Opin Immunol. 2016;39:103–13. PMID:26851637.
- Greten TF, Forner A, Korangy F, N'Kontchou G, Barget N, Ayuso C, Ormandy LA, Manns MP, Beaugrand M, Bruix J, et al. A phase II open label trial evaluating safety and efficacy of a telomerase peptide vaccination in patients with advanced hepatocellular carcinoma. BMC Cancer. 2010;10:209. PMID:20478057.
- Sawada Y, Yoshikawa T, Nobuoka D, Shirakawa H, Kuronuma T, Motomura Y, Mizuno S, Ishii H, Nakachi K, Konishi M, et al. Phase I trial of a glypican-3-derived peptide vaccine for advanced hepatocellular carcinoma: immunologic evidence and potential for improving overall survival. Clin Cancer Res. 2012;18:3686–96. PMID:22577059.
- Mizejewski GJ. Alpha-fetoprotein (AFP)-derived peptides as epitopes for hepatoma immunotherapy: a commentary. Cancer Immunol Immunother. 2009;58:159–70. PMID:18612637.
- Gnjatic S, Nishikawa H, Jungbluth AA, Gure AO, Ritter G, Jager E, Knuth A, Chen YT, Old LJ. NY-ESO-1: review of an immunogenic tumor antigen. Adv Cancer Res. 2006;95:1–30. PMID:16860654.
- Lu Q, Lanford GW, Hong H, Chen YH. delta-Catenin as a potential cancer biomarker. Pathol Int. 2014;64:243–6. PMID:24888779.
- Dai SD, Wang Y, Zhang JY, Zhang D, Zhang PX, Jiang GY, Han Y, Zhang S, Cui QZ, Wang EH, et al. Upregulation of delta-catenin is associated with poor prognosis and enhances transcriptional activity through Kaiso in non-small-cell lung cancer. Cancer Sci. 2011;102:95–103. PMID:21070476.
- Fang Y, Li Z, Wang X, Zhang S. Expression and biological role of delta-catenin in human ovarian cancer. J Cancer Res Clin Oncol. 2012;138:1769–76. PMID:22699932.
- Huang F, Chen J, Wang Z, Lan R, Fu L, Zhang L. delta-Catenin promotes tumorigenesis and metastasis of lung adenocarcinoma. Oncology reports. 2018;39:809–17. PMID:29251319.
- Minami M, Daimon Y, Mori K, Takashima H, Nakajima T, Itoh Y, Okanoue T. Hepatitis B virus-related insertional mutagenesis in chronic hepatitis B patients as an early drastic genetic change leading to hepatocarcinogenesis. Oncogene. 2005;24:4340–8. PMID:15806150.
- Grivennikov SI, Greten FR, Karin M. Immunity, inflammation, and cancer. Cell. 2010;140:883–99. PMID:20303878.
- Owais M, Zubair S, Agrawal A, Chang YF. Cancer Immunology and Immunotherapy. Biomed Res Int. 2015;2015:393454. PMID:26199939.
- Vaupel P, Mayer A. Hypoxia in cancer: significance and impact on clinical outcome. Cancer Metastasis Rev. 2007;26:225–39. PMID:17440684.
- Wu XZ, Xie GR, Chen D. Hypoxia and hepatocellular carcinoma: The therapeutic target for hepatocellular carcinoma. J Gastroenterol Hepatol. 2007;22:1178–82. PMID:17559361.
- Semenza GL. Hypoxia-inducible factors: mediators of cancer progression and targets for cancer therapy. Trends Pharmacol Sci. 2012;33:207–14. PMID:22398146.
- Lin D, Wu J. Hypoxia inducible factor in hepatocellular carcinoma: A therapeutic target. World J Gastroenterol. 2015;21:12171–8. PMID:26576101.
- Lugade AA, Moran JP, Gerber SA, Rose RC, Frelinger JG, Lord EM. Local radiation therapy of B16 melanoma tumors increases the generation of tumor antigen-specific effector cells that traffic to the tumor. J Immunol. 2005;174:7516–23. PMID:15944250.
- Joyce JA, Fearon DT. T cell exclusion, immune privilege, and the tumor microenvironment. Science. 2015;348:74–80. PMID:25838376.
- Kammertoens T, Friese C, Arina A, Idel C, Briesemeister D, Rothe M, Ivanov A, Szymborska A, Patone G, Kunz S. Tumour ischaemia by interferon-gamma resembles physiological blood vessel regression. Nature. 2017;545:98–102. PMID:28445461.
- Zhang N, Bevan MJ. CD8(+) T cells: foot soldiers of the immune system. Immunity. 2011;35:161–8. PMID:21867926.
- Smith-Garvin JE, Koretzky GA, Jordan MS. T cell activation. Annu Rev Immunol. 2009;27:591–619. PMID:19132916.
- Yu B, Zhang K, Milner JJ, Toma C, Chen R, Scott-Browne JP, Pereira RM, Crotty S, Chang JT, Pipkin ME. Epigenetic landscapes reveal transcription factors that regulate CD8+ T cell differentiation. Nat Immunol. 2017;18:573–82. PMID:28288100.
- Pearce EL, Mullen AC, Martins GA, Krawczyk CM, Hutchins AS, Zediak VP, Banica M, DiCioccio CB, Gross DA, Mao CA, et al. Control of effector CD8+ T cell function by the transcription factor Eomesodermin. Science. 2003;302:1041–3. PMID:14605368.
- Joshi NS, Cui W, Chandele A, Lee HK, Urso DR, Hagman J, Gapin L, Kaech SM. Inflammation directs memory precursor and short-lived effector CD8(+) T cell fates via the graded expression of T-bet transcription factor. Immunity. 2007;27:281–95. PMID:17723218.
- Bruix J, Sherman M. American Association for the Study of Liver D. Management of hepatocellular carcinoma: an update. Hepatology. 2011;53:1020–2. PMID:21374666.
- Milani A, Sangiolo D, Montemurro F, Aglietta M, Valabrega G. Active immunotherapy in HER2 overexpressing breast cancer: current status and future perspectives. Ann Oncol. 2013;24:1740–8. PMID:23585514.
- Bachmann MF, Whitehead P. Active immunotherapy for chronic diseases. Vaccine. 2013;31:1777–84. PMID:23415932.
- Ophir E, Bobisse S, Coukos G, Harari A, Kandalaft LE. Personalized approaches to active immunotherapy in cancer. Biochim Biophys Acta. 2016;1865:72–82. PMID:26241169.
- Palucka K, Banchereau J. Cancer immunotherapy via dendritic cells. Nat Rev Cancer. 2012;12:265–77. PMID:22437871.
- van der Sluis TC, Sluijter M, van Duikeren S, West BL, Melief CJ, Arens R. Therapeutic Peptide Vaccine-Induced CD8 T Cells Strongly Modulate Intratumoral Macrophages Required for Tumor Regression. Cancer Immunol Res. 2015;3:1042–51. PMID:25888578.
- Talmadge JE, Gabrilovich DI. History of myeloid-derived suppressor cells. Nat Rev Cancer. 2013;13:739–52. PMID:24060865.
- Hailemichael Y, Dai Z, Jaffarzad N, Ye Y, Medina MA, Huang XF, Dorta-Estremera SM, Greeley NR, Nitti G, Peng W, et al. Persistent antigen at vaccination sites induces tumor-specific CD8(+) T cell sequestration, dysfunction and deletion. Nat Med. 2013;19:465–72. PMID:23455713.
- Gajewski TF, Schreiber H, Fu YX. Innate and adaptive immune cells in the tumor microenvironment. Nat Immunol. 2013;14:1014–22. PMID:24048123.
- Facciabene A, Motz GT, Coukos G. T-regulatory cells: key players in tumor immune escape and angiogenesis. Cancer Res. 2012;72:2162–71. PMID:22549946.
- Topalian SL, Hodi FS, Brahmer JR, Gettinger SN, Smith DC, McDermott DF, Powderly JD, Carvajal RD, Sosman JA, Atkins MB, et al. Safety, activity, and immune correlates of anti-PD-1 antibody in cancer. N Engl J Med. 2012;366:2443–54. PMID:22658127.
- Pardoll DM. The blockade of immune checkpoints in cancer immunotherapy. Nat Rev Cancer. 2012;12:252–64. PMID:22437870.
- Duraiswamy J, Freeman GJ, Coukos G. Dual blockade of PD-1 and CTLA-4 combined with tumor vaccine effectively restores T-cell rejection function in tumors-response. Cancer Res. 2014;74:633–4. discussion 5. PMID:24408920.