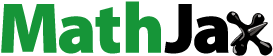
ABSTRACT
Glioblastoma multiforme (GBM) is the most common primary malignant brain tumor in adults with a dismal prognosis. We previously reported that vaccination with heat shock protein peptide complex-96 (HSPPC-96) improves survival in patients with newly diagnosed GBM (NCT02122822). Especially for patients with a strong antitumor immune response after vaccination, a durable survival benefit can be achieved. Here, we conducted T cell receptor (TCR) sequencing to retrospectively examine the TCR repertoires of tumor-infiltrating lymphocytes in long-term survivors (LTS) and short-term survivors (STS). We found that LTS exhibit lower TCR repertoire diversity compared with STS, indicating the prevalence of dominant TCR clones in LTS tumors. Accordingly, the LTS group showed increased inter-patient similarity, especially among high-frequency TCR clones, implying some of these dominant clones are shared among LTS. Indeed, we discovered four TCR clones significantly enriched in the LTS group: the presence of these clones has predictive value for stratifying patients prior to vaccination. Together, these findings uncover a group of preexisting TCR clones shared in LTS that can be utilized as candidate biomarkers to select GBM patients most likely to durably respond to HSPPC-96 treatment.
Introduction
Glioblastoma multiforme (GBM) is the most common and invasive primary malignant brain tumor in adults, with a dismal 3-y survival rate of just 9.9%.Citation1 The median overall survival is shorter than 15 months in patients with newly-diagnosed GBM receiving standard of care treatments including surgery, radiotherapy, and temozolomide-based chemotherapy.Citation2 Therefore, new therapeutic modalities are urgently needed for patients with this deadly cancer. As such, we previously conducted an open-label, single-arm, phase I clinical trial to evaluate the safety and efficacy of heat shock protein peptide complex-96 (HSPPC-96) vaccination in patients with newly diagnosed GBM. This clinical trial revealed a high tolerance to treatment and promising clinical efficacy in a cohort of 20 patients.Citation3
Despite these encouraging results, clinical outcomes were highly variable, as reflected by the wide time range of patient survival from 7.5 to 51.0 months.Citation3 We also observed significantly improved outcomes in patients with a tumor-specific immune response (TSIR) post-vaccination over the median level of all included patients. These patients appeared to achieve a durable survival with an average overall survival longer than 41.5 months.Citation3 Since HSPPC-96 immunotherapy is costly and time-consuming, it is beneficial to select potential durable responders prior to vaccination. However, the presence of a TSIR before vaccination was unable to predict a survival benefit.
Since the HSPPC-96 vaccine is constituted with a protein–peptide complex consisting of heat shock protein-gp96 (glycoprotein 96) and an array of gp96-associated peptides from tumor cells, we reasoned that the clinical efficacy of HSPCC-96 vaccination partly relies on boosting a preexisting T-cell response against these antigens produced by tumor cells.Citation4,Citation5 These tumor antigen-specific T cells are likely enriched but suppressed in untreated tumor tissue, and vaccination may stimulate the activation and tumor-infiltration of new waves of T cells for tumor cell elimination. Thus, we hypothesized that the precursor frequency of tumor antigen-specific T cells might reflect the activity of tumor-infiltrating T cells (TIL), as well as the overall capacity of patients to mount an anti-tumor response, which would ultimately correlate with the clinical outcome post HSPCC-96 vaccination. To test this, we profiled the TCR repertoire within pre-vaccine tumors using next-generation sequencing of T cell receptor β (TCR-β). This approach revealed remarkable differences in TCR diversity between HSPPC-96 vaccine long- and short-term survivors, such that these measurable characteristics of the TCR repertoire might effectively stratify patients suitable for HSPPC-96 vaccination against GBM.
Results
Updated survival analysis of the HSPPC-96 vaccinated GBM cohort
We continued our follow-up with all survivors approximately 1 y after our previous study.Citation3 Of the 20 vaccinated patients (19 of which had complete follow-up), 6 patients were alive with 2 of these progression-free until the last follow-up date of 15 April 2019 (). After a median follow-up of 58.9 months, progression-free survival (PFS) was 20% (4/20) and overall survival (OS) was 40% (8/20) at 3 y for all vaccinated patients. This 3-y OS rate was much higher than the 9.9% reported for all GBM patientsCitation1 or 16.0% for patients receiving standard-of-care postoperative chemo-radiation.Citation6 While half of living patients had follow-up times shorter than 5 y, the 5-y survival rate was still 15% (3/20), exceeding the 5.4%Citation1 or 9.8%Citation6 previously cited as 5-y survival rates for GBM. These findings corroborate the clinical efficacy of HSPCC-96 vaccination for treating newly diagnosed GBM as described in our previous study.Citation3
Figure 1. Updated survival analysis of patients with newly diagnosed GBM receiving HSPPC-96 vaccination. (a and b) Kaplan-Meier estimates of (a) progression-free survival and (b) overall survival in patients receiving HSPPC-96 vaccination in treating newly diagnosed GBM. (c and d) Based on the tumor-specific immune response (TSIR) after vaccination, patients were divided into a high TSIR after vaccination (post-vac) group (TSIR post-vac ≥ median) and a low TSIR after vaccination group (TSIR post-vac <median). Kaplan-Meier estimates of (c) progression-free survival and (d) overall survival in vaccinated GBM patients, stratified as high and low TSIR post-vac groups. Log-rank test was applied to estimate the difference. Vertical lines indicate censored time points. Dotted lines denote the 95% confidence interval
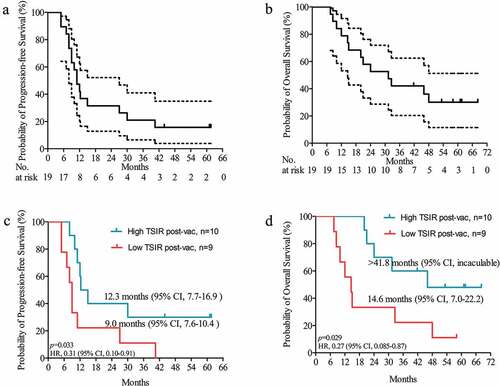
The clinical outcomes in these vaccinated patients notwithstanding were highly variable, such that OS ranged from 7.5 to 68.2 months. Thus, it remains critical to identify patients who will show a long-term survival benefit from HSPPC-96 vaccination. To explore this, we utilized an IFN-gamma release enzyme-linked immunospot (ELISPOT) assayCitation7 to measure the peripheral tumor-specific immune response (TSIR). This revealed a positive correlation between the post-vaccination TSIR with patient outcomes.Citation3 In our updated survival analysis, patients with high TSIR post-vaccination (≥median) had a 3-y OS of 60% (6/10), which is approximately threefold higher than in patients with low TSIR post-vaccination (<median; 22.2% (2/9); ). Therefore, the post-vaccination TSIR could represent a post-treatment indicator of a successful long-term response to HSPPC-96 vaccination.
Baseline clinical characteristics of long- and short-term survivors
Because HSPPC-96 immunotherapy is costly and time-consuming, the identification of baseline biomarkers capable of predicting patient response before the initiation of treatment is of central importance to optimize therapeutic efficacy and avoid unnecessary costs and undesirable side effects. However, the TSIR state before vaccination was insufficient to predict survival benefit (Supplemental Figure 1). Therefore, we sought to identify other variables that could potentially predict clinical outcomes prior to vaccination initiation. Because 3-y survival is a very common cutoff to define LTS in GBM clinical studies,Citation8–Citation11 we first stratified patients into long-term survivors (LTS, >3 y) and short-term survivors (STS, ≤3 y).
A total of 16 patients with available tumor tissues were selected for this study. The other four patients, including one lost to follow-up after vaccination (without survival data), another two LTS, and one in the STS group (without tumor tissues for TCR sequencing), were excluded from this study. Focusing on these 16 included patients, 6 patients were LTS and 10 patients were STS. Comparing these two groups, we did not observe any significant difference in epidemiological or clinical characteristics, including gender, age, and Karnofsky performance score (). Isocitrate dehydrogenase (IDH) mutations and O6-methylguanine-DNA methyltransferase (MGMT) promoter methylation are the most important genetic features correlating with favorable prognosis in GBM.Citation12 Consistent with this, the LTS group included two patients with IDH mutations and two patients with MGMT promoter methylation, although the prevalence of these features did not reach statistical significance between LTS and STS. We further examined TSIR-related indicators, including pre-vaccination TSIR, post-vaccination TSIR, ratio of post- to pre-vaccination TSIR, as well as absolute TSIR increase from pre-to post-vaccination, in each of the two groups. Although there was a trend that LTS exhibited higher TSIR states as measured by ELSPOT, this difference did not reach statistical significance (Supplemental Figure 2). Based on these results, we conclude that IDH mutations, MGMT promoter methylation, and pre-vaccination TSIR are insufficient to predict clinical responsiveness to HSPPC-96 vaccination in our GBM patient cohort.
Table 1. Characteristics of 16 patients with TCR-seq information
Long-term survivors exhibit decreased TCR repertoire diversity with enhanced inter-patient similarity
Since HSPCC-96 vaccination partly relies on boosting a preexisting T cell immune response,Citation4,Citation5 we hypothesized that LTS might exhibit distinct TIL activity within pre-vaccine tumors. To unbiasedly assess the T cell clonality difference between TILs from LTS and STS, we performed a comparative analysis of the TCR-β repertoire. Notably, this TCR analysis focused on complementarity determining region 3 (CDR3), which is the main region determining the antigen specificity of a given TCR.Citation13,Citation14
We used the Shannon entropy indexCitation15 to evaluate TCR diversity, with higher index scores indicating increased repertoire diversity. We observed that TCR repertoire diversity trended to decrease with OS (p = .051, )). That is, compared to STS, TCR repertoire diversity was lower in LTS ()), such that this index score alone could be used as an indicator to distinguish between LTS and STS with relatively high accuracy ()). Because a higher post-vaccination TSIR is associated with improved PFS and OS ()), we next evaluated whether this difference in TCR diversity was mediated by TSIR bias between LTS and STS. As shown in Supplemental , we did not observe any association between the post-vaccination TSIR and TCR diversity. Therefore, TCR repertoire diversity may inform extended survival from HSPCC-96 vaccination independently of post-vaccination TSIR status.
Figure 2. Long-term survivors exhibit lower TCR repertoire diversity with enhanced inter-patient similarity. TCR repertoire diversity was evaluated by Shannon entropy index of CDR3 in TCR-β. (a) Pearson correlation of CDR3 diversity with overall survival in 16 vaccinated patients with available tumor tissue for TCR-β sequencing; (b) Comparison of CDR3 diversity between long-term survivors (LTS, ≥3-y overall survival) and short-term survivors (STS, <3-y overall survival), Wilcoxon nonparametric test; (c) Receiver Operating Characteristic (ROC) curve analysis for evaluating the ability of CDR3 diversity to distinguish between LTS and STS; (d) Comparison of inter-patient similarity (evaluated by Jaccard index) in different top percent portions of TCR clonotype abundance between LTS and STS. Wilcoxon nonparametric test. *P < .05; **P < .01; ***P < .001, ****P < .0001
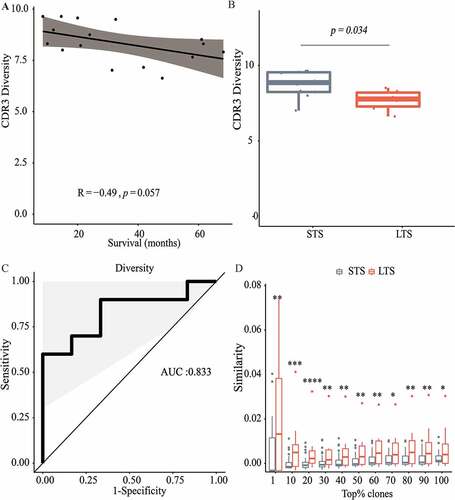
Figure 3. TCR clonotypes specific for long-term survivors. (a-d) CDR3-1 through CDR3-4 represents a higher abundance in LTS compared with STS. (e) Heat map showing the distribution of TSIR-related variables, common genetic features of gliomas, and CDR3 presence between LTS and STS. Variables with significantly higher prevalence in LTS than STS are in red. TSIR-related variables: TSIR_Abs. Increase, absolute TSIR increase from pre-to post-vaccination; TSIR_Ratio, ratio of post- to pre-vaccination TSIR; TSIR_pre-Vac, pre-vaccination TSIR; TSIR_post-Vac, post-vaccination TSIR. Red cells represent “≥ median level” and white cells indicate “< median level.” Genetic features: MGMT, O6-methylguanine-DNA methyltransferase promoter methylation; IDH, Isocitrate dehydrogenase mutations; TERT, Telomerase Reverse Transcriptase promoter mutations. Red cells represent “mutated” or “methylated” and white cells indicate “wild-type” or “un-methylated.” CDR3 s: red cells represent “presence” and white cells indicate “absence.” Categorical data were compared using Fisher’s exact test. Continuous data were compared using Wilcoxon nonparametric test
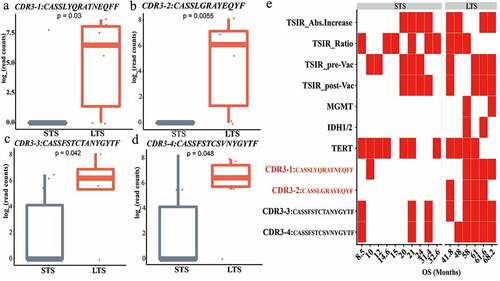
Low TCR diversity is indicative of a contracted TCR repertoire due to the diminishing of clonotypes or preferential expansion of certain dominant TCR clones.Citation16,Citation17 Our data analysis showed there is no significant difference in the number of unique clonotypes (Figure S4), which suggested the generation of a prevalent clone within the TIL population. We then asked whether public clonotypes can be identified across different patients within the response group. The sharing of high-frequency clones in different patients may indicate the sharing of common antigens eliciting this preferential expansion. For this, we applied a Jaccard indexCitation18 to quantify TCR repertoire inter-patient similarity within each group. Compared with the STS group, LTS exhibited significantly enhanced inter-patient similarity in all of the most abundant TCR clonotypes ()). Moreover, inter-patient similarity reduced gradually from the top 1% to top 20% TCR clonotypes in the LTS group alone, such that the highest level of sharing was observed among the most abundant TCR clonotypes ()). These findings indicate that certain preexisting dominant TCR clones are shared between GBM patients who will respond to immunotherapy.
TCR clonotypes enrich for long-term survivors
To explore TCR clones related to GBM patient survival benefit, we extracted shared TCR clonotypes in LTS and compared their abundances with those in STS by Wilcoxon nonparametric analysis. This revealed four TCR clones with a significantly higher abundance in LTS compared with STS ()). Regardless of the absolute abundance of these TCR clones, CDR3-1 and CDR3-2 had a significantly higher prevalence in LTS than in STS, further indicating the close association of these TCRs with clinical outcomes ()). Meanwhile, the occurrence of these TCRs was not significantly associated with other possible prognosticators, including TSIR-related variables and common GBM genetic features ()). In sum, these findings uncover a group of LTS-specific TCR clones that can independently predict clinical responsiveness to HSPCC-96 vaccination.
LTS-enriched TCR clonotypes predict prognosis
Most immunotherapies are capable of inducing durable survival in only a fraction of patients, as reflected by a plateau at the end of the OS curve.Citation19 To investigate whether LTS-specific TCR clones can predict durable survival, we first applied a log-rank analysis to estimate differences in OS between groups with the presence or absence of a specific TCR. Interestingly, the four dominant TCR clones shown to be enriched in LTS were all significantly correlated with improved patient survival (). Furthermore, we observed that patients harboring CDR3-1 or CDR3-2 exhibited classic durable survival curves (), indicating a prognostic value of these two TCR clones in recognizing durable survivors prior to vaccination. Indeed, as of the last follow-up date, 5 of 16 patients were still alive with a minimum OS of 41.8 months. If these survivors are categorized as durable survivors, 80% (4/5) of CDR3-1 carriers and 100% (4/4) of CDR3-2 carriers are durable survivors, values much higher than the corresponding percentages of non-carriers. Based on these results, we conclude that the pre-treatment presence of specific intratumoral TCR clones is capable of predicting a durable response to immunotherapy.
Figure 4. LTS-specific TCR clones predict durable survival. Kaplan-Meier estimates of overall survival in HSPPC-96 vaccinated GBM patients, grouped by the presence or absence of CDR3-1 (a), CDR3-2 (b), CDR3-3 (c), and CDR3-4 (d). Log-rank tests were applied to estimate differences. Vertical lines indicate censored time points
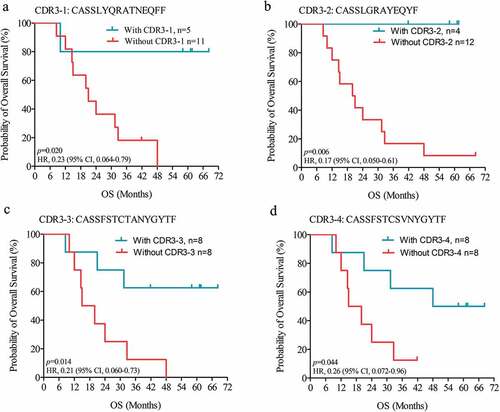
Discussion
During the last 5 y, immunotherapeutics such as CAR-T cells and checkpoint inhibitors have achieved remarkable success in treating several late-stage malignancies such as leukemia,Citation19 lymphoma,Citation20 advanced melanoma,Citation21 and non-small-cell lung cancer.Citation22 The prominent feature of these immunotherapies is that they induce durable survival in a fraction of treated patients, which is usually reflected by a plateau at the end of the OS curve.Citation19 These durable survivors are the patients who benefit most from a given immunotherapeutic. Thus, the identification of biomarkers capable of predicting these durable survivors in order to select patients suitable for treatment is critical for the optimal application of anti-cancer immunotherapy. Here, we uncovered a group of TCR clones capable of identifying durable survivors in HSPCC-96 vaccine-treated GBM patients. Specifically, 4 out of 5 patients with intratumoral CDR3-1 were durable survivors with minimum survival times exceeding 58 months, while all patients with intratumoral CDR3-2 exhibited a durable survival curve. Moreover, one CDR3 sequence (CDR3-2) was recurrently identified in peripheral lymphocytes from a glioma patient treated with a personalized peptide vaccine.Citation23 Interestingly, this patient had the longest survival of all patients treated with this peptide vaccine.Citation23 Therefore, these CDR3s appear to represent valuable biomarkers to select patients for appropriate clinical treatments.
HSPCC-96 is a personalized polyvalent vaccine that can trigger a specific antitumor responseCitation24,Citation25 as well as boost innate immune responseCitation24,Citation26 after being taken up by antigen-presenting cells. Previous studies have demonstrated its safety and preliminary clinical efficacy in treating recurrentCitation27,Citation28 and newly-diagnosed GBM.Citation3 Next-generation TCR sequencing has enabled investigators to obtain a comprehensive profile of the T cell response within the glioma microenvironment, thereby gaining deep insights into the underlying mechanisms of anti-cancer immunotherapy.Citation29 Our results show that LTS exhibit low TCR repertoire diversity, indicating some dominant TCR clones prevail in tumors from patients benefiting from this vaccine. A similar association between TCR repertoire diversity and clinical efficacy has been observed in other tumor types including bladder cancer,Citation30 pancreatic ductal adenocarcinoma,Citation31 and breast cancer.Citation32 This prevalence of dominant TCR clones likely originates from clonal expansion of T cells within the tumor.Citation31,Citation32 This implies that a preexisting T-cell-mediated immune response, reflected by local intratumoral TCR clonal expansion, would be a pre-requisite for successful treatment outcomes to these immunotherapies. In contrast, several studies have observed an opposite direction of the association between TCR repertoire diversity and treatment outcomes in other solid tumor types,Citation33,Citation34 underscoring the complex interactions between the immune system and advanced malignancies.Citation35 This complexity notwithstanding, these reports also suggest the direct measurement of TCR repertoire diversity within tumors may be utilized to predict clinical immunotherapeutic efficacy.
In this study, we focused on analyzing the TCR β-chain CDR3 repertoire to understand T cell immune responses within pre-vaccine GBM. Since CDR3 mainly determines the antigen specificity of a TCR clone,Citation13,Citation14 differences in the CDR3 repertoire likely represent differential distributions of tumor antigens between LTS and STS. We identified TCR clones shared by LTS that correlate with favorable outcomes. Based on these findings, we speculated that a group of antigens present in LTS tumors might trigger an antitumor immune response before treatment, which would be intensified following HSPPC-96 vaccination and thereby mediate a survival benefit. The future identification of these antigens and their corresponding TCR clones will greatly improve our understanding of T cell immunity in GBM, and more importantly, could facilitate the development of new immunotherapeutics targeting these antigens to improve GBM patient outcomes.
However, given the limited sample size and phase one design of our study, these results were obtained in a select set of patients, specifically in postoperative patients with a Karnofsky performance status of ≥70% and a relatively young median age.Citation3 Thus, these patients likely have increased general physical activity and less immune compromise as compared with others, limiting the extension of these results to all GBM patients. As such, the predictive potential of the TCR repertoire and related biomarkers for HSPCC-96 vaccination efficacy should be validated in another larger cohort, which ideally includes more diverse GBM patients receiving the vaccination.
In conclusion, we utilized TCR sequencing to reveal remarkable differences in the intratumoral TCR repertoire between LTS and STS. This approach uncovered a group of expanded T cell clones shared in GBM patients responding to immunotherapy. Therefore, we propose that these TCR sequences could be utilized as candidate biomarkers to predict a durable response to HSPPC-96 vaccination.
Materials and methods
Patients enrolled for TCR sequencing
We previously conducted an open-label, single-arm, phase I clinical trial to determine the safety and efficacy of treating newly-diagnosed GBM with HSPCC-96 vaccination combined with standard-of-care therapy.Citation3 The included patients received a total of six doses of the vaccine, which was derived from each patient’s own tumor tissue. The start of vaccination was concurrent with the beginning of adjunctive temozolomide chemotherapy. Vaccines were administered via subcutaneous injection in 25-μg doses every week for 6 weeks. Cyclophosphamide (400 mg) was given through intravenous injection before each injection.Citation3 The primary endpoint of this study was progression-free survival at 6 months and the frequency of adverse events. Secondary endpoints included overall survival and progression-free survival at the end of the study and tumor-specific immune response (TSIR) during the vaccinations.
This trial demonstrated a promising efficacy of HSPCC-96 vaccine in treating GBM.Citation3 A total of 20 patients with histologically confirmed GBM, who aged from 18 to 75 y, received a ≥80% microsurgical resection of a contrast-enhancing tumor and had a Karnofsky performance status of ≥70% before vaccination, were enrolled in the trial, whereas 19 patients with complete follow-up were available for clinical efficacy analysis. We initiated the present study to identify biomarkers capable of predicting durable survivors among these 19 patients; among those, 16 with available tumor tissues were finally included for TCR sequencing and analysis. Differences in clinical characteristics between the included 16 and excluded 3 patients were also analyzed. No statistical significance was observed between them. The clinical trial was approved by the ethics committee at Beijing Tiantan Hospital (JS2012-001-03) and was registered at ClinicalTrials.gov (NCT02122822) and http://www.chictr.org.cn/enindex.aspx (ChiCTR-ONC-13003309). Written informed consent was obtained from all participants.
Enzyme-linked immunospot (ELISPOT) assay
TSIR was evaluated by the number of stimulated peripheral blood mononuclear cells (PBMCs) in response to autologous tumor lysate in an IFN-γ release enzyme-linked immunospot (ELISPOT) assay, as previously reported.Citation3 Briefly, sterile 96-well polyvinylidene fluoride plates (Millipore Sigma) were coated with IFN-γ monoclonal antibody at 15 μg/ml (100 μl/well, clone 1-D1 K, Mabtech) for 2 h at 37°C and then blocked with complete media (200 μl/well, 10% fetal calf serum) for 1 h at 37°C. After removal of the complete media, autologous tumor lysate was added to each well at a concentration of 20 μg/ml. PMA (Millipore Sigma) was included as a positive control and wells without added tumor lysates or PMA was used as negative controls. IL-2 (Millipore Sigma) was also added to each well (50 IU/ml). A total of 3 × 105 PBMCs were added to each well and incubated for 16–24 h at 37°C. After washing, wells were incubated with 1 μg/ml biotinylated IFN-γ monoclonal antibody (100 μl/well, clone 7-B6-1-Biotin, Mabtech) for 2 h at room temperature. After washing, streptavidin alkaline phosphatase (1:1000 in PBS/0.5% FCS, Streptavidin-ALP, Mabtech) was added at 100 μl/well. Following a 1-h incubation at room temperature, the plates were washed and developed with 100 μl/well substrate (BCIP-NBT, Bio-Rad). Spots were counted using an ELISPOT reader (Cellular Technology Ltd.). Numbers of PBMCs responding to tumor lysate, as measured by IFN-γ release, were calculated by subtracting the background number of IFN-γ spots in the negative control well.
TCR library construction and TCR-β deep-sequencing
TCR library construction and repertoire sequencing were completed by Beijing TCRCure Co. Ltd. Briefly, RNA was extracted from frozen GBM tissues by Trizol Reagent RNeasy Kit (Thermo Fisher, USA). After determining RNA concentration and quality, extracted RNA (0.1–5 µg of total RNA) was subjected to reverse transcription by RevertAid First Strand cDNA Synthesis Kit (Thermo Fisher, USA). The TCR library was constructed by Multiplex PCR (Takara, Japan) to amplify the entire CDR3 region, in the presence of forward primers targeting V segments and reverse primers targeting the C region of the TCR-β locus. After purification and quality control using gel electrophoresis and a QIAGEN gel extraction kit (Germany), as well as concentration measurement with Qubit 2.0 (Thermo Fisher, USA), the same amount of each constructed library was mixed then subjected to emulsion PCR and deep-sequencing on the Ion Torrent PGM platform (Thermo Fisher, USA).
Raw data analysis
Raw data processing was performed by Beijing TCRCure according to standardized procedures, including data preprocessing, quality control, cleaning, and alignment to CDR3 regions (Torrent Suite software, Life Technologies). Numbers of CDR3 types and their frequencies were calculated using MiTCR.Citation36 CDR3 is a direct binding site within the TCR that recognizes a presented peptide, thereby determining antigen specificity for a given TCR clonotype.Citation13,Citation14 For simplicity, we equated a CDR3 sequence with an antigen-specific TCR clonotype in this study.
Diversity analysis
The Shannon entropy indexCitation15 was used to measure TCR repertoire diversity in the CDR3 region. This index (H) was calculated using the following equation:
where pi is the frequency of CDR3 clonotype i and N represents the total number of CDR3 clonotype for sample x.
Similarity analysis
Jaccard indexCitation18 was utilized to quantify TCR repertoire similarity between each pair of patients. This index value ranges from 0 to 1, representing being completely different to fully identical. The analysis was based on amino acid sequences within the CDR3 region.
Statistical analysis
Categorical data were compared utilizing Fisher’s exact test. Continuous data were compared using the 2-tailed Student’s t-test and Wilcoxon nonparametric test. Pearson correlation was applied to determine the association between CDR3 diversity and clinical survival. PFS and OS were estimated by the Kaplan–Meier method, and the difference between groups was assessed by the log-rank test. Statistical significance was set as a 2-tailed p value of less than 0.05. Statistical analysis of TCR diversity and similarity was calculated under the R statistic environment and relevant figures were generated using R “ggplot2” and “ggpubr” packages. All other statistics were analyzed using SPSS version 20 (IBM, USA) and figures were generated using GraphPad Prism 5 software (USA).
KPS: Karnofsky Performance Score; MGMT: O6-methylguanine-DNA methyltransferase; IDH: Isocitrate dehydrogenase
Disclosure of Potential Conflict of Interest
Qi-Jing Li is a scientific co-founder and shareholder of TCRCure Biopharma.
Supplemental Material
Download ()Supplementary material
Supplemental data for this article can be accessed on the publisher’s website.
Additional information
Funding
References
- Gittleman H, Boscia A, Ostrom QT, Truitt G, Fritz Y, Kruchko C, Barnholtz-Sloan JS. Survivorship in adults with malignant brain and other central nervous system tumor from 2000-2014. Neuro-oncology. 2018;20:vii6–9. doi:10.1093/neuonc/noy090.
- Stupp R, Mason WP, van den Bent MJ, Weller M, Fisher B, Taphoorn MJB, Belanger K, Brandes AA, Marosi C, Bogdahn U, et al. Radiotherapy plus concomitant and adjuvant temozolomide for glioblastoma. N Engl J Med. 2005;352(10):987–996. doi:10.1056/NEJMoa043330.
- Ji N, Zhang Y, Liu Y, Xie J, Wang Y, Hao S, Gao Z. Heat shock protein peptide complex-96 vaccination for newly diagnosed glioblastoma: a phase I, single-arm trial. JCI Insight. 2018;3(10). doi:10.1172/jci.insight.99145.
- Colaco CA, Bailey CR, Walker KB, Keeble J. Heat shock proteins: stimulators of innate and acquired immunity. Biomed Res Int. 2013;2013:461230. doi:10.1155/2013/461230.
- Srivastava PK, Amato RJ. Heat shock proteins: the ‘Swiss Army Knife’ vaccines against cancers and infectious agents. Vaccine. 2001;19(17–19):2590–2597. doi:10.1016/S0264-410X(00)00492-8.
- Stupp R, Hegi ME, Mason WP, van den Bent MJ, Taphoorn MJ, Janzer RC, Ludwin SK, Allgeier A, Fisher B, Belanger K, et al. Effects of radiotherapy with concomitant and adjuvant temozolomide versus radiotherapy alone on survival in glioblastoma in a randomised phase III study: 5-year analysis of the EORTC-NCIC trial. Lancet Oncol. 2009;10(5):459–466. doi:10.1016/S1470-2045(09)70025-7.
- Yan X, Zhang X, Wang Y, Li X, Wang S, Zhao B, Li Y, Ju Y, Chen L, Liu W, et al. Regulatory T-cell depletion synergizes with gp96-mediated cellular responses and antitumor activity. Cancer Immunol Immunother. 2011;60:1763–1774. doi:10.1007/s00262-011-1076-5.
- Krauze AV, Mackey M, Rowe L, Chang MG, Holdford DJ, Cooley T, Shih J, Tofilon PJ, Camphausen K. Late toxicity in long-term survivors from a phase 2 study of concurrent radiation therapy, temozolomide and valproic acid for newly diagnosed glioblastoma. Neurooncol Pract. 2018;5:246–250. doi:10.1093/nop/npy009.
- Cantero D, Rodríguez de Lope Á, Moreno de la Presa R, Sepúlveda JM, Borrás JM, Castresana JS, D’Haene N, García JF, Salmon I, Mollejo M, et al. Molecular study of long-term survivors of glioblastoma by gene-targeted next-generation sequencing. J Neuropathol Exp Neurol. 2018;77:710–716. doi:10.1093/jnen/nly048.
- Krex D, Klink B, Hartmann C, von Deimling A, Pietsch T, Simon M, Sabel M, Steinbach JP, Heese O, Reifenberger G, et al. Long-term survival with glioblastoma multiforme. Brain. 2007;130:2596–2606. doi:10.1093/brain/awm204.
- Michaelsen SR, Urup T, Olsen LR, Broholm H, Lassen U, Poulsen HS. Molecular profiling of short-term and long-term surviving patients identifies CD34 mRNA level as prognostic for glioblastoma survival. J Neurooncol. 2018;137:533–542. doi:10.1007/s11060-017-2739-7.
- Molenaar RJ, Verbaan D, Lamba S, Zanon C, Jeuken JWM, Boots-Sprenger SHE, Wesseling P, Hulsebos TJM, Troost D, van Tilborg AA, et al. The combination of IDH1 mutations and MGMT methylation status predicts survival in glioblastoma better than either IDH1 or MGMT alone. Neuro-oncology. 2014;16:1263–1273. doi:10.1093/neuonc/nou005.
- Bolotin DA, Mamedov IZ, Britanova OV, Zvyagin IV, Shagin D, Ustyugova SV, Turchaninova MA, Lukyanov S, Lebedev YB, Chudakov DM, et al. Next generation sequencing for TCR repertoire profiling: platform-specific features and correction algorithms. Eur J Immunol. 2012;42:3073–3083. doi:10.1002/eji.201242517.
- Danska JS, Livingstone AM, Paragas V, Ishihara T, Fathman CG. The presumptive CDR3 regions of both T cell receptor alpha and beta chains determine T cell specificity for myoglobin peptides. J Exp Med. 1990;172:27–33. doi:10.1084/jem.172.1.27.
- Nazarov VI, Pogorelyy MV, Komech EA, Zvyagin IV, Bolotin DA, Shugay M, Chudakov DM, Lebedev YB, Mamedov IZ. tcR: an R package for T cell receptor repertoire advanced data analysis. BMC Bioinformat. 2015;16:175. doi:10.1186/s12859-015-0613-1.
- Covacu R, Philip H, Jaronen M, Almeida J, Kenison J, Darko S, Chao -C-C, Yaari G, Louzoun Y, Carmel L, et al. System-wide analysis of the T cell response. Cell Rep. 2016;14:2733–2744. doi:10.1016/j.celrep.2016.02.056.
- Keane C, Gould C, Jones K, Hamm D, Talaulikar D, Ellis J, Vari F, Birch S, Han E, Wood P, et al. The T-cell receptor repertoire influences the tumor microenvironment and is associated with survival in aggressive B-cell lymphoma. Clin Cancer Res. 2017;23:1820–1828. doi:10.1158/1078-0432.CCR-16-1576.
- Pacholczyk R, Ignatowicz H, Kraj P, Ignatowicz L. Origin and T cell receptor diversity of Foxp3+CD4+CD25+ T cells. Immunity. 2006;25:249–259. doi:10.1016/j.immuni.2006.05.016.
- Ben-Aharon O, Magnezi R, Leshno M, Goldstein DA. Association of Immunotherapy with durable survival as defined by value frameworks for cancer care. JAMA Oncology. 2018;4:326–332. doi:10.1001/jamaoncol.2017.4445.
- Neelapu SS, Locke FL, Bartlett NL, Lekakis LJ, Miklos DB, Jacobson CA, Braunschweig I, Oluwole OO, Siddiqi T, Lin Y, et al. Axicabtagene ciloleucel CAR T-cell therapy in refractory large B-cell lymphoma. N Engl J Med. 2017;377:2531–2544. doi:10.1056/NEJMoa1707447.
- Topalian SL, Sznol M, McDermott DF, Kluger HM, Carvajal RD, Sharfman WH, Brahmer JR, Lawrence DP, Atkins MB, Powderly JD, et al. Survival, durable tumor remission, and long-term safety in patients with advanced melanoma receiving nivolumab. J Clin Oncol. 2014;32:1020–1030. doi:10.1200/JCO.2013.53.0105.
- Rizvi NA, Mazières J, Planchard D, Stinchcombe TE, Dy GK, Antonia SJ, Horn L, Lena H, Minenza E, Mennecier B, et al. Activity and safety of nivolumab, an anti-PD-1 immune checkpoint inhibitor, for patients with advanced, refractory squamous non-small-cell lung cancer (CheckMate 063): a phase 2, single-arm trial. Lancet Oncol. 2015;16:257–265. doi:10.1016/S1470-2045(15)70054-9.
- Keskin DB, Anandappa AJ, Sun J, Tirosh I, Mathewson ND, Li S, Oliveira G, Giobbie-Hurder A, Felt K, Gjini E, et al. Neoantigen vaccine generates intratumoral T cell responses in phase Ib glioblastoma trial. Nature. 2019;565:234–239. doi:10.1038/s41586-018-0792-9.
- Suto R, Srivastava PK. A mechanism for the specific immunogenicity of heat shock protein-chaperoned peptides. Science. 1995;269:1585–1588. doi:10.1126/science.7545313.
- Tamura Y, Peng P, Liu K, Daou M, Srivastava PK. Immunotherapy of tumors with autologous tumor-derived heat shock protein preparations. Science. 1997;278:117–120. doi:10.1126/science.278.5335.117.
- Zheng H, Dai J, Stoilova D, Li Z. Cell surface targeting of heat shock protein gp96 induces dendritic cell maturation and antitumor immunity. J Immunol. 2001;167:6731–6735. doi:10.4049/jimmunol.167.12.6731.
- Crane CA, Han SJ, Ahn B, Oehlke J, Kivett V, Fedoroff A, Butowski N, Chang SM, Clarke J, Berger MS, et al. Individual patient-specific immunity against high-grade glioma after vaccination with autologous tumor derived peptides bound to the 96 KD chaperone protein. Clin Cancer Res. 2013;19:205–214. doi:10.1158/1078-0432.CCR-11-3358.
- Bloch O, Crane CA, Fuks Y, Kaur R, Aghi MK, Berger MS, Butowski NA, Chang SM, Clarke JL, McDermott MW, et al. Heat-shock protein peptide complex–96 vaccination for recurrent glioblastoma: a phase II, single-arm trial. Neuro Oncol. 2014;16:274–279. doi:10.1093/neuonc/not203.
- Hsu M, Sedighim S, Wang T, Antonios JP, Everson RG, Tucker AM, Du L, Emerson R, Yusko E, Sanders C, et al. TCR sequencing can identify and track glioma-infiltrating T cells after DC vaccination. Cancer Immunol Res. 2016;4:412–418. doi:10.1158/2326-6066.CIR-15-0240.
- Choudhury NJ, Kiyotani K, Yap KL, Campanile A, Antic T, Yew PY, Steinberg G, Park JH, Nakamura Y, O’Donnell PH, et al. Low T-cell receptor diversity, high somatic mutation burden, and high neoantigen load as predictors of clinical outcome in muscle-invasive bladder cancer. Eur Urol Focus. 2016;2:445–452. doi:10.1016/j.euf.2015.09.007.
- Hopkins AC, Yarchoan M, Durham JN, Yusko EC, Rytlewski JA, Robins HS, Laheru DA, Le DT, Lutz ER, Jaffee EM, et al. T cell receptor repertoire features associated with survival in immunotherapy-treated pancreatic ductal adenocarcinoma. JCI Insight. 2018;3. doi:10.1172/jci.insight.122092.
- Park J-H, Jang M, Tarhan YE, Katagiri T, Sasa M, Miyoshi Y, Kalari KR, Suman VJ, Weinshilboum R, Wang L, et al. Clonal expansion of antitumor T cells in breast cancer correlates with response to neoadjuvant chemotherapy. Int J Oncol. 2016;49:471–478. doi:10.3892/ijo.2016.3540.
- Hosoi A, Takeda K, Nagaoka K, Iino T, Matsushita H, Ueha S, Aoki S, Matsushima K, Kubo M, Morikawa T, et al. Increased diversity with reduced “diversity evenness” of tumor infiltrating T-cells for the successful cancer immunotherapy. Sci Rep. 2018;8:1058. doi:10.1038/s41598-018-19548-y.
- Cui J-H, Lin K-R, Yuan S-H, Jin Y-B, Chen X-P, Su X-K, Jiang J, Pan Y-M, Mao S-L, Mao X-F, et al. TCR repertoire as a novel indicator for immune monitoring and prognosis assessment of patients with cervical cancer. Front Immunol. 2018;9:2729. doi:10.3389/fimmu.2018.02729.
- Jackson CM, Choi J, Lim M. Mechanisms of immunotherapy resistance: lessons from glioblastoma. Nat Immunol. 2019;20:1100–1109. doi:10.1038/s41590-019-0433-y.
- Bolotin DA, Shugay M, Mamedov IZ, Putintseva EV, Turchaninova MA, Zvyagin IV, Britanova OV, Chudakov DM. MiTCR: software for T-cell receptor sequencing data analysis. Nat Methods. 2013;10:813–814. doi:10.1038/nmeth.2555.