ABSTRACT
Graft-versus-host disease (GvHD) remains a significant impediment to allogeneic hematopoietic cell transplantation (HCT) success, necessitating studies focused on alleviating GvHD, while preserving the graft-versus-leukemia (GvL) effect. Based on our previous studies showing bendamustine with total body irradiation (BEN-TBI) conditioning reduces GvHD compared to the current clinical standard of care cyclophosphamide (CY)-TBI in a murine MHC-mismatched bone marrow transplantation (BMT) model, this study aimed to evaluate the role and fate of donor T-cells following BEN-TBI conditioning. We demonstrate that BEN-TBI reduces GvHD compared to CY-TBI independently of T regulatory cells (Tregs). BEN-TBI conditioned mice have a smaller proportion and less activated donor T-cells, with lower CD47 expression, early post-transplant, but no sustained phenotypic differences in T-cells. In BEN-TBI conditioned mice, donor T-cells gain tolerance specific to host MHC antigens. Though these T-cells are tolerant to host antigens, we demonstrate that BEN-TBI preserves a T-cell-dependent GvL effect. These findings indicate that BEN-TBI conditioning reduces GvHD without compromising GvL, warranting its further investigation as a potentially safer and more efficacious clinical alternative to CY-TBI.
KEYWORDS:
Introduction
The success of allogeneic hematopoietic cell transplantation (HCT) as a treatment for hematological disorders and malignancies is limited by prevalent and often lethal graft-versus-host disease (GvHD). While GvHD prophylaxis and treatment is necessary, it is associated with suppression of graft-versus-leukemia (GvL).Citation1 This necessitates investigating novel approaches that can optimally reduce GvHD while preserving GvL. We have previously shown in a murine model that administration of bendamustine (BEN) post-haploidentical bone marrow transplantation (BMT) can reduce GvHD and preserve GvL.Citation2 Furthermore, we have shown that post-transplant (PT)-BEN administration results in protection from GvHD comparable to and GvL effects superior to that of PT-cyclophosphamide (CY),Citation2 the current clinical standard of care following haploidentical BMT.Citation3-Citation5
Based on our data indicating BEN performs favorable immunomodulatory activities when given post-transplant to reduce GvHD, we explored its effects when employed as part of the conditioning regimen. BEN has previously been shown to be a safe and effective component of chemotherapy-based conditioning regimens for autologousCitation6-Citation8 and allogeneicCitation9,Citation10 transplants, but it has not been studied in combination with total body irradiation (TBI). CY with TBI is the most widely applied conditioning regimen for acute lymphoblastic leukemia (ALL)Citation11 and is also used to condition for unrelated HCT to treat severe aplastic anemia.Citation12-Citation16 However, it is associated with severe tissue damage and GvHD.Citation17,Citation18 We previously reported that substituting BEN for CY, followed by TBI, as pre-transplant conditioning in an MHC-mismatched murine model significantly reduces GvHD morbidity and mortality.Citation19 Our previous study showed that this effect was at least partially dependent on myeloid-derived suppressor cells (MDSCs),Citation19 leading us to, in this study, explore the impact of BEN conditioning on the function and phenotype of donor T-cells post-transplant. We examined changes in T-cell phenotype to explain the suppression of GvHD, as Th-1/Th-2 skewing,Citation20,Citation21 T regulatory cell (Treg) frequency,Citation22-Citation24 and co-stimulatory/co-inhibitory molecule expression,Citation25-Citation29 among other factors, have been shown to strongly impact GvHD. We found that BEN-TBI conditioning reduces GvHD compared to CY-TBI, independently of Tregs, and without appreciable differences in T-cell numbers, proportions, and phenotype in our murine model of MHC-mismatched BMT. We demonstrate further that donor-derived T-cells develop tolerance to host MHC antigens, which is observed both in vitro and in vivo. Finally, despite the induction of tolerance to host MHC antigens, we demonstrate that BEN-TBI conditioning preserves a T-cell-dependent GvL effect against A20 B-cell leukemia.
Materials and methods
Mice
Age-matched 6–10 week-old female C57BL/6, BALB/c, BoyJ, and FVB/N mice were purchased from The Jackson Laboratory. Mice were housed in specific pathogen-free conditions and cared for according to the guidelines of the University of Arizona Institutional Animal Care and Use Committee (IACUC). All murine experiments were conducted within IACUC approval and guidelines.
BMT model
Recipient BALB/c (H-2d) mice received 40 mg/kg of BEN (Selleckchem S1212) intravenously (iv) or 200 mg/kg of CY (Sigma-Aldrich C0768) intraperitoneally (ip) on day −2 and 400 cGy TBI on day −1 using a Cesium 137 irradiator.Citation19 CY and BEN were reconstituted and diluted as previously reported.Citation2 Studies indicate that the drugs are cleared by 24 hours post-administration,Citation30-Citation32 negating direct effects on the donor graft. On day 0, mice received 107 C57BL/6 (H-2b) bone marrow (BM) cells with 3 × 106 spleen cells (SC) or 107 T-cell depleted BM cells (TCD-BM) with 3 × 106 isolated CellTrace Violet-stained (Invitrogen; C34557) total T-cells (tT) iv. In some experiments, donor tT or SC were isolated from congenic CD45.1+ BoyJ mice. Moribund mice were euthanized according to IACUC-approved criteria and procedures and survival was monitored daily. Mice were weighed and scored clinically every three to four days on skin integrity, fur texture, posture, and activity and cumulative GvHD scores were calculated.Citation33 Mice given a cumulative score of 8 following day +8 were euthanized. We additionally evaluated graft-versus-host disease free, relapse free survival (GRFS). This is a composite end-point used frequently in clinical studiesCitation34-Citation36 where incidents are defined as acute grade III–IV GvHD, chronic GvHD, relapse, or death.Citation37 We adapted this for our mouse model, defining incidents as a total GvHD score of 6 or more, analogous to grade III–IV GvHD in humans, quantifiable tumor burden, both following day +8, or death.
Isolations and depletions
Total T-cells were isolated from naïve BoyJ spleens by negative selection (mouse Pan T-Cell Isolation Kit II, Miltenyi Biotec; 130-095-130) with a purity of >97%. T-cells were depleted from BM using Miltenyi Biotec’s CD3ε MicroBead Kit (130-094-973), with less than 0.3% CD3ε+ cells remaining. CD25+ cells were depleted from tT cells using CD25 Microbeads (Miltenyi Biotec; 130-091-072), resulting in less than 0.4% remaining CD25+ FoxP3+ cells (data not shown).
Treg generation, isolation, and suppression assays
Tregs were generated as previously reported.Citation38 Briefly, splenic CD4+ CD62L+ cells were isolated from BALB/c mice (Miltenyi Biotec; 130-106-643) and cultured with anti-CD3/CD28 beads (Gibco; 11453D) and TGF-β (Peprotech; 100–21) for 3 days. Viability was determined by Trypan Blue staining (Hyclone GE Healthcare Life Sciences; SV3008401). Tregs were isolated from conditioned spleens using Miltenyi Biotec’s Treg Isolation Kit (130-091-041). Suppression assays were conducted and analyzed as previously described.Citation2 T-cells were isolated from spleens of naïve C57BL/6 mice, CellTrace Violet stained, and stimulated with anti-CD3/CD28 beads. Tregs were co-incubated with stimulated T-cells at various ratios for 3 days. Flow cytometry was performed, followed by Modfit (Verity Software House) analysis to determine the proliferation index (PI) of the T-cells and calculate % proliferation.
Flow cytometry
Prior to analysis by flow cytometry, blood was collected by tail tipping or cardiac puncture and spleens were processed to single cell suspension. Red blood cells were lysed (BD Pharm Lyse, BD Biosciences; 555899) and flow cytometry was performed as previously reported.Citation39 Flow cytometric data were collected using an LSRFortessa cell analyzer (BD Biosciences, San Jose, CA, USA) and analyzed using FlowJo 2 (Tree Star). Antibodies used were anti-mouse H2kb PerCP-eFluor710 (clone AF6-88.5.5.3; 46–5958), CD8α PE-Cy7 (53–6.7; 25–0081), CD4 APC (RM4-5; 50-148-54), FoxP3 APC (FJK-16s; 17–5773), CD45.1 APC (A20; 17–0453), GATA3 PE-Cy7 (TWAJ; 25–9966), CD134 PE-Cy7 (OX-86; 25–1341), FoxP3 PE (150D/E4; 12–4774), CD69 PE-Cy5 (H1.2F3; 15–0691), RORγt APC (AFKJS-9; 17–6988), IFN gamma PE (XMG1.2, 12–7311) (Thermo Fisher Scientific), CD45.1 PE-CF594 (A20; 562452), CD3ε PE-CF594 (145–2 C11; 562286), CD44 BB515 (IM7; 565941), CD44 BV510 (IM7; 563114) (BD Biosciences), CD47 PE-Vio770 (REA170; 130-102-383), TIM-3 VioBright FITC (REA602; 130-109-449), TIM-3 PE (REA602; 130-118-563), CD278 VioGreen (REA192; 130-100-739), CD272 PE (REA224; 130-102-689), CD4 APC-Vio770 (GK1.5; 130-102-786), CD4 VioGreen (GK1.5; 130-102-444) (Miltenyi Biotec), Tbet PE-Dazzle594 (4B10; 644828), CTLA-4 PE-Dazzle594 (UC10-4B9; 106318), CD8α Brilliant Violet 421 (53–6.7; 100738), CCR7 PE-Cy5 (4B12; 120114), PD-1 APC (29F.1A12; 135210), CD25 AlexaFluor700 (PC61; 102024), and TNF-α Brilliant Violet 510 (MP6-XT22, 506339) (Biolegend). To determine absolute cell numbers in blood, white blood cell counts were determined using a HemaVet 950 (Drew Scientific, Miami Lakes, FL, USA).Citation40
Plasma and DC stimulation
BALB/c mice received BEN or CY on day −2, TBI on day −1, and were sacrificed on day 0. Blood was collected by cardiac puncture for plasma isolation and splenic dendritic cells (DCs) were isolated using a Pan DC Isolation Kit (Miltenyi Biotec; 130-100-875). T-cells were isolated from the spleens of naïve C57BL/6 mice and plated with 50% plasma for four hours or plated with pan-DCs (1 DC:10 T-cells) for 16 hours. Assays were incubated at 37°C and 5% CO2.
Mixed Leukocyte Reaction (MLR)
Recipient BALB/c (H-2d) mice received BEN iv on day −2, TBI on day −1, and 106 BM cells with 3 × 106 SC from naïve C57BL/6 (H-2b) mice on day 0. Survival was monitored and moribund mice were euthanized. Between day +100 and day +150, mice were sacrificed, splenic T-cells isolated, and their donor origin (H2kb+) was confirmed by flow cytometry. These T-cells were plated at 100,000 cells/well in a 96-well U-bottom plate. Splenocytes from naïve C57BL/6 (H-2b), BALB/c (H-2d), and FVB/N (H-2q) mice were used as stimulators. Spleens were processed to single cell suspensions and red blood cells were lysed. Splenocytes then received 1500 cGy irradiation using a Cesium 137 irradiator and were plated 800,000 cells/well with the T-cells. The cells were co-incubated in DMEM high glucose media (Corning; 10-013-CV) with 10% FBS (Hyclone; SH30071), MEM nonessential amino acids (Hyclone; SH30598), 2 mM L-glutamine (Gibco; 25030081), 55 μM β-mercaptoethanol (Gibco; 21985023), and penicillin-streptomycin (Gibco; 15140122) at 37°C and 7.5% CO2 for 3 days.Citation41 0.5 μCi of tritiated-thymidine (PerkinElmer; NET027001MC) was then added for an additional 18 hours of co-culture. Plates were harvested using a Brandel Wash Pump (CH-696; Gaithersburg, MD, USA), allowed to dry overnight, and counts per minute (CPM) were determined using a MicroBeta2 2450 Microplate Counter (PerkinElmer, Waltham, MA, USA).
Secondary transplant
Primary Transplant: Recipient BALB/c (H-2d) mice received 40 mg/kg of BEN iv on day −2, 400 cGy TBI on day −1, and 106 BM cells with 3 × 106 SC from naïve BoyJ (H-2b) mice on day 0. Survival was monitored and moribund mice were euthanized. Between day +100 and day +150, mice were sacrificed and splenic T-cells were isolated. Secondary transplant: Recipient BALB/c mice (H-2d) received 850 cGy TBI on day −1 and 106 TCD-BM from naïve C57BL/6 mice (H-2b) on day 0. These recipient mice additionally received 106 T-cells of confirmed donor BoyJ origin (H-2b; H2kb+CD45.1+) isolated from the surviving primary transplant recipients. Survival was monitored and moribund mice were euthanized. Engraftment of the T-cells (H2kb+CD45.1+) and BM (H2kb+CD45.2+) were monitored over time.
Tumor cells, imaging, and in vivo depletions
A20-luciferase (A20-luc), a BALB/c B-cell lymphoblastic leukemia cell line, was generously provided by Dr. Xue-Zhong Yu, MD (Medical University of South Carolina) and has been used previously in murine BMT studies.Citation2 A20-luc was cultured in RPMI 1640 (Hyclone SH30027) with 10% FBS, MEM, and sodium pyruvate (Hyclone SH30239) at 37°C and 5% CO2 and administered iv (0.1x106) on day 0, with the BMT. To image tumor burden, A20-luc bearing mice were given luciferin (GoldBio; LUCK) ip 0.15 mg/g, anesthetized with isoflurane (Piramal Critical Care; 440532079), and imaged using a LagoX (Spectral Instruments Imaging, Tucson, AZ, USA). Luminescence was quantified using AmiView software (Spectral Instruments Imaging) and presented as ln(photons/second). For depletion experiments, injections of depletion antibodies (NK1.1, BE0036; GK1.5, BE0003-1; 2.43, BE0061) were given ip 200 μg weekly beginning on day +3 (Bio X Cell).
Statistics
Kaplan–Meier survival curves were generated and the log-rank statistic was used to evaluate differences between conditions.Citation42,Citation43 Mann–Whitney tests were used to determine other differences between groups. P values less than 0.05 were considered statistically significant and are indicated in the graph or figure legend. If statistics are not indicated, the differences were nonsignificant.
Results
BEN-TBI conditioning improves survival and decreases morbidity from GvHD
Using a fully MHC-mismatched murine BMT model (C57BL/6 → BALB/c), comparing BEN-TBI to CY-TBI conditioning, we confirmed that BEN-TBI conditioning significantly protects recipients from GvHD lethality and morbidity, demonstrated by reduced GvHD score and weight loss (–)). We have previously reported that the BEN and CY doses used are comparable, comprising ~50% of the maximum tolerated dose in BALB/c mice, and that BEN-TBI and CY-TBI show comparable rates of complete engraftment.Citation19 Additionally, when applied in a syngeneic transplant setting, BEN-TBI and CY-TBI conditioning do not result in clinical toxicity or lethality, confirming that the difference in morbidity and mortality in this MHC-mismatched setting is due to GvHD.Citation19
Figure 1. BEN-TBI conditioning improves survival and decreases morbidity from GvHD. BALB/c recipient mice received 40 mg/kg BEN iv or 200 mg/kg CY ip on day −2, 400 cGy TBI on day −1, and 107 BM with 3 × 106 SC from naïve C57BL/6 mice on day 0. (a) Survival is shown. Pooled data from 3 experiments are shown, n = 15 mice/group, p < .0001. (b) Average weekly GvHD score with SEM is shown. Representative data from 3 experiments is shown, n = 5 mice/group. (c) Average % weight change from starting weight with SEM is shown. Representative data from 3 experiments is shown, n = 5 mice/group. * p < .05, ** p < .01, *** p < .001, **** p < .0001.
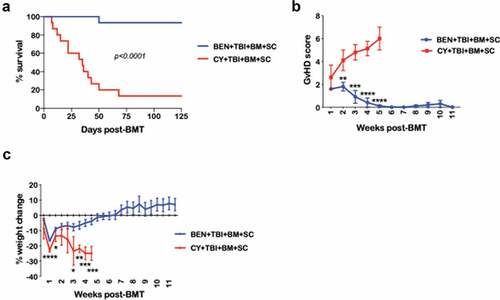
BEN-TBI conditioning improves GvHD independently of donor Tregs
Having previously correlated the reduction of GvHD with BEN-TBI to an increase in MDSCs,Citation19 we sought to evaluate the effect of BEN on Treg number and function, as well as the necessity of Tregs for the reduction of GvHD seen with BEN-TBI. We found no difference in number ()) or proportion (data not shown) of Tregs between BEN-TBI and CY-TBI conditioned mice. Representative flow from day +35 is shown. We further investigated the effect of BEN-TBI on this subset of cells by isolating splenic Tregs from BEN-TBI or CY-TBI conditioned mice just prior to transplant and on days +7 and +14 post-BMT. We plated isolated Tregs with CD3/CD28 stimulated, CellTrace Violet-stained T-cells from naïve C57BL/6 mice to evaluate the suppressive function of the conditioned Tregs. While we observed increased suppressive function post-conditioning compared to Tregs from untreated naïve mice, there was no difference in suppressive function between BEN-TBI and CY-TBI conditioning ()).
Figure 2. BEN-TBI conditioning improves GvHD independently of donor Tregs. (a) BALB/c recipient mice received 40 mg/kg BEN iv or 200 mg/kg CY ip on day −2, 400 cGy TBI on day −1, and 107 BM with 3 × 106 SC from naïve C57BL/6 mice on day 0. Peripheral blood was collected on days +7, +14, +21, +35, and +70 and stained for CD4, CD25, and FoxP3 for flow cytometric analysis. CD4+ CD25+ FoxP3+ cells were considered Tregs. Representative flow cytometry histograms from day +35 are shown. CBCs were determined and used to calculate absolute cell numbers. Average absolute numbers of cells per μL of blood are shown with SEM. Pooled data from 4 experiments are shown, n = 19 mice/group. (b) BALB/c recipient mice received 40 mg/kg BEN iv or 200 mg/kg CY ip on day −2, 400 cGy TBI on day −1, and 107 BM with 3 × 106 SC from naïve C57BL/6 mice on day 0. Splenic Tregs were isolated on day 0 (prior to transplant), +7, and +14. Tregs were plated with anti-CD3/CD28 bead activated, CellTrace Violet-stained T-cells from naïve C57BL/6 mice in a suppression assay. T-cells were plated at 100,000 cells per well in a 96-well U-bottom plate. Tregs were plated at a ratio of 1 Treg to 2 T-cells. Proliferation was assessed by flow after 3 days of co-culture. Average % proliferation with SEM is shown, compared to a control with no Tregs. Pooled data from 2 experiments are shown, n = 4–7 mice/group. (c) BALB/c recipient mice received 40 mg/kg BEN iv or 200 mg/kg CY ip on day −2, 400 cGy TBI on day −1, and 107 TCD-BM with 3 × 106 tT with or without CD25+ cell depletion from naïve C57BL/6 mice on day 0. Survival is shown. CY+TBI+TCD-BM+tT vs. CY+TBI+TCD-BM+CD25- tT p = .0857, BEN+TBI+TCD-BM+CD25- tT vs. CY+TBI+TCD-BM+CD25- tT p = .0005. (d) CD4+ CD62L+ T-cells were isolated from naïve BALB/c mouse spleens and cultured with 5 ng/mL TGF-β and a 1:1 ratio of anti-CD3/CD28 beads for 3 days in the presence of various concentrations of BEN. Cells were phenotyped and the % CD4+ CD25+ FoxP3+ of all cells remaining at the end of the culture was assessed, as well as viability. BEN was washed out and cells were plated in a suppression assay at a ratio of 1 Treg to 1 T-cell (CD3/CD28 activated, CellTrace Violet-stained, from naïve C57BL/6 mice). After 3 days of co-culture, proliferation was assessed by flow cytometry. Representative flow cytometry plots are shown, with proliferation index (PI) indicated, with higher proliferation indices representing greater proliferation. The T-cells + beads plot represents activated T-cells without Tregs (a positive control for proliferation). Averages are shown with SEM. Pooled data from 3 experiments is shown, n = 3/condition.
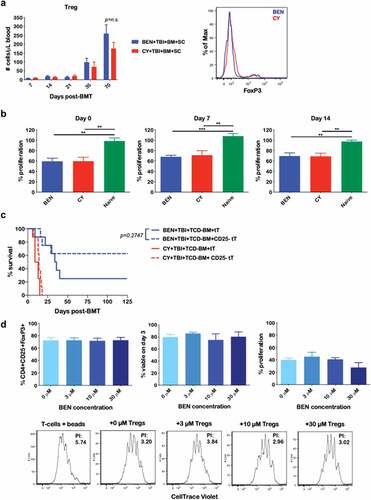
We additionally investigated whether Tregs are required for the reduction of GvHD observed with BEN-TBI. It is well-established that depletion of CD25+ cells, and thus, Tregs, from the donor graft exacerbates GvHD.Citation22,Citation44-Citation46 However, in our model, we demonstrate that depleting CD25+ cells from the donor T-cell graft does not significantly impact GvHD survival in BEN-TBI conditioned mice ()). This indicates that Tregs are not necessary for the reduction of GvHD observed with BEN-TBI conditioning. In fact, though not significant, with BEN-TBI conditioning, we see a trend towards an increase in survival following depletion of CD25+ cells from the graft. CD25+ depletion successfully eliminates FoxP3+ cells, as well as a portion of the FoxP3- conventional CD4+ T-cell population, resulting in an increase in the ratio of CD8 to CD4 T-cells infused (data not shown). We have found that, in this model, GvHD is less severe when isolated CD8+ T-cells are transplanted in the absence of CD4+ T-cells compared to an equal number of total T-cells (Supplemental Figure 1), potentially explaining the decreased GvHD lethality observed with CD25+ depletion.
Lastly, we investigated the effect of BEN on the in vitro generation of Tregs by generating Tregs in the presence of various BEN concentrations. Following a three-day culture, we observed no difference in percentage of CD4+ CD25+ FoxP3+ cells (); left) or cell viability (); middle) regardless of BEN concentration. To evaluate Treg function, we washed the BEN out and plated the Tregs with CD3/CD28 activated, CellTrace Violet-stained T-cells from naïve mice. The generated Tregs were, in fact, suppressive and we saw no difference in suppressive function with the addition of BEN (); right). Representative CellTrace Violet dilution by flow cytometry and PIs, indicating comparable suppression, are shown (); bottom). These data indicate that in vitro exposure to BEN does not affect Treg development or function.
BEN-TBI does not result in appreciable donor T-cell phenotypic differences post-transplant when compared to CY-TBI
Following the exclusion of Tregs as the mechanism by which BEN-TBI results in suppression of GvHD, we focused our studies on assessing differences in donor T-cell phenotype and effector function following transplant. We initially sought to investigate the fate of adoptively transferred donor T-cells in the early post-transplant period, as we hypothesized that the host environment of BEN-TBI conditioned mice might skew the donor T-cells toward phenotypes that minimize GvHD. Prior to infusion, we stained CD45.1+ donor T-cells with CellTrace Violet to monitor their proliferation in vivo, then collected spleen and blood on day +3. In both the blood and spleen, BEN-TBI conditioned mice had a lower proportion of donor T-cells, with no difference in absolute donor T-cell number compared to CY-TBI ()). We analyzed CellTrace Violet dilution to evaluate proliferation and noted no significant difference in the proliferation of donor T-cells ()). We further phenotyped the T-cells, grouping them into CellTracelow cells (those that had proliferated) and CellTracehigh cells (those that had not proliferated) and observed a lower percentage of CD25+ cells among the proliferating T-cells in BEN-TBI mice in the blood on day +3 ()). This difference persisted through day +5 (data not shown). Representative stratification of CellTrace Violet populations is shown in Supplemental Figure 2. This shows that although T-cells from BEN-TBI conditioned mice proliferate similarly to those from CY-TBI conditioned mice, they are less activated, shown by CD25 expression. We also evaluated intracellular TNFα and IFNγ, both pro-inflammatory cytokines, and saw reduced intracellular TNFα in the proliferated donor T-cells in the blood of BEN-TBI conditioned mice compared to CY-TBI (Supplemental Figure 3). Lastly, we evaluated CD47 expression, as it has been shown to exacerbate GvHD by preventing the clearance of alloreactive T-cells,Citation47 and observed a lower expression of CD47 on donor T-cells in the blood on day +3 in BEN-TBI conditioned mice ()). In summary, in the early post-transplant period, we observed a lower proportion and less activated donor T-cells in BEN-TBI mice, with decreased expression of CD47, compared to CY-TBI, all of which favors a reduction of GvHD.
Figure 3. BEN-TBI does not result in appreciable donor T-cell phenotypic differences post-transplant when compared to CY-TBI. (a–c) BALB/c recipient mice received 40 mg/kg BEN iv or 200 mg/kg CY ip on day −2, 400 cGy TBI on day −1, and 107 TCD-BM from naïve C57BL/6 mice with 3 × 106 CellTrace Violet-stained tT from naïve BoyJ mice on day 0. Blood and spleen were collected on day +3. (a) % donor T-cells (CD45.1+) was determined by flow cytometry. Using CBCs determined by HemaVet analysis, absolute number of donor T-cells was calculated. (b) After gating on CD45.1+ cells (representing donor T-cells), CellTrace Violet dilution was analyzed using ModFit software to determine proliferation index. Representative CellTrace Violet dilution is shown. (c) Within the CD45.1+ gate, cells were stratified by CellTracehigh (non- proliferative) and CellTracelow (proliferative) and CD25 and CD47 expression were analyzed by flow cytometry. Pooled data from 2 experiments with line at mean are shown, n = 6–7 mice/group. * p < .05, ** p < .01. (d-g) BALB/c recipient mice received 40 mg/kg BEN iv or 200 mg/kg CY ip on day −2, 400 cGy TBI on day −1, and 107 BM with 3 × 106 SC from naïve C57BL/6 mice on day 0. Peripheral blood was collected on days +7, +14, +21, +35, and +70 and stained for CD8, CD4, Tbet, GATA3, RORγt, CD134, CD278, PD-1, TIM3, CTLA-4, and CD272. CBCs were determined and used to calculate absolute cell numbers. Average absolute numbers of cells per μL of blood are shown with SEM. Representative flow plots from day +7 with fluorescence minus one (FMO) controls are shown (e). (d) Pooled data from 4 experiments are shown, n = 19 mice/group. (e-g) Pooled data from 2 experiments are shown, n = 10 mice/group. ** p < .01.
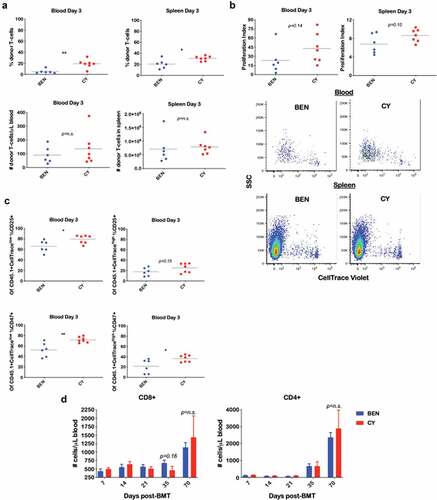
We then examined the peripheral blood T-cell phenotype through day +70. We observed no difference in absolute numbers of CD8+ and CD4+ T-cells in the blood ()). We evaluated the absolute numbers of Th-1, Th-2, and Th-17 cells, by Tbet, GATA3, and RORγt expression, respectively. We found more Th-2 cells, which have been associated with less GvHD,Citation20,Citation48 in BEN-TBI mice compared to CY-TBI mice on day +7 ()), a significant difference that was not sustained. We also found fewer Th-17 cells in BEN-TBI conditioned mice on day 14 ()), a modest but significant difference. Th-17 cells are generally thought to contribute to GvHD pathogenesis, though some groups have concluded they can ameliorate GvHD.Citation49 Representative histograms from day +7 are shown ()). We additionally evaluated co-stimulatory molecule expression, typically associated with increases in GvHD, including CD278 (ICOS)Citation29 and CD134 (OX40),Citation50 and co-inhibitory molecule expression, typically associated with decreases in GvHD, including CTLA-4,Citation26 PD-1,Citation27,Citation51 CD272Citation52 (BTLA), and TIM3.Citation25,Citation53 We saw no differences in absolute numbers of cells expressing these markers in peripheral blood (). Representative CD4+ flow plots from day +35 are shown in Supplemental Figure 4. We also saw no lasting differences in the percentage of T-cells expressing these markers (data not shown). We assessed effector memory, central memory, and naïve T-cells, as well as CD25 and CD47 expression, and again found no sustained differences in absolute cell numbers in peripheral blood (Supplemental Figure 5). These data fail to demonstrate any reliable trends in T-cell phenotypic differences between mice conditioned with BEN-TBI and those receiving CY-TBI.
Given the critical roles of cytokine milieuCitation54,Citation55 and host antigen presenting cellsCitation56-Citation58 in the pathophysiology of GvHD, we evaluated the effect of each on T-cell phenotype. On day 0, we collected plasma (Supplemental Figure 6a) and isolated splenic pan-DCs (Supplemental Figure 6b-D) from BEN-TBI and CY-TBI conditioned mice. We co-cultured each with T-cells from naïve C57BL/6 mice to examine potential effects on T-cell phenotype. We saw a modest increase in CD4 PD-1 expression, consistent with decreases in GvHD, as well as CD8 CD134 expression, when T-cells were plated with DCs from BEN-TBI conditioned mice, but no other differences.
BEN-TBI results in T-cell tolerance to host, but not third party, MHC antigens in vitro
Though we did not find clear phenotypic differences in donor T-cells post-transplant between the two conditioning regimens, we proceeded to evaluate their function. As shown in , the vast majority of BEN-TBI conditioned mice survive and have little to no remaining GvHD beyond five weeks post-BMT. Insufficient numbers of CY-TBI conditioned mice survive, precluding their use for comparison. We euthanized surviving BEN-TBI conditioned mice after day +100, isolated splenic total T-cells (H-2b, of C57BL/6 donor origin), and co-cultured them with C57BL/6 (syngeneic control), BALB/c (H-2d, representing MHC-mismatched host cells), and FVB/N (H-2q, third-party MHC-mismatch) irradiated splenocytes as stimulators. We used tritiated-thymidine to measure T-cell proliferation. As expected, reconstituted donor (C57BL/6, H-2b) T-cells from surviving BEN-TBI conditioned mice showed no proliferative response to syngeneic C57BL/6 irradiated spleen cells ()). Interestingly, reconstituted T-cells demonstrated significantly suppressed proliferation in response to splenocytes expressing host MHC (BALB/c, H-2d) when compared to the proliferation in response to third party splenocytes from FVB/N mice (H-2q) ()). This ~3-fold difference indicates that the T-cells retain the ability to respond to MHC-disparate antigens, but develop tolerance specifically to recipient host MHC antigens. We also compared these post-BEN-TBI conditioned BMT T-cells to T-cells taken from naïve, healthy age-matched C57BL/6 mice. When stimulated with FVB/N splenocytes, we saw comparable levels of proliferation between the BEN-TBI conditioned post-BMT T-cells and the T-cells from naïve mice. However, in contrast to the BEN-TBI T-cells, T-cells from naïve mice responded equally to stimulation by FVB/N and BALB/c splenocytes ()). To further confirm that the T-cells taken from BEN-TBI conditioned mice were not simply dysfunctional, we stimulated them with anti-CD3/CD28 beads and saw a significant proliferative response ()).
Figure 4. BEN-TBI results in T-cell tolerance to host, but not third party, MHC antigens in vitro. BALB/c recipient mice received 40 mg/kg BEN iv on day −2, 400 cGy TBI on day −1, and 107 BM with 3 × 106 SC from naïve C57BL/6 mice on day 0. ≥+100 days post-BMT, splenic T-cells of surviving mice were isolated and plated in an MLR with irradiated (1500 cGy) SC from naïve C57BL/6, BALB/c, and FVB/N mice. On day 3 of co-culture, 0.5 μCi tritiated-thymidine was added to each well and on day 4, cells were harvested and counts per minute (CPM), denoting proliferation, were measured using a gamma counter. T-cells from naïve C57BL/6 mice were also plated with C57BL/6, BALB/c, and FVB/N SC as a control (a). Additionally, BEN T-cells were stimulated with anti-CD3/CD28 beads and CPM were determined (b). Average CPM with SEM is shown. Pooled data from 3 experiments are shown, n = 6–12 mice/condition. **** p < .0001.
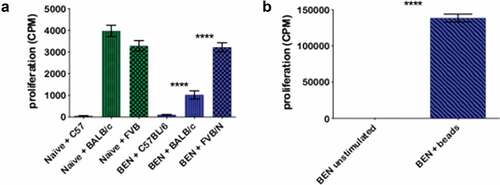
BEN-TBI results in T-cell tolerance to host MHC antigens in vivo
We next evaluated whether donor-derived T-cells following BEN-TBI conditioning remained tolerant to BALB/c MHC antigens in vivo when infused in a secondary BMT. We isolated splenic T-cells from surviving BEN-TBI conditioned mice and injected them into naïve BALB/c mice, conditioned with myeloablative TBI, along with TCD-BM from naïve C57BL/6 mice. These T-cells did not induce GvHD and resulted in complete survival (106 tT MHC-mismatched; )), as did the TCD-BM only control. For comparison, splenic T-cells from age-matched C57BL/6 mice that received BEN-TBI conditioning and a syngeneic primary transplant were used as controls. Syngeneic T-cells were in their primary recipient for the same amount of time as the MHC-mismatched T-cells, but without exposure to BALB/c MHC antigens. When transplanted into secondary BALB/c recipients, T-cells from syngeneic primary transplant recipients resulted in severe GvHD and complete lethality (106 tT syngeneic; )). This indicates that when mice are conditioned with BEN-TBI and receive an MHC-mismatched BMT, the engrafted T-cells become tolerant to host MHC, resulting in suppression of GvHD. We phenotyped the tolerant T-cells from mice receiving an MHC-mismatched BMT, as well as those from syngeneic primary BMT recipients. We saw no difference in Tbet, GATA3, and FoxP3 expression ()) or proportion of CD4 versus CD8 ()). We saw increased expression of the co-stimulatory molecule CD278 on the CD8+ T-cells from the syngeneic primary transplant, which is consistent with increased GvHD ()). However, though there are trends, we did not see any other significant or consistent differences in co-stimulatory or co-inhibitory molecules (). We saw no differences in CD47 or CD25 expression or effector memory, central memory, and naïve T-cells (Supplemental Figure 7). We confirmed the persistence of these tolerant T-cells in the blood following the secondary transplant. Our data indicate that the cells not only persist, but increase in number through day +70 following the secondary transplant ()), while causing no discernible GvHD.
Figure 5. BEN-TBI results in T-cell tolerance to host MHC antigens in vivo. BALB/c recipient mice received 40 mg/kg BEN iv on day −2, 400 cGy TBI on day −1, and 107 BM with 3 × 106 SC from naïve BoyJ mice on day 0. ≥+100 days post-BMT, splenic T-cells of surviving mice were isolated and given as donor T-cells in a secondary transplant. Secondary recipients received 850 cGy TBI on day −1 and 107 TCD-BM from naïve C57BL/6 mice on day 0 with or without T-cells from surviving BEN-TBI conditioned mice (called MHC-mismatched). As a control, secondary recipients received T-cells from C57BL/6 mice that had previously received BEN-TBI conditioning and a syngeneic BMT (called syngeneic). (a) Survival is shown. MHC-mismatched vs. syngeneic p < .0001. Pooled data from 3 experiments are shown, n = 12 mice/group. Average GvHD score and % weight change from starting weight are shown with SEM. Representative data from 3 experiments are shown, n = 5 mice/group. * p < .05, ** p < .01, *** p < .001. (b–e) On day 0, prior to infusion in the secondary transplant, isolated T-cells from MHC-mismatched and syngeneic primary transplants were phenotyped. Average % expression with SEM is shown. Pooled data from 3 experiments is shown, n = 3/condition. * p < .05. (f) Following the secondary transplant, peripheral blood was collected on days +7, +14, +21, +35, and +70 and stained for CD45.1, denoting T-cells from the primary transplant. CBCs were determined and used to calculate absolute number of donor T-cells per μL of blood. Average number of donor T-cells with SEM is shown. Pooled data from 2 experiments are shown, n = 8 mice/group.
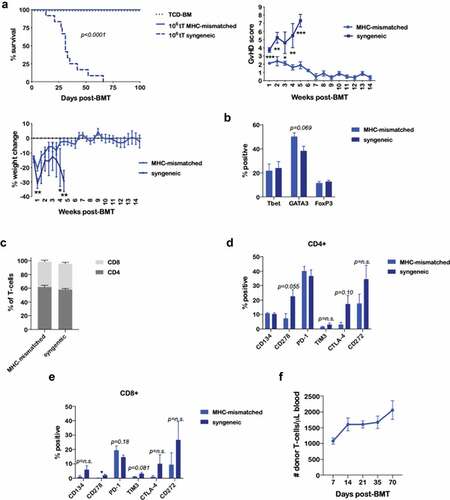
BEN-TBI preserves GvL
Having established that BEN-TBI conditioning reduces GvHD significantly and yields T-cells that are tolerant to host MHC (H-2d) antigens, we investigated the GvL associated with this conditioning regimen. We showed that, when luciferase-expressing BALB/c (H-2d) derived A20 leukemia cells were injected on the day of transplant, BEN-TBI conditioning followed by an MHC-mismatched BMT significantly improved survival over BEN-TBI conditioning followed by a syngeneic transplant ()). This indicates that GvL effects are preserved following BEN-TBI conditioning, despite suppression of GvHD. We also showed that in an allogeneic transplant setting with leukemia, BEN-TBI conditioning significantly improves survival over CY-TBI conditioning ()). includes deaths from tumor, GvHD, and combinations of the two. When we censor deaths that occurred with no visible tumor (i.e. deaths due solely to GvHD), BEN-TBI still had significantly improved survival over CY-TBI, indicating that the difference in survival was not solely attributable to a difference in GvHD lethality ()). We additionally evaluated graft-versus-host disease free, relapse-free survival (GRFS). BEN-TBI conditioning had a significantly higher percentage of mice without incidence ()), indicating a superior BMT outcome with BEN-TBI conditioning, when accounting for both tumor and GvHD. Of note, in a syngeneic transplant setting, there was no significant difference between BEN-TBI and CY-TBI survival (Supplemental Figure 8), indicating that the difference in GvL observed in our MHC-mismatched BMT setting is likely due to favorable immune modulatory effects of BEN-TBI. We further confirmed superior GvL with BEN-TBI conditioning by imaging mice serially and quantifying luminescence as photons/second, indicative of their A20-luc burden. BEN-TBI conditioned mice that received a syngeneic transplant consistently demonstrated significantly more photons/second than BEN-TBI or CY-TBI conditioned mice receiving an MHC-mismatched transplant. Following day +14, CY-TBI mice showed on average significantly more luminescence than BEN-TBI, indicating a greater tumor burden ()). This is also evident visually through bioluminescent imaging ()). These data indicate that BEN-TBI conditioning preserves a GvL effect superior to that seen with a syngeneic transplant or with CY-TBI conditioning.
Figure 6. BEN-TBI preserves GvL. BALB/c recipient mice received 40 mg/kg BEN iv or 200 mg/kg CY ip on day −2, 400 cGy TBI on day −1, and 107 BM with 3 × 106 SC from naïve C57BL/6 mice on day 0 with 0.1 × 106 A20-luc tumor cells. (a) As a control, BEN-TBI conditioned mice received syngeneic BM and SC with A20-luc. Survival of BEN-TBI conditioned mice receiving an MHC-mismatched BMT and syngeneic BMT are shown, p < .0001. (b) Survival of BEN-TBI and CY-TBI conditioned mice receiving an MHC-mismatched BMT with A20-luc cells are shown, p < .0001. (c) Survival of BEN-TBI and CY-TBI conditioned mice receiving MHC-mismatched BMT with A20-luc are shown with deaths occurring without visible tumor censored. Only deaths with measurable tumor are included. BEN vs. CY p < .01. (d) Graft-versus-host disease free, relapse free survival (GRFS) is shown. A GvHD score ≥6 or visible tumor following day +8, as well as death, were considered incidents. % without incidence is shown. Pooled data from 4 experiments are shown, n = 10–20 mice/group. (e) Following BMT and A20-luc infusion, mice were imaged and luminescence was measured twice a week. Average ln(photons/sec/mouse) with SEM is shown. Representative data from 4 experiments is shown, n = 5 mice/group. * p < .05, ** p < .01. (f) Luminescent imaging representative of 4 experiments is shown, n = 5 mice/group.
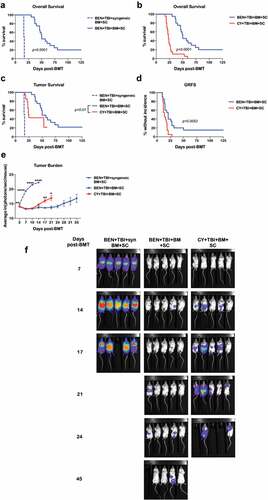
GvL with BEN-TBI is T-cell dependent
We lastly sought to further characterize the effector cells responsible for the GvL effect observed with BEN-TBI conditioning by depleting natural killer (NK), CD4+, or CD8+ cells. Control mice received an isotype antibody injection. Depleting antibodies were injected ip weekly beginning on day +3 following transplant. We confirmed that depletion of target lymphocyte subsets in the blood endured the entire interval between injections (data not shown). There was no difference in survival between the isotype control group and the NK cell depleted group. When CD4+ cells were depleted, survival was significantly decreased. Depletion of CD8+ cells resulted in the greatest loss of GvL with significantly decreased survival even in comparison to the CD4+ depleted mice ()). These data indicate NK cells do not play a significant role in the GvL effect seen following BEN-TBI conditioning, while CD4+ cells, and even more so, CD8+ cells do play a significant role. When tumor-free deaths (deaths due solely to GvHD) were censored, the same differences were observed ()). Bioluminescent imaging confirmed these results, with no differences between isotype control and NK cell depletion, and significantly increased photons/second in the CD4+ and CD8+ depletion groups (–)).
Figure 7. GvL with BEN-TBI is T-cell dependent. BALB/c recipient mice received 40 mg/kg BEN iv on day −2, 400 cGy TBI on day −1, and 107 BM with 3 × 106 SC from naïve C57BL/6 mice on day 0 with 0.1 × 106 A20-luc tumor cells. Mice received syngeneic BM and SC as a control. All mice received weekly ip antibody injections of 200 μg beginning on day +3 (isotype, NK1.1, CD4, or CD8). (a) Survival is shown. Pooled data from 2 experiments is shown, n = 10 mice/group. Syngeneic vs. all other conditions p < .0001; isotype vs. NK1.1 p = n.s., vs. CD4 p = .0068, vs. CD8 p < .0001. (b) Deaths without measurable tumor are censored and survival is shown. Syngeneic vs. all other conditions p < .0001; isotype vs. NK1.1 p = n.s., vs. CD4 p = .0011, vs. CD8 p < .0001. (c) Following BMT and A20-luc infusion, mice were imaged and luminescence was measured twice weekly. Average ln(photons/sec/mouse) with SEM is shown. Syngeneic vs. all other conditions p < .05 at all time points; isotype vs. NK1.1 p = n.s. at all time points, vs. CD4 p < .05 day +12, +21, +26, +29, vs. CD8 p < .05 at all time points. (d) Representative luminescent imaging of 2 experiments is shown, n = 5 mice/group.
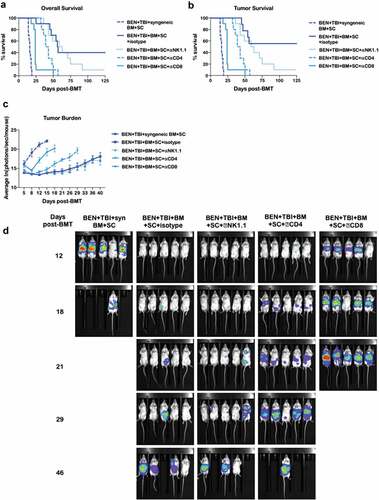
Discussion
GvHD remains a serious, often fatal impediment to the success of HCT. There are many strategies used to prevent GvHD, such as graft modification and post-transplant immunosuppression. Another, less-studied strategy to prevent GvHD is modification of the conditioning regimen. Pre-transplant conditioning regimens have been shown to damage host tissues, creating an inflammatory environment, leading to activation of antigen presenting cells and induction of GvHD. CY-TBI is a myeloablative conditioning regimen most commonly used clinically for ALL, though it is associated with severe tissue damage and GvHD.Citation11,Citation17,Citation18 We have previously published that substituting BEN for CY, combined with TBI, leads to reduced mortality and morbidity from GvHD in a murine MHC-mismatched BMT model.Citation19 Here, we confirm our previous findings that BEN-TBI reduces GvHD compared to CY-TBI and further show that although we could not ascertain a specific tolerant phenotype, the engrafted donor T-cells following BEN-TBI conditioning are indeed tolerant to host MHC without compromising GvL.
We show that the donor-derived T-cells taken from BEN-TBI conditioned mice are tolerant, specifically to BALB/c antigens, but remain functional otherwise, capable of responding to other foreign antigens and retaining the capacity to be activated by anti-CD3/CD28 beads (). Antigen-specific T-cell anergic states have been shown to be a mechanism of peripheral tolerance in transplant.Citation28,Citation59,Citation60 There are many ways in which tolerance can be achieved following HCT, including through expression of co-inhibitory molecules.Citation28,Citation60,Citation61 For this reason, we evaluated the expression of various co-inhibitory and, conversely, co-stimulatory molecules on T-cells in the peripheral blood. We saw that when BEN-TBI DCs were co-cultured with T-cells overnight, the T-cells expressed more PD-1 (Supplemental Figure 6c), a co-inhibitory molecule that has been shown to suppress GvHD.Citation25,Citation27,Citation28,Citation51,Citation62 Though this could be impacting GvHD, the difference between the groups is modest (p = .047). Additionally, when evaluated prior to infusion in the secondary transplant, BEN-TBI T-cells expressed lower levels of the co-stimulatory molecule CD278 ()). This also may have contributed to these cells causing less GvHD.Citation29,Citation63 We also demonstrated that BEN-TBI increased GATA3 expression, a marker of Th2 cells, in the first week following transplant ()). Though these cells have typically been considered innocuous in terms of GvHD,Citation20,Citation48 other studies have found the role of Th2 cells in GvHD to be more pleiotropic, with these cells sometimes causing GvHD.Citation21,Citation64,Citation65 Of note, we saw decreased CD47 expression early post-transplant ()), which could indicate that alloreactive T-cells were more effectively eliminated following BEN-TBI than CY-TBI.Citation47 Despite these observed differences, we did not find a clear, durable phenotypic difference that fully explained the significant tolerance achieved. One possible explanation for this is that the differences lie in markers we did not evaluate. Though we attempted to evaluate a variety of markers associated with increases and decreases in GvHD, it was by no means an exhaustive list. Another limitation of this methodology is that while the majority of BEN-TBI conditioned mice survive past day +70, the majority of CY-TBI conditioned mice die prior to day +35, meaning later time points are skewed toward CY-TBI mice with increased survival.
Additionally, the reduction of GvHD may simply be explained by the effect of BEN-TBI on other immune cell subsets. Though we do not have a well-defined picture of the mechanism by which BEN is reducing GvHD, we have clearly shown in previous publications that BEN leads to an increase in the number of MDSCs.Citation2,Citation19 MDSCs have been shown to induce T-cell tolerance, potentially explaining the tolerance to host antigens observed in our study.Citation66,Citation67 As the ratio of MDSC to T-cell is higher in BEN-TBI mice than CY-TBI mice,Citation19 the large number of MDSCs may allow BEN-TBI conditioned mice to achieve tolerance, while CY-TBI mice do not have enough MDSCs to overcome the alloreactive T-cell response. The higher numbers of MDSCs in BEN-TBI mice shown previously is also congruent with the data in this manuscript demonstrating a lower percentage of donor T-cells in the spleens and blood of BEN-TBI conditioned mice on day 3 ()). Though Tregs have been shown to be essential for GvHD control in other settings,Citation22,Citation44-Citation46 our data do not support a vital role of Tregs in our BEN-TBI model ()). Our data also indicate that though we have shown that BEN can effectively increase the suppressive function of MDSCs through in vitro exposure,Citation2 the same is not true for Tregs ()).
Importantly, there are many other factors that can impact GvHD and peripheral tolerance that have not been addressed in this study. For example, T-cells in target tissues may express differing levels of co-inhibitory and co-stimulatory molecules that were not apparent in the blood.Citation68 Cytokine levels can also both promote and prevent GvHD. We observed a transient decrease in TNFα in BEN-TBI circulating donor T-cells, which is consistent with decreased GvHD,Citation69 but many cytokines remain unexplored in this model. IL-10, for example, has been shown to mitigate GvHD,Citation54,Citation70,Citation71 while IL1-β and IL-6 have been shown to enhance GvHD.Citation56,Citation72 Interestingly, in a purely in vitro study, BEN has been shown to increase IL-10 production by a B-cell cancer line.Citation73 This makes the cytokine profile following BEN administration an interesting candidate for further investigation of the mechanism by which BEN is reducing GvHD. Other possible factors include BEN resulting in differing chemokine expression, as chemokine inhibition can reduce GvHD by preventing homing of alloreactive T-cells to target tissues.Citation74,Citation75 This is a particularly interesting potential explanation as we have previously shown fewer T-cells in the intestines of BEN-TBI conditioned mice than CY-TBI conditioned mice.Citation19
Though blood T-cell phenotype does not appear to differ between BEN-TBI and CY-TBI conditioning, we do see significant differences in tumor control. Disease recurrence remains a major cause of mortality following HCT and efforts to reduce GvHD frequently lead to increased relapse rates.Citation1,Citation76 Having confirmed that BEN-TBI conditioning significantly reduces GvHD, it was vital that we determine the impact of BEN-TBI on GvL effects. We have previously shown that administration of BEN post-haploidentical BMT yields superior GvL effects over administration of CY.Citation2 Herein, we provide the first evidence that BEN-TBI conditioning, while reducing GvHD (), also preserves GvL, yielding superior survival over CY-TBI (). Additionally, this GvL effect is almost entirely dependent on T-cells (), confirming that their tolerance to host antigens does not negatively impact their anti-tumor effect.
In summary, we have built upon our previous studies by confirming the reduction of GvHD following substitution of BEN for CY in the traditionally applied CY-TBI conditioning regimen. We demonstrate that BEN-TBI conditioning generates T-cells that are tolerant to host antigens, but that retain their anti-leukemia activity. BEN-TBI warrants further exploration both mechanistically and clinically, as it may provide a safer option than CY-TBI, in terms of both GvHD and relapse.
Author contributions
J.S. designed and performed experiments, analyzed and reviewed data, and wrote the manuscript. E.H. designed and performed experiments, analyzed and reviewed data, and edited the manuscript. M.M. designed and performed experiments and edited the manuscript. N.K. performed experiments and edited the manuscript. R.S. contributed to data discussion and edited the manuscript. Y.Z. contributed to the experimental design, data interpretation and discussion, and edited the manuscript. E.K. designed the project, supervised and advised on the implementation and conduction of experiments, reviewed and interpreted data, and co-wrote the manuscript.
Disclosure of Potential Conflicts of Interest
The authors report no conflict of interest.
Supplemental Material
Download ()Acknowledgments
The authors wish to thank Min Hahn, Jelena Eremija, and Cecilia Machado for technical assistance and Vanessa Frisinger for administrative assistance. We would like to thank Dr. John Streicher’s lab for technical assistance and for generously sharing their plate harvester and gamma counter and the University of Arizona’s Flow Cytometry Core facility for the use of their analysis software. Lastly, we would like to thank the University of Arizona’s University Animal Care staff for taking excellent care of our mice.
Supplementary material
Supplemental data for this article can be accessed on the publisher’s website.
Additional information
Funding
References
- van den Brink MRM, Porter DL, Giralt S, Lu SX, Jenq RR, Hanash A, Bishop MR. Relapse after allogeneic hematopoietic cell therapy. Biol Blood Marrow Trans. 2010;16(1):S138–S145. doi:10.1016/j.bbmt.2009.10.023.
- Stokes J, Hoffman EA, Zeng Y, Larmonier N, Katsanis E. Post-transplant bendamustine reduces GvHD while preserving GvL in experimental haploidentical bone marrow transplantation. Br J Haematol. 2016;174:102–17. doi:10.1111/bjh.14034.
- Luznik L, Fuchs EJ. High-dose, post-transplantation cyclophosphamide to promote graft-host tolerance after allogeneic hematopoietic stem cell transplantation. Immunol Res. 2010;47:65–77. doi:10.1007/s12026-009-8139-0.
- Luznik L, O’Donnell PV, Fuchs EJ. Post-transplantation cyclophosphamide for tolerance induction in HLA-haploidentical bone marrow transplantation. Semin Oncol. 2012;39:683–693. doi:10.1053/j.seminoncol.2012.09.005.
- Katsanis E, Sapp LN, Varner N, Koza S, Stea B, Zeng Y. Haploidentical bone marrow transplantation with post-transplant cyclophosphamide/bendamustine in pediatric and young adult patients with hematologic malignancies. Biol Blood Marrow Transplant. 2018;24:2034–2039. doi:10.1016/j.bbmt.2018.06.007.
- Gilli S, Novak U, Taleghani BM, Baerlocher GM, Leibundgut K, Banz Y, Zander T, Betticher D, Egger T, Rauch D, et al. BeEAM conditioning with bendamustine-replacing BCNU before autologous transplantation is safe and effective in lymphoma patients. Ann Hematol. 2017;96(3):421–429. doi:10.1007/s00277-016-2900-y.
- Martino M, Tripepi G, Messina G, Vincelli ID, Console G, Recchia AG, Gentile M, Molica S, Morabito F. A phase II, single-arm, prospective study of bendamustine plus melphalan conditioning for second autologous stem cell transplantation in de novo multiple myeloma patients through a tandem transplant strategy. Bone Marrow Transplant. 2016;51:1197–1203. doi:10.1038/bmt.2016.94.
- Hueso T, Gastinne T, Garciaz S, Tchernonog E, Delette C, Casasnovas R-O, Durot E, Houot R, Tessoulin B, Tournilhac O, et al. Bendamustine-EAM versus BEAM regimen in patients with mantle cell lymphoma undergoing autologous stem cell transplantation in the frontline setting: a multicenter retrospective study from Lymphoma Study Association (LYSA) centers. Bone Marrow Transplant. 2020. doi:10.1038/s41409-020-0783-y.
- Khouri IF, Wei W, Korbling M, Turturro F, Ahmed S, Alousi A, Anderlini P, Ciurea S, Jabbour E, Oran B, et al. BFR (bendamustine, fludarabine, and rituximab) allogeneic conditioning for chronic lymphocytic leukemia/lymphoma: reduced myelosuppression and GVHD. Blood. 2014;124(14):2306–2312. doi:10.1182/blood-2014-07-587519.
- Khouri IF, Sui D, Jabbour EJ, Samuels BI, Turturro F, Alatrash G, Anderlini P, Ahmed S, Oran B, Ciurea SO, et al. Bendamustine added to allogeneic conditioning improves long-term outcomes in patients with CLL. Bone Marrow Transplant. 2017;52:28–33. doi:10.1038/bmt.2016.204.
- Holter-Chakrabarty JL, Pierson N, Zhang M-J, Zhu X, Akpek G, Aljurf MD, Artz AS, Baron F, Bredeson CN, Dvorak CC, et al. The sequence of cyclophosphamide and myeloablative total body irradiation in hematopoietic cell transplant for patients with acute leukemia. Biol Blood Marrow Transplant. 2015;21:1251–1257. doi:10.1016/j.bbmt.2015.03.017.
- Deeg HJ, O’Donnell M, Tolar J, Agarwal R, Harris RE, Feig SA, Territo MC, Collins RH, McSweeney PA, Copelan EA, et al. Optimization of conditioning for marrow transplantation from unrelated donors for patients with aplastic anemia after failure of immunosuppressive therapy. Blood. 2006;108:1485–1491. doi:10.1182/blood-2006-03-005041.
- Bacigalupo A, Socie’ G, Lanino E, Prete A, Locatelli F, Locasciulli A, Cesaro S, Shimoni A, Marsh J, Brune M, et al. Fludarabine, cyclophosphamide, antithymocyte globulin, with or without low dose total body irradiation, for alternative donor transplants, in acquired severe aplastic anemia: a retrospective study from the EBMT-SAA Working Party. Haematologica. 2010;95(6):976–982. doi:10.3324/haematol.2009.018267.
- Svenberg P, Remberger M, Svennilson J, Mattsson J, Leblanc K, Gustafsson B, Aschan J, Barkholt L, Winiarski J, Ljungman P, et al. Allogenic stem cell transplantation for nonmalignant disorders using matched unrelated donors. Biol Blood Marrow Transplant. 2004;10(12):877–882. doi:10.1016/j.bbmt.2004.08.002.
- Deeg HJ, Amylon MD, Harris RE, Collins R, Beatty PG, Feig S, Ramsay N, Territo M, Khan SP, Pamphilon D, et al. Marrow transplants from unrelated donors for patients with aplastic anemia: minimum effective dose of total body irradiation. Biol Blood Marrow Transplant. 2001;7(4):208–215. doi:10.1053/bbmt.2001.v7.pm11349807.
- Brodsky RA, Luznik L, Bolaños-Meade J, Leffell MS, Jones RJ, Fuchs EJ. Reduced intensity HLA-haploidentical BMT with post transplantation cyclophosphamide in nonmalignant hematologic diseases. Bone Marrow Transplantation. 2008;42(8):523–527. doi:10.1038/bmt.2008.203.
- Nagler A, Rocha V, Labopin M, Unal A, Ben Othman T, Campos A, Volin L, Poire X, Aljurf M, Masszi T, et al. Allogeneic hematopoietic stem-cell transplantation for acute myeloid leukemia in remission: comparison of intravenous busulfan plus cyclophosphamide (Cy) versus total-body irradiation plus Cy as conditioning regimen–a report from the acute leukemia working party of the European group for blood and marrow transplantation. J Clin Oncol. 2013;31:3549–3556. doi:10.1200/JCO.2013.48.8114.
- Gyurkocza B, Sandmaier BM. Conditioning regimens for hematopoietic cell transplantation: one size does not fit all. Blood. 2014;124:344–353. doi:10.1182/blood-2014-02-514778.
- Stokes J, Hoffman EA, Molina MS, Eremija J, Larmonier N, Zeng Y, Katsanis E. Bendamustine with total body irradiation limits murine graft-versus-host disease in part through effects on myeloid-derived suppressor cells. Biol Blood Marrow Transplant. 2019;25:405–416. doi:10.1016/j.bbmt.2018.10.009.
- Betts BC, Bastian D, Iamsawat S, Nguyen H, Heinrichs JL, Wu Y, Daenthanasanmak A, Veerapathran A, O’Mahony A, Walton K, et al. Targeting JAK2 reduces GVHD and xenograft rejection through regulation of T cell differentiation. Proc Natl Acad Sci U S A. 2018;115(7):1582–1587. doi:10.1073/pnas.1712452115.
- Nikolic B, Lee S, Bronson RT, Grusby MJ, Sykes M. Th1 and Th2 mediate acute graft-versus-host disease, each with distinct end-organ targets. J Clin Invest. 2000;105:1289–1298. doi:10.1172/JCI7894.
- Ganguly S, Ross DB, Panoskaltsis-Mortari A, Kanakry CG, Blazar BR, Levy RB, Luznik L. Donor CD4(+) Foxp3(+) regulatory T cells are necessary for posttransplantation cyclophosphamide-mediated protection against GVHD in mice. Blood. 2014;124:2131–2141. doi:10.1182/blood-2013-10-525873.
- Hoffmann P, Ermann J, Edinger M, Fathman CG, Strober S. Donor-type CD4+CD25+ regulatory T Cells suppress lethal acute graft-versus-host disease after allogeneic bone marrow transplantation. J Exp Med. 2002;196:389–399. doi:10.1084/jem.20020399.
- Hotta M, Yoshimura H, Satake A, Tsubokura Y, Ito T, Nomura S. GM-CSF therapy inhibits chronic graft-versus-host disease via expansion of regulatory T cells. Eur J Immunol. 2019;49(1):179–191. doi:10.1002/eji.201847684.
- Anderson AC, Joller N, Kuchroo VK. Lag-3, Tim-3, and TIGIT: co-inhibitory receptors with specialized functions in immune regulation. Immunity. 2016;44:989–1004. doi:10.1016/j.immuni.2016.05.001.
- Blazar BR, Taylor PA, Panoskaltsis-Mortari A, Sharpe AH, Vallera DA. Opposing roles of CD28: b7and CTLA-4: b7Pathways in Regulating In Vivo alloresponses in murine recipients of MHC disparate T cells. J Immunol. 1999;162:6368.
- Blazar BR, Carreno BM, Panoskaltsis-Mortari A, Carter L, Iwai Y, Yagita H, Nishimura H, Taylor PA. Blockade of programmed Death-1 engagement accelerates graft-versus-host disease lethality by an IFN-γ-dependent mechanism. J Immunol. 2003;171:1272. doi:10.4049/jimmunol.171.3.1272.
- Bour-Jordan H, Esensten JH, Martinez-Llordella M, Penaranda C, Stumpf M, Bluestone JA. Intrinsic and extrinsic control of peripheral T-cell tolerance by costimulatory molecules of the CD28/ B7 family. Immunol Rev. 2011;241(1):180–205. doi:10.1111/j.1600-065X.2011.01011.x.
- Taylor PA, Panoskaltsis-Mortari A, Freeman GJ, Sharpe AH, Noelle RJ, Rudensky AY, Mak TW, Serody JS, Blazar BR. Targeting of inducible costimulator (ICOS) expressed on alloreactive T cells down-regulates graft-versus-host disease (GVHD) and facilitates engraftment of allogeneic bone marrow (BM). Blood. 2005;105:3372–3380. doi:10.1182/blood-2004-10-3869.
- Chandrashekar DV, Suresh P, Kumar R, Bhamidipati R, Mullangi R, Richter W, Srinivas N. Sensitive LC-MS/MS method for the simultaneous determination of bendamustine and its active metabolite, gamma-hydroxybendamustine in small volume mice and dog plasma and its application to a pharmacokinetic study in mice and dogs. Drug Res. 2017;67:497–508. doi:10.1055/s-0043-108124.
- Sadagopan N, Cohen L, Roberts B, Collard W, Omer C. Liquid chromatography-tandem mass spectrometric quantitation of cyclophosphamide and its hydroxy metabolite in plasma and tissue for determination of tissue distribution. J Chromatogr B Biomed Sci Appl. 2001;759:277–284. doi:10.1016/S0378-4347(01)00243-2.
- Srinivas NR, Richter W, Devaraj V, Suresh P, Bhamdipati R, Mullangi R. Infusion rate dependent pharmacokinetics of bendamustine with altered formation of gamma-hydroxybendamustine (M3) metabolite following 30- and 60-min infusion of bendamustine in rats. Drug Res. 2016;66:351–356. doi:10.1055/s-0042-105745.
- Cooke KR, Kobzik L, Martin TR, Brewer J, Delmonte JJ, Crawford JM, Ferrara JL. An experimental model of idiopathic pneumonia syndrome after bone marrow transplantation: I. The roles of minor H antigens and endotoxin. Blood. 1996;88(8):3230–3239. doi:10.1182/blood.V88.8.3230.bloodjournal8883230.
- Gatwood KS, Labopin M, Savani BN, Finke J, Socie G, Beelen D, Yakoub-Agha I, Chevallier P, Ganser A, Blaise D, et al. Transplant outcomes for patients with therapy-related acute myeloid leukemia with prior lymphoid malignancy: an ALWP of EBMT study. Bone Marrow Transplant. 2020;55(1):224–232. doi:10.1038/s41409-019-0673-3.
- Rubio MT, D’Aveni-Piney, M., Labopin, M., Hamladji, R.M., Sanz, M.A., Blaise, D., Ozdogu, H., Daguindeau, E., Richard, C., Santarone, S, et al. Impact of in vivo T cell depletion in HLA-identical allogeneic stem cell transplantation for acute myeloid leukemia in first complete remission conditioned with a fludarabine iv-busulfan myeloablative regimen: a report from the EBMT Acute Leukemia Working Party. J Hematol Oncol. 2017;10:31.
- Konuma T, Tsukada N, Kanda J, Uchida N, Ohno Y, Miyakoshi S, Kanamori H, Hidaka M, Sakura T, Onizuka M, et al. Comparison of transplant outcomes from matched sibling bone marrow or peripheral blood stem cell and unrelated cord blood in patients 50 years or older. Am J Hematol. 2016;91(5):E284–E292. doi:10.1002/ajh.24340.
- Holtan SG, DeFor TE, Lazaryan A, Bejanyan N, Arora M, Brunstein CG, Blazar BR, MacMillan ML, Weisdorf DJ. Composite end point of graft-versus-host disease-free, relapse-free survival after allogeneic hematopoietic cell transplantation. Blood. 2015;125(8):1333–1338. doi:10.1182/blood-2014-10-609032.
- Centuori SM, Trad M, LaCasse CJ, Alizadeh D, Larmonier CB, Hanke NT, Kartchner J, Janikashvili N, Bonnotte B, Larmonier N. Myeloid-derived suppressor cells from tumor-bearing mice impair TGF-β-induced differentiation of CD4+CD25+FoxP3+ Tregs from CD4+CD25-FoxP3- T cells. J Leukoc Biol. 2012;92:987–997. doi:10.1189/jlb.0911465.
- Zeng Y, Stokes J, Hahn S, Hoffman E, Katsanis E. Activated MHC-mismatched T helper-1 lymphocyte infusion enhances GvL with limited GvHD. Bone Marrow Transplant. 2014;49:1076. doi:10.1038/bmt.2014.91.
- Zeng Y, Hahn S, Stokes J, Hoffman EA, Schmelz M, Proytcheva M, Chernoff J, Katsanis E. Pak2 regulates myeloid-derived suppressor cell development in mice. Blood Adv. 2017;1:1923–1933. doi:10.1182/bloodadvances.2017007435.
- Kruisbeek AM, Shevach E, Thornton AM. Proliferative assays for T cell function. Curr Protoc Immunol. 2004;60(1):3. 12.11–13.12.20. doi:10.1002/0471142735.im0312s60.
- Kaplan EL, Meier P. Nonparametric estimation from incomplete observations. J Am Stat Assoc. 1958;53:457–481. doi:10.1080/01621459.1958.10501452.
- Peto R, Peto J. Asymptotically efficient rank invariant test procedures. J R Stat Soc Ser A (General). 1972;135:185–207. doi:10.2307/2344317.
- Taylor PA, Lees CJ, Blazar BR. The infusion of ex vivo activated and expanded CD4(+)CD25(+) immune regulatory cells inhibits graft-versus-host disease lethality. Blood. 2002;99:3493–3499. doi:10.1182/blood.V99.10.3493.
- Mutis T, van Rijn RS, Simonetti ER, Aarts-Riemens T, Emmelot ME, van Bloois L, Martens A, Verdonck LF, Ebeling SB. Human regulatory T cells control xenogeneic graft-versus-host disease induced by autologous T cells in RAG2−/−γc−/− Immunodeficient mice. Clin Cancer Res. 2006;12:5520. doi:10.1158/1078-0432.CCR-06-0035.
- Pierini A, Colonna L, Alvarez M, Schneidawind D, Nishikii H, Baker J, Pan Y, Florek M, Kim B-S, Negrin RS, et al. Donor requirements for regulatory T cell suppression of murine graft-versus-host disease. J Immunol. 2015;195(1):347. doi:10.4049/jimmunol.1402861.
- Blazar BR, Lindberg FP, Ingulli E, Panoskaltsis-Mortari A, Oldenborg P-A, Iizuka K, Yokoyama WM, Taylor PA. CD47 (integrin-associated protein) engagement of dendritic cell and macrophage counterreceptors is required to prevent the clearance of donor lymphohematopoietic cells. J Exp Med. 2001;194(4):541–549. doi:10.1084/jem.194.4.541.
- Zeiser R, Youssef S, Baker J, Kambham N, Steinman L, Negrin RS. Preemptive HMG-CoA reductase inhibition provides graft-versus-host disease protection by Th-2 polarization while sparing graft-versus-leukemia activity. Blood. 2007;110(13):4588–4598. doi:10.1182/blood-2007-08-106005.
- Malard F, Gaugler B, Lamarthee B, Mohty M. Translational opportunities for targeting the Th17 axis in acute graft-vs.-host disease. Mucosal Immunol. 2016;9:299–308. doi:10.1038/mi.2015.143.
- Blazar BR, Sharpe AH, Chen AI, Panoskaltsis-Mortari A, Lees C, Akiba H, Yagita H, Killeen N, Taylor PA. Ligation of OX40 (CD134) regulates graft-versus-host disease (GVHD) and graft rejection in allogeneic bone marrow transplant recipients. Blood. 2003;101(9):3741–3748. doi:10.1182/blood-2002-10-3048.
- Cassady K, Martin PJ, Zeng D. Regulation of GVHD and GVL activity via PD-L1 interaction with PD-1 and CD80. Front Immunol. 2018;9:3061. doi:10.3389/fimmu.2018.03061.
- Albring JC, Sandau MM, Rapaport AS, Edelson BT, Satpathy A, Mashayekhi M, Lathrop SK, Hsieh C-S, Stelljes M, Colonna M, et al. Targeting of B and T lymphocyte associated (BTLA) prevents graft-versus-host disease without global immunosuppression. J Exp Med. 2010;207(12):2551–2559. doi:10.1084/jem.20102017.
- Sabatos CA, Chakravarti S, Cha E, Schubart A, Sánchez-Fueyo A, Zheng XX, Coyle AJ, Strom TB, Freeman GJ, Kuchroo VK, et al. Interaction of Tim-3 and Tim-3 ligand regulates T helper type 1 responses and induction of peripheral tolerance. Nat Immunol. 2003;4(11):1102–1110. doi:10.1038/ni988.
- Zhang P, Hill GR. Interleukin-10 mediated immune regulation after stem cell transplantation: mechanisms and implications for therapeutic intervention. Semin Immunol. 2019;44:101322. doi:10.1016/j.smim.2019.101322.
- Henden AS, Hill GR. Cytokines in graft-versus-host disease. J Immunol. 2015;194(10):4604. doi:10.4049/jimmunol.1500117.
- Wilkinson AN, Chang K, Kuns RD, Henden AS, Minnie SA, Ensbey KS, Clouston AD, Zhang P, Koyama M, Hidalgo J, et al. IL-6 dysregulation originates in dendritic cells and mediates graft-versus-host disease via classical signaling. Blood. 2019;134(23):2092–2106. doi:10.1182/blood.2019000396.
- Yu H, Tian Y, Wang Y, Mineishi S, Zhang Y. Dendritic Cell Regulation of Graft-Vs.-Host Disease: immunostimulation and Tolerance. Front Immunol. 2019;10:93. doi:10.3389/fimmu.2019.00093.
- MacDonald KP, Shlomchik WD, Reddy P. Biology of graft-versus-host responses: recent insights. Biol Blood Marrow Trans. 2013;19(1):S10–S14. doi:10.1016/j.bbmt.2012.11.005.
- Priyadharshini B, Greiner DL, Brehm MA. T-cell activation and transplantation tolerance. Transplantation Reviews. 2012;26(3):212–222. doi:10.1016/j.trre.2011.09.002.
- Thangavelu G, Blazar BR. Achievement of tolerance induction to prevent acute graft-vs.-host disease. Front Immunol. 2019;10:309. doi:10.3389/fimmu.2019.00309.
- Bluestone JA. Mechanisms of tolerance. Immunol Rev. 2011;241(1):5–19. doi:10.1111/j.1600-065X.2011.01019.x.
- Brennan TV, Yang Y. PD-L1 serves as a double agent in separating GVL from GVHD. J Clin Invest. 2017;127(5):1627–1630. doi:10.1172/JCI94196.
- Burlion A, Brunel S, Petit NY, Olive D, Marodon G. Targeting the human T-cell inducible costimulator molecule with a monoclonal antibody prevents graft-vs-host disease and preserves graft vs leukemia in a xenograft murine model. Front Immunol. 2017;8:756. doi:10.3389/fimmu.2017.00756.
- Yi T, Chen Y, Wang L, Du G, Huang D, Zhao D, Johnston H, Young J, Todorov I, Umetsu DT, et al. Reciprocal differentiation and tissue-specific pathogenesis of Th1, Th2, and Th17 cells in graft-versus-host disease. Blood. 2009;114(14):3101–3112. doi:10.1182/blood-2009-05-219402.
- Murphy WJ, Welniak LA, Taub DD, Wiltrout RH, Taylor PA, Vallera DA, Kopf M, Young H, Longo DL, Blazar BR, et al. Differential effects of the absence of interferon-gamma and IL-4 in acute graft-versus-host disease after allogeneic bone marrow transplantation in mice. J Clin Invest. 1998;102(9):1742–1748. doi:10.1172/JCI3906.
- Nagaraj S, Gupta K, Pisarev V, Kinarsky L, Sherman S, Kang L, Herber DL, Schneck J, Gabrilovich DI. Altered recognition of antigen is a mechanism of CD8+ T cell tolerance in cancer. Nat Med. 2007;13(7):828. doi:10.1038/nm1609.
- Yang F, Li Y, Wu T, Na N, Zhao Y, Li W, Han C, Zhang L, Lu J, Zhao Y, et al. TNFα-induced M-MDSCs promote transplant immune tolerance via nitric oxide. J Mol Med (Berl). 2016;94(8):911–920. doi:10.1007/s00109-016-1398-z.
- Veenstra RG, Flynn R, Kreymborg K, McDonald-Hyman C, Saha A, Taylor PA, Osborn MJ, Panoskaltsis-Mortari A, Schmitt-Graeff A, Lieberknecht E, et al. B7-H3 expression in donor T cells and host cells negatively regulates acute graft-versus-host disease lethality. Blood. 2015;125(21):3335–3346. doi:10.1182/blood-2014-09-603357.
- Levine JE. Implications of TNF-α in the pathogenesis and management of GVHD. Int J Hematol. 2011;93:571–577. doi:10.1007/s12185-011-0803-1.
- D’andrea A, Aste-Amezaga M, Valiante NM, Ma X, Kubin M, Trinchieri G. Interleukin 10 (IL-10) Inhibits human lymphocyte interferon γ-production by suppressing natural killer cell stimulatory factor/IL-12 synthesis in accessory cells. J Exp Med. 1993;178:1041–1048. doi:10.1084/jem.178.3.1041.
- Chaudhry A, Samstein R, Treuting P, Liang Y, Pils M, Heinrich J-M, Jack R, Wunderlich F, Brüning J, Müller W, et al. Interleukin-10 signaling in regulatory T cells is required for suppression of Th17 cell-mediated inflammation. Immunity. 2011;34(4):566–578. doi:10.1016/j.immuni.2011.03.018.
- Park MJ, Lee SH, Lee S-H, Lee E-J, Kim E-K, Choi JY, Cho M-L. IL-1 receptor blockade alleviates graft-versus-host disease through downregulation of an interleukin-1beta-dependent glycolytic pathway in Th17 cells. Mediators Inflamm. 2015;2015:631384. doi:10.1155/2015/631384.
- Lu L, Yoshimoto K, Morita A, Kameda H, Takeuchi T. Bendamustine increases interleukin-10 secretion from B cells via p38 MAP kinase activation. Int Immunopharmacol. 2016;39:273–279. doi:10.1016/j.intimp.2016.07.033.
- Reshef R, Luger SM, Hexner EO, Loren AW, Frey NV, Nasta SD, Goldstein SC, Stadtmauer EA, Smith J, Bailey S, et al. Blockade of lymphocyte chemotaxis in visceral graft-versus-host disease. N Engl J Med. 2012;367:135–145. doi:10.1056/NEJMoa1201248.
- Reshef R, Ganetsky A, Acosta EP, Blauser R, Crisalli L, McGraw J, Frey NV, Hexner EO, Hoxie JA, Loren AW, et al. Extended CCR5 blockade for graft-versus-host disease prophylaxis improves outcomes of reduced-intensity unrelated donor hematopoietic cell transplantation: a phase II clinical trial. Biol Blood Marrow Transplant. 2019;25:515–521. doi:10.1016/j.bbmt.2018.09.034.
- Munchel AT, Kasamon YL, Fuchs EJ. Treatment of hematological malignancies with nonmyeloablative, HLA-haploidentical bone marrow transplantation and high dose, post-transplantation cyclophosphamide. Best Pract Res Clin Haematol. 2011;24(3):359–368. doi:10.1016/j.beha.2011.05.001.