ABSTRACT
Novel therapies are needed for effective treatment of AML. In the relapsed setting, prognosis is very poor despite salvage treatment with chemotherapy. Evidence suggests that leukemic stem cells (LSCs) cause relapse. The cell surface receptor CD123 is highly expressed in blast cells and LSCs from AML patients and is a potential therapeutic target. CD123 cross-over dual-variable domain T-cell engager (CD123-CODV-TCE) is a bispecific antibody with an innovative format. One arm targets the CD3εδ subunit of T-cell co-receptors on the surface of T cells, while the other targets CD123 on malignant cells, leading to cell-specific cytotoxic activity. Here, we describe the preclinical activity of CD123-CODV-TCE. CD123-CODV-TCE effectively binds to human and cynomolgus monkey CD3 and CD123 and is a highly potent T-cell engager. It mediates T-cell activation and T-cell-directed killing of AML cells in vitro. In vivo, CD123-CODV-TCE suppresses AML tumor growth in leukemia xenograft mouse models, where it achieves an effective half-life of 3.2 days, which is a significantly longer half-life compared to other bispecific antibodies with no associated Fc fragment. The in vitro safety profile is as expected for compounds with similar modes of action. These results suggest that CD123-CODV-TCE may be a promising therapy for patients with relapsed/refractory AML.
KEYWORDS:
Introduction
AML is the most common form of acute leukemia in adults and the leading cause of fatal leukemia in the United States. AML induction regimens typically include cytosine arabinoside and anthracyclines. Allogeneic stem cell transplant is offered to patients with high-risk disease in first or subsequent remissions.Citation1 Despite these aggressive therapeutic approaches, from 2008 to 2014, only 27.4% of patients with AML survived 5 years (SEER Cancer Statistics Review, 1975–2015). Relapse is common, and salvage treatment with cytotoxic chemotherapy is rarely curative.Citation2 First-line treatment has changed little over the last 30 years, although the recent approval of several new drugs, including midostaurin,Citation3 enasidenib,Citation4 and liposomal formulation of conventional cytotoxic therapy,Citation5 indicates a new era of AML therapy is beginning.
Evidence from in vitro and in vivo models suggests AML may derive from leukemic stem cells (LSCs) that give rise to leukemic blasts, and persistence of LSCs is hypothesized to cause relapse after an initial remission.Citation6–8 Thus, eradication of LSCs becomes an important therapeutic goal. CD123, the surface interleukin-3 (IL-3) receptor alpha chain, is expressed in normal hematopoietic cells and stimulates cell proliferation in response to IL-3. Interestingly, CD123 is very frequently overexpressed in AML blasts and LSCs;Citation9–14 indeed, it is 1 of only 7 transcripts in the AML gene expression signature that is overexpressed in leukemic blasts compared with normal hematopoietic cells from healthy controls.Citation15 The majority of the AML cells from human patients expresses IL-3 receptors and proliferates when cultured in the presence of IL-3.Citation16,Citation17 In most patients, CD123 is strongly expressed in LSCs and blasts, regardless of their cytogenetic or mutational profile.Citation18 High expression of CD123 on blast cells at diagnosis correlates with a higher blast count, a lower rate of complete response (36% vs 70%; P < .05), and a shorter median duration of complete response.Citation17–19 Thus, CD123 represents an attractive therapeutic target in patients with AML.Citation20
Herein, we evaluate the preclinical activity of a bispecific monoclonal antibody T-cell engager (TCE), CD123-cross-over dual-variable TCE (CD123-CODV-TCE), as a potential therapy for AML. One arm of CD123-CODV-TCE targets the CD3εδ subunit of the T-cell co-receptor at the surface of the T cell; the other targets CD123 on the malignant cell. Bispecific TCEs can co-engage T cells and CD123-positive malignant cells, leading to the formation of a cytolytic synapse,Citation21 which induces T-cell activation, resulting in tumor cell-specific killing. CD123-CODV-TCE is a potential therapeutic agent that demonstrates activity in vitro and in vivo, against human cell lines overexpressing CD123, as well as primary human AML samples including LSCs.
Materials and methods
Cell lines and human material
The human AML-derived cell lines expressing CD123 THP-1 and Molm-13 was obtained from Deutsche Sammlung von Mikroorganismen und Zellkulturen (Braunschweig, Germany). FreeStyle™ 293-F cells were obtained from Invitrogen (Invitrogen/Thermo Fisher Scientific, Waltham, MA, USA). EA.hy926 cells were obtained from ATCC (Manassas, VA, USA). Additional cell line descriptions and growth conditions are described in the Supplementary Information.
Fresh human peripheral blood mononuclear cells (PBMCs) were isolated from healthy donors’ whole blood samples supplied by EFS (Etablissement Français du Sang, Île-de-France, France), internal blood donation service after informed consent (Sanofi, Frankfurt, Germany), or by OneBlood® Blood Bank (Orlando, FL, USA) (Supplementary Information). The primary AML samples and patient histories were obtained from Groupe Ouest Est d’Etude des Leucémies et Autres Maladies du Sang (GOELAMS) (Supplementary Information). For all biologic samples, written consent was obtained, and samples were taken in accordance with the Declaration of Helsinki.
Engineering of CD123-CODV-TCE
CD123-CODV-TCE is a FC-fusion protein and was generated by engineering humanized anti-CD123 and anti-CD3 coding sequences into a bispecific CODV format. The heavy and the light chain of the CODV-Fab module were fused to a human immunoglobulin (Ig)G1-Fc backbone with reduced effector function. VL2-CD123 is separated from VL1-CD3 by a 10 amino acid linker (L1). An additional 10 amino acid linker (L2) was introduced to separate VL1-CD3 from the CL domain (). Moreover, the CD123 silenced-CODV-Fab control antibody used as negative control was generated as the CD123 bispecific CODV Fab antibody in which CD123 variable sequences were replaced by an irrelevant non-binding sequence. Production details of CD123-CODV-TCE and additional compounds are described in the Supplementary Information. Additional sequence information is described in patent (WO 2016 116626).
Figure 1. Structural design and biochemical properties of bispecific T-cell engager CD123-CODV-TCE. (a) Proprietary CODV format that has a fully humanized IgG1 backbone with reduced Fc functionality. VH1/VL1 and VH2/VL2 correspond to CD3 and CD123 domains, respectively. CODV, cross-over dual-variable domain; Ig, immunoglobulin; TCE, T-cell engager; VH, Ig heavy chain variable domain; VL, Ig light chain variable domain; CH, Ig heavy chain constant domain; CL, Ig light chain constant domain; L, 10 amino acid linker. (b) CD123-CODV-TCE binds to human CD3 and CD123 proteins. Recombinant human CD3εδ and human CD123 were immobilized on a His capture sensor chip. Binding affinity of CD123-CODV-TCE to the respective antigens was measured by surface plasmon resonance using Biacore T200 (GE Healthcare Life Sciences, Marlborough, MA, USA) in multicycle kinetics experiments. After injecting each dilution of CD123-CODV-TCE of a 1:2 dilution series in assay buffer to measure the association kinetics, followed by an injection of buffer to measure the dissociation kinetics, the sensor surface was regenerated by inject of a regeneration solution. Sensorgrams were fitted using a 1:1 Langmuir interaction model to generate association rate constant, dissociation rate constant, and equilibrium dissociation rate constant values. Representative sensorgrams for binding of CD123-CODV-TCE to human CD3εδ and CD123 are presented. Data shown are means ± standard deviation from 3 independent experiments. CODV, cross-over dual-variable domain; TCE, T-cell engager
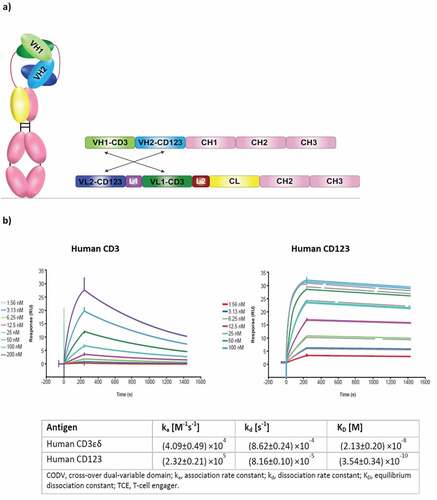
In vitro characterization
Assessment of CD3εδ and CD123 binding affinities using surface plasmon resonance
Binding affinities of CD123-CODV-TCE to CD3εδ and CD123 were measured using surface plasmon resonance with Biacore T200 (GE Healthcare Life Sciences, Marlborough, MA, USA) and 4-(2-hydroxyethyl)-1-piperazineethanesulfonic acid (HEPES)-buffered saline (HBS-EP+; GE Healthcare Life Sciences) assay buffer. Two-fold dilutions of CD123-CODV-TCE were performed in HBS-EP+ for CD3εδ-Fc-His (1.56–200 nM) and CD123-Fc-His (1.56–100 nM). The fusion proteins were captured using the His capture kit (GE Healthcare Life Sciences). Data were analyzed using Biacore T200 Evaluation software version 3.0 (GE Healthcare Life Sciences) and sensorgrams were fitted using a 1:1 Langmuir model to generate association rate constant (ka), dissociation rate constant (kd), and equilibrium dissociation constant (KD) values.
T-cell activation and cytotoxicity assays with THP-1 AML cell line
Briefly, for T-cell activation and cytotoxicity assays, isolated primary human T cells were isolated from healthy donors PBMC by negative selection (pan T cell isolation Kit, Miltenyi Biotech). Purified untouched T cells were seeded in 96-well culture plate in the presence and absence of the THP-1 target cells,Citation22 at an effector-to-target (E:T) ratio of 10:1 for a total volume of 100 µl. CD123-CODV-TCE or control was added at increasing concentrations for 20 h (Supplementary Information). To detect primary human T-cell activation, the percentage of CD69 + T cells was analyzed by flow cytometry.Citation23 For the cytotoxicity assay, the percentage of dead target cells was analyzed via live/dead staining by flow cytometry (Supplementary Information).
Cytotoxicity assay on primary AML
Primary AML bone marrow or blood cells from patients (Supplementary Table 1) were thawed one day prior to the assay and seeded in cell culture microplate at 106 AML cells/well. Apoptosis was measured by flow cytometry on day 0 (untreated) and on day 3 and 7 of culture in presence of CD123-CODV-TCE and CD123 silenced-CODV-Fab control at 0.025, 0.25, and 2.5 nM final concentrations. Annexin V-FITC (BD Biosciences Inc, San Jose, CA, USA) and TO-PRO-3 iodide (Molecular Probes, Eugene, OR, USA) were used to gate the apoptotic cell population.
Table 1. CD123-CODV-TCE induces cytokine release in healthy donor whole blood: Major responding cytokines. Whole-blood derivatives from 26 human donors were incubated for 20 h with CD123-CODV-TCE (0.03–300 ng/ml) using the VaxDesign MIMIC® system (Sanofi Pasteur, Orlando, FL, USA), followed by assessment of 41 cytokines/chemokines in the supernatant with Bioplex® technology (Bio-Rad, Hercules, CA, USA). For each donor, each cytokine, and each treatment concentration, the fold change of cytokine concentration vs baseline concentration were calculated. Major responding cytokines are defined as cytokines for which the fold change from baseline is greater than 250-fold for at least 1 CD123-CODV-TCE tested concentration and 1 donor of 26. For these cytokines, the higher CD123-CODV-TCE concentration (ng/ml) producing less than 250-fold change for all the donors is reported
Cytotoxic assay on CD123 positive normal blood cells
A flow cytometry-based cytotoxicity assay was performed on fresh human PBMCs isolated from healthy donors to evaluate the activity of CD123-CODV-TCE against human CD123 positive monocyte and plasmacytoid dendritic cells (pDCs) (Supplementary Information).
Cytokine release in a whole-blood–derived 3D culture assay
A preliminary evaluation of product safety was performed with the VaxDesign MIMIC® system (VaxDesign/Sanofi Pasteur, Swiftwater, PA, USA),Citation24 a relevant human in vitro model. Briefly, the effect of CD123-CODV-TCE on cytokine release was assessed in a 3D culture composed of extracellular matrix, the human endothelial cell line EA.hy926 and fresh red-blood cell (RBC) – depleted adult whole blood – but supplemented with 1:2 dilution of autologous plasma and serum-free media. After 20 h of incubation with increasing concentrations of CD123-CODV-TCE (from 0.03 to 300 ng/ml), the concentrations of 41 cytokines/chemokines were measured in the supernatant with Bioplex® technology (Bio-Rad, Hercules, CA, USA) (Supplementary Information). The experiment was reproduced with 26 different healthy donor samples.
In vivo characterization
All in vivo experiments were approved by the Sanofi Ethical Committee and conducted in accordance with local and institutional Laws, Ethics and guidance in AAALAC accredited facilities.
Efficacy in CD123+ Molm-13-luc disseminated AML model
Development of the Molm-13-luc disseminated AML xenograft model is described in the Supplementary Information. Human T cells were isolated from healthy donor PBMCs using the Pan T Cell Isolation Kit (Miltenyi Biotec). CD123-CODV-TCE anti-tumor activity was evaluated after AML engraftment in non-irradiated NOD.Cg-Prkdcscid Il2rgtm1Wjl/SzJ (NSG) mice (Charles River Laboratories, Saint-Germain-Nuelles, France). Female animals aged 6–8 weeks were implanted intravenously (IV) with 106/0.2 ml Molm-13-luc cells per mouse on day 0 and intraperitoneally implanted with 107/0.2 ml human T cells per mouse on day 1. Longitudinal in vivo bioluminescence imaging (BLI) was performed to monitor disseminated tumor growth. CD123-CODV-TCE was administered intravenously at test doses of 164, 16.4, and 1.64 µg/kg (1.3, 0.13, and 0.013 nmol/kg, respectively) every 3 days on days 4, 7, 10, and 13 (Q3Dx4) post-tumor implantations. Mice were sacrificed on day 14 and autopsied under BLI. Tumor regressions and growth inhibition in bone marrow, spleen, and ovary were validated by terminal histology (Supplementary Information).
Pharmacokinetic and biodistribution analysis in naive or Molm-13-luc/effector T cells-implanted NSG mice
89Zr-CD123-CODV-TCE preparation is described in the Supplementary Information. Pharmacokinetics and biodistribution were evaluated following a single bolus administration in naive NSG mice and in the Molm-13-luc disseminated xenograft AML model implanted in NSG mice engrafted with human effector T cells. 89Zr-CD123-CODV-TCE concentrations were calculated from total radioactivity measured by gamma counting in serial blood samples over time for pharmacokinetic characterization, and at necropsy in sampled organs for terminal biodistribution (Supplementary Information).Citation25
Efficacy in primary AML xenograft model
Chemo-resistant primary AML cells sample from patient #323 was selected from a panel of 5 AML patients’ samples according to immunophenotype, tumor engraftment rate in NOD.Cg-Prkdcscid Il2rgtm1Wjl/SzJ (NSG) mice, and in vitro sensitivity to CD123-CODV-TCE treatment. NSG mice were infused on day 0 with blood from patient #323 containing 106 blast cells and ~2 × 105 CD3+ autologous T cells (Supplementary Information).Citation26 164ug/kg or 16.4ug/kg CD123-CODV-TCE or phosphate buffered saline was administered intraperitoneally starting on Day1 and repeated every 3 to 4 days for 13 weeks. Animal weight was monitored weekly. From week 4, xenograft engraftment and blood counts were assessed weekly. At week 13, mice were sacrificed, and blood, spleen, and bone marrow samples were evaluated by flow cytometry (Supplementary Information).
Biostatistical analysis
In vitro results of relative half maximal effective concentrations (RelEC50) were obtained using the 4-parameter logistic model, according to Ratkovsky and Reedy (1986; Supplementary Information). The adjustment was obtained by non-linear regression using the Levenberg-Marquardt algorithm in SAS software.
Tumor growth based on bioluminescence signal curves over time was measured for each animal of each treatment group. For longitudinal in-vivo BLI data, a two non-parametric two-way analysis of variance-Type (ANOVA-Type), followed by two contrast analyses with Bonferroni-Holm adjustment for multiplicity were performed. For terminal ex-vivo BLI data, a one-way ANOVA with factor group on rank-transformed bioluminescence signal was performed. For both studies, descriptive statistics with medians ± median absolute deviation were provided by group and day of measurement.
For efficacy assessments in the primary AML xenograft model, longitudinal parameters were analyzed by a two-way ANOVA-Type, followed by a contrast analysis performed with Bonferroni-Holm correction. For terminal human CD45 percentage tissue infiltration in bone marrow and spleen, pairwise two-sided Wilcoxon tests vs control corrected with a Bonferroni-Holm adjustment were undertaken. For body weight, a two-way ANOVA followed by a Dunnett’s test was performed. Statistical significance thresholds were P ≤ 0.05. SAS® version 9.4 for Windows 7 (SAS Institute, Cary, NC, USA) was used for the analyses.
Results
CD123-CODV-TCE binds to CD3 and CD123 proteins
CD123-CODV-TCE is a bispecific antibody with a human IgG1 backbone that has been engineered to target CD3 and CD123 (); the molecular mass of the recombinant heterodimer is 126 kDa (Supplementary Figure 1). The bispecific antibody has been engineered to carry an L234A L235A double mutation (so-called LALA mutation) in the lower hinge region to reduce Fc effector function.Citation27 CD123-CODV-TCE bound to recombinant CD3 and CD123 proteins from human and cynomolgus monkey with similar affinities. The mean binding affinity of CD123-CODV-TCE to recombinant human CD3εδ and CD123 was 2.13 (±0.20) × 10−8 M and 3.54 (±0.34) × 10−10 M, respectively, presented with raw representative sensorgrams for binding of CD123-CODV-TCE (), while the mean binding affinity of CD123-CODV-TCE to recombinant cynomolgus monkey CD3εδ and CD123 was 3.40 (±0.67) × 10−8 M and 3.07 (±0.25) × 10−10 M, respectively (Supplementary Figure 2).
Figure 2. CD123-CODV-TCE mediates activation and cytotoxic activity of T cells against CD123-expressing THP-1 target cells and primary AML blasts in vitro. Isolated primary human T cells from healthy donors were seeded in the presence (solid black) or absence (dashed black) of THP-1 (E:T ratio 10:1) followed by incubation with CD123-CODV-TCE (0.025–2.5 nM) for 20 hours. (a) T-cell activation was assessed by measuring the CD69 expression in CD8+ or CD4 + T cells. (b) Cytotoxic activity was assessed by measuring the percentage of THP-1 cells staining for 7-amino-actinomycin D. Data presented are mean with SEM of 5 to 7 independent experiments. The solid gray line indicates the relative estimated concentration producing half effect (relEC50). (c) Flow cytometry CD123 expression of CD123 on THP-1 cells (anti-CD123-APC, Clone 7G3, BD Pharmingen #560087, Isotype IgG2a, κ, APC BD Pharmingen, # 555576). (d) Cytotoxic activity against primary human AML cells was evaluated in vitro. Unfractionated blood sample from human patient #378 and unfractionated bone marrow from the human patients #323, #132, and #11 were incubated with CD123-CODV-TCE or CD123 silenced-CODV-Fab control (0.025, 0.25, or 2.5 nM) or cell culture medium and assessed for apoptosis on day 0, 3, and 7 (black, blue, and green bars, respectively). Data shown are means with standard deviation of triplicates. AML, acute myeloid leukemia; CODV, cross-over dual-variable domain; E:T, effector to target; SEM, standard error of the mean
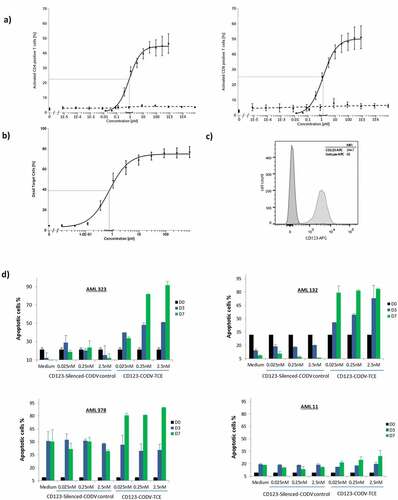
CD123-CODV-TCE mediates T-cell activation in vitro
CD123-CODV-TCE induced the activation of both human CD4+ and CD8 + T cells in the presence of THP-1 CD123-positive AML target cells in a concentration-dependent manner (). The relEC50 was 0.85 pM (95% CI 0.49; 1.5 pM) for CD4 + T cells, and 1.29 pM (95% CI: 0.69; 2.42 pM) for CD8 + T cells; T cell activation at CD123-CODV-TCE maximal concentration (7.94 × 104 pM) was estimated at 44.69% for CD4 + T cells, and 49.99% for CD8 + T cells (Supplementary Table 2). Even with a 100-fold increase TCE concentration, no T-cell activation was observed in the absence of target cells showing that binding to both CD3 and CD123 are necessary for T-cell activation ().
Table 2. Pharmacokinetic analysis in naive or Molm-13-luc/effector T cells-implanted NSG mice
CD123-CODV-TCE mediates potent cytotoxic activity of T cells against CD123-expressing THP-1 target cells and primary AML blasts in vitro
To assess the cytotoxicity of CD123-CODV-TCE, THP-1 cells exhibiting a consistent CD123 antigen expression were seeded in the presence of human T cells from seven different healthy donors. CD123-CODV-TCE stimulated human T cells to lyse THP-1 AML cells in a concentration-dependent manner with a relEC50 of 0.78 pM (95% CI: 0.54; 1.11) (, Supplementary Figure 3). Moreover, cytotoxicity assessment on three AML cell lines expressing different levels of CD123 showed a higher level of target cell killing in the AML cell line expressing the highest CD123 antigen density and suggested that CD123-CODV-TCE can induce cytotoxicity activity against AML cells with low CD123 expression. In addition, CD123-CODV-TCE does not lead to significant cytotoxic activity against K562 cell line which does not express CD123. In addition, CD123-silenced-CODV control antibody does not lead to activity at the highest tested concentration. (Supplementary Figure 4).
Figure 3a. CD123-CODV-TCE induces anti-leukemic effect in Molm-13-luc AML xenograft model in vivo. CD123-CODV-TCE was administered at 1.64, 16.4, and 164 µg/kg IV on days 4, 7, 10, and 13 post-tumor implantations in NSG mice bearing disseminated Molm-13-luc CD123+ AML cells in presence of human effector T cells. Global and long-bone tumor growth was followed by in vivo terminal bioluminescence imaging. (a) illustrates anti-tumor activity of CD123-CODV-TCE at all tested doses corroborated by longitudinal quantitation of photon flux for whole body (b) and long bones (log scale) (c). Anti-leukemic effect of CD123-CODV-TCE in spleen, lung and liver tissues was assessed by terminal ex-vivo BLI imaging. Graphs represent individual bioluminescent signal values associated with the median value for each group of treatment. Medians with median absolute deviation were represented (d). Tumor regression in long bones was validated by bone hematoxylin eosin saffron terminal histology (HSE) (e)
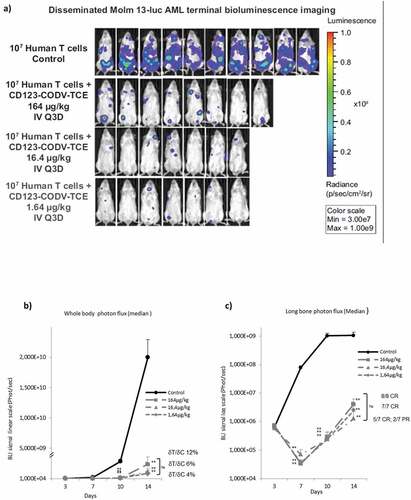
Figure 4. CD123-CODV-TCE depletes blast cells and LSCs in primary AML disseminated model in NSG mice in vivo. NSG mice were engrafted IV with whole blood from chemo-resistant AML patient #323. (a) AML engraftment was evaluated in each animal’s peripheral blood by ex-vivo flow cytometry using human CD45 as a marker. After week 9, tumor engraftment was detected only in the phosphate-buffered saline-treated control group. (b) At week 13, terminal detection by flow cytometry of the percentage of human CD45+ blast AML cells (left y-axis, solid dots), as well as the percentage of human LSCs (right Y-axis, empty dots) in blood, bone marrow, and spleen was determined. Blasts and LSCs were detected in peripheral blood, spleen, and bone marrow of control animals, but no LSCs or human CD45+ cells could be detected in these tissues in mice treated with CD123-CODV-TCE
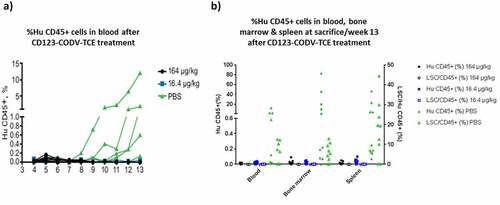
To investigate the CD123-CODV-TCE–mediated cytotoxicity against primary human AML cells, unfractionated blood from AML patient #378 and unfractionated bone marrow from AML patients #323, #343, #132, and #11 (Supplementary Table 3) were incubated with CD123-CODV-TCE or CD123 silenced-CODV-Fab control and assessed for apoptosis on days 0, 3, and 7. Cytotoxic activity of CD123-CODV-TCE was evaluable in 4 out of 5 patient samples. CD123-CODV-TCE, but not CD123 silenced-CODV-Fab control, which binds CD3 but not CD123 antigen, showed a dose-dependent cytotoxicity. For AML #323 and #132, induction of apoptosis was observed on days 3 and 7 in a concentration-dependent manner; for AML #378, in spite of a basal signal of 50% in medium and control on day 7, treatment led to >80% apoptosis at day 7 at all doses tested, while AML #11 showed a marginal response with 29.4% apoptosis at day 7 compared to 18.2% for control (). In AML #343, high spontaneous apoptosis prevented evaluation of CD123-CODV-TCE cytotoxic activity (Supplementary Figure 5, Supplementary Figure 6a). These primary AML samples exhibit variable CD123 expression levels (Supplementary Table 3 and Supplementary Figure 6b), these data suggest that CD123-CODV-TCE can induce cytotoxic activity against blasts with low CD123 expression (AML#378), bearing in mind that possibly other parameters, such as the number of T-cells and their status, that is, active or exhausted, could impact the response.
CD123-CODV-TCE mediates cytotoxic activity against CD123-expressing normal blood cells and induces cytokine release in whole blood
As part of the preliminary in vitro safety evaluation, we evaluated the CD123-CODV-TCE activity against circulating normal blood cells, pDCs and monocytes that show CD123 expression of 10,000 and 600 antigens per cell, respectively. CD123-CODV-TCE depleted monocytes and pDCs isolated from 6 to 11 healthy donors in a picomolar activity range with a mean relEC50 of 1.02 pM (95% CI: 0.48; 2.15 pM) and 0.51 pM (95% CI: 0.03; 7.99 pM) for monocytes and pDCs respectively (Supplementary Figure 7).
We next examined the CD123-CODV-TCE cytokine release profile in a physiologically relevant assay using whole blood from 26 different healthy donors (Supplementary Information). CD123-CODV-TCE significantly induced the release of 36 (out of a total of 41) cytokines (, Supplementary Table 4A). Of these cytokines, 22 exhibited a > 250-fold change from baseline for at least 1 donor () including the major predictors of cytokine release syndrome (CRS), interferon (IFN)γ, IL-1β, IL-2, IL-6, and tumor necrosis factor (TNF)α. Regarding the IL-6 cytokine, the 0.3 ng/ml CD123-CODV-TCE dose was the highest concentration at which the fold change in IL-6 from baseline was <250-fold for all the donors (from 4 to 140-fold; Supplementary Table 4B). Also, CD123-CODV-TCE activity showed a shifted IL-6 dose curve response with a higher median value compared with the positive control anti-CD3 OKT3 antibody (Supplementary Information), tested at the same concentration range (Supplementary Figure 8). High variability in response profiles among donors was noted, but this is consistent with observations made in other human cell-based assays to predict CRS.Citation28
Pharmacokinetics and biodistribution in naive and Molm-13-luc/effector T cells-implanted NSG mice
To determine the pharmacokinetic properties and the distribution in mice of CD123-CODV-TCE, the compound was radiolabeled with 89Zirconium isotope. After IV injection of 89Zr-CD123-CODV-TCE at 164 µg/kg in naive NSG mice, inter-individual concentration variability was low. The mean clearance was calculated to be 37.6 ml/day/kg, with an effective half-life (t1/2eff) of 3.5 days (); exposure (area under the blood concentration–time curve, calculated from 0 to infinity, [AUC]) was 4.41 µg equivalent * day per ml (µg.Eq.day/ml). In the Molm-13-luc/effector T cells-implanted NSG mice, the mean clearance was 75.9 ml/day/kg, with a t1/2eff of 3.2 days. AUC was 2.29 μg.Eq.day/ml ().
The tissue biodistribution of 89Zr-CD123-CODV-TCE was similar in naive and xenografted NSG mice (Supplementary Figure 9). In naive NSG mice, the concentration (mean concentration measurement/g of tissue) was low within muscle (0.018 µg equivalent per gram [μg.Eq/g]), almost undetectable in brain but prominent in spleen (0.293 μg.Eq/g), liver (0.264 μg.Eq/g), kidney (0.188 μg.Eq/g), and bone (0.170 μg.Eq/g). In Molm-13-luc/effector T cells-implanted NSG mice at 6 days post-injection, 89Zr-CD123-CODV-TCE concentration was low in muscle (0.019 μg.Eq/g), almost undetectable in brain but prominent in liver (0.415 μg.Eq/g), bone (0.236 μg.Eq/g), spleen (0.196 μg.Eq/g), and kidney (0.135 μg.Eq/g).
CD123-CODV-TCE induces anti-leukemic effect in Molm-13-luc AML xenograft model in vivo
CD123-CODV-TCE anti-tumor activity was evaluated in Molm-13-luc AML xenograft model in vivo. In vitro, similar relEC50 activity was found on Molm-13 AML cells compared to THP-1 cells (Supplementary Figure 10a). In order to achieve optimal anti-tumor activity, a schedule allowing continuous compound exposure based on the half-life of 3.2 days (see above) was applied. CD123-CODV-TCE administered IV every 3 days in the Molm-13-luc xenograft model in the presence of human effector T cells was well tolerated at all dosages. No evidence of adverse events or alterations of body weight were observed under treatment (Supplementary Figure 10b). As shown by BLI, CD123-CODV-TCE treatment-controlled AML tumor growth in the whole body and induced tumor regressions in the bone marrow compartment following co-injection of primary human T cells ().
CD123-CODV-TCE inhibited tumor growth throughout the whole body at all doses, with ratio of change in the tumor signal variations from baseline between treated and control groups (δT/δC) of 12% at 164 µg/kg (P = .0087), 6% at 16.4 µg/kg (P < .0001), and 4% at 1.64 µg/kg (P < .0001) at day 14 (). Tumor growth inhibition was associated with tumor regression in long bones (complete regression for 8/8 mice at 164 µg/kg [P = .0018 at day 14], 5/7 complete regression and 2/7 partial regression at 16.4 µg/kg [P < .0001], and 7/7 complete regression at 1.64 µg/kg [P < .0001]) (). No statistically significant difference among the three doses for both whole body and bone signals was demonstrated. Besides efficacy in the myeloid compartment, CD123-CODV-TCE significantly inhibited Molm-13-luc tumor development in all non-myeloid tissues colonized by the tumor in control animals except in abdominal fat and ovary, which exhibit apparent ex-vivo BLI signal; a partial recurrent signal in long bones at the end of the study was attributed to light diffusion from abdominal fat and ovary into long bone segmented region. At the terminal endpoint, CD123-CODV-TCE induced pronounced inhibition of tumor growth in spleen, liver, as well as in lung (). Terminal examination by hematoxylin eosin saffron confirmed BLI analysis: all five immunohistochemistry-tested control animals had bone marrow tumor colonization associated with osteolytic lesions and surrounding muscle invasion (Figure 3e), as well as tumor invasion of spleen and ovaries. In the seven CD123-CODV-TCE (1.64 µg/kg)-treated animals, no tumor was detected in spleen or in bone marrow of the right posterior leg in any animal, and only one presented residual tumor in the bone surrounding the muscle. Residual tumor was confirmed in the ovaries of one animal by terminal immunohistochemistry. CD123 silenced-CODV-Fab control failed to inhibit Molm-13-luc tumor growth (Supplementary Figure 11). Antitumor activity for CD123-CODV-TCE was also observed on the THP-1-luc disseminated AML model using a similar protocol (Supplementary Figure 12).
CD123-CODV-TCE depletes blast cells and LSCs in primary AML disseminated model in NSG mice in vivo
We finally assessed the in vivo activity of CD123-CODV-TCE on primary AML blasts and LSCs. In this study, using blood from the chemo-resistant AML patient #323, CD123-CODV-TCE treatment did not affect animal body weight but suppressed blast and LSC events number (as defined in Supplementary Information) in bone marrow at the 164- and 16.4-μg/kg doses. There was no major irreversible impact of the treatment on Hb concentration, white blood cells, and platelets count (Supplementary Figure 13). Tumor engraftment was detectable from week 9 in the phosphate-buffered saline–treated control group. At 13 weeks post-engraftment, leukemia was detected in the peripheral blood in 5/10 engrafted animals, in the spleen in 5/10 engrafted animals, and in the bone marrow in 7/10 engrafted animals. In these, seven latter control animals, engraftment consisted of 0.2%─83% infiltration of human CD45+ cells as 56%–93% blast cells (huCD45+, CD34+), including 2%–13% LSCs (huCD45+, CD34+, CD38low/–, CD123+) in bone marrow (Supplementary Figure 14). Significant anti-tumor activity was observed with complete primary AML tumor growth inhibition at both CD123-CODV-TCE doses by study end. No human CD45+ cells, including CD34+, CD38low/–, and CD123+ LSCs, were detected in blood, spleen or bone marrow following treatment with 164 and 16.4 μg/kg CD123-CODV-TCE, respectively. Complete AML tumor growth inhibition of human blast and leukemic stem cells was observed in peripheral blood, bone marrow, and spleen at study end with both tested doses of CD123-CODV-TCE (), Supplementary Figure 14).
Discussion
Elimination of CD123-expressing leukemic cells holds particular promise for patients with AML, as the majority of AML cells from human patients express IL-3 receptors, proliferate in response to IL-3, and a high expression of CD123 on blast cells at diagnosis is a negative prognostic indicator.Citation16,Citation17
Moreover, it has been suggested that the stem cell compartment is the origin of minimal residual disease (MRD).Citation8,Citation29 In fact, CD123 is one of the main biomarkers to monitor MRD.Citation30,Citation31 Importantly, the extent of MRD correlates with the probability of relapse and the risk of adverse outcomes both in adult and pediatric AML. Targeting LSCs may be a requirement for cure and is an important therapeutic goal, since eradication of LSC potentially may eliminate MRD and prevents relapse.
Here we assessed the preclinical activity of CD123-CODV-TCE, a potent bispecific antibody in a new format, which consists of anti-CD123 and anti-CD3 coding sequences engineered into a bispecific CODV arrangement.Citation32The CODV architecture provides a unique circular format that fixes the distance between antigen-binding moiety of the molecule and their relative orientation, which in turn facilitates concomitant antibody binding to large antigens on the surfaces of different cells.Citation33 CD123-CODV-TCE successfully induces activation of CD4+ and CD8 + T cells by co-engaging CD3 on donor-derived T cells and CD123 on leukemic cells. The highly potent (picomolar range) T-cell-dependent cytotoxicity of CD123-CODV-TCE against CD123+ target cells supports the efficacy of the CODV design.
We showed that CD123-CODV-TCE suppressed AML tumor growth in the bone marrow compartment of an in vivo disseminated human AML model (cell line or chemo-resistant primary AML blast including LSCs in bone marrow). The mouse xenograft model that we constructed recapitulated the late phase of AML in patients with involvement of both the myeloid and extra-myeloid compartments in tumor development. CD123-CODV-TCE exhibited anti-tumor activity against Molm-13-luc AML, with tumor regression observed in both compartments demonstrating the potential therapeutic applicability of CD123-CODV-TCE in patients with AML.
CD123-CODV-TCE is not the only engineered protein that targets CD123 with therapeutic potential for AML. Other agents target CD123, including monospecific monoclonal antibodies,Citation31,Citation34 fusion proteins,Citation35–38 and bispecific antibodies.Citation39–41 The advantage of these engineered proteins, compared to cellular therapies, such as CD123-directed chimeric antigen receptor T cells,Citation41,Citation42,Citation43,Citation44 is that they do not require lymphodepletion or apheresis, practices that may be difficult in patients who are heavily pre-treated or who present with additional comorbidities. Varying levels of efficacy have been seen after treatment with these agents. For example, monospecific antibodies targeting CD123 have been shown to kill human AML cells ex vivo and decrease AML burden in murine xenograft models,Citation10,Citation34 while a phase 1 trial of the anti-CD123 monospecific Ig1 CSL-360 in patients with relapsed or refractory AML found the agent was tolerated, with 2/40 patients responding.Citation31 Finally, CSL-362, a humanized version of CSL-360 that was engineered for increased affinity to CD16 (FcγRIIIa), demonstrated superior activity to CSL-360 in preclinical studies.Citation34 The first reports of CD123-directed TCE clinical data have been phase 1 data of flotetuzumab (MGD006).Citation45,Citation46 It shows efficacy in preclinical AML models.Citation39,Citation40 In addition, recent reports document flotetuzumab’s clinical efficacy, in patients with relapsed or refractory AML.Citation46–48
Despite promising clinical results, studies of TCEs have reported potentially severe toxicities, including CRS.Citation49 This side effect was reported for patients treated with the first approved antineoplastic CD19-directed CD3 bispecific TCE blinatumomabCitation50 and with the CD3xCD123 TCE, flotetuzumab.Citation40 Clinical CRS has been associated with an increase of IL-10, IL-6, and IFN-γCitation40,Citation51 in peripheral blood levels, while the side effect can be effectively managed with anti–IL-6 therapy, such as tocilizumab.Citation48 The in vitro safety assessment performed here demonstrated that CD123-CODV-TCE depleted CD123+ normal blood cells and induced the release of a wide range of cytokines into human blood, thus indicating potential risk of CRS induction. Similarly, preclinical evaluation revealed that flotetuzumab (MGD006) ex vivo treatment of PBMCs from AML patients, induced cytokine release, particularly IFN-γ and IL-6.Citation40 In addition, we have demonstrated the cross-reactivity of our compound for NHP antigens. Potential safety issue in this relevant species was conducted to design the clinical development of the compound. However, the clinical use of corticosteroids and anti–IL-6 blockade has already improved the management of patients with CRS.Citation52 Such an approach may be similarly considered for CD123-CODV-TCE.
Finally, TCEs lacking the Fc portion exhibit short terminal half-lives, e.g., 3.5 h for flotetuzumab in nonhuman primates,Citation53 and 1.25 h for blinatumomab in humans,Citation51 which mandates continuous IV infusion to maintain adequate drug levels. In this study, we demonstrated that CD123-CODV-TCE exhibits favorable pharmacokinetic properties in AML-bearing mice, with a predicted t1/2eff of 3.2 days, which allows effective control of AML dissemination with twice-weekly dosing. This favorable characteristic results from the format of CD123-CODV-TCE, which has been engineered to contain an Fc domain. This inclusion enables the protein to bind to the neonatal Fc receptor that salvages Fc-containing proteins from intracellular degradation.Citation54 CD123-CODV-TCE bio-distribution was prominent within liver, bone, spleen, and kidney, although there was no central nervous system penetration. This observation was expected; indeed, available estimates suggest that <0.1% of injected IgG reaches the brain after peripheral administration.Citation55
As CD123-CODV-TCE exhibits highly specific and potent cytotoxic activity against CD123-expressing leukemic blast and stem cells both in vitro and in vivo, with a favorable half-life of 3 days and an in vitro safety profile expected for such TCEs, CD123-CODV-TCE represents a potential candidate for future clinical development in AML and is currently being evaluated in a first-in-human clinical trial.
Author contributions
All authors contributed to the conception, design, data acquisition and analyses presented in this manuscript. All authors were involved in the drafting and development of the manuscript and provided approval of the final version. The authors are accountable for all aspects of the work and any questions related to the accuracy or integrity of any part of the work.
Supplemental Material
Download ()Acknowledgments
The research in this manuscript was funded by Sanofi. Editorial and medical writing assistance was provided by Joanne Franklin, PhD, CMPP, and Sandra Mendes, PhD, CMPP, TRM Oncology, The Hague, The Netherlands, funded by Sanofi.
We wish to thank E. Beys, A. Deslandes, A.L. Bauchet, N. Fagniez, A. Casse, Y. Baudat, F. Windenberger, M. Mangin, P. Delay-Goyet, L. Bassinet, A. Gaumet, A. Vergezac, and S. D’Heilly for their contribution and support.
Disclosure statement
HB, SG, JA, EJ, TDG, CB, CL, WDL, MS, CL, AC, CA, CB, AV, ER, PW, CC: Employees of Sanofi.
JS: employee of University Paris 7.
VBa: employee of University Versailles Saint Quentin en Yvelines and Assistance Publique Hôpitaux de Paris;
VBl, KH, DW, PGF: was an employee of Sanofi at the time this work was performed.
EL, PA, DRDIII: Employee of Sanofi-Pasteur, Orlando FL, USA.
Supplementary material
Supplemental data for this article can be accessed on the publisher’s website.
Additional information
Funding
Refernces
- Cassileth PA, Harrington DP, Appelbaum FR, Lazarus HM, Rowe JM, Paietta E, Willman C, Hurd DD, Bennett JM, Blume KG, et al. Chemotherapy compared with autologous or allogeneic bone marrow transplantation in the management of acute myeloid leukemia in first remission. N Engl J Med. 1998;339(23):1649–13. doi:10.1056/NEJM199812033392301.
- Breems DA, Van Putten WL, Huijgens PC, Ossenkoppele GJ, Verhoef GE, Verdonck LF, Vellenga E, De Greef GE, Jacky E, Van der Lelie J, et al. Prognostic index for adult patients with acute myeloid leukemia in first relapse. J Clin Oncol. 2005;23(9):1969–1978. doi:10.1200/JCO.2005.06.027.
- Stone RM, Mandrekar SJ, Sanford BL, Laumann K, Geyer S, Bloomfield CD, Thiede C, Prior TW, Döhner K, Marcucci G, et al. Midostaurin plus Chemotherapy for Acute Myeloid Leukemia with a FLT3 Mutation. N Engl J Med. 2017;377(5):454–464. doi:10.1056/NEJMoa1614359.
- Stein EM, DiNardo CD, Pollyea DA, Fathi AT, Roboz GJ, Altman JK, Stone RM, DeAngelo DJ, Levine RL, Flinn IW, et al. Enasidenib in mutant IDH2 relapsed or refractory acute myeloid leukemia. Blood. 2017;130(6):722–731. doi:10.1182/blood-2017-04-779405.
- Cortes JE, Goldberg SL, Feldman EJ, Rizzeri DA, Hogge DE, Larson M, Pigneux A, Recher C, Schiller G, Warzocha K, et al. Phase II, multicenter, randomized trial of CPX-351 (cytarabine:daunorubicin) liposome injection versus intensive salvage therapy in adults with first relapse AML. Cancer. 2015;121(2):234–242. doi:10.1002/cncr.28974.
- Corces-Zimmerman MR, Hong WJ, Weissman IL, Medeiros BC, Majeti R. Preleukemic mutations in human acute myeloid leukemia affect epigenetic regulators and persist in remission. Proc Natl Acad Sci U S A. 2014;111(7):2548–2553. doi:10.1073/pnas.1324297111.
- Hirsch P, Zhang Y, Tang R, Joulin V, Boutroux H, Pronier E, Moatti H, Flandrin P, Marzac C, Bories D, et al. Genetic hierarchy and temporal variegation in the clonal history of acute myeloid leukaemia. Nat Commun. 2016;7(1):12475. doi:10.1038/ncomms12475.
- van Rhenen A, Feller N, Kelder A, Westra AH, Rombouts E, Zweegman S, Van Der Pol MA, Waisfisz Q, Ossenkoppele GJ, Schuurhuis GJ, et al. High stem cell frequency in acute myeloid leukemia at diagnosis predicts high minimal residual disease and poor survival. Clin Cancer Res. 2005;11(18):6520–6527. doi:10.1158/1078-0432.CCR-05-0468.
- Cummins KD, Gill S. Anti-CD123 chimeric antigen receptor T-cells (CART): an evolving treatment strategy for hematological malignancies, and a potential ace-in-the-hole against antigen-negative relapse. Leuk Lymphoma. 2018;59(7):1539–1553. doi:10.1080/10428194.2017.1375107.
- Jin L, Lee EM, Ramshaw HS, Busfield SJ, Peoppl AG, Wilkinson L, Guthridge MA, Thomas D, Barry EF, Boyd A, et al. Monoclonal antibody-mediated targeting of CD123, IL-3 receptor alpha chain, eliminates human acute myeloid leukemic stem cells. Cell Stem Cell. 2009;5(1):31–42. doi:10.1016/j.stem.2009.04.018.
- De Waele M, Renmans W, Vander Gucht K, Jochmans K, Schots R, Otten J, Trullemans F, Lacor P, Van Riet I. Growth factor receptor profile of CD34 + cells in AML and B-lineage ALL and in their normal bone marrow counterparts. Eur J Haematol. 2001;66(3):178–187. doi:10.1034/j.1600-0609.2001.00320.x.
- Testa U, Riccioni R, Diverio D, Rossini A, Lo Coco F, Peschle C. Interleukin-3 receptor in acute leukemia. Leukemia. 2004;18(2):219–226. doi:10.1038/sj.leu.2403224.
- Bras AE, De Haas V, van Stigt A, Jongen-Lavrencic M, Beverloo HB, Te Marvelde JG, Zwaan CM, Dongen JJM, Leusen JHW, Velden VHJ, et al. CD123 expression levels in 846 acute leukemia patients based on standardized immunophenotyping. Cytometry B Clin Cytom. 2019;96(2):134–142. doi:10.1002/cyto.b.21745.
- Haubner S, Perna F, Kohnke T, Schmidt C, Berman S, Augsberger C, Schnorfeil FM, Krupka C, Lichtenegger FS, Liu X, et al. Coexpression profile of leukemic stem cell markers for combinatorial targeted therapy in AML. Leukemia. 2019;33(1):64–74. doi:10.1038/s41375-018-0180-3.
- Stirewalt DL, Meshinchi S, Kopecky KJ, Fan W, Pogosova-Agadjanyan EL, Engel JH, Cronk MR, Dorcy KS, McQuary AR, Hockenbery D, et al. Identification of genes with abnormal expression changes in acute myeloid leukemia. Genes Chromosomes Cancer. 2008;47(1):8–20. doi:10.1002/gcc.20500.
- Budel LM, Touw IP, Delwel R, Clark SC, Lowenberg B. Interleukin-3 and granulocyte-monocyte colony-stimulating factor receptors on human acute myelocytic leukemia cells and relationship to the proliferative response. Blood. 1989;74(2):565–571. doi:10.1182/blood.V74.2.565.565.
- Testa U, Riccioni R, Militi S, Coccia E, Stellacci E, Samoggia P, Latagliata R, Mariani G, Rossini A, Battistini A, et al. Elevated expression of IL-3Ralpha in acute myelogenous leukemia is associated with enhanced blast proliferation, increased cellularity, and poor prognosis. Blood. 2002;100(8):2980–2988. doi:10.1182/blood-2002-03-0852.
- Ehninger A, Kramer M, Rollig C, Thiede C, Bornhauser M, von Bonin M, Wermke M, Feldmann A, Bachmann M, Ehninger G, et al. Distribution and levels of cell surface expression of CD33 and CD123 in acute myeloid leukemia. Blood Cancer J. 2014;4(6):e218. doi:10.1038/bcj.2014.39.
- Vergez F, Green AS, Tamburini J, Sarry JE, Gaillard B, Cornillet-Lefebvre P, Pannetier M, Neyret A, Chapuis N, Ifrah N, et al. High levels of CD34+CD38low/-CD123+ blasts are predictive of an adverse outcome in acute myeloid leukemia: a Groupe Ouest-Est des Leucemies Aigues et Maladies du Sang (GOELAMS) study. Haematologica. 2011;96(12):1792–1798. doi:10.3324/haematol.2011.047894.
- Liu K, Zhu M, Huang Y, Wei S, Xie J, Xiao Y. CD123 and its potential clinical application in leukemias. Life Sci. 2015;122:59–64. doi:10.1016/j.lfs.2014.10.013.
- Offner S, Hofmeister R, Romaniuk A, Kufer P, Baeuerle PA. Induction of regular cytolytic T cell synapses by bispecific single-chain antibody constructs on MHC class I-negative tumor cells. Mol Immunol. 2006;43(6):763–771. doi:10.1016/j.molimm.2005.03.007.
- Tsuchiya S, Yamabe M, Yamaguchi Y, Kobayashi Y, Konno T, Tada K. Establishment and characterization of a human acute monocytic leukemia cell line (THP-1). Int J Cancer. 1980;26(2):171–176. doi:10.1002/ijc.2910260208.
- Marzio R, Mauel J, Betz-Corradin S. CD69 and regulation of the immune function. Immunopharmacol Immunotoxicol. 1999;21(3):565–582. doi:10.3109/08923979909007126.
- Dhir V, Fort M, Mahmood A, Higbee R, Warren W, Narayanan P, Wittman V. A predictive biomimetic model of cytokine release induced by TGN1412 and other therapeutic monoclonal antibodies. J Immunotoxicol. 2012;9(1):34–42. doi:10.3109/1547691X.2011.613419.
- Warnders FJ, Waaijer SJ, Pool M, Lub-de Hooge MN, Friedrich M, Terwisscha van Scheltinga AG, Deegen P, Stienen SK, Pieslor PC, Cheung HK, et al. Biodistribution and PET Imaging of Labeled Bispecific T Cell-Engaging Antibody Targeting EpCAM. J Nucl Med. 2016;57(5):812–817. doi:10.2967/jnumed.115.168153.
- Leyton JV, Williams B, Gao C, Keating A, Minden M, Reilly RM. MicroSPECT/CT imaging of primary human AML engrafted into the bone marrow and spleen of NOD/SCID mice using 111In-DTPA-NLS-CSL360 radioimmunoconjugates recognizing the CD123+/CD131- epitope expressed by leukemia stem cells. Leuk Res. 2014;38(11):1367–1373. doi:10.1016/j.leukres.2014.09.005.
- Wines BD, Powell MS, Parren PW, Barnes N, Hogarth PM. The IgG Fc contains distinct Fc receptor (FcR) binding sites: the leukocyte receptors Fc gamma RI and Fc gamma RIIa bind to a region in the Fc distinct from that recognized by neonatal FcR and protein A. J Immunol. 2000;164(10):5313–5318. doi:10.4049/jimmunol.164.10.5313.
- Finco D, Grimaldi C, Fort M, Walker M, Kiessling A, Wolf B, Salcedo T, Faggioni R, Schneider A, Ibraghimov A, et al. Cytokine release assays: current practices and future directions. Cytokine. 2014;66(2):143–155. doi:10.1016/j.cyto.2013.12.009.
- Bradbury C, Houlton AE, Akiki S, Gregg R, Rindl M, Khan J, Ward J, Khan N, Griffiths M, Nagra S, et al. Prognostic value of monitoring a candidate immunophenotypic leukaemic stem/progenitor cell population in patients allografted for acute myeloid leukaemia. Leukemia. 2015;29(4):988–991. doi:10.1038/leu.2014.327.
- Schuurhuis GJ, Heuser M, Freeman S, Bene MC, Buccisano F, Cloos J, Grimwade D, Haferlach T, Hills RK, Hourigan CS, et al. Minimal/measurable residual disease in AML: a consensus document from the European LeukemiaNet MRD working party. Blood. 2018;131(12):1275–1291. doi:10.1182/blood-2017-09-801498.
- Kandeel EZ, El Sharkawy N, Hanafi M, Samra M, Kamel A. Tracing leukemia stem cells and their influence on clinical course of adult acute myeloid leukemia. Clin Lymphoma Myeloma Leuk. 2020;20(6):383–393. doi:10.1016/j.clml.2019.11.018.
- Steinmetz A, Vallee F, Beil C, Lange C, Baurin N, Beninga J, Capdevilla C, Corvey C, Dupyu A, Ferrari P et al. CODV-Ig, a universal bispecific tetravalent and multifunctional immunoglobulin format for medical applications. MAbs. 2016;8(5):867–878. doi:10.1080/19420862.2016.1162932.
- He SZ, Busfield S, Ritchie DS, Hertzberg MS, Durrant S, Lewis ID, Marlton P, McLachlan AJ, Kerridge I, Bradstock KF, et al. A Phase 1 study of the safety, pharmacokinetics and anti-leukemic activity of the anti-CD123 monoclonal antibody CSL360 in relapsed, refractory or high-risk acute myeloid leukemia. Leuk Lymphoma. 2015;56(5):1406–1415. doi:10.3109/10428194.2014.956316.
- Busfield SJ, Biondo M, Wong M, Ramshaw HS, Lee EM, Ghosh S, Braley H, Panousis C, Roberts AW, He SZ, et al. Targeting of acute myeloid leukemia in vitro and in vivo with an anti-CD123 mAb engineered for optimal ADCC. Leukemia. 2014;28(11):2213–2221. doi:10.1038/leu.2014.128.
- Angelot-Delettre F, Roggy A, Frankel AE, Lamarthee B, Seilles E, Biichle S, Royer B, Deconinck E, Rowinsky EK, Brooks C, et al. In vivo and in vitro sensitivity of blastic plasmacytoid dendritic cell neoplasm to SL-401, an interleukin-3 receptor targeted biologic agent. Haematologica. 2015;100(2):223–230. doi:10.3324/haematol.2014.111740.
- Fan D, Li Z, Zhang X, Yang Y, Yuan X, Zhang X, Yang M, Zhang Y, Xiong D. AntiCD3Fv fused to human interleukin-3 deletion variant redirected T cells against human acute myeloid leukemic stem cells. J Hematol Oncol. 2015;8(1):18. doi:10.1186/s13045-015-0109-5.
- Zhang Y, Liu R, Fan D, Shi R, Yang M, Miao Q, Deng ZQ , Qian J , Zhen Y , Xiong D , Wang J. The novel structure make LDM effectively remove CD123+ AML stem cells in combination with interleukin 3. Cancer Biol Ther. 2015;16(10):1514–1525. doi:10.1080/15384047.2015.1071733.
- Frankel AE, Woo JH, Ahn C, Pemmaraju N, Medeiros BC, Carraway HE, Frankfurt O, Forman SJ, Yang XA, Konopleva M, et al. Activity of SL-401, a targeted therapy directed to interleukin-3 receptor, in blastic plasmacytoid dendritic cell neoplasm patients. Blood. 2014;124(3):385–392. doi:10.1182/blood-2014-04-566737.
- Al-Hussaini M, Rettig MP, Ritchey JK, Karpova D, Uy GL, Eissenberg LG, Gao F, Eades WC, Bonvini E, Chichili GR, et al. Targeting CD123 in acute myeloid leukemia using a T-cell-directed dual-affinity retargeting platform. Blood. 2016;127(1):122–131. doi:10.1182/blood-2014-05-575704.
- Chichili GR, Huang L, Li H, Burke S, He L, Tang Q, Jin L, Gorlatov S, Ciccarone V, Chen F, et al. A CD3xCD123 bispecific DART® for redirecting host T cells to myelogenous leukemia: preclinical activity and safety in nonhuman primates. Sci Transl Med. 2015;7(289):289ra82.
- Slade MJ, Uy GL. CD123 bi-specific antibodies in development in AML: what do we know so far? Best Pract Res Clin Haematol. 2020;33(4):101219. doi:10.1016/j.beha.2020.101219.
- Mardiros A, Dos Santos C, McDonald T, Brown CE, Wang X, Budde LE, Hoffman L, Aguilar B, Chang W-C, Bretzlaff W, et al. T cells expressing CD123-specific chimeric antigen receptors exhibit specific cytolytic effector functions and antitumor effects against human acute myeloid leukemia. Blood. 2013;122(18):3138–3148. doi:10.1182/blood-2012-12-474056.
- Pizzitola I, Anjos-Afonso F, Rouault-Pierre K, Lassailly F, Tettamanti S, Spinelli O, Biondi A, Biagi E, Bonnet D. Chimeric antigen receptors against CD33/CD123 antigens efficiently target primary acute myeloid leukemia cells in vivo. Leukemia. 2014;28(8):1596–1605. doi:10.1038/leu.2014.62.
- Arcangeli S, Rotiroti MC, Bardelli M, Simonelli L, Magnani CF, Biondi A, Breems DA. Balance of Anti-CD123 Chimeric Antigen Receptor Binding Affinity and Density for the Targeting of Acute Myeloid Leukemia. Mol Ther. 2017;25(8):1933–1945. doi:10.1016/j.ymthe.2017.04.017.
- Uy GL, Godwin J, Rettig MP, Vey N, Foster M, Arellano ML, Rizzieri DA, Topp MS, Huls G, Lowenberg B, et al. Preliminary results of a phase 1 study of flotetuzumab, a CD123 x CD3 Bispecific Dart® protein, in patients with relapsed/refractory acute myeloid leukemia and myelodysplastic syndrome. ASH; Atlanta (GA.): Blood; 2017. 637.
- Vey N, Davidson-Moncada JK, Uy GL, Rizzieri D, Khoury HJ, Foster MC, Godwin JE, Topp MS, Martinelli G, Ciceri F et al. A phase I, first-in-human study of MGD006/S80880 (CD123 x CD3 DART®) in AML/MDS. Journal of Clinical Oncology. 2017;35(15_suppl): TPS7070–TPS.
- Uy GL, Rettig MP, Vey N, Godwin J, Foster MC, Rizzieri DA, Arellano ML, Topp MS, Huls G, Jongen-Lavrencic M, et al. Phase 1 cohort expansion of flotetuzumab, a CD123×CD3 Bispecific Dart® protein in patients with relapsed/refractory Acute Myeloid Leukemia (AML). ASH Blood. 2018; 132(1_suppl):764.
- Uy GL, Aldoss I, Foster MC, Sayre PH, Wieduwilt MJ, Advani AS, Godwin JE, Arellano ML, Sweet KL, Emadi A, et al. Flotetuzumab as salvage immunotherapy for refractory acute myeloid leukemia. Blood. 2021;137(6):751–762. doi:10.1182/blood.2020007732.
- Shimabukuro-Vornhagen A, Godel P, Subklewe M, Stemmler HJ, Schlosser HA, Schlaak M, Kochanek M, Böll B, von Bergwelt-Baildon MS. Cytokine release syndrome. J Immunother Cancer. 2018;6(1):56. doi:10.1186/s40425-018-0343-9.
- Sanford M. Blinatumomab: first global approval. Drugs. 2015;75(3):321–327. doi:10.1007/s40265-015-0356-3.
- Klinger M, Brandl C, Zugmaier G, Hijazi Y, Bargou RC, Topp MS, Gökbuget N, Neumann S, Goebeler M, Viardot A, et al. Immunopharmacologic response of patients with B-lineage acute lymphoblastic leukemia to continuous infusion of T cell-engaging CD19/CD3-bispecific BiTE antibody blinatumomab. Blood. 2012;119(26):6226–6233. doi:10.1182/blood-2012-01-400515.
- Maude SL, Barrett D, Teachey DT, Grupp SA. Managing cytokine release syndrome associated with novel T cell-engaging therapies. Cancer J. 2014;20(2):119–122. doi:10.1097/PPO.0000000000000035.
- Campagne O, Delmas A, Fouliard S, Chenel M, Chichili GR, Li H, Alderson R, Scherrmann JM, Mager DE. Integrated pharmacokinetic/pharmacodynamic model of a bispecific CD3xCD123 DART molecule in nonhuman primates: evaluation of activity and impact of immunogenicity. Clin Cancer Res. 2018;24(11):2631–2641. doi:10.1158/1078-0432.CCR-17-2265.
- Roopenian DC, Akilesh S. FcRn: the neonatal Fc receptor comes of age. Nat Rev Immunol. 2007;7(9):715–725. doi:10.1038/nri2155.
- Pepinsky RB, Shao Z, Ji B, Wang Q, Meng G, Walus L, Lee X, Hu Y, Graff C, Garber E, Meier W et al. Exposure levels of anti-LINGO-1 Li81 antibody in the central nervous system and dose-efficacy relationships in rat spinal cord remyelination models after systemic administration. J Pharmacol Exp Ther. 2011;339(2):519–529. doi:10.1124/jpet.111.183483.