ABSTRACT
Radiotherapy can boost the therapeutic response to immune checkpoint inhibitors (ICIs) by recruiting T lymphocytes and upregulating PD-L1 expression within the tumor microenvironment (TME). However, in some cases, tumor PD-L1 expression cannot be induced, even in the presence of abundant T lymphocytes, in locally advanced colorectal cancer patients who receive preoperative neoadjuvant concurrent chemoradiotherapy (CCRT). In this study, we found that PD-L1 promoter methylation is negatively correlated with tumor PD-L1 expression and is an independent biomarker for locally advanced colorectal cancer patients. PD-L1 methylation (mCD274) was significantly associated with shorter disease-free survival (cg15837913 loci, p = .0124). By multivariate Cox proportional hazards analyses including influent factors, mCD274 was classified as an independent prognostic factor for poor 5-year DFS [cg15837913, hazard ratio: HR = 4.06, 95% CI = 1.407–11.716, p = .01]. We found that the immunomodulatory agent DNA methyltransferase inhibitor (DNMTi) led to demethylation of the PD-L1 promoter and increased radiotherapy-induced PD-L1 upregulation via interferon β (IFNβ). DNMTi not only induced tumor PD-L1 expression but increased the expression of immune-related genes as well as intratumoral T cell infiltration in vivo. Furthermore, DNMTi strongly enhanced the response to combined treatment with radiotherapy and anti-PD-L1 inhibitors, and prolonged survival in microsatellite stability (MSS) colorectal model. Therefore, DNMTi remodeled the tumor microenvironment to improve the effect of radiotherapy and anti-PD-L1 immunotherapy by directly triggering tumor PD-L1 expression and eliciting stronger immune responses, which may provide potential clinical benefits to colorectal cancer patients in the future.
Introduction
Colorectal cancer (CRC) is one of the death-related malignancies worldwide. The current treatments for CRC patients are radiotherapy, chemotherapy, targeted therapy and concurrent chemoradiotherapy (CCRT). For patients with locally advanced rectal carcinoma (LARC), preoperative CCRT has been utilized as the standard treatment to downstage tumor size for surgery. Even with the better response to CCRT in LARC patients, the occurrence of distant metastasis within 5 years is still high.Citation1,Citation2 Therefore, there is an urgent need to improve clinical efficacy by exploring new therapeutic targets for CRC, such as immune checkpoint inhibitors (ICIs).
Type I interferons (IFNs) play critical roles in tumor control by promoting dendritic cell (DC) maturation and antigen cross-priming for T cell activation.Citation3 Cancer cell autonomous type I IFN signaling controls therapeutic response to treatments including chemotherapy,Citation3 radiotherapyCitation4 and immunotherapy.Citation5 Radiotherapy effectively induces the secretion of type I IFN within the tumor microenvironments to generate anti-tumor immunity by activating and recruiting CD8+ T cell infiltration,Citation6 which is associated with a favorable prognosis in CRCCitation7 and are more responsive to immunotherapies.Citation8,Citation9 Therefore, radiotherapy immunologically reinvigorates “cold” TME to “inflammatory” TME for anti-tumor immune response. But CRC represents highly heterogeneous diseases characterized by genetic and epigenetic alterations such as genetic mutations and DNA methylation to regulate gene expression, tumor suppressor silencing and genomic stability, which is commonly dysregulated in cancer. DNA methylation has been reported to regulate PD-L1 expression in various malignancies including prostate cancer, colorectal cancer, acute myeloid leukemia and melanoma,Citation10–12 which conversely correlates with its expression and survival outcome. Therefore, reinvigorating T cell immunity for a suitable immunological TME to improve the efficacy of ICB by combinational therapeutics such as epigenetic therapy is urgently needed.Citation13
Epigenetic modulation by DNA methyltransferase inhibitors (DNMTi) has been reported to create long-lasting effects on anti-tumor immunity in non-small cell lung cancer (NSCLC).Citation14 Our previous studies showed that DNMTi can upregulate a wide range of genes involved in immune signaling in CRC, and increase the therapeutic response to anti-PD-L1 inhibitors.Citation15 In this study, we identify that methylation at PD-L1 promoter (loci cg15837913 and cg19724470) reduces the therapeutic efficacy of CCRT for LARC patients and is associated with poor survival outcome. DNMTi directly demethylates the PD-L1 promoter at these two loci. Moreover, DNMTi promotes the radiotherapy-induced type I IFN production and increases tumor immunogenicity. The IFNβ-activated STAT1/2 signaling can easily access PD-L1 promoter for its upregulation. These results indicate that DNMTi can improve cancer immunogenicity by remodeling the TME to enhance the infiltration of immune cells and improve the therapeutic efficacy of chemoradiotherapy in vivo. More importantly, our results showed that this TME remodeling improves the response to ICIs in vivo, which may benefit most CRC patients receiving immunotherapy, especially those with immunotherapy-refractory microsatellite stability (MSS)-CRC tumors.
Materials and methods
Cell culture and reagents
The human colon cancer cell lines SW620, HCT116 and SW480 and the mouse colon carcinoma CT26 cell line were obtained from the Bioresource Collection and Research Center (Hsinchu, Taiwan). Cells were cultured in a 37°C incubator with a humidified atmosphere of 5% CO2 and 95% air with RPMI 1640 growth medium supplemented with 10% fetal bovine serum (HyClone, California, USA), 3.5 g/L glucose, 10 mM HEPES and 1 mM sodium pyruvate.
The HA-DNMT1 overexpression plasmid was purchased from Addgene (Cambridge, MA, USA). Lentiviruses carrying shRNAs against DNMT1 were obtained from the National Core Facility for Manipulation of Gene Function by RNAi, miRNA, miRNA sponges, and CRISPR/Genomic Research Center (Academia Sinica, Taipei, Taiwan). Decitabine (2′-deoxy-5-azacytidine, DAC), azacitidine (5-Azacytidine, AZA) and STAT1 inhibitor fludarabine were purchased from Santa Cruz Biotechnology (CA, USA), and the INFAR1 inhibitor IFN alpha-IFNAR-IN-1 was obtained from MedChemExpress (NJ, USA). Recombinant human IFNβ protein was purchased from Sino Biological (Beijing, China).
The following antibodies were used in this study: anti-β-actin (sc-8432, Santa Cruz, CA, USA), anti-PD-L1 (ab205921, clone 28–8, Abcam, Cambridge, UK), anti-PD-L1 (#13684, clone E1L3N, Cell Signaling Technology, MA, USA), anti-DNMT1 (sc-271729, Santa Cruz), anti-p-STAT1 (sc-8394, Santa Cruz), anti-STAT1 (sc-464, Santa Cruz) anti-GAPDH (sc-25778, Santa Cruz), and anti-IRF9 (sc-514544, Santa Cruz). Horseradish peroxidase (HRP)-conjugated secondary antibodies were purchased from Santa Cruz Biotechnology.
For lentivirus production, 293 T packaging cells were cotransfected with packaging plasmids pCMV-Δ8.91, pMD.G mix and lentiviral vectors using jetPRIME transfection reagent (Polyplus transfection, NY, USA). Viruses were harvested 48–72 hrs later and filtered through a 0.45-μm filter to infect target cells.
Western blot analysis
Total lysates (30 μg) were resolved in an SDS-PAGE gel and transferred onto PVDF membranes (Millipore, MA, USA)Citation16,Citation17 for immunoblotting analysis with the indicated antibodies overnight at 4°C and probed with HRP-conjugated secondary antibodies for 2 hrs at room temperature. All antibodies were prepared with T-Pro Protein Free Blocking Buffer (BioLion Tech., Taipei, Taiwan).
The blot membrane was then incubated with Immobilon Western Chemiluminescent HRP Substrate (Millipore, CA, USA), visualized by an ImageQuant™ LAS 4000 biomolecular imager (GE Healthcare, Amersham, UK), processed using Adobe Photoshop and quantified using ImageJ software (NIH, MD, USA). Each blot was stripped by immunoblotting stripping buffer (BioLion Tech.) before incubation with the other antibodies.
qRT-PCR
Total RNA was extracted from cell lines with TRIzol (Invitrogen, CA, USA), quantitated by measuring the absorbance at 260 nm, and then reverse-transcribed into cDNA by iScript™ Reverse Transcription Supermix (Bio-Rad, CA, USA) according to the manufacturer’s instructions. Primers were designed by the Primer design tool (NCBI, USA) according to sequence information from the NCBI database. qRT-PCR was performed in a final reaction volume of 20 μL with iQ™ SYBR® Green Supermix (Bio-Rad, CA, USA) in the CFX96 Touch Real-Time PCR Detection System (Bio-Rad). All reactions were performed in triplicate for each sample, and GAPDH was employed as a reference gene for normalization. The relative values of gene expression were calculated using the 2−ΔΔCt method. The comparisons of gene expression levels were performed using the t-test.
Flow cytometry
SW480 and HCT116 cells were treated with the indicated chemotherapeutic drugs for 24 hrs, and then the cells were collected with dissociation buffer (Thermo Fisher Scientific, CA, USA). The cells were blocked with 5% BSA and then stained with an APC-conjugated anti-PD-L1 antibody (clone 29E.2A3, BioLegend, CA, USA). All events were acquired by a BD LSR-II flow cytometer equipped with BD FACSDiva software (BD Biosciences, Mountain View, CA, USA), and data were analyzed using FlowJo software (TreeStar, Ashland, Oregon, USA).
Concurrent chemoradiotherapy (CCRT) animal model
BALB/c mice (female, 4 weeks old) were maintained according to the institutional guidelines approved by the China Medical University Institutional Animal Care and Use Committee [Protocol No. CMUIACUC-2018-167]. In brief, CT26 cells (5 × 105 cells/mouse) were suspended in 100 μL of 50% Matrigel and inoculated subcutaneously into the right leg of each mouse. After 7 days, the mice were administered 5-FU (5 mg/kg) and AZA (0.5 mg/kg) for 2 cycles with consecutive 5-day and 1-day intervals between injections. Irradiation (5 Gy) was carried out on days 9 and 16. The tumor volume was measured every 3 days throughout the study.
The longest and shortest diameters (L and W, respectively) of the tumors were measured using Vernier calipers (Sata, Shanghai, China) every 3 days, and tumor volume (V) was calculated by the formula: V = (L × W2)/2. The mice were sacrificed at the termination of the experiments, and the tumor tissues were collected for lysis, subjected to immunoblotting analysis and stained by immunohistochemistry.
Immunohistochemistry
The antibodies used in this study were as follows: anti-human CD8 (ab4055, Abcam), anti-human IFNβ(NBP1-77288, Novus Biologicals, CO, USA), anti-human PD-L1 (ab205921, Abcam), anti-mouse CD3 (BioLegend) and anti-mouse CD8a (BioLegend). Tissue slides were deparaffinized and incubated for 10 min with 3% H2O2 in water to quench endogenous peroxidase activity and heat antigen retrieval by Antigen Unmasking Solutions (H3300, Vector Laboratories, Burlingame, CA). Tissue slides (3-µm thickness) were stained with the HRP-conjugated avidin biotin complex (ABC) from the Vectastain Elite ABC Kit (Vector Laboratories, Burlingame, CA) and DAB chromogen (Vector Laboratories) and counterstained with hematoxylin.
Staining for CD3 and CD8a was positive when detected in the tumor-infiltrating lymphocytes (TILs) and was evaluated using a microscope (OLYMPUS BX53, Tokyo, Japan). With respect to the detection of TILs, the tissue was reviewed at 40× magnification, and the area with the highest density of CD8+ TILs within the malignant cells was counted at 400× magnification (no. of TILs/high-power field). The average number of CD8+ TILs in five high-power fields was included in the evaluation.Citation7 The tumor IFNβ and PD-L1 staining patterns were evaluated and scored based on the intensity and percentage of positive cells for histoscore (H-score), which was calculated by a semiquantitative assessment of both the intensity of the staining (0: negative staining; 1: weak; 2: moderate; and 3: strong) and the percentage of immunopositive cells. The range of the H-score was from 0 to 300. The expression status was categorized as low or high according to the median value of the H-score.Citation15
RNA sequencing (RNA-seq) and data analysis
Total RNA was extracted from the resected tumors of the PBS, CCRT (5-FU+IR), AZA and CCRT/AZA groups on day 5 (1 day after completing AZA treatment) using TRIzol reagent. RNA quality was checked by an Agilent 2100 Bioanalyzer System (Santa Clara, CA, USA). mRNA was extracted from the total RNA using oligo (dT) magnetic beads and sheared into short fragments of approximately 200 bases. These fragmented mRNAs were then used as templates for cDNA synthesis. The cDNAs were subsequently amplified to prepare a complete library using PCR, and the cDNA library was subjected to paired-end sequencing using an Illumina HiSeqTM 2500 platformCitation18
Clean reads were obtained by removing low-quality reads and/or trimming the adaptor sequences from raw reads. The clean reads were employed for gene expression analysis. The mapped reads were used for further transcript annotation and for calculating expression levels using the fragments per kilobase per million reads (FPKM) method and the HTseq software package (version 0.6.1). DEGs were identified with the DESeq software package (version 1.12.0). The Benjamini-Hochberg false discovery rate was employed to correct the p values. Only genes with a p value < .05 were considered to be differentially expressed. The k-means method was used to cluster the DEGs based on their relative expression levels.Citation18
Patient tissue specimens and clinicopathological characteristics
The patient tissue samples used in our study were approved by the Institutional Research Ethics Committee at the China Medical University Hospital [protocol number: CMUH107-REC2-008], and the patients were informed prior to the use of their clinical material. Seventy patients were histologically and clinically diagnosed at the China Medical University Hospital between 2006 and 2014 (). The patients received preoperative CCRT and then surgery. The patients were followed up once every 6 months during the first and third postoperative years and once a year beginning in the fourth postoperative year. Patients who did not have follow-up information were excluded from this study.Citation19
Table 1. Characteristics of PD-L1 promoter locus in the LARC patients who received preoperative CCRT
DNA extraction, sodium bisulfite treatment and pyrosequencing
Genomic DNA from non-tumor and tumor tissues of rectal cancer patients was extracted from 3–5 (5-μm-thick) sequential sections of FFPE slides using a QuickExtract™ FFPE DNA Extraction Kit (QEF81050, Epicenter, WI, USA) according to the manufacturer’s instructions. All genomic DNA samples, as well as synthetic controls for methylated and unmethylated status, underwent bisulfite modification using the Epitect Bisulfite Kit (Qiagen).
Pyrosequencing assays were performed for all the study samples both on a PyroMark Q24 MDx and on a PyroMarkQ96 ID using PyroMark Gold reagents (Qiagen). Primers for PD-L1, targeting 2 CpGs (cg15837913 and cg19724470) in the gene promoter, were chosen according to the literature,Citation12,Citation15 and designed according to PyroMark Assay Design software version 2.0 (Qiagen). PCR analyses were performed in a total volume of 35 μl containing 1X buffer (KCl), 2 mM MgCl2, 200 μM dNTPs, 0.5 μM of each primer, 1.75 U Taq polymerase and 6 μl of bisulfite-modified DNA with the following cycling profile: 95°C for 1 min followed by 45 cycles of denaturation at 95°C for 1 min, annealing at the specific temperature for 1 min, and extension at 72°C for 1 min. Extension at 72°C for 10 min was finally performed. Amplicons were analyzed by gel electrophoresis on a 2% agarose gel stained with SafeView and visualized by ultraviolet trans-illumination. The residual PCR product (28 μl for AQ mode, 20 μl for CpG mode) was added to distilled water (12 μl for AQ mode, 18 μl for CpG mode) and incubated under shaking with binding buffer pH 7.6 (37 μl for AQ mode, 40 μl for CpG mode) containing 10 mM Tris-HCl, 2 M NaCl, 1 mM EDTA, and 0.1% Tween 20, added with Sepharose beads (3 μl for AQ mode, 2 μl for CpG mode) covered by streptavidin. PCR products were washed with 70% ethanol, denatured with 0.2 M and rewashed with 10 mM Tris-acetate, pH 7.6. The pyrosequencing reaction was performed for AQ mode in a total of 45 μl, including 44.82 μl of 20 mM Tris-acetate and 5 mM MgAc2, and 0.18 μl of 50 μM sequencing primer (final concentration 0.3 μM); for CpG mode, it was performed in a total of 25 μl, including 24.85 μl of 20 mM Tris-acetate, 5 mM MgAc2 and 0.15 μl of 50 μM sequencing primer (final concentration 0.3 μM). Assays were created according to the manufacturer’s instructions. The nucleotide dispensation order was outlined by Q24 2.0 software.
DNA methylation and mRNA data retrieved from The Cancer Genome Atlas (TCGA) database
The data of DNA methylation and gene expression in colorectal cancer were retrieved from TCGA Wanderer (http://maplab.imppc.org/wanderer).Citation20 The correlation between DNA methylation and CD274 mRNA (n = 218–242) were analyzed by GraphPad Prism 7 statistical software (GraphPad Software, CA, USA). Moreover, the gene expression of CD274, CD8a, CXCL9, CXCL10, IFI27 and ISG15 mRNA expression data in colorectal cancer (n = 438) were retrieved from the open-access resource named the Human Pathology Atlas as part of the Human Protein Atlas (HPA, www.proteinatlas.org/pathology).Citation21–23
The microarray gene expression before and after CCRT treatment of CRC patients from GEO database
For microarray gene expression data, we downloaded the gene expression profile of GSE93375 from the GEO database of the National Center for Biotechnology Information (NCBI). The GSE93375 dataset has gene expression data from pre-CCRT biopsies and post-CCRT surgical tissues.Citation24 We also downloaded the Series Matrix File of GSE93375 from the GEO database.
Treatment of mice with DNMTi and PD-L1 blockade
A total of 5 × 105 CT26 cells in 100 μl of 50% Matrigel were inoculated into the right leg of BALB/c mice. The treatments were initiated on day 7 after tumor inoculation: AZA (intraperitoneal injection, 0.5 mg/kg/mouse for 3 consecutive days) and 5-FU (intraperitoneal injection, 50 mg/kg/mouse, 5 times with 3-day intervals between administrations). On days 9 and 11, mice received radiotherapy (5 Gy), and on day 11, PD-L1 blockade was performed (100 μg/mouse, intraperitoneal injection, 4 times with 3-day intervals between administrations, Bio×Cell clone 10 F.9G2, NH, USA). The longest and shortest diameters (L and W, respectively) of the tumors were measured using Vernier calipers (Sata, Shanghai, China) every 3 days, and tumor volume (V) was calculated by the following formula: V = (L × W2)/2. The mice were sacrificed when the longest diameter reached 20 mm, and the survival of the tumor-bearing mice was observed and recorded every 3 days.-
Statistical analysis
All experiments were carried out at least 3 times. Briefly, statistical analysis was performed using GraphPad Prism 7 statistical software (GraphPad Software, CA, USA).Citation25 Data were analyzed using two-way ANOVA followed by Bonferroni’s post hoc test, one-way ANOVA followed by Dunnett’s post hoc test, or an unpaired t-test where appropriate. Data are expressed as mean±SEM. Student’s t-test was used to compare the differences in tumor sizes and positive cell counts between two groups. ANOVA was used for comparisons among the groups of the results involving combinations of DAC with PD-L1 blockade. Kaplan-Meier survival analysis and the log-rank test were used to compare the survival rates of the mice. p < .05 was considered to indicate a significant difference.
Results
Radiotherapy promotes tumor PD-L1 upregulation via type I IFN signaling
Type I IFNs can be autonomous secreted from irradiated cells to drive the infiltration of CD8+ immune cells for anti-tumor immunity, increase the therapeutic efficacy of RT as well as PD-L1 upregulation.Citation26–29 To examine the influence of CCRT on the levels of type I IFN signatures in CRC patients, we first compared 20 CRC patients who received CCRT from the GSE93375 dataset by gene set enrichment analysis (GSEA), and the results indicated that the type I IFN signatures was significantly increased in post-CCRT tissues compared with that in pre-CCRT biopsies ()). We then analyzed the correlation between the level of type I IFN signatures and PD-L1 mRNA. We found that type I IFN signatures such as CXCL9, CXCL10, IFI27, IFI44, ISG15 and ISG20 were positively correlated with PD-L1 mRNA in CRC patients of TCGA database ()). To prove the correlation between PD-L1 and type I IFN, we analyzed the expression of IFNβ, which is autonomous expressed in cancer cells after radiotherapy, and PD-L1 in post-CCRT surgical tissues (n = 130). We found cancer cell autonomous IFNβ and PD-L1 expression was highly expressed after CCRT (), p < .001). Tumor IFNβ expression was correlated with PD-L1 expression in post-CCRT surgical tissues (p = .0125, )), indicated that type I IFN might promote PD-L1 upregulation via autocrine and paracrine manners.
Figure 1. Radiotherapy triggered PD-L1 upregulation via autonomous IFNβ-mediated signaling pathway. (a) The gene expression data were downloaded from GSE93375 database. The type I IFN signatures were analyzed for heatmap (pre-CCRT:13 CRC patients; post-CCRT:9 CRC patients). (b) The correlation between type I IFN signatures (CXCL9, CXCL10, IFI27, IFI44, ISG15 and ISG20) and PD-L1 (CD274) were analyzed (n = 438, colon adenocarcinoma, TCGA) (c) Tumor IFNβ expression was significantly upregulated by CCRT (H-score, n = 130, p < .001). Tumor PD-L1 expression was also significantly upregulated by CCRT (H-score, p < .001). Tumor IFNβ expression was correlated with PD-L1 expression in post-CCRT surgical tissues (p = .0125). (d) SW480 and SW620 cells were treated with conditioned medium (CM) from irradiated cells (48 hrs post-irradiation) for 24 hrs and analyzed by immunoblotting. The quantification of these results is shown (n = 3). *p < .05 and **p < .01. (e) The neutralizing antibodies against IFNα and IFNβ were added into conditioned medium from irradiated SW480 and HCT116. Then SW480/HCT116 were treated with these conditioned medium for 24 hrs and analyzed qRT-PCR, respectively. (f) SW480 and CT26 cells were treated with IFNβ for 24 hrs and then examined by immunoblotting. (g) SW480 cells were treated with IFNβ for 24 hrs and then examined by qRT-PCR. The quantification of these results is shown on the right (n = 3). **p < .01 and ***p < .001. These data were obtained from three independent experiments, and the values represent the mean ± SEM. (F) CT26 cells were treated with IFNβ, STAT1 inhibitor (5 μM fludarabine) and IFNAR1 inhibitor (2 μM IFNAR1-IN) for 24 hrs and then analyzed by immunoblotting and flow cytometry
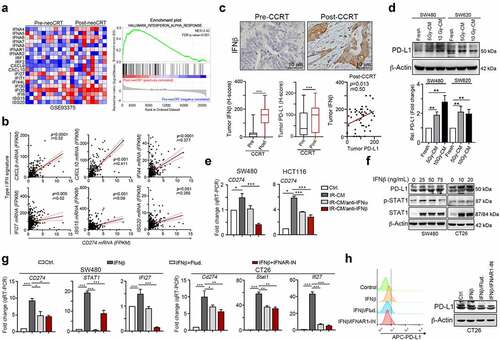
Moreover, the conditioned medium (CM) from irradiated colorectal cells profoundly upregulated tumor PD-L1 expression, suggesting that IR may provoke PD-L1 expression via autocrine or paracrine signaling such as type I IFN ()). Furthermore, by treatment with neutralizing antibodies against IFNα and IFNβ in CM, we found that both IFNα and IFNβ participated in regulating PD-L1 expression. But autonomous release of IFNβ from irradiated colorectal cancer cells mainly promoted tumor PD-L1 expression ()). Direct treatment with IFNβ on colorectal cancer cells also remarkably enhanced PD-L1 expression ()). The level of PD-L1(CD274) mRNA was dramatically decreased by IFNAR1 inhibitor IFNAR-IN-1 and STAT1 inhibitor fludarabine ()). Furthermore, the IFNβ-induced interferon-stimulated gene (ISG), IFI27, was significantly decreased after IFNAR1 blockade or STAT1 inhibition ()). Blocking IFNAR1 or inhibiting STAT1 profoundly inhibited PD-L1 expression and membrane distribution ()). Taken together, these results indicated that IFNβ-IFNAR1-STAT1 signaling was critical for radiotherapy-induced PD-L1 expression.
Methylation status on PD-L1 promoter foci was clinically associated with the response to radiotherapy and survival outcome in LARC patients
Our previous studies indicated that upregulated PD-L1 expression by CCRT regimen was associated with favorable 5-year disease-free survival, especially lymph node metastasis LARC patients.Citation30,Citation31 However, we found that ~50% patients still had low tumor PD-L1 expression after CCRT treatment, suggesting that other mechanisms may impede PD-L1 upregulation by CCRT. Recent studies indicated epigenetic regulation by methylation affected tumor PD-L1 expression and influenced the synergetic effect of immunogenic chemotherapy for anti-PD-L1 immunotherapy in vivo.Citation13,Citation15
By analyzing the DNA methylation on the PD-L1 (CD274) promoter and gene expression between normal and tumor tissues in colon carcinoma (COAD) in TCGA wanderer (http://maplab.imppc.org/wanderer/),Citation20 five methylation loci were located peripheral to the CpG-dense area of the PD-L1 (CD274) promoter (cg15837913, cg02823866, cg14305799, cg13474877 and cg19724470). Significant changes in methylation status were observed at the locus cg15837913 and cg14305799 [Fig. S1, normal tissues (n = 38) vs tumor tissues (n = 302), p < .05]. Moreover, two loci (cg15837913 and cg19724470) targeting CpGs within the upstream CpG-island located in the CD274 promoter were significantly associated with survival outcome in several malignancies, including colorectal cancer.Citation11
To further analyze whether PD-L1 promoter methylation influenced the therapeutic efficacy of CCRT in CRC patients, we extracted genomic DNA from post-CCRT surgical tissues in LARC patients and examined the extent of methylation on the CD274 (PD-L1) promoter after CCRT (). Hypermethylation was found in mucosa compared to tumor tissues at these two PD-L1 loci (cg15837913 and cg19724470, Fig. S2A). A significant difference was found in cg19724470 (p = .049, n = 57, Fig. S2A). Moreover, we found that methylation status on cg15937913 was negatively correlated with tumor PD-L1 expression (H-score, p = .0058, ) and Fig. S2B). Similarly, methylation status on cg15937913 was negatively correlated with tumor PD-L1 expression (p = .0003, ) and Fig. S2B). We then explore the association between status of mCD274 and PD-L1 mRNA in colon carcinoma (COAD) in TCGA wanderer.Citation20 The mCD274 on cg15837913 was inversely correlated with PD-L1 mRNA expression in the TCGA database (p = .0001, n = 218, r = −0.304, Pearson’s correlation, )). These results indicated that methylation on PD-L1 loci (cg15837913 and cg19724470) may suppress tumor PD-L1 expression induced by radiotherapy.
Figure 2. Methylation on PD-L1 promoter loci was clinically associated with the response to CCRT and survival outcome in LARC patients
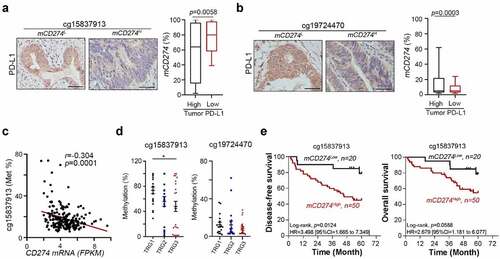
Moreover, by stratifying the patients with response degree to radiotherapy (tumor regression grade, TRG), we found that low methylation rate on cg15837913 was associated with better response to radiotherapy (), p = .0464, TRG3: near complete response; TRG2: moderate response; TRG1: minimal response). But methylation rate on cg19724470 was not associated with response to radiotherapy ()), suggesting that cg15837913 may be the critical loci for tumor PD-L1 expression and response to radiotherapy.
We then investigated the relationship between the methylation status of the PD-L1 promoter and survival outcome in LARC patients receiving CCRT. The methylation status of PD-L1 (mCD274) was dichotomized using an optimized cutoff according to previous studies (35% methylation at cg15837913 loci and 15% methylation at cg19724470).Citation10, Kaplan–Meier survival analysis and log-rank tests revealed that high mCD274 at cg15837913 loci was associated with poor 5-year disease-free survival (DFS, 78.5% vs 45.1%, log-rank p = .0124, )), and correlated with a high risk of poor 5-year DFS (Univariate Cox analysis, HR = 3.498, 95% CI = 1.665–7.349, )). Moreover, patients with high mCD274 at the cg15837913 locus had a tendency toward poor 5-year overall survival (OS, 78.5% vs 54.1%, log-rank p = .0588, )). Furthermore, high mCD274 at the cg19724470 locus was associated with poor 5-year OS (70.6% vs 41.7%, log-rank p = .0445, Fig. S2C), and correlated with a high risk of poor 5-year OS (univariate Cox analysis, HR = 3.243, 95% CI = 1.029–10.22, Fig. S2C). High mCD274 at the cg19724470 locus also had a tendency toward poor 5-year DFS (61.7% vs 41.7%, log-rank p = .2377, Fig. S2C). These results suggested that methylation of the PD-L1 promoter may reduce the therapeutic response to CCRT and associate with poor survival outcomes in locally advanced colorectal cancer.Citation11
By univariate and multivariate Cox proportional hazards regression analysis, mCD274 at cg15837913 loci served as an independent prognostic factor for 5-year DFS (HR = 4.06, 95% CI = 1.407–11.716, p = .01, ) and cg19724470 loci for 5-year OS (HR = 2.832, 95% CI = 1.093–7.337, p = .032, ) after adjustment for the risk parameters pT stage, pN stage, lymphovascular invasion (LVI) and perineural invasion (PNI). Collectively, this analysis strongly indicates that methylated PD-L1 promoter loci may serve as a potential independent prognostic factor for DFS and OS in LARC patients who received preoperative CCRT.
Table 2. Univariate and multivariate analysis of PD-L1 promoter loci (cg15837913 and cg19724470) in locally advanced colorectal cancer who received preoperative CCRT
Targeting DNA methylation promoted type I interferon response for PD-L1 upregulation by radiotherapy
To understand whether the methylation status of PD-L1 promoter influenced its accesses to IFNβ-activated transcription factor STAT1/STAT2, we first analyzed the association between tumor IFNβ and PD-L1 expression according to the status of PD-L1 promoter methylation (mCD274, )). In high tumor IFNβ group, 68.9% patients with high methylation on cg15837913 loci (mCD274H on cg15837913) and 31.1% patients with mCD274L. We found that 65.0% patients with high tumor PD-L1 in IFNβH/mCD274H group. But 100.0% patients with high tumor PD-L1 in IFNβH/mCD274L group, suggesting that methylation status on cg15837913 may influence its response to RT-mediated IFNβ signaling.
Figure 3. Methylation on cg15837913 constrains PD-L1 upregulation in response to radiotherapy (a) The status of tumor IFNβ within tumor microenvironment in post-CCRT surgical tissues. In high tumor IFNβ group, there was 31.1% patients with mCD274L (cg15837913 loci) after CCRT treatment. Moreover, all mCD274L patients with high tumor PD-L1 expression. (b) SW480 cells were treated with DNMT inhibitor (DAC, 1–2 μM) for 48 hrs. The methylation status on two loci (cg15837913 and cg19724470) were analyzed by pyrosequencing (n = 3). (c) SW480 and CT26 cells were treated with DNMT inhibitor (2 μM DAC) and IR (2 Gy) for 48 hrs and analyzed by immunoblotting. The quantification of these results is shown (n = 3). *p < .05, **p < .01 and ***p < .001. (d) SW480 and CT26 cells were treated with DNMTi (2 μM DAC) and IR (2 Gy) for 48 hrs and analyzed by flow cytometry. The quantification of these results is shown (n = 3). *p < .05, **p < .01 and ***p < .001
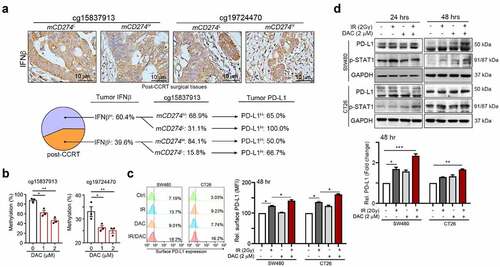
To demonstrate the impact of epigenetic inhibition on the two loci of PD-L1 promoter, we treated with DAC which is the FDA-approved DNA methyltransferase (DNMT) inhibitor with colorectal cancer cells. We found that the status of mCD274 on these two loci was decreased after treatment with DAC ()). Moreover, after administration with DAC, we found that DNMTi significantly induced total tumor PD-L1 expression in a dose-dependent and time-dependent manner (Fig. S3A), and surface PD-L1 was also gradually increased following DAC treatment (Fig. S3B). The expression of tumor PD-L1 was also induced by irradiation (IR) in a dose-dependent and time-dependent manner (Fig. S3C and S3D). These results suggest that DNMT1 may epigenetically regulate radiotherapy-induced tumor PD-L1 upregulation via influencing the access of IFNβ-activated transcription factor STAT1/STAT2. Indeed, the PD-L1 level was profoundly increased by combinational treatment with IR and DAC in SW480 and CT26 cells after 24 and 48 hrs ()), and the surface PD-L1 was also synergistically increased by IR and DAC treatment after 48 hrs, suggesting that demethylation agents may sensitize cancer cells to radiotherapy for PD-L1 induction ()). Moreover, PD-L1 protein expression was slightly increased in SW480shDNMT1 cells compared to SW480shNC cells (Fig. S4A). However, PD-L1 expression was significantly upregulated in SW480shDNMT1 cells in response to a low dose of IR (Fig. S4A). The level of surface PD-L1 was also increased (Fig. S4B). In contrast, transient transfection of DNMT1 dramatically reduced PD-L1 expression and its membrane distribution, even after exposure to a high dose of IR (Fig. S4C and S4D). Knockdown of DNMT1 directly increased the upregulation by IFNβ (Fig. S4E). Overexpression of DNMT1 significantly reduced IFNβ-mediated PD-L1 expression (Fig. S4F). Taken together, these results suggested that epigenetic modification by DNMT1 may decrease the accessibility to IR-mediated IFNβ signaling to influence the PD-L1 expression by in cancer cells.
Epigenetic inhibition upregulates tumor immunogenicity to potentiate IR-induced anti-tumor immunity
Epigenetic therapy by DNMTi was reported to activate type I IFN signaling, increased MHC class I expression, recruited CTL infiltration, reduced immunosuppression and increased the therapeutic efficacy of immunotherapy.Citation32,Citation33 Therefore, we assume IR and DNMTi synergetic increased type I IFN signaling for PD-L1 upregulation. We found that both IR and DNMTi similarly triggered an interferon response, including the type I interferon pathway (IFNβ1, IRF7, IRF9 and STAT1), the interferon-stimulated gene IFI27, antigen processing and presentation genes TAP-1 and HLA-C, and PD-L1 in CT26 and SW480 cell lines ()). Moreover, the level of secreted IFNβ was significantly detected 2 days after DNMTi and IR treatment of SW480 colorectal cancer cells ()).
Figure 4. Inhibition of DNMT1 remarkably inhibited IR-induced PD-L1 upregulation and immunogenicity via IFNβ/IFNAR1/STAT1 signaling (a) CT26 cells were treated with DAC (5 μg/mL) and IR (2 Gy) for 48 hrs, and gene expression was examined by qRT-PCR. The quantification of these results is shown (n = 3). **p < .01 and ***p < .001. (b) SW480 cells were treated with DAC (5 μg/mL) and IR (2 Gy) for 48 hrs, and gene expression was examined by qRT-PCR. The quantification of these results is shown (n = 3). **p < .01 and ***p < .001. (c) The supernatant of SW480 cells after treatment with DAC (5 μg/mL) and IR (2 Gy) for 48 hrs, and the level of IFNβ was evaluated by ELISA and immunoblotting (n = 3). *p < .05 and **p < .01. (d) SW480 and CT26 cells were treated with DAC (2 μM DAC) and IR (2 Gy) for 24 hrs, treated with STAT1 inhibitor (10 μM) and IFNAR1 inhibitor (10 μM) for 24 hrs. The conditioned medium (CM) was added into fresh SW480 and CT26 cells for 24 hrs, and then analyzed by flow cytometry. (e) SW480 and CT26 cells were treated with DAC (2 μM DAC) and IR (2 Gy) for 24 hrs, treated with the STAT1 inhibitor fludarabine (10 μM) and the IFNAR1 inhibitor IFNAR1-IN (10 μM) for 24 hrs. The conditioned medium (CM) was added into fresh SW480 and CT26 cells for 24 hrs, and then analyzed by immunoblotting. These data were obtained from three independent experiments, and the values represent the mean ± SEM
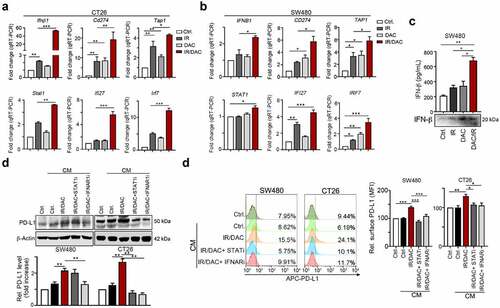
We then examined whether blockade of the IFNβ receptor IFNAR1 or inhibition of STAT1 influenced the impact of conditioned medium (CM) on PD-L1 expression. We found that the expression of total PD-L1 was decreased after inhibition of IFNAR1 and STAT1 ()). Moreover, both the IFNAR antagonist and STAT1 inhibitor fludarabine drastically reduced surface PD-L1 levels in SW480 and CT26 cells ()). Taken together, these results indicated that cancer cell autonomous IFNβ release by RT and DNMTi promotes PD-L1 expression via IFNAR1-STAT1 signaling pathway.
DNMTi dramatically triggered radiotherapy-induced immune signatures for antitumor immunity in vivo
To further evaluate whether the DNMTi prompted the therapeutic efficacy of chemoradiotherapy, we inoculated BALB/c mice with CT26 mouse colon carcinoma cells and treated the mice with the FDA-approved small-molecule DNMT inhibitor azacitidine (5-Azacytidine, AZA, 0.5 mg/kg by i.p. injection), IR (5 Gy, )) and compared with 5-FU-based chemoradiotherapy [CCRT, 5-FU (5 mg/kg by i.p. injection) and IR (5 Gy)]. As shown in ), AZA alone decreased tumor growth (Dunnett’s test, p = .046), and CCRT (5-FU/IR) significantly decreased tumor growth (Dunnett’s test, p = .01). However, a profound effect was observed in the CCRT/AZA group (Dunnett’s test, p < .01, )). The resected tumor weight was dramatically decreased in CCRT/AZA group ()). The immunoblotting results showed that the tumor PD-L1 protein level was highly increased, and the phosphorylation of STAT1 was significantly increased in the tumors resected from the CCRT/AZA group ()), suggesting that interferon signaling is required for the induction of PD-L1. Moreover, the infiltration of immune cells was significantly increased in the CCRT/AZA group ()). These results show that DNMTi prompted the therapeutic efficacy of radiotherapy by increasing immunogenicity and inducing the immune response in vivo.
Figure 5. AZA synergistically increased the therapeutic response to radiotherapy in vivo. (a) Tumor-bearing BALB/c mice (n = 6) were treated with AZA (0.5 mg/kg, i.p. injection), CCRT [5-FU (5 mg/kg, i.p. injection) and IR (5 Gy)] at the indicated times shown on the timeline. Tumor volume was measured and calculated every 3 days. The quantification of these results is shown (n = 6). **p < .01 and ***p < .001. (b) Resected tumors from representative mice were collected for lysis and analyzed by immunoblotting. The quantification of these results is shown (n = 3). *p < .05, **p < .01 and ***p < .01. (c) The infiltration of CD3+ and CD8+ immune cells in resected tumors from representative mice were analyzed by immunohistochemistry. Bar: 50 μm. (d) The quantification of these results is shown (n = 5). *p < .05, **p < .01 and *** p < .001
![Figure 5. AZA synergistically increased the therapeutic response to radiotherapy in vivo. (a) Tumor-bearing BALB/c mice (n = 6) were treated with AZA (0.5 mg/kg, i.p. injection), CCRT [5-FU (5 mg/kg, i.p. injection) and IR (5 Gy)] at the indicated times shown on the timeline. Tumor volume was measured and calculated every 3 days. The quantification of these results is shown (n = 6). **p < .01 and ***p < .001. (b) Resected tumors from representative mice were collected for lysis and analyzed by immunoblotting. The quantification of these results is shown (n = 3). *p < .05, **p < .01 and ***p < .01. (c) The infiltration of CD3+ and CD8+ immune cells in resected tumors from representative mice were analyzed by immunohistochemistry. Bar: 50 μm. (d) The quantification of these results is shown (n = 5). *p < .05, **p < .01 and *** p < .001](/cms/asset/16103803-9211-4888-a100-124e3d290e88/koni_a_1989790_f0005_oc.jpg)
To confirm the importance of interferon signatures for antitumor immunity induced by DNMTi and IR, we conducted an RNA-seq analysis of the resected tumors from the groups ()). Compared to the untreated PBS group, the AZA alone group slightly increased IFNβ-related genes, but the CCRT group and CCRT/AZA group exhibited similar upregulated gene expression profiles, especially IFNβ-related genes, including IFI27, IRF9, STAT1, CXCL10, STAT2, IRF7, IRF1, CD274, and TAP1 ()). The gene ontology results also showed that CCRT and CCRT/AZA group had profoundly activation of type I IFN signaling ()).
Figure 6. DNMTi enhanced the therapeutic efficacy of concurrent chemoradiotherapy and anti-PD-L1 immunotherapy in CRC in vivo.(a) Resected tumors from representative mice were analyzed by RNA-seq (n = 2). The IFNβ-related signatures are shown (n = 2 per group). (b) The most enriched upregulated genes list (GO analysis) in CCRT and PBS group are shown (CCRT vs PBS and CCRT/ATZ vs PBS). (c) The result of GSEA (Gene Set Enrichment analysis) indicated that type I IFN signaling was activated in CCRT group compared to PBS group, and in CCRT/AZA group compared to PBS group. (d) The mRNA level of type I IFN signatures in the resected tumors were analyzed by qRT-PCR (n = 3–4). *p < .05, **p < .01 and ***p < .001. (e) Tumor-bearing BALB/c mice (n = 6) were treated with AZA (0.5 mg/kg, i.p. injection), CCRT [IR (5 Gy) and 5-FU (5 mg/kg, i.p. injection)] and anti-PD-L1 blockade (100 μg/mouse, i.p. injection) at the indicated times shown on the timeline. Tumor volume was measured and calculated every 3 days. The relative tumor volume is shown (n = 6). **p < .01 and ***p < .001. Mouse survival was assessed with Kaplan-Meier survival curves (n = 6)
![Figure 6. DNMTi enhanced the therapeutic efficacy of concurrent chemoradiotherapy and anti-PD-L1 immunotherapy in CRC in vivo.(a) Resected tumors from representative mice were analyzed by RNA-seq (n = 2). The IFNβ-related signatures are shown (n = 2 per group). (b) The most enriched upregulated genes list (GO analysis) in CCRT and PBS group are shown (CCRT vs PBS and CCRT/ATZ vs PBS). (c) The result of GSEA (Gene Set Enrichment analysis) indicated that type I IFN signaling was activated in CCRT group compared to PBS group, and in CCRT/AZA group compared to PBS group. (d) The mRNA level of type I IFN signatures in the resected tumors were analyzed by qRT-PCR (n = 3–4). *p < .05, **p < .01 and ***p < .001. (e) Tumor-bearing BALB/c mice (n = 6) were treated with AZA (0.5 mg/kg, i.p. injection), CCRT [IR (5 Gy) and 5-FU (5 mg/kg, i.p. injection)] and anti-PD-L1 blockade (100 μg/mouse, i.p. injection) at the indicated times shown on the timeline. Tumor volume was measured and calculated every 3 days. The relative tumor volume is shown (n = 6). **p < .01 and ***p < .001. Mouse survival was assessed with Kaplan-Meier survival curves (n = 6)](/cms/asset/7108cb37-fd1b-47ad-9c48-2e787c067519/koni_a_1989790_f0006_oc.jpg)
To fully capitalize the gene signatures of the whole transcriptome, we performed unbiased GSEA (gene set enrichment analysis) using whole tumor transcriptomes. These pathways and genes indicate a significant activation of IFNβ in the CCRT and CCRT/AZA group ()). These gene profiles were validated by qRT-PCR ()). Taken together, these results indicated that DNMTi clearly remodulated the epigenetic control of tumor immunogenicity within the TME, which promoted the therapeutic effect of radiotherapy via antitumor immunity.
The therapeutic efficacy of ICB in colorectal cancer was currently limited because microsatellite instability (MSI)-CRC patients was minority (7–15%).Citation15 Since DNMTi could reinvigorate tumor immunogenicity to recruit immune cell infiltration and induce interferon signatures to upregulate PD-L1 expression, which are two biomarkers for ICBs, we evaluate whether this combination can increase the therapeutic efficacy of ICBs in immunotherapy-refractory microsatellite stability (MSS) in vivo. We inoculated the CT26 cells, which is the murine MSS-CRC cells, and assessed the therapeutic response to anti-PD-L1 antibodies (100 μg/mouse by i.p. injection) with the addition of low-dose AZA (0.5 mg/kg by i.p. injection), CCRT [IR (5 Gy) and 5-FU (5 mg/ kg by i.p. injection), )]. The results showed that the therapeutic response to anti-PD-L1 antibodies alone is poor (relative tumor volume: PBS/αPD-L1 vs PBS/αIgG = 82.8 ± 11.4% vs 100.0 ± 12.3%, p = .88). But CCRT treated together with anti-PD-L1 antibodies had a remarkable effect on tumor growth (relative tumor volume: CCRT/αPD-L1 vs PBS/αPD-L1 = 25.4 ± 8.7% vs 82.8 ± 11.4%, p = .0042, )) and improved the survival rate compared to PBS/anti-PD-L1 antibodies (), median survival in days: 42.5 vs 29 days). AZA treatment together with anti-PD-L1 antibodies also had a synergistic effect on tumor growth, and the relative tumor volume was largely decreased (relative tumor volume: DNMTi/αPD-L1 vs PBS/αPD-L1 = 42.14 ± 7.59% vs 82.8 ± 11.4%, p = .0758, )), but the survival time was not extended (), median survival in days: 29.5 vs 29 days). Notably, combinational administration with CCRT and AZA strongly promoted the therapeutic efficacy of anti-PD-L1 antibodies (relative tumor volume: CCRT/DNMTi/αPD-L1 vs PBS/αPD-L1 = 12.09 ± 3.98% vs 82.8 ± 11.4%, p = .001, )), and the survival rate was improved compared to PBS with anti-PD-L1 antibodies (), median survival in days: 61 vs 29 days, respectively, log-rank test, p < .0001). Therefore, our results suggested that DNMTi combined with CCRT can directly provoke tumor PD-L1 expression and T cell infiltration, which is more feasible for anti-PD-L1 immunotherapy in CRC, especially for immunotherapy-refractory MSS-CRC.
Discussion
In this study, we found that methylation on the loci (cg15837913 and cg19724470) within the PD-L1 promoter was clinically associated with the response to CCRT and survival outcome in LARC patients who received preoperative CCRT. Moreover, methylation status on these two loci was negatively correlated with tumor PD-L1 expression. CCRT triggered type I IFN release from irradiated cells to promote tumor PD-L1 expression. But the methylation status on cg15837913 may significantly influence its response to tumor IFNβ. Pharmacological inhibition of DNMT1 activity upregulated the PD-L1 level via amplifying IFNβ response as well as cancer immunogenicity within the TME in vivo. Combining DNMT inhibitor together with CCRT remarkably enhanced the therapeutic response to anti-PD-L1 immunotherapy in CRC, especially in MSS-CRC. Therefore, our results showed that DNMTi profoundly enhances PD-L1 expression by IFNβ as well as reactivates antitumor immunity for targeting by ICBs.
Targeting the immune checkpoint PD-1/PD-L1 signaling has become a promising therapy with durable benefits and minimal toxicity in several solid tumors.Citation33,Citation34 However, objective responses rate (ORR) are observed in a minority of patients because of low cancer immunogenicity, such as CRC.Citation35 Therefore, predictive biomarkers to stratify patients and therapeutic strategies to reinvigorate anticancer immunity for immunotherapy are urgently needed. Local radiotherapy has been demonstrated to trigger antigen-specific adaptive immunity by inducing immunogenic cell death (ICD) that effectively exposes tumor antigens for the anticancer immune response, which is called “in situ” vaccination. Radiotherapy can remarkably trigger cytotoxic T cell infiltration to eradicate cancer cells and improve the therapeutic efficiency of PD-1/PD-L1 immunotherapy.Citation36 In our previous studies, cytotoxic T lymphocyte recruitment and tumor PD-L1 level expression were significantly provoked within the TME by comparing pre-CCRT biopsies and post-CCRT surgical tissues in LARC patients.Citation30 The presence of heavy lymphocyte infiltration and high tumor PD-L1 were significantly associated with improved survival outcome in LARC patients, suggesting that reactivation of cancer immunogenicity by CCRT within the TME induces PD-L1 expression as a predictive biomarker for stratifying LARC patients to eradicate residual cancer cells.Citation7,Citation37 Notably, we found that tumor PD-L1 did not upregulated by CCRT in 40–50% of LARC patients, even in the presence of heavy CD8+ lymphocyte infiltration, suggesting that other mechanisms may inhibit PD-L1 upregulation by IFNγ from CD8+ T lymphocytes, such as epigenetic modification.
Two targeting loci (cg15837913 and cg19724470) within the PD-L1 promoter regions were associated with survival outcome in several malignancies, such as prostate cancer, colorectal cancer, and lymphoma.Citation11–12,Citation38 Consistent with previous studies, our results show that patients with hypomethylated PD-L1 promoter regions have better therapeutic efficacy and improved survival outcomes after CCRT therapy. Moreover, the addition of a demethylation agent directly promotes PD-L1 expression and sensitizes cancer cells to radiotherapy-induced IFNβ for PD-L1 upregulation in vitro and in vivo. IFNβ can directly upregulate PD-L1 expression through IFNAR1-STAT1 signaling, which is consistent with the findings of previous studies.Citation39 Furthermore, our results showed that expression of cancer cell autonomous IFNβ were significantly correlated with tumor PD-L1 expression within tumor microenvironment after CCRT treatment. By neutralizing with individual anti-IFNα and anti-IFNβ antibodies, we found that intrinsic IFNβ may mainly participate in regulating PD-L1 expression, which is consistent with previous studies that IFNβ had highest affinity to IFNAR1/2 compared to all IFNα subtypes.Citation40 Several studies have demonstrated that epigenetic therapy activates type I interferon signaling to reactivate anticancer immunity in preclinical and clinical studies.Citation27,Citation33,Citation41 Leu et al. indicated that treatment with a novel hypomethylating agent, guadecitabine, upregulated MHC-I expression for antigen presentation and recruited CD8+ T cells into the microenvironment in vivo and in breast cancer patients.Citation27 Moreover, DNMTi treatment sensitizes cells to anti-CTLA4 immunotherapy through the type I IFN response in a preclinical melanoma model.Citation32 These studies indicated that DNMTi remodels the tumor microenvironment by inducing tumor immunogenicity, as well as the IFNβ immune response, thereby enhancing the therapeutic efficacy of immunotherapy.Citation42 Supporting these findings, Liang et al. recently demonstrated that exogenous type I IFN creates feedforward antitumor responses to overcome checkpoint blockade resistance in colorectal cancer.Citation43 Taken together, the effects of DNMTi promote radiotherapy-induced antitumor immunity by not only upregulating cancer immunogenicity but also inducing the IFNβ immune response to enhance anti-PD-L1 immunotherapy in colorectal cancer. Molecular studies have demonstrated that this effect may contribute to direct promoter demethylation of the PD-L1 promoter, as well as stimulation of cancer immunity. However, we cannot exclude other regulatory mechanisms on PD-L1 expression by radiotherapy, such as DNA damage response and acetylation.Citation44 Sato et al. indicated DNA double-strand break (DSB) may trigger ATM/ATR/Chk1 kinases to promote PD-L1 upregulation.Citation44 Moreover, inhibition of acetylation by HDAC inhibitor also enhances anti-PD-L1-mediated tumor suppression.Citation45 Therefore, the detailed mechanisms on PD-L1 expression by radiotherapy to increase the clinical response to ICB are required in future studies.
In summary, DNMTi potentiated T cell recruitment, enhanced antitumor immunity, and exerted combinatorial activity with radiotherapy and PD-L1 immunotherapy in colorectal cancer. Given these results, combining DNMTi with radiotherapy and anti-PD-L1 immunotherapy may be a viable approach to converting immunotherapy-refractory tumors, such as MSS-CRC tumors, to immunotherapy-responsive tumors and should be tested in future clinical trials.
Authors’ contributions
Kevin Chih-Yang Huang, Shu-Fen Chiang, Pei-Chen Yang, Ching-Han Hu and Hsin-Yu Chang conducted and performed the experiments; William Tzu-Liang Chen, Tao-Wei Ke and Tsung-Wei Chen enrolled colon cancer patients and performed the IHC evaluation; Kevin Chih-Yang Huang performed the statistical analysis; Shu-Fen Chiang, Ji-An Liang and K. S. Clifford Chao supervised this study; and Kevin Chih-Yang Huang, Shu-Fen Chiang and K. S. Clifford Chao analyzed the data and wrote the manuscript.
Ethical approval
This study was reviewed and approved by the Internal Review Board (IRB) of China Medical University Hospital [Protocol number: CMUH107-REC2-008]. The study was carried out in accordance with the committee’s approved guidelines.
Informed consent
Informed consent was obtained from all participants in the study.
Data sharing statement
The original dataset is available on request from the corresponding author.
Supplemental Material
Download MS Word (1.4 MB)Acknowledgments
We thank the National RNAi Core Facility at Academia Sinica in Taiwan for providing shRNA reagents and related services. This study was partially based on data from the China Medical University Hospital cancer registry. This study was supported in part by China Medical University (CMU109-MF-97), China Medical University Hospital (DMR-CELL-2103 and DMR-CELL-2102), Ministry of Science and Technology (MOST110-2628-B-039-005, MOST108-2320-B-039-045, MOST110-2314-B-039-032, Taiwan), and the Health and welfare surcharge on tobacco products, China Medical University Hospital Cancer Research Center of Excellence (MOHW110-TDU-B-212-144024). Experiments and data analysis were performed in part through the use of the Medical Research Core Facilities Center, Office of Research and Development at China Medical University, Taichung, Taiwan, R.O.C.
Disclosure statement
No potential conflict of interest was reported by the author(s).
Supplementary material
Supplemental data for this article can be accessed on the publisher’s website
Additional information
Funding
References
- Adlard JW, Richman SD, Seymour MT, Quirke P. Prediction of the response of colorectal cancer to systemic therapy. Lancet Oncol. 2002;3(2):75–14. doi:10.1016/S1470-2045(02)00648-4.
- Goldberg RM, Sargent DJ, Morton RF, Fuchs CS, Ramanathan RK, Williamson SK, Findlay BP, Pitot HC, Alberts SR. A randomized controlled trial of fluorouracil plus leucovorin, irinotecan, and oxaliplatin combinations in patients with previously untreated metastatic colorectal cancer. J Clin Oncol. 2004;22(1):23–30. doi:10.1200/JCO.2004.09.046.
- Sistigu A, Yamazaki T, Vacchelli E, Chaba K, Enot DP, Adam J, Vitale I, Goubar A, Baracco EE, Remédios C, et al. Cancer cell-autonomous contribution of type I interferon signaling to the efficacy of chemotherapy. Nat Med. 2014;20(11):1301–1309. doi:10.1038/nm.3708.
- Deng L, Liang H, Xu M, Yang X, Burnette B, Arina A, Li X-D, Mauceri H, Beckett M, Darga T, et al. STING-Dependent Cytosolic DNA Sensing Promotes Radiation-Induced Type I Interferon-Dependent Antitumor Immunity in Immunogenic Tumors. Immunity. 2014;41(5):843–852. doi:10.1016/j.immuni.2014.10.019.
- Su T, Zhang Y, Valerie K, Wang XY, Lin S, Zhu G. STING activation in cancer immunotherapy. Theranostics. 2019;9(25):7759–7771. doi:10.7150/thno.37574.
- Vanpouille-Box C, Formenti SC, Demaria S. Toward Precision Radiotherapy for Use with Immune Checkpoint Blockers. Clin Cancer Res. 2018;24(2):259–265. doi:10.1158/1078-0432.CCR-16-0037.
- Huang CY, Chiang S-F, Ke T-W, Chen T-W, You Y-S, Chen WTL, Chao KSC. Clinical significance of programmed death 1 ligand-1 (CD274/PD-L1) and intra-tumoral CD8+ T-cell infiltration in stage II-III colorectal cancer. Sci Rep. 2018;8(1):15658. doi:10.1038/s41598-018-33927-5.
- Pardoll DM. The blockade of immune checkpoints in cancer immunotherapy. Nat Rev Cancer. 2012;12(4):252–264. doi:10.1038/nrc3239.
- Tumeh PC, Harview CL, Yearley JH, Shintaku IP, Taylor EJM, Robert L, Chmielowski B, Spasic M, Henry G, Ciobanu V, et al. PD-1 blockade induces responses by inhibiting adaptive immune resistance. Nature. 2014;515(7528):568–571. doi:10.1038/nature13954.
- Gevensleben H, Holmes EE, Goltz D, Dietrich J, Sailer V, Ellinger J, Dietrich D, Kristiansen G. PD-L1 promoter methylation is a prognostic biomarker for biochemical recurrence-free survival in prostate cancer patients following radical prostatectomy. Oncotarget. 2016:7(48):79943-79955. doi: 10.18632/oncotarget.13161.
- Goltz D, Gevensleben H, Dietrich J, Dietrich D. PD-L1 (CD274) promoter methylation predicts survival in colorectal cancer patients. Oncoimmunology. 2017;6(1):e1257454. doi:10.1080/2162402X.2016.1257454.
- Goltz D, Gevensleben H, Grünen S, Dietrich J, Kristiansen G, Landsberg J, Dietrich D. Leukemia. 2017;31(3):738–743. doi:10.1038/leu.2016.328
- Emran AA, Chatterjee A, Rodger EJ, Tiffen JC, Gallagher SJ, Eccles MR, Hersey P. Targeting DNA Methylation and EZH2 Activity to Overcome Melanoma Resistance to Immunotherapy. Trends Immunol. 2019;40(4):328–344. doi:10.1016/j.it.2019.02.004.
- Perrier A, Didelot A, Laurent-Puig P, Blons H, Garinet S. Epigenetic Mechanisms of Resistance to Immune Checkpoint Inhibitors. Biomolecules. 2020;10(7):1061. doi: 10.3390/biom10071061.
- Huang KC, Chiang SF, Chen WT, Chen TW, Hu CH, Yang PC, Ke TW, Chao KSC. Decitabine Augments Chemotherapy-Induced PD-L1 Upregulation for PD-L1 Blockade in Colorectal Cancer. Cancers (Basel). 2020;12(2):462. doi: 10.3390/cancers12020462.
- Lin TY, Fan C-W, Maa M-C, Leu T-H. Lipopolysaccharide-promoted proliferation of Caco-2 cells is mediated by c-Src induction and ERK activation. Biomedicine (Taipei). 2015;5(1):5. doi:10.7603/s40681-015-0005-x.
- Huang CY, Chiang S-F, Chen WTL, Ke T-W, Chen T-W, You Y-S, Lin C-Y, Chao KSC, Huang CY. HMGB1 promotes ERK-mediated mitochondrial Drp1 phosphorylation for chemoresistance through RAGE in colorectal cancer. Cell Death Dis. 2018;9(10):1004. doi:10.1038/s41419-018-1019-6.
- Wan Y, Jin S, Ma C, Wang Z, Fang Q, Jiang R. RNA-Seq reveals seven promising candidate genes affecting the proportion of thick egg albumen in layer-type chickens. Sci Rep. 2017;7(1):18083. doi:10.1038/s41598-017-18389-5.
- Zhou R, Qiu L, Liu X, Ling L, Li N, Zhou K, Sun J, Yan J, Tan C, Huang X, et al. RASSF6 downregulation promotes the epithelial-mesenchymal transition and predicts poor prognosis in colorectal cancer. Oncotarget. 2017;8(33):55162–55175. doi:10.18632/oncotarget.19181.
- Diez-Villanueva A, Mallona I, Peinado MA. Wanderer, an interactive viewer to explore DNA methylation and gene expression data in human cancer. Epigenetics Chromatin. 2015;8(1):22. doi:10.1186/s13072-015-0014-8.
- Uhlen M, Zhang C, Lee S, Sjostedt E, Fagerberg L, Bidkhori G, Benfeitas R, Arif M, Liu Z, Edfors F, et al. A pathology atlas of the human cancer transcriptome. Science. 2017;357(6352):eaan2507. doi:10.1126/science.aan2507.
- Wang G, Kang X, Chen KS, Jehng T, Jones L, Chen J, Huang XF, Chen SY. An engineered oncolytic virus expressing PD-L1 inhibitors activates tumor neoantigen-specific T cell responses. Nat Commun. 2020;11(1):1395. doi:10.1038/s41467-020-15229-5.
- Uhlen M, Fagerberg L, Hallstrom BM, Lindskog C, Oksvold P, Mardinoglu A, Sivertsson A, Kampf C, Sjostedt E, Asplund A. et al. Proteomics. Tissue-based map of the human proteome. Science. 2015;347(6220):1260419. doi: 10.1126/science.1260419.
- Goncalves-Ribeiro S, Sanz-Pamplona R, Vidal A, Sanjuan X, Guillen Díaz-Maroto N, Soriano A, Guardiola J, Albert N, Martínez-Villacampa M, López I, et al. Prediction of pathological response to neoadjuvant treatment in rectal cancer with a two-protein immunohistochemical score derived from stromal gene-profiling. Ann Oncol. 2017;28(9):2160–2168. doi:10.1093/annonc/mdx293.
- Wang X, Sheu JJC, Lai M-T, Yin-Yi Chang C, Sheng X, Wei L, Gao Y, Wang X, Liu N, Xie W, et al. RSF-1 overexpression determines cancer progression and drug resistance in cervical cancer. Biomedicine (Taipei). 2018;8(1):4. doi:10.1051/bmdcn/2018080104.
- Budhwani M, Mazzieri R, Dolcetti R. Plasticity of Type I Interferon-Mediated Responses in Cancer Therapy: from Anti-tumor Immunity to Resistance. Front Oncol. 2018;8:322. doi:10.3389/fonc.2018.00322.
- Luo N, Nixon MJ, Gonzalez-Ericsson PI, Sanchez V, Opalenik SR, Li H, Zahnow CA, Nickels ML, Liu F, Tantawy MN. et al. DNA methyltransferase inhibition upregulates MHC-I to potentiate cytotoxic T lymphocyte responses in breast cancer. Nat Commun. 2018;9(1):248. doi: 10.1038/s41467-017-02630-w.
- Burnette BC, Liang H, Lee Y, Chlewicki L, Khodarev NN, Weichselbaum RR, Fu Y-X, Auh SL. The efficacy of radiotherapy relies upon induction of type i interferon-dependent innate and adaptive immunity. Cancer Res. 2011;71(7):2488–2496. doi:10.1158/0008-5472.CAN-10-2820.
- Bald T, Landsberg J, Lopez-Ramos D, Renn M, Glodde N, Jansen P, Gaffal E, Steitz J, Tolba R, Kalinke U, et al. Immune cell-poor melanomas benefit from PD-1 blockade after targeted type I IFN activation. Cancer Discov. 2014;4(6):674–687. doi:10.1158/2159-8290.CD-13-0458.
- Chen TW, Huang KCY, Chiang S-F, Chen WTL, Ke T-W, Chao KSC. Prognostic relevance of programmed cell death-ligand 1 expression and CD8+ TILs in rectal cancer patients before and after neoadjuvant chemoradiotherapy. J Cancer Res Clin Oncol. 2019;145(4):1043–1053. doi:10.1007/s00432-019-02874-7.
- Chiang SF, Huang C-Y, Ke T-W, Chen T-W, Lan Y-C, You Y-S, Chen WTL, Chao KSC. Upregulation of tumor PD-L1 by neoadjuvant chemoradiotherapy (neoCRT) confers improved survival in patients with lymph node metastasis of locally advanced rectal cancers. Cancer Immunol Immunother. 2019;68(2):283–296. doi:10.1007/s00262-018-2275-0.
- Chiappinelli KB, Strissel P, Desrichard A, Li H, Henke C, Akman B, Hein A, Rote N, Cope L, Snyder A, et al. Inhibiting DNA Methylation Causes an Interferon Response in Cancer via dsRNA Including Endogenous Retroviruses. Cell. 2015;162(5):974–986. doi:10.1016/j.cell.2015.07.011.
- Stone ML, Chiappinelli KB, Li H, Murphy LM, Travers ME, Topper MJ, Mathios D, Lim M, Shih I-M, Wang T-L, et al. Epigenetic therapy activates type I interferon signaling in murine ovarian cancer to reduce immunosuppression and tumor burden. Proc Natl Acad Sci U S A. 2017;114(51) E10981-E10990 doi: 10.1073/pnas.1712514114.
- Xu-Monette ZY, Zhang M, Li J, Young KH. PD-1/PD-L1 Blockade: Have We Found the Key to Unleash the Antitumor Immune Response? Front Immunol. 2017;8:1597. doi: 10.3389/fimmu.2017.01597.
- Sharma P, Hu-Lieskovan S, Wargo JA, Ribas A. Primary, Adaptive, and Acquired Resistance to Cancer Immunotherapy. Cell. 2017;168(4):707–723. doi:10.1016/j.cell.2017.01.017
- Hwang WL, Niemierko A, Hwang KL, Hubbeling H, Schapira E, Gainor JF, Keane FK. Clinical Outcomes in Patients With Metastatic Lung Cancer Treated With PD-1/PD-L1 Inhibitors and Thoracic Radiotherapy. JAMA Oncol. 2018;4(2):253–255. doi:10.1001/jamaoncol.2017.3808
- Droeser RA, Hirt C, Viehl CT, Frey DM, Nebiker C, Huber X, Zlobec I, Eppenberger-Castori S, Tzankov A, Rosso R, et al. Clinical impact of programmed cell death ligand 1 expression in colorectal cancer. Eur J Cancer. 2013;49(9):2233–2242. doi:10.1016/j.ejca.2013.02.015.
- Micevic G, Thakral D, McGeary M, Bosenberg MW. PD-L1 methylation regulates PD-L1 expression and is associated with melanoma survival. Pigment Cell Melanoma Res. 2019;32(3):435–440. doi:10.1111/pcmr.12745.
- Schreiner B, Mitsdoerffer M, Kieseier BC, Chen L, Hartung H-P, Weller M, Wiendl H. Interferon-beta enhances monocyte and dendritic cell expression of B7-H1 (PD-L1), a strong inhibitor of autologous T-cell activation: relevance for the immune modulatory effect in multiple sclerosis. J Neuroimmunol. 2004;155(1–2):172–182. doi:10.1016/j.jneuroim.2004.06.013.
- Piehler J, Thomas C, Garcia KC, Schreiber G. Structural and dynamic determinants of type I interferon receptor assembly and their functional interpretation. Immunol Rev. 2012;250(1):317–334. doi:10.1111/imr.12001.
- McGough JM, Yang D, Huang S, Georgi D, Hewitt SM, Röcken C, Tänzer M, Ebert MP, Liu K. DNA methylation represses IFN-gamma-induced and signal transducer and activator of transcription 1-mediated IFN regulatory factor 8 activation in colon carcinoma cells. Mol Cancer Res. 2008;6(12):1841–1851.
- Yu G, Wu Y, Wang W, Xu J, Lv X, Cao X, Wan T. Low-dose decitabine enhances the effect of PD-1 blockade in colorectal cancer with microsatellite stability by re-modulating the tumor microenvironment. Cell Mol Immunol. 2019;16(4):401–409. doi:10.1038/s41423-018-0026-y.
- Liang Y, Tang H, Guo J, Qiu X, Yang Z, Ren Z, Sun Z, Bian Y, Xu L, Xu H, Shen J, Han Y, Dong H, Peng H, Fu YX. Targeting IFNalpha to tumor by anti-PD-L1 creates feedforward antitumor responses to overcome checkpoint blockade resistance. Nat Commun. 2018;9(1):4586. doi:10.1038/s41467-018-06890-y
- Sato H, Niimi A, Yasuhara T, Permata TBM, Hagiwara Y, Isono M, Nuryadi E, Sekine R, Oike T, Kakoti S, et al. DNA double-strand break repair pathway regulates PD-L1 expression in cancer cells. Nat Commun. 2017;8(1):1751. doi:10.1038/s41467-017-01883-9.
- Li X, Su X, Liu R, Pan Y, Fang J, Cao L, Feng C, Shang Q, Chen Y, Shao C, et al. HDAC inhibition potentiates anti-tumor activity of macrophages and enhances anti-PD-L1-mediated tumor suppression. Oncogene. 2021;40(10):1836–1850. doi:10.1038/s41388-020-01636-x.