Abstract
Measles virus (MeV) represents one of the main causes of death among young children, particularly in developing countries. Upon infection, MeV controls both interferon induction (IFN) and the interferon signaling pathway which results in a severe host immunosuppression that can persists for up to 6 mo after infection. Despite the global biology of MeV infection is well studied, the role of the plasmacytoid dendritic cells (pDCs) during the host innate immune response after measles vaccination remains largely uncharacterized. Here we investigated the role of pDCs, the major producers of interferon in response to viral infections, in the development of adaptive immune response against MeV vaccine. We report that there is a strong correlation between pDCs population and the humoral immune response to Edmonston Zagreb (EZ) measles vaccination in 9-month-old mexican infants. Five infants were further evaluated after vaccination, showing a clear increase in pDCs at baseline, one week and 3 months after immunization. Three months postvaccination they showed increase in memory T-cells and pDCs populations, high induction of adaptive immunity and also observed a correlation between pDCs number and the humoral immune response. These findings suggest that the development and magnitude of the adaptive immune response following measles immunization is directly dependent on the number of pDCs of the innate immune response.
Abbreviations:
- MeV, Measles virus
- IFN, interferon
- DCs, dendritic cells
- mDCs, myeloid dendritic cells
- pDCs, plasmacytoid dendritic cells
- EZ, Edmonston Zagreb
- (-) ssRNA, nonsegmented negative single-stranded RNA
- PBMCs, peripheral blood mononuclear cells
- MMR, measles, mumps, rubella vaccine
- PRN, plaque reduction neutralization
- GMT, Geometric mean titers
Introduction
Measles virus, a member of the Morbillivirus genus from the Paramyxoviridae family, is one of the most contagious human pathogens.Citation1 Despite the availability of an efficient vaccine based on attenuated MeV strains, measles remains one of the most important causes of death among children under the age of 5-years-old especially in developing countries.Citation2 This disease affects more than 20 million people each year worldwide and causes approximately 140,000 deaths in 2010.Citation3
MeV possesses a 15,894 nucleotides nonsegmented negative single-stranded RNA ((-) ssRNA) containing 6 genes encoding for 6 structural proteins, namely RNA polymerase-associated phosphoprotein (P), large polymerase (L) protein, nucleocapsid-nucleoprotein (N), haemagglutinin (H), fusion (F) envelope glycoproteins, matrix protein (M), and the non-structural proteins V and C.Citation4 The latter 2 are involved in the suppression of innate immune response, controlling the induction of interferon (IFN)Citation5-8 as well as the interferon signalingCitation9-17 pathways.
Infection with MeV results in lymphopenia, prolonged Th2 responses, and T-cell non-responsiveness. Although vaccination can cause similar effects, including a predominant Th2 response and depression of ex vivo mitogen-stimulated T-cell responses, it usually does not result in immunosuppression. Accordingly, it has been shown that using a live-attenuated MeV vaccine induces protective immunity without a clinically significant immune suppression.Citation18,19
According to their phenotype and functional characteristics, 2 types of dendritic cells (DCs) have been described in human peripheral blood,Citation20 the myeloid dendritic cells (mDCs) and plasmacytoid dendritic cells (pDCs). While mDCs are present in virtually all organsCitation21 and perform virtually all tasks involved in triggering immune response,Citation21,22 pDCs constitute a unique cell population comprising only 0.2–0.8% of all peripheral blood mononuclear cells (PBMCs).Citation23,24 The pDCs express surface molecules CD4, CD45RA, α-chain of IL-3 and Major Histocompatibility Complex (MHC II) molecules (HLA-DRII), but lack typical markers of other common cell lineages, CD3, CD11c, CD11b, CD14, CD16, CD19 and CD56. Additionally, the pDCs express specific markers such as CD303 (BDCA-2-type II transmembrane glycoprotein-C-type lectin) and CD304 (BDCA-4- neuropilin 1).Citation25,26 These cells are called the natural type I IFN-producing cells because they are able to produce 1–2 IU of IFN in response to viral stimulus, 100–1000 times more than other cells.Citation27,28 Indeed, type I and type III IFNs account for 60% of gene expression activity in activated pDCs.Citation29 This extraordinary ability to secrete high levels of IFN in response to virus infection directly depends on both, constitutive expression of Interferon Regulatory Factor 7 (IRF7) and the cell ability to retain DNA in early endosomes promoting the intimate contact with TLR9.Citation30,31 The IFN secreted mostly by pDCs is also important for pDCs survival, mDCs-mediated CD4+ and CD8+ T cell responses, mDCs maturation to antigen presenting cells, cross presentation, upregulation of MHC I and II, co-stimulatory molecules and activation of natural killer cells (NK).Citation32,33 IFN also stimulates B cells to produce antibodies, promotes the establishment of long-lasting B cell memory,Citation34 and induces Th1, Th2, or Treg populations.Citation35,36 Finally, in response to virus stimulation, activated pDCs also produce TNF-α, IL-6, IL-8, CCL2, CCL3, CCL5, CXCL10Citation37,38 and anti-microbial peptide human β-defensin 1 (HBD-1).Citation39 These cytokines play crucial immunomodulatory roles and affect both the innate and adaptive immune responses.Citation40,41 At the same time, these cells express surface receptors such as CXCR3, CXCR4, CCR5 and CD62L,Citation42 which allow pDCs to migrate to regional lymph nodes through high endothelial venules in response to SDF1 and other chemokines regulating the immune response.Citation43,44
The development of the adaptive immunity to measles vaccine has been widely described. However, the role of pDCs in measles vaccination has not been studied. pDCs play a crucial role in the innate immunity and IFN production that is important for the development of adaptive immunity. The aim of the study was to detect differences in the number of pDCs in children and adults and the role of the number of pDCs on the impact of the development of the adaptive immunity after measles immunization.
Results
Identification of pDCs
Different methods have been described to identify pDCs from total PBMCs using different cell markers.Citation28,45 In this study, we identified the pDCs population as described by Jego et al.Citation34 with slight modifications. We first identified total PBMCs by Forward and Side Scatter. Next, we isolated non-monocytes B, T and NK (Linneg) and HLA-DR-positive cells, from PBMCs (R2 inset). To further identify pDCs, we performed a second staining using a mouse anti–CD123-PE-Cy5 antibody, which allowed us to select HLADR+, CD123+ pDCs ().
Figure 1. Detection and measurement of pDCs. (A) Flow cytometry gating strategy to detect pDCs. Total peripheral blood mononuclear cells (PBMCs) were gated on the basis of forward and side scatter (FSC and SSC) (R1). Total DCs are identified as negative for the lineage markers, CD3, CD14, CD16, CD19 and CD56 (Lin-1), and positive for HLA-DR (R2). pDCs (R4) DCs were separated by HLA-DR high and then were phenotyped as pDCs (HLA-DR high, CD123 high). (B) A higher number of pDCs by mL of peripheral blood was detected in children (15) compared to adults (15)(*P < 0.001) but the percentage of the total number of all PBMCs were comparable.
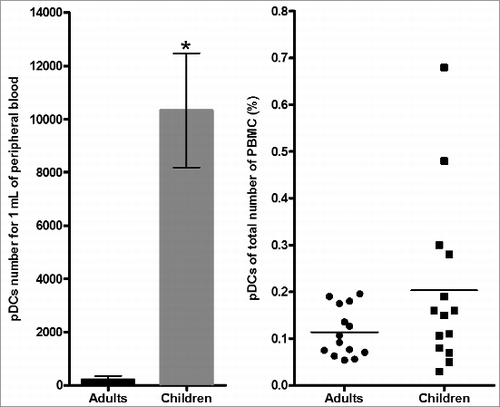
Infants and adults show different frequencies of pDCs
First, we determined the total number of pDCs per milliliter of blood by flow cytometry. We found a statistically significant higher number of pDCs between 9 month-old infants (mean ± SD, 10,328 ± 2,147 cells/ml) compared to adults (mean ± SD, 210 ± 141 cells/ml) (P < 0.001). In contrast the percentage of cells from the total cell population showed no differences between children (0.20%) and adults (0.11%) (p = 0.26) (). No significant difference in both gender and age between the 2 groups was found. Our results are consistent with previous studies.Citation46,47
Analysis of pDCs from healthy 9-months-old infants after measles vaccination
We immunized 14 children with the live attenuated measles vaccine by subcutaneous administration. No child presented side effects associated with vaccination. We analyzed the percentages of pDCs before, one week and 3 months after vaccination (prior to receiving their MMR). Only five out of 14 children were able to return for the second and third sample one week and 3 months post-vaccination. Although a statistical analysis using the Friedman test didn't show differences (p = 0.16) in the number of pDCs, , (Left) and percentages (Right) at the 3 time points analyzed, we observed a strong tendency in the increase in the number of pDCs (, Left). As shown in , we observed values of 9,904 cells/ml (0.18%) before vaccination, 15,305 cells/ml (0.58%) at one week, and 17,887 cells/ml (0.30%) at 3 months post-vaccination. A representative image of pDCs percentage prior and after measles vaccination is shown in .
Figure 2. Number and percentage of pDCs from 9 months old healthy children after measles vaccination. (A) The number of pDCs/mL from peripheral blood increased over time, but the percentage of pDCs from total number of PBMCs did not. (B) Representative results of the number of pDCs before, one week and 3 months after measles immunization in 9 months old children. p = NS
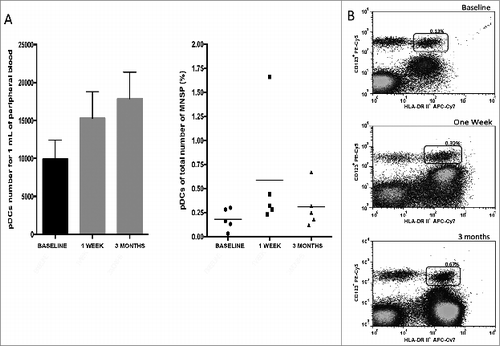
Evaluation of cell-mediated immune response and neutralizing antibodies to measles vaccine
All five children showed a SI < 3 before vaccination (mean + SE (simulation index) 1.22 ± 0.37). Three out of 5 children (60%) showed SI > 3 (mean + SE 3.99 ± 1.49) 3 months after immunization. In this evaluation, Wilcoxon test didn't proved a statistically significant difference in SI prior and 3 months after vaccination (p = 0.08; ). Furthermore, we assessed memory T-cell population stimulated with measles antigen prior and 3 months post-vaccination. Memory T-cells quantitation was performed by using the specific surface-markers CD4+, CD8+, CD62L− and CCR7−.Citation48 We observed an increase of the mean CD4+ T-cell memory population mean + SE 0.82 ± 0.17 vs 12.2 ± 3.55 and CD8+ T-cell memory population mean + SE 1.5 ± 0.50 vs 14.07 ± 3.64, before and 3 months after vaccination, respectively (). Mann Whitney U test showed statistical significance for T-cell frequencies (p = 0.028).
Figure 3. Immune response to measles vaccination in 9 months old healthy children. (A) The percentage of memory T CD4 and TCD8 lymphocytes increased 3 months after measles vaccination (n = 5). (*p = 0.028). (B) The specific IgG antibodies for measles virus GMT increased 3 months after measles immunization (n = 5). (*P < 0.05).
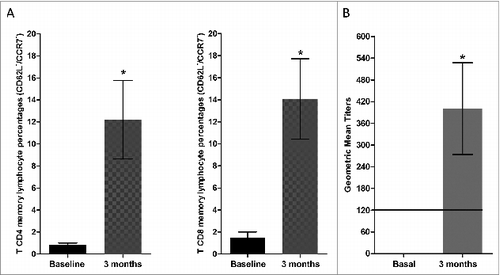
Table 1. Adaptive immune response in healthy 9 months-old children after measles vaccination
We next evaluated specific IgGs in serum by the PRN (plaque reduction neutralization assay) method. A PRN value of ≥120 mIU/ml is considered protective.Citation49 All 5 children had undetectable PRN measles antibodies before vaccination. Four out of 5 children showed seroconversion rates 3 months post-vaccination (80%, p=0.18). Geometric mean titers (GMT) showed a mean of 400 mIU/ml (SE ± 126) at 3 months (). Wilcoxon test showed that GMT difference was statistically significant 3 months after immunization (P < 0.05; ).
Adaptive immune response to measles vaccine
Considering that the adaptive immune response is protective with either cellular and/or humoral immunity, we analyzed specific T-cell and neutralizing antibody responses to measles antigen. We observed that all 5 children had a protective adaptive immune response due to the development of either response. At three months post-vaccination, 2/5 (40%) infants had both cellular and humoral immune responses, 2/5 (40%) developed a humoral immunity, and 1/5 (20%) presented only cellular immune response. Altogether, our results show that all vaccinated infants (100%) developed a protective immune response to measles virus after primary immunization ().
Correlation of pDCs and the humoral and cellular immune response
We assessed whether pDCs population had an impact on the development of the immune response. To address this question, we analyzed the MeV-mediated cellular and humoral response and the number of pDCs. We didn´t find a significant correlation between the basal numbers of pDCs with the cellular immune response to measles vaccination (R = 0.50; p = 0.39), neither between the baseline and one week pDCs population with MeV specific antibodies 3 months after vaccination (R = 0.60; p = 0.20) and (R = 0.48; p = 0.32) respectively. In contrast, we observed a strong positive correlation between pDCs and the humoral immune response at 3 months post vaccination, (R = 0.90 and P < 0.05) (). Interestingly, we note that the only patient who did not develop specific measles protective neutralizing antibodies presented the lowest number of pDCs at baseline as well as 3 months after measles vaccination (data not shown).
Figure 4. Correlation between the number of pDCs and geometric mean titers to measles, at baseline (Rho 0.60, p = 0.20), one week (Rho 0.48, p = 0.32) and 3 months (Rho 0.90, P < 0.05) with the humoral immune response 3 months after measles vaccination in 9 months old healthy children (each dot represent one subject).
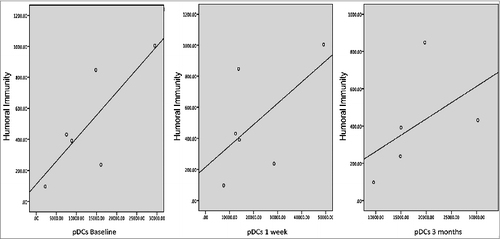
Discussion
We used a CD123 strategy for detecting only the pDCs phenotype from PBMCs,Citation28,34,45 rather than using CD303/CD304,Citation25,26,50 which is a better strategy to evaluate its function. In this study we observed a higher number of pDCs in 9 month-old infants compared to adults, very few publications include this age population.Citation51 Previous studies by Shodell et al.Citation47 revealed a progressive loss of pDCs in adults during aging. Moreover, in agreement with our results, children 2 to 5 y old showed higher levels of pDCs than adults.Citation46,51,52 However, we couldn't find another work that describes the number and percentage of pDCs of total PBMCs in healthy 9 months-old children after measles vaccination. Another novelty of the study is that other publications have looked at the number and percentage of pDCs only but no correlation with the humoral or cellular immunity after measles vaccination has been described before. The number and percentage of the pDCs is the baseline of the study and the differences between adults and children could set the basis to further explore the immune response to vaccination with the reference of the initial number of pDCs.
One possible explanation for adults to have a lower number of pDCs in peripheral blood has to do with its activation and movement toward high endothelial venules into lymph nodes, as already demonstrated.Citation43 The pDCs play an important role in the innate immune response against viruses and have the ability to link the adaptive immune response to develop an appropriate response.Citation53,54 We consider that the pDCs are particularly important in the early years of life, when different antigenic stimuli begin, while developing a protective adaptive immune response against several pathogens, then the pDCs begin to decrease in peripheral blood maybe because of a lower production in the bone marrow or because they migrate to lymph nodes. However, we didn't perform lymph node biopsies to measure this cell population, to confirm this hypothesis.
Several studies have established that pDCs play a crucial role in the development of innate immune response and its link with adaptive immune response and some experiments performed in cultured cells confirm the relevance of pDCs in this process. It is well known that pDCs have an important role in the defense against several viruses. They were first described in 1999 as a small subset of human leukocytes specialized in high levels of type I interferon secretion playing an important role as inmmunomodulators linking the innate and adaptive immune responses.Citation28,36,53,54 The IFNs are involved in the establishment of an antiviral state, blocking viral replication but also diminishing cellular processes as cell growth and promoting cell death by apoptosis in infected cells.Citation55 At the same time, once they recognize viral genetic material they have the ability to produce proinflammatory cytokines and chemokines inducing a Th1 polarization of human helper T cells and antibody production by B cells.Citation56 All these functions are an alert for the immune system to be aware of dangers and to activate the whole spectrum of effective immune reactions. However, whether or not measles vaccination has an impact on pDCs population in humans in vivo has not been studied. The identification of these DCs subsets is important because it provides information that may be useful for the evaluation of the function of these cells in disease. In this regard, several studies using a singleCitation57 or doubleCitation46 platform approach have demonstrated a significant and progressive reduction in the frequency of pDCs in peripheral blood, particularly in the early years of lifeCitation46,51,52,58,59 and decreases even more in the elderly.Citation47,51,52,59 In the current study we observed an increase in pDCs number/mL after measles vaccination at one week and 3 months. All patients were under strict medical supervision allowing us to attribute the increased number of pDCs to the effect of measles vaccination and not to another intercurrent illness. Despite the increase of pDCs at one week, we observed a decline in the percentage of pDCs out of the total number of mononuclear cells 3 months post vaccination. However, we also observed a significant and direct correlation between the number of pDCs and the development of protective humoral immune response at 3 months. The finding of the higher number of pDCs correlates with a higher level of protective measles antibodies and might suggest that according to the number of cells, pDCs produce a high level of IFN that induced a higher stimulation of T cells and B cells to produce higher levels of antibodies, in a direct correlation. One observation that also supports this is the fact that the child with the lowest number of pDCs did not develop a protective level of measles neutralizing antibodies, and only developed cellular mediated immunity. These data suggest that the pDCs population size might play a crucial role in the development of humoral immune response to MeV vaccination. To our knowledge, this is the first work that attempts to correlate the increase in pDCs with the development of subsequent adaptive protective immune response to measles vaccination.
One limitation in this study was to separate PBMCs by a gradient since many pDCs can be lost. However, the previous studies described in this paper where the number and percentage of pDCs phenotype is consistent with our results is performed in gradient separated PBMCs and not in whole blood. Another limitation of the study is the sample size. However, even with such a small number of children, we found a statistically significant difference. With a larger number, these observations might be even more pronounced. Further studies are needed to confirm these findings.
Patients and Methods
Study population
Fourteen healthy 9-months-old children (±4 weeks), identified from birth records from the Hospital General de Mexico (Mexico City), were recruited; it is noteworthy that these infants come from a cohort previously studied by Wong-Chew et.al.Citation60 Parents provided written informed consent, following the national ethics committee protocols. Infants received subcutaneous measles vaccine according to established exclusion criteria, namely history of previous measles vaccination, family history of allergy to egg proteins, gestation <36 weeks, birth weight <2500 g, malnutrition, and acute or chronic illnesses. Enrollment took place from April, 2008 to April, 2009. No measles cases were identified in Mexico City during this time interval or the previous year. Blood samples from 14 adults were obtained from the blood bank of the Hospital.
Measles vaccine and vaccine administration
Live attenuated EZ measles vaccine, with a potency of 103.85 TCID50/0.5 mL, produced by the Serum Institute of India, was used. Lyophilized vaccine was provided in 10 dose vials to which 5mL of diluent was added and used immediately. The vaccine was (103.85 TCID50 /0.5 mL) injected subcutaneously in left deltoid area.
According to the National immunization program in Mexico all infants received measles, mumps, rubella (MMR) vaccine (Serum Institute of India, LTD) by subcutaneous injection at 12 months of age.
Adverse reactions following immunization
Post-vaccination phone-evaluation for adverse reactions was performed every other day for 14-days and at day 30. In the event of any adverse reaction sign and/or symptom, parents could bring their children for examination.
Assays for measles immunity
Blood samples were collected from 14 infants (5 mL) prior to, 5 and 30 d after immunization and from 15 adults (20 mL) to evaluate pDCs populations, and from 5 infants before and 3 months after immunization (prior to their MMR vaccination), to assess measles-specific T-cell and neutralizing antibody responses to measles vaccine.
Frequencies of pDCs in peripheral blood
Five mL of freshly heparinized blood was obtained and analyzed by flow cytometry for quantitation and immunophenotyping of pDCs. PBMCs were isolated using a density-gradient separation with Ficoll Hypaque (GE Healthcare Life Sciencies) and were resuspended in complete RPMI medium (RPMI 1640 medium supplemented with 2 mmol/L-glutamine, 50 IU/mL penicillin, 50 μg/mL streptomycin [Gibco], and 10% fetal calf serum [FCS; Gibco]). One to 3 million PBMCs in 100 μL of PBS 1x (Invitrogen) supplemented with 10% FCS as blocking solution were incubated with monoclonal antibodies for 30 minutes in the dark at 4°C. To exclude cells other than DCs, we used a cocktail of phycoerythrin (PE)-conjugated monoclonal antibodies against CD3, CD14, CD16, CD19, CD20 and CD56 (Lineage Cocktail) and was defined as lineage negative. For general DCs immunophenotyping, 3-colour staining was performed with negative Lineage Cocktail: (I) mDCs were HLA-DR-Allophycocyanin-Cyanine7 (APC-Cy7) and CD11c-Fluorescein isothiocyanate (FITC) positive cells. (II) pDCs were defined as HLA-DR-APC-Cy7 and CD123-Phycoerytrin-Cyanine5 (PE-Cy5) positive cells. All antibodies were from Affymetrix eBioscience. After staining, cells were washed twice with cold phosphate-buffered saline (PBS) containing 0.6% bovine serum albumin and 0.01% sodium azide, and fixed in 2% paraformaldehyde in PBS. Flow cytometry was performed using a FacsCanto-II (Becton Dickinson). pDCs subpopulation within PBMCs were expressed as HLA-DR+, CD123high. After excluding dead cells based on threshold parameters, we obtained 1 × 106–3 × 106 events for each sample. The absolute number of circulating pDCs was calculated using the percentage of cells with respect to the white blood cell counts, as determined by an automated differential blood count. Acquired data were analyzed using the FlowJo software (TreeStar). The gating strategy used for pDC subsets discrimination is depicted in .
T cell proliferation assay
Fresh PBMCs were added to 96-well microtiter plates at 3 × 105 per well in RPMI 1640 (Gibco), and 40% normal human sera (Sigma). Measles antigen (Enders strain), prepared from either infected or uninfected (control) Vero cell lysates was added in triplicate at dilutions of 1:8 and 1:16. T-cell proliferation was measured after 5 d of incubation by adding tritiated thymidine (2.5 µCi per well) for 18 hours. Phytohemagglutinin (Difco) was used as positive control. Proliferation was expressed as the stimulation index (SI) expressed as the ratio of mean counts per minute (cpm) of measles antigen-stimulated samples over the mean cpm of control samples. An SI≥3 is considered a positive response.Citation61-65
Cellular immune response was also measured by flow cytometry as follows, 3 × 105 PBMCs per well were incubated in RPMI 1640 (Gibco) containing 40% normal human serum (Sigma) for 5 d and then stimulated either with measles antigen or Vero cell control. Cells were gated on the basis of forward and side scatter in the lymphocyte region. T cells were initially separated and stained with monoclonal antibodies against CD4-PECy5 and CD8-PECy7. The memory T cells were determined using CD62L-APCCy7 and CCR7-APC markers (Affymetrix eBioscience). The CD4+ and CD8+ memory cells were gated and identified as negative for these 2 markers CD62Lneg/CCR7neg. Four colors per well were measured and compensation was performed with non-stimulated cells with simple staining for each color; 10,000 cells per patient were acquired in the cytometer FacsCanto-II (Becton Dickinson) and analyzed with FlowJo software (TreeStar). Mean cell frequencies before and 3 months after vaccination were compared.
Plaque reduction neutralizing antibody assay
Sera were stored at −70°C. Paired specimens prior and 3 months after vaccination were tested for neutralizing measles antibodies in parallel with the WHO Measles Reference Serum II, 66/202, using a modified plaque reduction neutralization (PRN) assay.Citation66 PRN titer was defined as the serum dilution that reduced the number of plaques by 50%. Titers less than 1:4 were considered negative. Seroconversion was defined as a 4-fold rise in antibody titer above levels prior to vaccination. Measles seroprotection against severe disease is defined as an IgG PRN titer ≥1:120Citation49 which is equivalent to 120 mIU/mL based on the performance of the WHO Measles Reference Serum II, 66/202 in this assay.
Statistical analysis
Analysis of immune response data was limited to children whose blood was obtained for testing both pre- and post-immunization. Baseline characteristics of children were summarized with descriptive statistics. Statistical analysis were carried out using SPSS for Mac version 20.0 (IBM) and Graphpad Prism 6. The Mann-Whitney U test was used to compare differences in cell frequencies, Friedman test to compare increases of cell frequencies in repeated measures, Chi squared tests to compare the difference in humoral immunity, Spearman test to find correlations between variables.
Disclosure of Potential Conflicts of Interest
No potential conflicts of interest were disclosed.
Acknowledgments
This work is part of the DSc dissertation submitted by García-León ML. in partial fulfillment for the degree requirements of “Doctorado en Ciencias Biomédicas de la Universidad Nacional Autónoma de México”. Also we want to thank Judy A. Beeler and Susette Audet from Division of Viral Products, The Food and Drug Administration, Silver Spring, Maryland for the measurement of measles specific IgGs by the PRN method; the Serum Institute of India, LTD. for the generous donation of measles vaccine; the families of Mexico City who agreed to participate in the study and finally to the Liver, Pancreas and Motility unit of the Universidad Nacional Autónoma de México for the use of the FacsCanto II cytometer. The Institutional Review Board of the School of Medicine, Universidad Nacional Autónoma de México and the Institutional Review Board of the Hospital General de México approved the study; written consent was obtained from parents or guardians and adults.
Funding
This work was supported by a grant from the Fogarty International Center and the Office of Research on Women's Health (Grant #TW006193, PI RM Wong-Chew). The author wants to thank “Consejo Nacional de Ciencia y Tencnología” CONACYT for the support of Miguel García Leon for his doctoral studies by grant #165414.
References
- Moss WJ, Griffin DE. Measles. Lancet 2012; 379:153–64; PMID: 21855993; http://dx.doi.org/10.1016/S0140-6736(10)62352-5
- Rall GF. Measles virus 1998–2002: progress and controversy. Annu Rev Microbiol 2003; 57:343–67; PMID:14527283; http://dx.doi.org/10.1146/annurev.micro.57.030502.090843
- Simons E, Ferrari M, Fricks J, Wannemuehler K, Anand A, Burton A, Strebel P. Assessment of the 2010 global measles mortality reduction goal: results from a model of surveillance data. Lancet 2010; 379:2173–8; PMID:22534001; http://dx.doi.org/10.1016/S0140-6736(12)60522-4
- Griffin D. Measles virus. In Fields Virology, Knipe DMGDE, Howley PM (ed), 5 ed, vol 2, Philadelphia, PA: Lippincott Williams & Wilkins; 2007:1551–81.
- Nakatsu Y, Takeda M, Ohno S, Koga R, Yanagi Y. Translational inhibition and increased interferon induction in cells infected with C protein-deficient measles virus. J Virol 2006; 80:11861–7; PMID:1642609; http://dx.doi.org/10.1128/JVI.00751-06
- Nakatsu Y, Takeda M, Ohno S, Shirogane Y, Iwasaki M, Yanagi Y. Measles virus circumvents the host interferon response by different actions of the C and V proteins. J Virol 2008; 82:8296–306; PMID:2519641; http://dx.doi.org/10.1128/JVI.00108-08
- Bellini WJ, Englund G, Rozenblatt S, Arnheiter H, Richardson CD. Measles virus P gene codes for two proteins. J Virol 1985; 53:908–19; PMID:254726,
- Escoffier C, Manie S, Vincent S, Muller CP, Billeter M, Gerlier D. Nonstructural C protein is required for efficient measles virus replication in human peripheral blood cells. J Virol 1999; 73:1695–8; PMID:104001
- Andrejeva J, Childs KS, Young DF, Carlos TS, Stock N, Goodbourn S, Randall RE. The V proteins of paramyxoviruses bind the IFN-inducible RNA helicase, mda-5, and inhibit its activation of the IFN-beta promoter. Proc Natl Acad Sci U S A 2004; 101:17264–9; PMID:535396; http://dx.doi.org/10.1073/pnas.0407639101
- Caignard G, Guerbois M, Labernardiere JL, Jacob Y, Jones LM, Wild F, Tangy F, Vidalain PO. Measles virus V protein blocks Jak1-mediated phosphorylation of STAT1 to escape IFN-alpha/beta signaling. Virology 2007; 368:351–62; PMID:17686504; http://dx.doi.org/10.1016/j.virol.2007.06.037
- Childs KS, Andrejeva J, Randall RE, Goodbourn S. Mechanism of mda-5 Inhibition by paramyxovirus V proteins. J Virol 2009; 83:1465–73; PMID:2620892; http://dx.doi.org/10.1128/JVI.01768-08
- Devaux P, von Messling V, Songsungthong W, Springfeld C, Cattaneo R. Tyrosine 110 in the measles virus phosphoprotein is required to block STAT1 phosphorylation. Virology 2007; 360:72–83; PMID:17112561; http://dx.doi.org/10.1016/j.virol.2006.09.049
- Ohno S, Ono N, Takeda M, Takeuchi K, Yanagi Y. Dissection of measles virus V protein in relation to its ability to block alpha/beta interferon signal transduction. J Gen Virol 2004; 85:2991–9; PMID:15448362; http://dx.doi.org/10.1099/vir.0.80308-0
- Pfaller CK, Conzelmann KK. Measles virus V protein is a decoy substrate for IkappaB kinase alpha and prevents Toll-like receptor 7/9-mediated interferon induction. J Virol 2008; 82:12365–73; PMID:2593327; http://dx.doi.org/10.1128/JVI.01321-08
- Ramachandran A, Parisien JP, Horvath CM. STAT2 is a primary target for measles virus V protein-mediated alpha/beta interferon signaling inhibition. J Virol 2008; 82:8330–8; PMID:2519631; http://dx.doi.org/10.1128/JVI.00831-08
- Sparrer KM, Pfaller CK, Conzelmann KK. Measles virus C protein interferes with interferon beta transcription in the nucleus. J virol 2012; 86(2):796-805; DOI: 10.1128/IVI.05899-11
- Takeuchi K, Kadota SI, Takeda M, Miyajima N, Nagata K. Measles virus V protein blocks interferon (IFN)-alpha/beta but not IFN-gamma signaling by inhibiting STAT1 and STAT2 phosphorylation. FEBS Lett 2003; 545:177–82; PMID:17686504
- Fireman P, Friday G, Kumate J. Effect of measles vaccine on immunologic responsiveness. Pediatrics 1969; 43:264–72; PMID:4179145
- Hussey GD, Goddard EA, Hughes J, Ryon JJ, Kerran M, Carelse E, Strebel PM, Markowitz LE, Moodie J, Barron P, et al. The effect of Edmonston-Zagreb and Schwarz measles vaccines on immune response in infants. J Infect Dis 1996; 173:1320–6; PMID:8648203
- O'Doherty U, Peng M, Gezelter S, Swiggard WJ, Betjes M, Bhardwaj N, Steinman RM. Human blood contains two subsets of dendritic cells, one immunologically mature and the other immature. Immunology 1994; 82:487–93; PMID:1414873
- Banchereau J, Steinman RM. Dendritic cells and the control of immunity. Nature 1998; 392:245–52; PMID:9521319; http://dx.doi.org/10.1038/32588
- Cella M, Sallusto F, Lanzavecchia A. Origin, maturation and antigen presenting function of dendritic cells. Curr Opin Immunol 1997; 9:10–6; PMID:9039784
- Hosmalin A, Lichtner M, Louis S. Clinical analysis of dendritic cell subsets: the dendritogram. Methods Mol Biol 2008; 415:273–90; PMID:18370160; http://dx.doi.org/10.1007/978-1-59745-570-1_16
- Feldman SB, Milone MC, Kloser P, Fitzgerald-Bocarsly P. Functional deficiencies in two distinct interferon alpha-producing cell populations in peripheral blood mononuclear cells from human immunodeficiency virus seropositive patients. J Leukoc Biol 1995; 57:214–20; PMID:7852835
- Dzionek A, Inagaki Y, Okawa K, Nagafune J, Rock J, Sohma Y, Winkels G, Zysk M, Yamaguchi Y, Schmitz J. Plasmacytoid dendritic cells: from specific surface markers to specific cellular functions. Hum Immunol 2002; 63:1133–48; PMID:12480257
- Dzionek A, Fuchs A, Schmidt P, Cremer S, Zysk M, Miltenyi S, Buck DW, Schmitz J. BDCA-2, BDCA-3, and BDCA-4: three markers for distinct subsets of dendritic cells in human peripheral blood. J Immunol 2000; 165:6037–46; PMID:11086035; http://dx.doi.org/10.4049/jimmunol.165.11.6037
- Swiecki M, Colonna M. Unraveling the functions of plasmacytoid dendritic cells during viral infections, autoimmunity, and tolerance. Immunol Rev 2010; 234:142–62; PMID:3507434; http://dx.doi.org/10.1111/j.0105-2896.2009.00881.x
- Siegal FP, Kadowaki N, Shodell M, Fitzgerald-Bocarsly PA, Shah K, Ho S, Antonenko S, Liu YJ. The nature of the principal type 1 interferon-producing cells in human blood. Science 1999; 284:1835–7; PMID:10364556
- Ito T, Kanzler H, Duramad O, Cao W, Liu YJ. Specialization, kinetics, and repertoire of type 1 interferon responses by human plasmacytoid predendritic cells. Blood 2006; 107:2423–31; PMID:16293610; http://dx.doi.org/10.1182/blood-2005-07-2709
- Izaguirre A, Barnes BJ, Amrute S, Yeow WS, Megjugorac N, Dai J, Feng D, Chung E, Pitha PM, Fitzgerald-Bocarsly P. Comparative analysis of IRF and IFN-alpha expression in human plasmacytoid and monocyte-derived dendritic cells. J Leukoc Biol 2003; 74:1125–38; PMID:12960254; http://dx.doi.org/10.1189/jlb.0603255
- Honda K, Ohba Y, Yanai H, Negishi H, Mizutani T, Takaoka A, Taya C, Taniguchi T. Spatiotemporal regulation of MyD88-IRF-7 signalling for robust type-I interferon induction. Nature 2005; 434:1035–40; PMID:15815647; http://dx.doi.org/10.1038/nature03547
- Le Bon A, Etchart N, Rossmann C, Ashton M, Hou S, Gewert D, Borrow P, Tough DF. Cross-priming of CD8+ T cells stimulated by virus-induced type I interferon. Nat Immunol 2003; 4:1009–15; PMID:14502286; http://dx.doi.org/10.1038/ni978
- Le Bon A, Schiavoni G, D'Agostino G, Gresser I, Belardelli F, Tough DF. Type i interferons potently enhance humoral immunity and can promote isotype switching by stimulating dendritic cells in vivo. Immunity 2001; 14:461–70; PMID:11336691
- Jego G, Palucka AK, Blanck JP, Chalouni C, Pascual V, Banchereau J. Plasmacytoid dendritic cells induce plasma cell differentiation through type I interferon and interleukin 6. Immunity 2003; 19:225–34; PMID:12932356
- Cella M, Facchetti F, Lanzavecchia A, Colonna M. Plasmacytoid dendritic cells activated by influenza virus and CD40L drive a potent TH1 polarization. Nat Immunol 2000; 1:305–10; PMID:11017101; http://dx.doi.org/10.1038/79747
- Kadowaki N, Antonenko S, Lau JY, Liu YJ. Natural interferon alpha/beta-producing cells link innate and adaptive immunity. J Exp Med 2000; 192:219–26; PMID:2193254
- Penna G, Vulcano M, Roncari A, Facchetti F, Sozzani S, Adorini L. Cutting edge: differential chemokine production by myeloid and plasmacytoid dendritic cells. J Immunol 2002; 169:6673–6; PMID:12471096; http://dx.doi.org/10.4049/jimmunol.169.12.6673
- Penna G, Vulcano M, Sozzani S, Adorini L. Differential migration behavior and chemokine production by myeloid and plasmacytoid dendritic cells. Hum Immunol 2002; 63:1164–71; PMID:12480260
- Ryan LK, Diamond G, Amrute S, Feng Z, Weinberg A, Fitzgerald-Bocarsly P. Detection of HBD1 peptide in peripheral blood mononuclear cell subpopulations by intracellular flow cytometry. Peptides 2003; 24:1785–94; PMID:15019211; http://dx.doi.org/10.1016/j.peptides.2003.09.021
- Le Bon A, Durand V, Kamphuis E, Thompson C, Bulfone-Paus S, Rossmann C, Kalinke U, Tough DF. Direct stimulation of T cells by type I IFN enhances the CD8+ T cell response during cross-priming. J Immunol 2006; 176:4682–9; PMID:16585561
- Coro ES, Chang WL, Baumgarth N. Type I IFN receptor signals directly stimulate local B cells early following influenza virus infection. J Immunol 2006; 176:4343–51; PMID:16547272
- Vanbervliet B, Bendriss-Vermare N, Massacrier C, Homey B, de Bouteiller O, Briere F, Trinchieri G, Caux C. The inducible CXCR3 ligands control plasmacytoid dendritic cell responsiveness to the constitutive chemokine stromal cell-derived factor 1 (SDF-1)/CXCL12. J Exp Med 2003; 198:823–30; PMID:2194187; http://dx.doi.org/10.1084/jem.20020437
- Yoneyama H, Matsuno K, Zhang Y, Nishiwaki T, Kitabatake M, Ueha S, Narumi S, Morikawa S, Ezaki T, Lu B, et al. Evidence for recruitment of plasmacytoid dendritic cell precursors to inflamed lymph nodes through high endothelial venules. Int Immunol 2004; 16:915–28; PMID:15159375; http://dx.doi.org/10.1093/intimm/dxh093
- Diacovo TG, Blasius AL, Mak TW, Cella M, Colonna M. Adhesive mechanisms governing interferon-producing cell recruitment into lymph nodes. J Exp Med 2005; 202:687–96; PMID:2212867; http://dx.doi.org/10.1084/jem.20051035
- Grouard G, Rissoan MC, Filgueira L, Durand I, Banchereau J, Liu YJ. The enigmatic plasmacytoid T cells develop into dendritic cells with interleukin (IL)-3 and CD40-ligand. J Exp Med 1997; 185:1101–11; PMID:2196227
- Teig N, Moses D, Gieseler S, Schauer U. Age-related changes in human blood dendritic cell subpopulations. Scand J Immunol 2002; 55:453–7; PMID:11975756
- Shodell M, Siegal FP. Circulating, interferon-producing plasmacytoid dendritic cells decline during human ageing. Scand J Immunol 2002; 56:518–21; PMID:12410802
- Moulton VR, Farber DL. Committed to memory: lineage choices for activated T cells. Trends Immunol 2006; 27:261–7; PMID:16684621; http://dx.doi.org/10.1016/j.it.2006.04.006
- Chen RT, Markowitz LE, Albrecht P, Stewart JA, Mofenson LM, Preblud SR, Orenstein WA. Measles antibody: reevaluation of protective titers. J Infect Dis 1990; 162:1036–42; PMID:2230231
- Colonna M, Trinchieri G, Liu YJ. Plasmacytoid dendritic cells in immunity. Nat Immunol 2004; 5:1219–26; PMID: 15549123; http://dx.doi.org/10.1038/ni1141
- Heinze A, Elze MC, Kloess S, Ciocarlie O, Konigs C, Betz S, Bremm M, Esser R, Klingebiel T, Serban M, et al. Age-matched dendritic cell subpopulations reference values in childhood. Scand J Immunol 2013; 77:213–20; PMID:23298344; http://dx.doi.org/10.1111/sji.12024
- Jyonouchi H, Cui C, Geng L, Yin Z, Fitzgerald-Bocarsly P. Age-dependent changes in peripheral blood dendritic cell subsets in normal children and children with specific polysaccharide antibody deficiency (SPAD). Eur J Pediatr 2010; 169:1233–9; PMID:3579493; http://dx.doi.org/10.1007/s00431-010-1210-y
- McKenna K, Beignon AS, Bhardwaj N. Plasmacytoid dendritic cells: linking innate and adaptive immunity. J Virol 2005; 79:17–27; PMID:538703; http://dx.doi.org/10.1128/JVI.79.1.17-27.2005
- Zhang Z, Wang FS. Plasmacytoid dendritic cells act as the most competent cell type in linking antiviral innate and adaptive immune responses. Cell Mol Immunol 2005; 2:411–7; PMID:16426490
- Fontana JM, Bankamp B, Rota PA. Inhibition of interferon induction and signaling by paramyxoviruses. Immunol Rev 2008; 225:46–67; PMID:18837775; http://dx.doi.org/10.1111/j.1600-065X.2008.00669.x
- Colonna M, Krug A, Cella M. Interferon-producing cells: on the front line in immune responses against pathogens. Curr Opin Immunol 2002; 14:373–9; PMID:11973137
- Vuckovic S, Gardiner D, Field K, Chapman GV, Khalil D, Gill D, Marlton P, Taylor K, Wright S, Pinzon-Charry A, et al. Monitoring dendritic cells in clinical practice using a new whole blood single-platform TruCOUNT assay. J Immunol Methods 2004; 284:73–87; PMID:14736418
- Upham JW, Zhang G, Rate A, Yerkovich ST, Kusel M, Sly PD, Holt PG. Plasmacytoid dendritic cells during infancy are inversely associated with childhood respiratory tract infections and wheezing. J Allergy Clin Immunol 2009; 124:707–13.e2; PMID:19733903; http://dx.doi.org/10.1016/j.jaci.2009.07.009
- Chen X, Makala LH, Jin Y, Hopkins D, Muir A, Garge N, Podolsky RH, She JX. Type 1 diabetes patients have significantly lower frequency of plasmacytoid dendritic cells in the peripheral blood. Clin Immunol 2008; 129:413–8; PMID:2602829; http://dx.doi.org/10.1016/j.clim.2008.08.013
- Wong-Chew RM, Garcia-Leon ML, Espinosa-Torres Torrija B, Hernandez-Perez B, Cardiel-Marmolejo LE, Beeler JA, Audet S, Santos-Preciado JI. Increasing the time of exposure to aerosol measles vaccine elicits an immune response equivalent to that seen in 9-month-old Mexican children given the same dose subcutaneously. J Infect Dis 2011; 204:426–32; PMID:3165943; http://dx.doi.org/10.1093/infdis/jir278
- Gans H AA, Galinus J, Logan L, DeHovitz R, Maldonado Y. Deficiency of the humoral immune response to measles vaccine in infants immunized at age 6 months. JAMA 1998; 280:527–32; PMID:9707142
- Gans H, Yasukawa L, Rinki M, DeHovitz R, Forghani B, Beeler J, Audet S, Maldonado Y, Arvin AM. Immune responses to measles and mumps vaccination of infants at 6, 9, and 12 months. J Infect Dis 2001; 184:817–26; PMID:11528592; http://dx.doi.org/10.1086/323346
- Bautista-Lopez N, Ward BJ, Mills E, McCormick D, Martel N, Ratnam S. Development and durability of measles antigen-specific lymphoproliferative response after MMR vaccination. Vaccine 2000; 18:1393–401; PMID:10618537; http://dx.doi.org/ S0264410´99003965
- Wong-Chew RM, Islas-Romero R, Garcia-Garcia Mde L, Beeler JA, Audet S, Santos-Preciado JI, Gans H, Lew-Yasukawa L, Maldonado YA, Arvin AM, et al. Induction of cellular and humoral immunity after aerosol or subcutaneous administration of Edmonston-Zagreb measles vaccine as a primary dose to 12-month-old children. J Infect Dis 2004; 189:254–7; PMID:14722890; http://dx.doi.org/10.1086/380565
- Wong-Chew RM, Islas-Romero R, Garcia-Garcia Mde L, Beeler JA, Audet S, Santos-Preciado JI, Gans H, Lew-Yasukawa L, Maldonado YA, Arvin AM, et al. Immunogenicity of aerosol measles vaccine given as the primary measles immunization to nine-month-old Mexican children. Vaccine 2006; 24:683–90; PMID:16154241; http://dx.doi.org/10.1016/j.vaccine.2005.08.045
- Albrecht P, Herrmann K, Burns GR. Role of virus strain in conventional and enhanced measles plaque neutralization test. J Virol Methods 1981; 3:251–60; PMID:7334066