Abstract
Clinical observations of spontaneous disease regression in some renal cell carcinoma (RCC) patients implicate a role for tumor immunity in controlling this disease. Puzzling, however, are findings that high levels of tumor infiltrating lymphocytes (TIL) are common to RCC. Despite expression of activation markers by TILs, functional impairment of innate and adaptive immune cells has been consistently demonstrated contributing to the failure of the immune system to control RCC. Immunotherapy can overcome the immunosuppressive effects of the tumor and provide an opportunity for long-term disease free survival. Unfortunately, complete response rates remain sub-optimal indicating the effectiveness of immunotherapy remains limited by tumor-specific factors and/or cell types that inhibit antitumor immune responses. Here we discuss immunotherapies and the function of multiple immune system components to achieve an effective response. Understanding these complex interactions is essential to rationally develop novel therapies capable of renewing the immune system's ability to respond to these tumors.
Introduction
Renal cell carcinoma (RCC) is the third most common urogenital cancer in the world, and affects ∼60,000 people in the US each year.Citation1 Clinical management of RCC is variable, and depends on tumor stage at the time of diagnosis. Standard treatment for localized RCC tumors with no evidence of metastases or a single metastasis is surgery to remove the tumor-bearing kidney (nephrectomy) and metastatic lesion, and 5-year survival rates for these patients are high (˜90%).Citation2 Yet, ∼30% of RCC patients have multiple metastases at diagnosis, and a third of patients who undergo nephrectomy will develop metastases. Metastatic RCC (mRCC) is incurable, with a median survival time of only 7–11 months and a 5-year survival rate of less than 10%; poor survival is mainly attributed to resistance to chemotherapy and radiotherapy in these patients.Citation3
Patients with locally mRCC can benefit from surgical resection of the tumor-bearing kidney and metastatic lesions. Rare patients with mRCC (∼0.1%) can experience spontaneous remission following nephrectomy supporting the notion that RCC could be an immunologically controlled malignancy.Citation4 However, patients with multiple, systemic metastases generally do not benefit from nephrectomy and require augmented immune intervention. Two immunotherapies have shown efficacy for the treatment of mRCC: interleukin (IL)-2 and interferon (IFN)-αCitation5. The exact mechanisms by which these therapies work are not completely understood, but it is thought that both stimulate an immune response to the tumor.Citation6-9 IFN-α is also thought to have direct anti-proliferative effects on tumor cells and anti-angiogenic effects on the tumor microenvironment.Citation10 Though these agents have demonstrated some efficacy, the effects have been limited. IFN-α has only increased the median survival of mRCC patients by 7 months, with a response rate of less than 20%.Citation11 IL-2 has demonstrated slightly greater survival benefits (reaching 13–17 months); however, the high dose needed to achieve these results is often intolerable to the patient.Citation12 Small molecule inhibitors have demonstrated efficacy in extending survival. The multi-kinase inhibitor, Sunitnib, was able to prolong survival by 6 months compared to IFN-α, but this benefit was only afforded to 31% of patients.Citation13,14 Sorafenib, another multi-kinase inhibitor approved for the treatment of RCC, can extend progression-free survival for 10% of patients an additional 3 months over Sunitnib.Citation15 While these therapies have shown “significant” clinical increases in response rate and progression-free survival compared to placebos and controls, it is clear that improved therapies are needed for mRCC.
The Goal of Cancer Immunotherapy
Immunotherapy for cancer is designed to mobilize an antitumor immune response sufficient to selectively kill malignant cells and promote long-term tumor-free survival.Citation16 Clinical trials with tumor vaccines utilizing synthetic peptides derived from tumor antigens have had limited success.Citation16,17 One limitation of peptide-based tumor vaccines is major histocompatibility complex (MHC) restriction, and therefore diminished utility in diverse populations.Citation18 Suboptimal efficacy could also result from tumor heterogeneity, emergence of tumor cells that lose the targeted antigen, and immunological modulation that limit a productive antitumor immune response. Autologous whole tumor cells include all antigens that a person's immune system could potentially respond to, representing a more exhaustive repertoire of tumor antigens. However, generation of autologous whole tumor cell vaccines requires tumor tissue collection with careful and costly ex vivo processing.Citation19 To circumvent these technical/financial constraints, but at the same time harness the most relevant tumor antigens for an individual patient, protocols based on the premise of generating an in situ whole tumor cell-based vaccine may prove most beneficial. The success of such an approach requires the coordinated function of multiple immune system components to achieve an effective response, as well as reducing the immunosuppressive activity of other cells and molecules augmented by the presence of the tumor.
Tumoricidal Agents
The first step in the generation of an antitumor immune response is the production of a tumor antigen-laden debris field. A number of treatments can induce tumor cell death within the kidney, including radiation, chemotherapy, radiofrequency ablation, and thermal ablation.Citation20-22 These techniques can potently induce tumor cell death; however, none discriminate between tumor cells and healthy cells. As a consequence of these treatments, cell death occurs via an apoptotic or necrotic mechanism. It is becoming more appreciated that the means by which a cell dies can determine the degree of immunogenicity or tolerogenicity of the dead cells.Citation23,24 An alternative to these cytotoxic approaches is the use of reagents that specifically target the genes or proteins expressed by tumor cells or the surrounding tumor microenvironment. For example, drugs that target tyrosine kinases (TKIs; sorafenib, sunitinib, pazopanib, and axitinib) are designed to inhibit vascular endothelia growth factor (VEGF) and angiogenesis.Citation25,26 TKIs can work well in slowing the growth of kidney tumors, but their use can be associated with significant side-effects and tumor cell growth resumes when treatment stops. Selective tumor cell death can also result from use of TNF-related apoptosis-inducing ligand (TRAIL).Citation27 Considerable effort has been devoted to development of recombinant TRAIL protein, small molecules to augment TRAIL-induced killing, agonistic antibodies specific for the death-inducing TRAIL receptors, and TRAIL-based gene therapies for cancer therapy.Citation28-36 Direct induction of tumor cell apoptosis is the typical means by which a TRAIL receptor agonist is thought to function in vivo, but recent data suggest that endothelial cells within a tumor also selectively express TRAIL receptor.Citation37 Disruption of tumor vasculature by TRAIL can also lead to tumor size reduction – even for tumors that lack TRAIL receptor. Regardless of the means by which tumor cell death occurs, generation of a debris field of tumor antigens can serve as a powerful stimulus for an antitumor immune response.
Dendritic Cells
Following tumor cell death, the next component important for the generation of an antitumor immune response is dendritic cells (DCs). DCs sample their environment and capture, process and present antigens; functions for which they are known as professional antigen-presenting cells (APC).Citation38 The ability of DCs to process and present antigens acquired from internalized apoptotic cells is well recognized,Citation39-41 and immunity or tolerance can be induced depending on the context of antigen uptake by DCs.Citation42 Phagocytosis of apoptotic cells by immature DCs can be subimmunogenic and even tolerogenic under normal conditions or within the immunosuppressive tumor microenvironment.Citation43 Thus, a successful immunotherapeutic approach for treating cancer should consider the possible necessity for an adjuvant to activate DCs and elicit a strong antitumor immune response.
DCs present antigen as peptide fragments on MHC molecule complexes to T cells to induce adaptive immunity. Typically, DCs in peripheral tissues and circulation capture antigen and migrate into draining lymph nodes where they present antigen to naïve T cells. Alternatively, antigens can reach the lymph node-resident DCs through the lymphatic drainage system. In addition to capturing and processing antigens, DCs must also become activated to upregulate costimulatory molecules. DCs express a plethora of “sensing” molecules including pattern recognition receptors (PRRs) that detect pathogen-associated molecular patterns (PAMPs). Activation of DCs, specifically plasmacytoid DC (pDC) and CD8α DC, via toll-like receptors (TLRs) leads to upregulation of costimulatory molecules CD40, CD80 and CD86. Though both CD8α DC and pDC express MHC II at basal levels, MHC II expression increases with DC activation.
Following TLR-mediated activation, both pDC and CD8α DC are capable of making type I IFN, albeit to varying degrees, and CD8α DC can also produce IL-12 and IL-15, all of which are essential for optimal antitumor immunity.Citation44-50 Type I IFN is important for activation of the adaptive immune response, and additional mechanisms of DC action.Citation51,52 Data suggest that for DCs, in particular CD8α DC, type I IFN is important for optimal functionality, such as uptake, processing and retention of antigens.Citation53 The efficiency of these functions by CD8α DC is essential for the cross-presentation and priming of CD8+ T cells. With costimulatory molecules expressed and a peptide-loaded MHC I complex, these cross-presenting DC are capable of activating naïve CD8+ T cells into antitumor effector cells.
Because of their potent immunostimulatory capacity, DC-based immunotherapy for RCC has been tested in a number of clinical trials.Citation54,55 The DCs used in these clinical protocols are typically generated from autologous peripheral blood monocytes loaded with RCC-derived mRNA or dead RCC cells and infused back into the patient in the form of a “vaccine.” Such an approach has been tolerated well by the recipients, with few adverse effects that lead to toxicity or decreased quality-of-life.Citation56 Cellular immune responses, evidenced by the increased activity of tumor-specific CD8 T cells and NK cells, have been reported in many patients.Citation57,58 Unfortunately, objective response rates have been low in mRCC patients receiving DC therapy.Citation59 It is becoming evident that use of DC-based immunotherapy for RCC may require additional components to enhance the stimulatory capacity of DCs. For example, the combination of DCs with other immunostimulatory cytokines (e.g., IL-2), TLR agonists, agents that reverse tumor-induced immune suppression (e.g.,, denileukin diftitox to deplete regulatory T cells,Citation60 or drugs to alter myeloid-derived suppressor cells number/recruitmentCitation61-65), and/or chemotherapeutics to reduce tumor burden may stimulate a more robust antitumor immune response.Citation66
CD8+ T Cells
Eliciting a therapeutic antitumor immune response in cancer has been a long-standing goal of immunology. While chemotherapeutics and radiation can confer some initial benefit, the ultimate goal of cancer therapy is to induce antitumor immunity sufficient to clear detectable cancerous lesions and small undetectable foci and inhibit recurrence. However, activation of a robust antitumor CD8+ T cell response is a multifaceted process. Tumors adopt various mechanisms to escape and/or suppress the antitumor immune response, and therapies must not only activate tumor-specific CD8+ T cells but also overcome immunosuppression exerted by the tumor.
Activation and subsequent development of effector and memory CD8+ T cellsCitation67 requires that T cells must come in contact with their cognate peptide bound to MHC I on an APC and be costimulated by the APCs and cytokines they produce. The activation of antitumor CD8+ T cells results from cross-presentation of tumor cell-derived antigens by APCs. Concomitantly, as CD8+ T cells are binding their cognate peptide, they also engage costimulatory molecules on the APC.Citation68 Activation typically occurs via ligation of CD28 on the T cell to CD80/CD86 on the APC, leading to increased cytokine gene expression, promotion of T cell survival and maintenance of T cell responsiveness.Citation68 Lack of costimulation results in T cells becoming hyporesponsive (anergic).Citation69 Following TCR/CD8 activation and costimulation, T cells must be exposed to cytokines, such as type I IFN and IL-12 for optimal activation, proliferation/survival and subsequent effector function.Citation67,69,70 Moreover, additional stimuli generated in response to ligation of a number of other costimulatory receptors found on T cells, such as CD27, CD40, CD120b (TNFR2), CD134 (OX-40), and CD137 (4–1BB), are needed to achieve maximal T cell responses.Citation71 Together, the presentation of tumor-derived peptides in the context of MHC I on an APC, combined with costimulation and cytokines provided via activated APC are all necessary for the induction of a robust antitumor CD8+ T cell effector response. Several studies have examined the therapeutic benefit of administering agonists specific for key costimulatory receptors on CD8 T cells in preclinical models of RCC. In particular, agonists against CD40, CD134, and CD137 have proven effective in stimulating CD8+ T cell-mediated antitumor responses alone or in combination with immunostimulatory cytokines or chemotherapeutics.Citation9,72-74
Once CD8+ T cells have been primed and activated via antigen-loaded DC and cytokine stimulation, massive clonal expansion of the tumor antigen-specific T cells will follow. These CD8+ T cells acquire “effector” function and homing capabilities to traffic to the tumor bed where they will recognize their cognate antigen (presumably being expressed by the tumor) and subsequently carryout destruction of the tumor.Citation67,69,70,75 These effector functions include the ability to directly kill tumor cells via TRAILCitation76 or FasL mechanisms,Citation77 or to secrete cytolytic molecules such as IFN-γ, TNF-α, perforin and granzymeB,Citation77 all of which can play a role in direct tumor cell death.Citation78,79 IFN-γ and TNF-α can also have cytolytic effects on the tumor stroma and vasculature,Citation80 as well as activating effects on other immune cells that can offer help in the antitumor response. Once the tumor has been eradicated, the majority of the expanded CD8+ T cells will undergo apoptosis, leaving behind a small but effective memory population.Citation67
Natural Killer Cells
Natural killer (NK) cells are innate immune cells that have the capacity to kill cancerous cells while sparing normal cells. In humans, mature NK cells make up approximately 10% of the nucleated cells in the peripheral blood, and are identified as CD3− lymphocytes that express CD56 with or without CD16 (also called FcγRIIIA). Most (90–95%) peripheral NK (pNK) cells are CD56dim and express high levels of CD16; the remaining fraction is CD56bright CD16dim/neg 81. It was originally thought that NK cells in the blood and tissues were the same; however, recent work has revealed the existence of unique sub-populations of NK cells within uterus,Citation82 thymus,Citation83 skin,Citation84 and liver.Citation85 In these tissues, CD56bright NK cells are more abundant than CD56dim counterparts, a finding that contrasts the peripheral blood. The significance of these differences is that CD56dim NK cells have potent cytotoxic activity, while CD56bright versions have limited cytolytic responses and are more efficient producers of cytokines and chemokines.
NK cells express a number of inhibitory and activating receptors on the cell surface.Citation86,87 Activating receptors include natural cytotoxicity receptors (NCRs: NKp46, NKp30 and NKp44), C-type lectin receptors (CD94/NKG2C, NKG2D, NKG2E/H and NKG2F) and killer cell immunoglobulin-like receptors (KIRs: KIR-2DS and KIR-3DS). Inhibitory receptors comprise additional members of the C-type lectin (CD94/NKG2A/B) and KIR (KIR-2DL and KIR-3DL) receptor families. Unsolicited killing of host cells by NK cells is controlled by inhibitory receptor recognition of MHC I.88 NK cells are activated by and destroy host cells that lack or have abnormal MHC I expression. The critical role of NK cells in tumor immunosurveillance has fueled a long-standing interest in therapeutic strategies designed to stimulate antitumor responses by exploiting the natural activity of NK cells.
NK cells are the predominant lymphocyte populations in the decidua during embryo implantation. These decidua NK (dNK) cells are CD56brightCD16neg and demonstrate little-to-no cytotoxicity capacity. Interestingly, dNK cells have an increased ability to secrete cytokines and angiogenic molecules,Citation82,89-91 particularly PGF and VEGF, angiopoietins, TGFβ and, in mice, vasoactive proteins such as IFN-γ, angiotensin II receptors and atrial natriuretic peptide.Citation92,93 Accumulation of dNK cells correlates with angiogenesis, lymphangiogenesis, endometrial edema and vasodilation leading to increased uterine artery blood flow.Citation94 Furthermore, CD56bright CD16neg NK cells are critical for lessening cardiac damage by increasing angiogenesis, vascular remodeling and cardiac functionCitation95 by inducing endothelial cell proliferation.Citation96 Mediators of this phenotypic and functional differentiation in endometrium are not well known, but one inducer of pNK to dNK cell phenotypic differentiation is TGFβ.Citation97 TGFβ is highly expressed in decidua in situCitation98 and mediates conversion of pNK cells from healthy donors to dNK cells in vitro.Citation97 Thus, CD56brightCD16NKneg cells could have significant, yet under-appreciated, roles in promoting angiogenesis in a number of pathological situations, including tumor growth and metastasis.
A distinct feature of RCC is high levels of tumor infiltrating lymphocytes (TIL), and NK cells are consistently identified.Citation99 Poor survival is indicated when NK cells make up less than 20% of the total TIL population for advanced RCC tumors.Citation100-103 Other studies suggested that insufficient activation of RCC tumor NK cells contributed to therapy failure for patients treated with combinations of IL-2, IFNα, and histamine.Citation101,104,105 Even when RCC tumors are heavily infiltrated with NK cells, these tumor-infiltrating NK cells (TiNK) were unable to lyse target cell lines.Citation106 Phenotypic analysis has demonstrated that RCC TiNK cells are different compared to matched peripheral blood samples for several receptors including the cytotoxic marker CD16.Citation103,107 Specifically, cytotoxic CD56+CD16+ NK cells, resembling pNK cells, were less frequent in RCC tumors. For some RCC tumor NK cells, these changes are transient and reversed by ex vivo culture in medium containing IL-2,Citation106,108 suggesting tumor-specific alterations.Citation108
Therapeutic use of NK cells in metastatic RCC was first attempted by infusion of lymphokine activated CD3−CD56+ cells (or LAKs) in combination with IL-2109-111. Alternative approaches include adoptive transfer of unmodified or ex vivo activated allogeneic NK cellsCitation112 and NK cell lines, such as NK-92.Citation113,114 Genetically modified NK cells engineered to express silencing RNA to inhibitory receptors or overexpress cytokines, activating receptors, or chimeric antigen receptor (CAR), are being studied for potential use in the clinic.
Tumor-Mediated Immune Suppression
High levels of tumor infiltrating lymphocytes (TIL) are common to RCC suggesting that immune mechanisms have a role in the natural course of disease.Citation99 Yet, aggressive tumors, like RCC, frequently adopt characteristics to evade immune surveillance. Immune escape and immune suppression are 2 of the main obstacles that need to be overcome when considering therapies to induce antitumor immune responses.Citation115 Cancerous cells frequently downregulate MHC I to escape recognition by tumor-specific CD8+ T cells, but this still renders them susceptible to NK cell lysis.Citation116 Tumors can also induce anergy of resident naïve T cells by presenting tumor-associated antigens in the absence of co-stimulation or by secreting suppressive molecules.Citation117 Engagement of inhibitory receptors, such as CTLA-4 and PD-1, on T cells is an additional direct mechanism that tumor cells employ to suppress T cells.Citation118 CTLA-4 and PD-1 are members of the CD28 family, but instead of activating the T cells, they induce anergy, and cells within the tumor milieu can express the ligands for these receptors.Citation117 Development of reagents that specifically block the immunosuppressive nature of CTLA-4 and PD-1 has been one of the most significant advances in cancer immunotherapy, and blocking these “checkpoint inhibitors” has shown clinical efficacy in RCC.Citation119,120 Continued testing and development of these reagents will likely lead to even greater antitumor responses and improved patient survival. Tumor cells can also secrete immunosuppressive molecules such as IL-10 and TGF-β, which can inhibit proliferation and development of CTL. These molecules also likely recruit immunosuppressive cell populations into the tumor microenvironment, including the above-described dNK-like cells.
Myeloid Derived Suppressor Cells (MDSC)
Extramedullary hematopoiesis and neutrophilia were first described as characteristics of tumor progression in the early 1900s.Citation121 These events were associated with atypical myeloid progenitor generation and differentiation resulting in abnormal myeloid cells that lacked conventional B cell, T cell, and macrophage lineage markers and were capable of decreasing CTL generation and function.Citation122 In recent years these “tumor-induced” cells have been identified as myeloid derived suppressor cells (MDSC), which are present in high numbers and correlate with poor prognosis, and tumor evasion of host immunity.Citation123 MDSC are readily induced by inflammation and the presence of tumors.Citation124-127 Expansion and activation of MDSC can be induced by multiple factors made primarily by tumor/stromal cells and activated immune cells within the tumor microenvironment. These secreted factors can promote MDSC expansion by stimulating myelopoiesis, blocking differentiation of mature myeloid cells, and direct activation of MDSC. Granulocyte-macrophage colony-stimulating factor (GM-CSF), prostaglandin E2 (PGE2),Citation128 and S100ACitation129-131 proteins are examples of molecules produced by tumor cells that induce the expansion and activation of MDSC both in the periphery (bone marrow, secondary lymphoid organs) and tumor. Molecules expressed by immune cells, such as activated T cells and myeloid cells, that can induce MDSC include IFN-γCitation127, IL-6Citation124, IL-1β and cyclooxygenase (COX)-2/PGE2.Citation128,132,133
MDSC that expand during a tumor challenge are not a defined population of cells; rather, they are a heterogeneous population of immature myeloid cells.Citation134 MDSC are poorly differentiated and lack differentiation markers expressed by other myeloid cells, such as mature DC and macrophages. Murine MDSC were historically characterized by expression of CD11b and Gr-1; however, it is now well appreciated that the Gr-1 antibodies used to identify MDSC bind to 2 epitopes from 2 different GPI-anchored cell surface glycoproteins, Ly6G and Ly6C.Citation135 Using differential antibodies to each Ly6G and Ly6C, 2 sub-populations of MDSC have been identified: CD11b+Ly6C+/loLy6Ghi granulocytic MDSC, and CD11b+Ly6ChiLy6G− monocytic MDSC.Citation127,136,137 While other markers have been identified on MDSC, including F4/80, IL-4α receptor and CD115, these markers seem to be tumor model specific, and are not always constitutively expressed on MDSC. Though neutrophils and macrophages can express some of the same phenotypic markers as MDSC, MDSC specifically expand during a tumor challenge and demonstrate immunosuppressive capacities via a variety of mechanisms. Suppressive function is the main denominator that distinguishes MDSC from other myeloid cells, such as neutrophils and macrophage. MDSC have a multitude of mechanisms to inhibit the antitumor immune response, 2 of which are significant upregulation and activity of the Arg1 and iNOS enzymes.Citation138-141 These mechanisms induce non-specific T cell inhibition by catabolism of L-arginine from the surrounding environment into urea by Arg1 and nitric oxide (NO), by iNOS. Reactive oxygen species (ROS) produced by MDSC in response to cytokines have also been implemented in mediating immunosuppression. Recently, peroxynitrite has been shown to inhibit T cell activation by making them unresponsive to antigen stimulation.Citation142 Many of these byproducts have direct effects on T cells by altering the CD3 and CD8 molecules on the surface of the T cell.
Though multiple MDSC subsets exist, they have all been linked to tumor outgrowth and poor prognosis in cancer patients. For these reasons, targeted therapy to deplete or modulate MDSC is currently the focus of many antitumor therapies. Over the last few years, therapeutic targeting of MDSC to reduce immune suppression for the purpose of mounting a robust antitumor immune response has become increasingly more common. Interestingly, many of the standard treatments for cancer may decrease or modulate the MDSC population in tumor-bearing hosts. Such chemotherapies as Gemcitabine and 5-Fluoruricil selectively deplete MDSC.Citation130,143 Small molecule inhibitors for the treatment of RCC, Sorafenib and Sunitnib, have the ability to decrease the suppressive capacity of MDSC in patients.Citation64,65 These agents are capable of alleviating immune suppression through altering the MDSC populations, but they possess off target effects and toxicities that can have negative effects on subsequent immune responses. Thus, immunotherapy strategies for overcoming tumor-associated MDSC immunosuppression that do not negatively affect global immune responses are of utmost importance.
Conclusions
Treatment options for RCC patients are poorly established and predominantly limited to surgical removal of either part or the entire kidney. While effective for individuals with localized early-stage disease, the majority of newly diagnosed patients will already have advanced (metastatic) disease at the time of diagnosis. The best opportunity for long-term survival is surgery in combination with therapies designed to enhance the body's natural immune responses. Unfortunately, the most advanced approaches are lacking efficacy; complete response rates remain <20%. One potential explanation for this shortfall is that therapeutic efficacy is limited by tumor-specific factors and/or cell types that inhibit natural and/or induced antitumor immune responses (). In addition, various risk factors (such as obesity, smoking, hypertension, renal disease, and viral hepatitis) can have profound effects on normal functioning of the immune system, which impacts tumor incidence/growth.Citation144-148 These points underscore efforts to achieve a more complete understanding of the mechanisms regulating RCC tumor immune suppression. Understanding these complex interactions has broad implications for successful application of immunotherapy for RCC.
Figure 1. Role for soluble RCC tumor products in reduced function of tumor infiltrating immune cells to promote tumor outgrowth and metastasis. Peripheral blood lymphocytes are recruited to the developing renal tumor (RCC) and their activity is impaired in the tumor environment when encountering soluble products. The effect is suppression and/or loss of cytotoxic capacity and conversion to a program that favors the production of angiogenic factors and cytokines that support tumor growth and promote dissemination (metastasis) to distant organ sites.
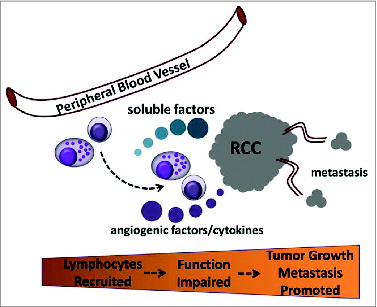
Disclosure of Potential Conflicts of Interest
No potential conflicts of interest were disclosed.
Authors' Contributions
All authors contributed to writing of the manuscript and approved the final version for submission. T.S.G. was responsible for organization of the effort.
Funding
This work was supported by grants from the National Institute of Child Health and Human Development (R15HD073868; D.S.T.) and National Cancer Institute (R15CA173657; D.S.T. and A.W.), (R01CA109446; T.S.G.). The funders had no role in preparation of the manuscript or decision to publish.
References
- Siegel RL, Miller KD, Jemal A. Cancer statistics, 2015. CA: Cancer J Clin 2015; 65:5-29; PMID:25559415; http://dx.doi.org/10.1017/S0009840X14001668
- Godley PA, Taylor M. Renal cell carcinoma. Curr Opin Oncol 2001; 13:199-203; PMID:11307065; http://dx.doi.org/10.1097/00001622-200105000-00012
- Ather MH, Masood N, Siddiqui T. Current management of advanced and metastatic renal cell carcinoma. Urology J 2010; 7:1-9
- Snow RM, Schellhammer PF. Spontaneous regression of metastatic renal cell carcinoma. Urology 1982; 20:177-81; PMID:7112827; http://dx.doi.org/10.1016/0090-4295(82)90356-9
- McDermott DF. Immunotherapy of metastatic renal cell carcinoma. Cancer 2009; 115:2298-305; PMID:19402060; http://dx.doi.org/10.1002/cncr.24236
- Dubrot J, Palazon A, Alfaro C, Azpilikueta A, Ochoa MC, Rouzaut A, Martinez-Forero I, Teijeira A, Berraondo P, Le Bon A, et al. Intratumoral injection of interferon-α and systemic delivery of agonist anti-CD137 monoclonal antibodies synergize for immunotherapy. Int J Cancer 2011; 128:105-18; PMID:20309938; http://dx.doi.org/10.1002/ijc.25333
- Hervas-Stubbs S, Perez-Gracia JL, Rouzaut A, Sanmamed MF, Le Bon A, Melero I. Direct effects of type I interferons on cells of the immune system. Clin Cancer Res 2011; 17:2619-27; PMID:21372217; http://dx.doi.org/10.1158/1078-0432.CCR-10-1114
- Rocha FG, Chaves KC, Chammas R, Peron JP, Rizzo LV, Schor N, Bellini MH. Endostatin gene therapy enhances the efficacy of IL-2 in suppressing metastatic renal cell carcinoma in mice. Cancer Immunol Immunother 2010; 59:1357-65; PMID:20490489; http://dx.doi.org/10.1007/s00262-010-0865-6
- Westwood JA, Darcy PK, Guru PM, Sharkey J, Pegram HJ, Amos SM, Smyth MJ, Kershaw MH. Three agonist antibodies in combination with high-dose IL-2 eradicate orthotopic kidney cancer in mice. J Transl Med 2010; 8:42; PMID:20426873; http://dx.doi.org/10.1186/1479-5876-8-42
- De Palma M, Mazzieri R, Politi LS, Pucci F, Zonari E, Sitia G, Mazzoleni S, Moi D, Venneri MA, Indraccolo S, et al. Tumor-targeted interferon-α delivery by Tie2-expressing monocytes inhibits tumor growth and metastasis. Cancer Cell 2008; 14:299-311; PMID:18835032; http://dx.doi.org/10.1016/j.ccr.2008.09.004
- Pyrhonen S, Salminen E, Ruutu M, Lehtonen T, Nurmi M, Tammela T, Juusela H, Rintala E, Hietanen P, Kellokumpu-Lehtinen PL. Prospective randomized trial of interferon alfa-2a plus vinblastine versus vinblastine alone in patients with advanced renal cell cancer. J Clin Oncol 1999; 17:2859-67; PMID:10561363
- Atkins MB, Sparano J, Fisher RI, Weiss GR, Margolin KA, Fink KI, Rubinstein L, Louie A, Mier JW, Gucalp R, et al. Randomized phase II trial of high-dose interleukin-2 either alone or in combination with interferon alfa-2b in advanced renal cell carcinoma. J Clin Oncol 1993; 11:661-70; PMID:8478661
- Motzer RJ, Hutson TE, Tomczak P, Michaelson MD, Bukowski RM, Oudard S, Negrier S, Szczylik C, Pili R, Bjarnason GA, et al. Overall survival and updated results for sunitinib compared with interferon alfa in patients with metastatic renal cell carcinoma. J Clin Oncol 2009; 27:3584-90; PMID:19487381; http://dx.doi.org/10.1200/JCO.2008.20.1293
- Motzer RJ, Hutson TE, Tomczak P, Michaelson MD, Bukowski RM, Rixe O, Oudard S, Negrier S, Szczylik C, Kim ST, et al. Sunitinib versus interferon alfa in metastatic renal-cell carcinoma. N Engl J Med 2007; 356:115-24; PMID:17215529; http://dx.doi.org/10.1056/NEJMoa065044
- Larkin JM, Eisen T. Renal cell carcinoma and the use of sorafenib. Ther Clin Risk Manag 2006; 2:87-98; PMID:18360584
- Mellman I, Coukos G, Dranoff G. Cancer immunotherapy comes of age. Nature 2011; 480:480-9; PMID:22193102; http://dx.doi.org/10.1038/nature10673
- Boon T, Coulie PG, Van den Eynde BJ, van der Bruggen P. Human T cell responses against melanoma. Annu Rev Immunol 2006; 24:175-208; PMID:16551247; http://dx.doi.org/10.1146/annurev.immunol.24.021605.090733
- Rohn TA, Reitz A, Paschen A, Nguyen XD, Schadendorf D, Vogt AB, Kropshofer H. A novel strategy for the discovery of MHC class II-restricted tumor antigens: identification of a melanotransferrin helper T-cell epitope. Cancer Res 2005; 65:10068-78; PMID:16267033; http://dx.doi.org/10.1158/0008-5472.CAN-05-1973
- Chiang CL, Kandalaft LE, Coukos G. Adjuvants for enhancing the immunogenicity of whole tumor cell vaccines. Int Rev Immunol 2011; 30:150-82; PMID:21557641; http://dx.doi.org/10.3109/08830185.2011.572210
- De Filippo M, Bozzetti F, Martora R, Zagaria R, Ferretti S, Macarini L, Brunese L, Rotondo A, Rossi C. Radiofrequency thermal ablation of renal tumors. Radiol Med 2014; 119:499-511; PMID:25024061; http://dx.doi.org/10.1007/s11547-014-0412-1
- Jonasch E, Gao J, Rathmell WK. Renal cell carcinoma. Bmj 2014; 349:g4797; PMID:25385470; http://dx.doi.org/10.1136/bmj.g4797
- Parashar B, Patro KC, Smith M, Arora S, Nori D, Wernicke AG. Role of radiation therapy for renal tumors. Semin Intervent Radiol 2014; 31:86-90; PMID:24596444; http://dx.doi.org/10.1055/s-0033-1363847
- Griffith TS, Ferguson TA. Cell death in the maintenance and abrogation of tolerance: the five Ws of dying cells. Immunity 2011; 35:456-66; PMID:22035838; http://dx.doi.org/10.1016/j.immuni.2011.08.011
- Ferguson TA, Stuart PM, Herndon JM, Griffith TS. Apoptosis, tolerance, and regulatory T cells–old wine, new wineskins. Immunol Rev 2003; 193:111-23; PMID:12752676; http://dx.doi.org/10.1034/j.1600-065X.2003.00042.x
- Daste A, Grellety T, Gross-Goupil M, Ravaud A. Protein kinase inhibitors in renal cell carcinoma. Expert Opin Pharmacother 2014; 15:337-51; PMID:24328606; http://dx.doi.org/10.1517/14656566.2014.869210
- Escudier B, Albiges L, Sonpavde G. Optimal management of metastatic renal cell carcinoma: current status. Drugs 2013; 73:427-38; PMID:23572408; http://dx.doi.org/10.1007/s40265-013-0043-1
- Wiley SR, Schooley K, Smolak PJ, Din WS, Huang CP, Nicholl JK, Sutherland GR, Smith TD, Rauch C, Smith CA, et al. Identification and characterization of a new member of the TNF family that induces apoptosis. Immunity 1995; 3:673-82; PMID:8777713; http://dx.doi.org/10.1016/1074-7613(95)90057-8
- Ashkenazi A, Herbst RS. To kill a tumor cell: The potential of proapoptotic receptor agonists. J Clin Invest 2008; 118:1979-90; PMID:18523647; http://dx.doi.org/10.1172/JCI34359
- Holland PM. Death receptor agonist therapies for cancer, which is the right TRAIL? Cytokine Growth Factor Rev 2014; 25:185-93; PMID:24418173; http://dx.doi.org/10.1016/j.cytogfr.2013.12.009
- Lemke J, von Karstedt S, Zinngrebe J, Walczak H. Getting TRAIL back on track for cancer therapy. Cell Death Differ 2014; 21:1350-64; PMID:24948009; http://dx.doi.org/10.1038/cdd.2014.81
- Griffith TS, Stokes B, Kucaba TA, Earel JK, Jr., VanOosten RL, Brincks EL, Norian LA. TRAIL gene therapy: from preclinical development to clinical application. Curr Gene Ther 2009; 9:9-19; PMID:19275567; http://dx.doi.org/10.2174/156652309787354612
- Holoch PA, Griffith TS. TNF-related apoptosis-inducing ligand (TRAIL): a new path to anti-cancer therapies. Eur J Pharmacol 2009; 625:63-72; PMID:19836385; http://dx.doi.org/10.1016/j.ejphar.2009.06.066
- Griffith TS, Anderson RD, Davidson BL, Williams RD, Ratliff TL. Adenoviral-mediated transfer of the TNF-related apoptosis-inducing ligand/Apo-2 ligand gene induces tumor cell apoptosis. J Immunol 2000; 165:2886-94; PMID:10946322; http://dx.doi.org/10.4049/jimmunol.165.5.2886
- Griffith TS, Broghammer EL. Suppression of tumor growth following intralesional therapy with TRAIL recombinant adenovirus. Mol Ther 2001; 4:257-66; PMID:11545617; http://dx.doi.org/10.1006/mthe.2001.0439
- Norian LA, Kresowik TP, Rosevear HM, James BR, Rosean TR, Lightfoot AJ, Kucaba TA, Schwarz C, Weydert CJ, Henry MD, et al. Eradication of metastatic renal cell carcinoma after adenovirus-encoded TNF-related apoptosis-inducing ligand (TRAIL)/CpG immunotherapy. PLoS One 2012; 7:e31085; PMID:22312440; http://dx.doi.org/10.1371/journal.pone.0031085
- VanOosten RL, Griffith TS. Activation of tumor-specific CD8+ T Cells after intratumoral Ad5-TRAIL/CpG oligodeoxynucleotide combination therapy. Cancer Res 2007; 67:11980-90; PMID:18089829; http://dx.doi.org/10.1158/0008-5472.CAN-07-1526
- Wilson NS, Yang A, Yang B, Couto S, Stern H, Gogineni A, Pitti R, Marsters S, Weimer RM, Singh M, et al. Proapoptotic activation of death receptor 5 on tumor endothelial cells disrupts the vasculature and reduces tumor growth. Cancer Cell 2012; 22:80-90; PMID:22789540; http://dx.doi.org/10.1016/j.ccr.2012.05.014
- Melief CJ. Cancer immunotherapy by dendritic cells. Immunity 2008; 29:372-83; PMID:18799145; http://dx.doi.org/10.1016/j.immuni.2008.08.004
- Albert ML, Sauter B, Bhardwaj N. Dendritic cells acquire antigen from apoptotic cells and induce class I-restricted CTLs. Nature 1998; 392:86-9; PMID:9510252; http://dx.doi.org/10.1038/32183
- Bellone M, Iezzi G, Rovere P, Galati G, Ronchetti A, Protti MP, Davoust J, Rugarli C, Manfredi AA. Processing of engulfed apoptotic bodies yields T cell epitopes. J Immunol 1997; 159:5391-9; PMID:9548479
- Blachere NE, Darnell RB, Albert ML. Apoptotic cells deliver processed antigen to dendritic cells for cross-presentation. PLoS Biol 2005; 3:e185; PMID:15839733; http://dx.doi.org/10.1371/journal.pbio.0030185
- Steinman RM. The dendritic cell system and its role in immunogenicity. Annu Rev Immunol 1991; 9:271-96; PMID:1910679; http://dx.doi.org/10.1146/annurev.iy.09.040191.001415
- Green DR, Ferguson T, Zitvogel L, Kroemer G. Immunogenic and tolerogenic cell death. Nat Rev Immunol 2009; 9:353-63; PMID:19365408; http://dx.doi.org/10.1038/nri2545
- Cowdery JS, Boerth NJ, Norian LA, Myung PS, Koretzky GA. Differential regulation of the IL-12 p40 promoter and of p40 secretion by CpG DNA and lipopolysaccharide. J Immunol 1999; 162:6770-5; PMID:10352297
- Dalod M, Salazar-Mather TP, Malmgaard L, Lewis C, Asselin-Paturel C, Briere F, Trinchieri G, Biron CA. Interferon α/β and interleukin 12 responses to viral infections: pathways regulating dendritic cell cytokine expression in vivo. J Exp Med 2002; 195:517-28; PMID:11854364; http://dx.doi.org/10.1084/jem.20011672
- Gautier G, Humbert M, Deauvieau F, Scuiller M, Hiscott J, Bates EE, Trinchieri G, Caux C, Garrone P. A type I interferon autocrine-paracrine loop is involved in Toll-like receptor-induced interleukin-12p70 secretion by dendritic cells. J Exp Med 2005; 201:1435-46; PMID:15851485; http://dx.doi.org/10.1084/jem.20041964
- Hochrein H, Shortman K, Vremec D, Scott B, Hertzog P, O'Keeffe M. Differential production of IL-12, IFN-α, and IFN-gamma by mouse dendritic cell subsets. J Immunol 2001; 166:5448-55; PMID:11313382; http://dx.doi.org/10.4049/jimmunol.166.9.5448
- James BR, Brincks EL, Kucaba TA, Boon L, Griffith TS. Effective TRAIL-based immunotherapy requires both plasmacytoid and CD8a DC. Cancer Immunol Immunother 2014; 63:685-97; PMID:24711083; http://dx.doi.org/10.1007/s00262-014-1548-5
- Liu RB, Engels B, Schreiber K, Ciszewski C, Schietinger A, Schreiber H, Jabri B. IL-15 in tumor microenvironment causes rejection of large established tumors by T cells in a noncognate T cell receptor-dependent manner. Proc Natl Acad Sci U S A 2013; 110:8158-63; PMID:23637340; http://dx.doi.org/10.1073/pnas.1301022110
- Tannenbaum CS, Tubbs R, Armstrong D, Finke JH, Bukowski RM, Hamilton TA. The CXC chemokines IP-10 and Mig are necessary for IL-12-mediated regression of the mouse RENCA tumor. J Immunol 1998; 161:927-32; PMID:9670971
- Gray RC, Kuchtey J, Harding CV. CpG-B ODNs potently induce low levels of IFN-alphabeta and induce IFN-alphabeta-dependent MHC-I cross-presentation in DCs as effectively as CpG-A and CpG-C ODNs. J Leukoc Biol 2007; 81:1075-85; PMID:17227820; http://dx.doi.org/10.1189/jlb.1006606
- Lorenzi S, Mattei F, Sistigu A, Bracci L, Spadaro F, Sanchez M, Spada M, Belardelli F, Gabriele L, Schiavoni G. Type I IFNs control antigen retention and survival of CD8alpha(+) dendritic cells after uptake of tumor apoptotic cells leading to cross-priming. J Immunol 2011; 186:5142-50; PMID:21441457; http://dx.doi.org/10.4049/jimmunol.1004163
- Lou Y, Liu C, Kim GJ, Liu YJ, Hwu P, Wang G. Plasmacytoid dendritic cells synergize with myeloid dendritic cells in the induction of antigen-specific antitumor immune responses. J Immunol 2007; 178:1534-41; PMID:17237402; http://dx.doi.org/10.4049/jimmunol.178.3.1534
- Figlin RA. A novel personalized vaccine approach in combination with targeted therapy in advanced renal cell carcinoma. Immunotherapy 2014; 6:261-8; PMID:24354908; http://dx.doi.org/10.2217/imt.13.168
- Wang J, Liao L, Tan J. Dendritic cell-based vaccination for renal cell carcinoma: challenges in clinical trials. Immunotherapy 2012; 4:1031-42; PMID:23148755; http://dx.doi.org/10.2217/imt.12.107
- Leonhartsberger N, Ramoner R, Falkensammer C, Rahm A, Gander H, Holtl L, Thurnher M. Quality of life during dendritic cell vaccination against metastatic renal cell carcinoma. Cancer Immunol Immunother 2012; 61:1407-13; PMID:22278360; http://dx.doi.org/10.1007/s00262-012-1207-7
- Draube A, Klein-Gonzalez N, Mattheus S, Brillant C, Hellmich M, Engert A, von Bergwelt-Baildon M. Dendritic cell based tumor vaccination in prostate and renal cell cancer: a systematic review and meta-analysis. PLoS One 2011; 6:e18801; PMID:21533099; http://dx.doi.org/10.1371/journal.pone.0018801
- Lion E, Smits EL, Berneman ZN, Van Tendeloo VF. NK cells: key to success of DC-based cancer vaccines? The oncologist 2012; 17:1256-70; PMID:22907975; http://dx.doi.org/10.1634/theoncologist.2011-0122
- Van Poppel H, Joniau S, Van Gool SW. Vaccine therapy in patients with renal cell carcinoma. Eur Urol 2009; 55:1333-42.
- Dannull J, Su Z, Rizzieri D, Yang BK, Coleman D, Yancey D, Zhang A, Dahm P, Chao N, Gilboa E, et al. Enhancement of vaccine-mediated antitumor immunity in cancer patients after depletion of regulatory T cells. J Clin Invest 2005; 115:3623-33; PMID:16308572; http://dx.doi.org/10.1172/JCI25947
- Poschke I, Mao Y, Adamson L, Salazar-Onfray F, Masucci G, Kiessling R. Myeloid-derived suppressor cells impair the quality of dendritic cell vaccines. Cancer Immunol Immunother 2012; 61:827-38; PMID:22080405; http://dx.doi.org/10.1007/s00262-011-1143-y
- Finke J, Ko J, Rini B, Rayman P, Ireland J, Cohen P. MDSC as a mechanism of tumor escape from sunitinib mediated anti-angiogenic therapy. Int Immunopharmacol 2011; 11:856-61; PMID:21315783; http://dx.doi.org/10.1016/j.intimp.2011.01.030
- Ko JS, Rayman P, Ireland J, Swaidani S, Li G, Bunting KD, Rini B, Finke JH, Cohen PA. Direct and differential suppression of myeloid-derived suppressor cell subsets by sunitinib is compartmentally constrained. Cancer Res 2010; 70:3526-36; PMID:20406969; http://dx.doi.org/10.1158/0008-5472.CAN-09-3278
- Ko JS, Zea AH, Rini BI, Ireland JL, Elson P, Cohen P, Golshayan A, Rayman PA, Wood L, Garcia J, et al. Sunitinib mediates reversal of myeloid-derived suppressor cell accumulation in renal cell carcinoma patients. Clin Cancer Res 2009; 15:2148-57; PMID:19276286; http://dx.doi.org/10.1158/1078-0432.CCR-08-1332
- Najjar YG, Finke JH. Clinical perspectives on targeting of myeloid derived suppressor cells in the treatment of cancer. Front Oncol 2013; 3:49; PMID:23508517; http://dx.doi.org/10.3389/fonc.2013.00049
- Gulley JL, Madan RA, Schlom J. Impact of tumour volume on the potential efficacy of therapeutic vaccines. Curr Oncol 2011; 18:e150-7; PMID:21655153; http://dx.doi.org/10.3747/co.v18i3.783
- Mescher MF, Curtsinger JM, Agarwal P, Casey KA, Gerner M, Hammerbeck CD, Popescu F, Xiao Z. Signals required for programming effector and memory development by CD8+ T cells. Immunol Rev 2006; 211:81-92; PMID:16824119; http://dx.doi.org/10.1111/j.0105-2896.2006.00382.x
- Fujii S, Liu K, Smith C, Bonito AJ, Steinman RM. The linkage of innate to adaptive immunity via maturing dendritic cells in vivo requires CD40 ligation in addition to antigen presentation and CD80/86 costimulation. J Exp Med 2004; 199:1607-18; PMID:15197224; http://dx.doi.org/10.1084/jem.20040317
- Curtsinger JM, Lins DC, Mescher MF. Signal 3 determines tolerance versus full activation of naive CD8 T cells: dissociating proliferation and development of effector function. J Exp Med 2003; 197:1141-51; PMID:12732656; http://dx.doi.org/10.1084/jem.20021910
- Curtsinger JM, Valenzuela JO, Agarwal P, Lins D, Mescher MF. Type I IFNs provide a third signal to CD8 T cells to stimulate clonal expansion and differentiation. J Immunol 2005; 174:4465-9; PMID:15814665; http://dx.doi.org/10.4049/jimmunol.174.8.4465
- Croft M. The TNF family in T cell differentiation and function–unanswered questions and future directions. Semin Immunol 2014; 26:183-90; PMID:24613728; http://dx.doi.org/10.1016/j.smim.2014.02.005
- Ju SA, Cheon SH, Park SM, Tam NQ, Kim YM, An WG, Kim BS. Eradication of established renal cell carcinoma by a combination of 5-fluorouracil and anti-4-1BB monoclonal antibody in mice. Int J Cancer 2008; 122:2784-90; PMID:18360825; http://dx.doi.org/10.1002/ijc.23457
- Sadun RE, Hsu WE, Zhang N, Nien YC, Bergfeld SA, Sabzevari H, Lutsiak ME, Khawli L, Hu P, Epstein AL. Fc-mOX40L fusion protein produces complete remission and enhanced survival in 2 murine tumor models. J Immunother 2008; 31:235-45; PMID:18317364; http://dx.doi.org/10.1097/CJI.0b013e31816a88e0
- Westwood JA, Potdevin Hunnam TC, Pegram HJ, Hicks RJ, Darcy PK, Kershaw MH. Routes of delivery for CpG and anti-CD137 for the treatment of orthotopic kidney tumors in mice. PLoS One 2014; 9:e95847; PMID:24788789; http://dx.doi.org/10.1371/journal.pone.0095847
- Spiotto MT, Rowley DA, Schreiber H. Bystander elimination of antigen loss variants in established tumors. Nat Med 2004; 10:294-8; PMID:14981514; http://dx.doi.org/10.1038/nm999
- Sayers TJ, Brooks AD, Seki N, Smyth MJ, Yagita H, Blazar BR, Malyguine AM. T cell lysis of murine renal cancer: multiple signaling pathways for cell death via Fas. J Leukoc Biol 2000; 68:81-6; PMID:10914493
- Seki N, Brooks AD, Carter CR, Back TC, Parsoneault EM, Smyth MJ, Wiltrout RH, Sayers TJ. Tumor-specific CTL kill murine renal cancer cells using both perforin and Fas ligand-mediated lysis in vitro, but cause tumor regression in vivo in the absence of perforin. J Immunol 2002; 168:3484-92; PMID:11907109; http://dx.doi.org/10.4049/jimmunol.168.7.3484
- Qin Z, Schwartzkopff J, Pradera F, Kammertoens T, Seliger B, Pircher H, Blankenstein T. A critical requirement of interferon gamma-mediated angiostasis for tumor rejection by CD8+ T cells. Cancer Res 2003; 63:4095-100; PMID:12874012
- Shresta S, Pham CT, Thomas DA, Graubert TA, Ley TJ. How do cytotoxic lymphocytes kill their targets? Curr Opin Immunol 1998; 10:581-7; PMID:9794837; http://dx.doi.org/10.1016/S0952-7915(98)80227-6
- Zhang B, Karrison T, Rowley DA, Schreiber H. IFN-gamma- and TNF-dependent bystander eradication of antigen-loss variants in established mouse cancers. J Clin Invest 2008; 118:1398-404; PMID:18317595; http://dx.doi.org/10.1172/JCI33522
- Caligiuri MA. Human natural killer cells. Blood 2008; 112:461-9; PMID:18650461; http://dx.doi.org/10.1182/blood-2007-09-077438
- Koopman LA, Kopcow HD, Rybalov B, Boyson JE, Orange JS, Schatz F, Masch R, Lockwood CJ, Schachter AD, Park PJ, et al. Human decidual natural killer cells are a unique NK cell subset with immunomodulatory potential. J Exp Med 2003; 198:1201-12; PMID:14568979; http://dx.doi.org/10.1084/jem.20030305
- Vosshenrich CA, Garcia-Ojeda ME, Samson-Villeger SI, Pasqualetto V, Enault L, Richard-Le Goff O, Corcuff E, Guy-Grand D, Rocha B, Cumano A, et al. A thymic pathway of mouse natural killer cell development characterized by expression of GATA-3 and CD127. Nat Immunol 2006; 7:1217-24; PMID:17013389; http://dx.doi.org/10.1038/ni1395
- Ebert LM, Meuter S, Moser B. Homing and function of human skin gammadelta T cells and NK cells: relevance for tumor surveillance. J Immunol 2006; 176:4331-6; PMID:16547270; http://dx.doi.org/10.4049/jimmunol.176.7.4331
- Peng H, Jiang X, Chen Y, Sojka DK, Wei H, Gao X, Sun R, Yokoyama WM, Tian Z. Liver-resident NK cells confer adaptive immunity in skin-contact inflammation. J Clin Invest 2013; 123:1444-56; PMID:23524967; http://dx.doi.org/10.1172/JCI66381
- Cerwenka A, Lanier LL. Natural killer cells, viruses and cancer. Nat Rev Immunol 2001; 1:41-9; PMID:11905813; http://dx.doi.org/10.1038/35095564
- Miller JS. The biology of natural killer cells in cancer, infection, and pregnancy. Exp Hematol 2001; 29:1157-68; PMID:11602317; http://dx.doi.org/10.1016/S0301-472X(01)00696-8
- Yokoyama WM, Kim S, French AR. The dynamic life of natural killer cells. Annu Rev Immunol 2004; 22:405-29; PMID:15032583; http://dx.doi.org/10.1146/annurev.immunol.22.012703.104711
- Lash GE, Schiessl B, Kirkley M, Innes BA, Cooper A, Searle RF, Robson SC, Bulmer JN. Expression of angiogenic growth factors by uterine natural killer cells during early pregnancy. J Leukoc Biol 2006; 80:572-80; PMID:16816146; http://dx.doi.org/10.1189/jlb.0406250
- Li XF, Charnock-Jones DS, Zhang E, Hiby S, Malik S, Day K, Licence D, Bowen JM, Gardner L, King A, et al. Angiogenic growth factor messenger ribonucleic acids in uterine natural killer cells. J Clin Endocrinol Metab 2001; 86:1823-34; PMID:11297624
- Santoni A, Carlino C, Gismondi A. Uterine NK cell development, migration and function. Reprod Biomed Online 2008; 16:202-10; PMID:18284874; http://dx.doi.org/10.1016/S1472-6483(10)60575-5
- Hatta K, Carter AL, Chen Z, Leno-Duran E, Ruiz-Ruiz C, Olivares EG, Tse MY, Pang SC, Croy BA. Expression of the vasoactive proteins AT1, AT2, and ANP by pregnancy-induced mouse uterine natural killer cells. Reprod Sci 2011; 18:383-90; PMID:20959647; http://dx.doi.org/10.1177/1933719110385136
- Zhang J, Chen Z, Smith GN, Croy BA. Natural killer cell-triggered vascular transformation: maternal care before birth? Cell Mol Immunol 2011; 8:1-11; PMID:20711229; http://dx.doi.org/10.1038/cmi.2010.38
- Quenby S, Nik H, Innes B, Lash G, Turner M, Drury J, Bulmer J. Uterine natural killer cells and angiogenesis in recurrent reproductive failure. Hum Reprod 2009; 24:45-54; PMID:18835875; http://dx.doi.org/10.1093/humrep/den348
- Ayach BB, Yoshimitsu M, Dawood F, Sun M, Arab S, Chen M, Higuchi K, Siatskas C, Lee P, Lim H, et al. Stem cell factor receptor induces progenitor and natural killer cell-mediated cardiac survival and repair after myocardial infarction. Proc Natl Acad Sci U S A 2006; 103:2304-9; PMID:16467148; http://dx.doi.org/10.1073/pnas.0510997103
- Bouchentouf M, Forner KA, Cuerquis J, Michaud V, Zheng J, Paradis P, Schiffrin EL, Galipeau J. Induction of cardiac angiogenesis requires killer cell lectin-like receptor 1 and alpha4beta7 integrin expression by NK cells. J Immunol 2010; 185:7014-25; PMID:20971926; http://dx.doi.org/10.4049/jimmunol.1001888
- Keskin DB, Allan DS, Rybalov B, Andzelm MM, Stern JN, Kopcow HD, Koopman LA, Strominger JL. TGFbeta promotes conversion of CD16+ peripheral blood NK cells into CD16- NK cells with similarities to decidual NK cells. Proc Natl Acad Sci U S A 2007; 104:3378-83; PMID:17360654; http://dx.doi.org/10.1073/pnas.0611098104
- Jones RL, Stoikos C, Findlay JK, Salamonsen LA. TGF-β superfamily expression and actions in the endometrium and placenta. Reproduction 2006; 132:217-32; PMID:16885531; http://dx.doi.org/10.1530/rep.1.01076
- Wierecky J, Muller MR, Wirths S, Halder-Oehler E, Dorfel D, Schmidt SM, Häntschel M, Brugger W, Schröder S, Horger MS, et al. Immunologic and clinical responses after vaccinations with peptide-pulsed dendritic cells in metastatic renal cancer patients. Cancer Res 2006; 66:5910-8; PMID:16740731; http://dx.doi.org/10.1158/0008-5472.CAN-05-3905
- Cozar JM, Canton J, Tallada M, Concha A, Cabrera T, Garrido F, Ruiz-Cabello Osuna F. Analysis of NK cells and chemokine receptors in tumor infiltrating CD4 T lymphocytes in human renal carcinomas. Cancer Immunol Immunother 2005; 54:858-66; PMID:15887015; http://dx.doi.org/10.1007/s00262-004-0646-1
- Donskov F, von der Maase H. Impact of immune parameters on long-term survival in metastatic renal cell carcinoma. J Clin Oncol 2006; 24:1997-2005; PMID:16648500; http://dx.doi.org/10.1200/JCO.2005.03.9594
- Kowalczyk D, Skorupski W, Kwias Z, Nowak J. Flow cytometric analysis of tumour-infiltrating lymphocytes in patients with renal cell carcinoma. Br J Urol 1997; 80:543-7; PMID:9352689; http://dx.doi.org/10.1046/j.1464-410X.1997.00408.x
- Schleypen JS, Baur N, Kammerer R, Nelson PJ, Rohrmann K, Grone EF, Hohenfellner M, Haferkamp A, Pohla H, Schendel DJ, et al. Cytotoxic markers and frequency predict functional capacity of natural killer cells infiltrating renal cell carcinoma. Clin Cancer Res 2006; 12:718-25; PMID:16467081; http://dx.doi.org/10.1158/1078-0432.CCR-05-0857
- Donskov F, Bennedsgaard KM, Von Der Maase H, Marcussen N, Fisker R, Jensen JJ, Naredi P, Hokland M. Intratumoural and peripheral blood lymphocyte subsets in patients with metastatic renal cell carcinoma undergoing interleukin-2 based immunotherapy: association to objective response and survival. Br J Cancer 2002; 87:194-201; PMID:12107842; http://dx.doi.org/10.1038/sj.bjc.6600437
- Toliou T, Stravoravdi P, Polyzonis M, Vakalikos J. Natural killer cell activation after interferon administration in patients with metastatic renal cell carcinoma: an ultrastructural and immunohistochemical study. Eur Urol 1996; 29:252-6; PMID:8647158
- Schleypen JS, Von Geldern M, Weiss EH, Kotzias N, Rohrmann K, Schendel DJ, Falk CS, Pohla H. Renal cell carcinoma-infiltrating natural killer cells express differential repertoires of activating and inhibitory receptors and are inhibited by specific HLA class I allotypes. Int J Cancer 2003; 106:905-12; PMID:12918068; http://dx.doi.org/10.1002/ijc.11321
- Frankenberger B, Noessner E, Schendel DJ. Immune suppression in renal cell carcinoma. Semin Cancer Biol 2007; 17:330-43; PMID:17656104; http://dx.doi.org/10.1016/j.semcancer.2007.06.004
- Gati A, Da Rocha S, Guerra N, Escudier B, Moretta A, Chouaib S, Angevin E, Caignard A. Analysis of the natural killer mediated immune response in metastatic renal cell carcinoma patients. Int J Cancer 2004; 109:393-401; PMID:14961578; http://dx.doi.org/10.1002/ijc.11730
- Law TM, Motzer RJ, Mazumdar M, Sell KW, Walther PJ, O'Connell M, Khan A, Vlamis V, Vogelzang NJ, Bajorin DF. Phase III randomized trial of interleukin-2 with or without lymphokine-activated killer cells in the treatment of patients with advanced renal cell carcinoma. Cancer 1995; 76:824-32; PMID:8625186; http://dx.doi.org/10.1002/1097-0142(19950901)76:5%3c824::AID-CNCR2820760517%3e3.0.CO;2-N
- Rosenberg SA, Lotze MT, Muul LM, Leitman S, Chang AE, Ettinghausen SE, Matory YL, Skibber JM, Shiloni E, Vetto JT, et al. Observations on the systemic administration of autologous lymphokine-activated killer cells and recombinant interleukin-2 to patients with metastatic cancer. N Engl J Med 1985; 313:1485-92; PMID:3903508; http://dx.doi.org/10.1056/NEJM198512053132327
- Rosenberg SA, Yang JC, Topalian SL, Schwartzentruber DJ, Weber JS, Parkinson DR, Seipp CA, Einhorn JH, White DE. Treatment of 283 consecutive patients with metastatic melanoma or renal cell cancer using high-dose bolus interleukin 2. JAMA 1994; 271:907-13; PMID:8120958; http://dx.doi.org/10.1001/jama.1994.03510360033032
- Miller JS, Soignier Y, Panoskaltsis-Mortari A, McNearney SA, Yun GH, Fautsch SK, McKenna D, Le C, Defor TE, Burns LJ, et al. Successful adoptive transfer and in vivo expansion of human haploidentical NK cells in patients with cancer. Blood 2005; 105:3051-7; PMID:15632206; http://dx.doi.org/10.1182/blood-2004-07-2974
- Klingemann HG. Natural killer cell-based immunotherapeutic strategies. Cytotherapy 2005; 7:16-22; PMID:16040380; http://dx.doi.org/10.1016/S1465-3249(05)70785-4
- Tam YK, Martinson JA, Doligosa K, Klingemann HG. Ex vivo expansion of the highly cytotoxic human natural killer-92 cell-line under current good manufacturing practice conditions for clinical adoptive cellular immunotherapy. Cytotherapy 2003; 5:259-72; PMID:12850795; http://dx.doi.org/10.1080/14653240310001523
- Drake CG, Jaffee E, Pardoll DM. Mechanisms of immune evasion by tumors. Adv Immunol 2006; 90:51-81; PMID:16730261; http://dx.doi.org/10.1016/S0065-2776(06)90002-9
- Algarra I, Cabrera T, Garrido F. The HLA crossroad in tumor immunology. Hum Immunol 2000; 61:65-73; PMID:10658979; http://dx.doi.org/10.1016/S0198-8859(99)00156-1
- Spranger S, Koblish HK, Horton B, Scherle PA, Newton R, Gajewski TF. Mechanism of tumor rejection with doublets of CTLA-4, PD-1/PD-L1, or IDO blockade involves restored IL-2 production and proliferation of CD8(+) T cells directly within the tumor microenvironment. J Immunother Cancer 2014; 2:3; PMID:24829760; http://dx.doi.org/10.1186/2051-1426-2-3
- Spranger S, Spaapen RM, Zha Y, Williams J, Meng Y, Ha TT, Gajewski TF. Up-regulation of PD-L1, IDO, and T(regs) in the melanoma tumor microenvironment is driven by CD8(+) T cells. Sci Transl Med 2013; 5:200ra116; PMID:23986400; http://dx.doi.org/10.1126/scitranslmed.3006504
- Godwin JL, Zibelman M, Plimack ER, Geynisman DM. Immune checkpoint blockade as a novel immunotherapeutic strategy for renal cell carcinoma: a review of clinical trials. Discov Med 2014; 18:341-50; PMID:25549705
- Xu KY, Wu S. Update on the treatment of metastatic clear cell and non-clear cell renal cell carcinoma. Biomark Res 2015; 3:5; PMID:25734007; http://dx.doi.org/10.1186/s40364-015-0030-7
- Sonnenfeld A. Leukamische reaktiones bei carcinoma. Zeitschrift f Klin Med 1929; 111:108
- Duwe AK, Singhal SK. The immunoregulatory role of bone marrow. I. Suppression of the induction of antibody responses to T-dependent and T-independent antigens by cells in the bone marrow. Cellular immunology 1979; 43:362-71; PMID:314345; http://dx.doi.org/10.1016/0008-8749(79)90180-1
- Serafini P, Borrello I, Bronte V. Myeloid suppressor cells in cancer: recruitment, phenotype, properties, and mechanisms of immune suppression. Semin Cancer Biol 2006; 16:53-65; PMID:16168663; http://dx.doi.org/10.1016/j.semcancer.2005.07.005
- Bunt SK, Yang L, Sinha P, Clements VK, Leips J, Ostrand-Rosenberg S. Reduced inflammation in the tumor microenvironment delays the accumulation of myeloid-derived suppressor cells and limits tumor progression. Cancer Res 2007; 67:10019-26; PMID:17942936; http://dx.doi.org/10.1158/0008-5472.CAN-07-2354
- Eruslanov E, Daurkin I, Ortiz J, Vieweg J, Kusmartsev S. Pivotal Advance: Tumor-mediated induction of myeloid-derived suppressor cells and M2-polarized macrophages by altering intracellular PGE(2) catabolism in myeloid cells. J Leukoc Biol 2010; 88:839-48; PMID:20587738; http://dx.doi.org/10.1189/jlb.1209821
- Haverkamp JM, Crist SA, Elzey BD, Cimen C, Ratliff TL. In vivo suppressive function of myeloid-derived suppressor cells is limited to the inflammatory site. Eur J Immunol 2011; 41:749-59; PMID:21287554; http://dx.doi.org/10.1002/eji.201041069
- Movahedi K, Guilliams M, Van den Bossche J, Van den Bergh R, Gysemans C, Beschin A, De Baetselier P, Van Ginderachter JA. Identification of discrete tumor-induced myeloid-derived suppressor cell subpopulations with distinct T cell-suppressive activity. Blood 2008; 111:4233-44; PMID:18272812; http://dx.doi.org/10.1182/blood-2007-07-099226
- Obermajer N, Wong JL, Edwards RP, Odunsi K, Moysich K, Kalinski P. PGE(2)-driven induction and maintenance of cancer-associated myeloid-derived suppressor cells. Immunol Invest 2012; 41:635-57; PMID:23017139; http://dx.doi.org/10.3109/08820139.2012.695417
- Cheng P, Corzo CA, Luetteke N, Yu B, Nagaraj S, Bui MM, Ortiz M, Nacken W, Sorg C, Vogl T, et al. Inhibition of dendritic cell differentiation and accumulation of myeloid-derived suppressor cells in cancer is regulated by S100A9 protein. J Exp Med 2008; 205:2235-49; PMID:18809714; http://dx.doi.org/10.1084/jem.20080132
- Geary SM, Lemke CD, Lubaroff DM, Salem AK. The combination of a low-dose chemotherapeutic agent, 5-fluorouracil, and an adenoviral tumor vaccine has a synergistic benefit on survival in a tumor model system. PLoS One 2013; 8:e67904; PMID:23840786; http://dx.doi.org/10.1371/journal.pone.0067904
- Sinha P, Okoro C, Foell D, Freeze HH, Ostrand-Rosenberg S, Srikrishna G. Proinflammatory S100 proteins regulate the accumulation of myeloid-derived suppressor cells. J Immunol 2008; 181:4666-75; PMID:18802069; http://dx.doi.org/10.4049/jimmunol.181.7.4666
- Mao Y, Poschke I, Wennerberg E, Pico de Coana Y, Egyhazi Brage S, Schultz I, Hansson J, Masucci G, Lundqvist A, Kiessling R. Melanoma-educated CD14+ cells acquire a myeloid-derived suppressor cell phenotype through COX-2-dependent mechanisms. Cancer Res 2013; 73:3877-87; PMID:23633486; http://dx.doi.org/10.1158/0008-5472.CAN-12-4115
- Serafini P. Editorial: PGE2-producing MDSC: a role in tumor progression? J Leukoc Biol 2010; 88:827-9; PMID:21041513; http://dx.doi.org/10.1189/jlb.0510303
- Gabrilovich DI, Nagaraj S. Myeloid-derived suppressor cells as regulators of the immune system. Nature Rev Immunol 2009; 9:162-74; http://dx.doi.org/10.1038/nri2506
- Fleming TJ, Fleming ML, Malek TR. Selective expression of Ly-6G on myeloid lineage cells in mouse bone marrow. RB6-8C5 mAb to granulocyte-differentiation antigen (Gr-1) detects members of the Ly-6 family. J Immunol 1993; 151:2399-408; PMID:8360469
- Elkabets M, Ribeiro VS, Dinarello CA, Ostrand-Rosenberg S, Di Santo JP, Apte RN, Vosshenrich CA. IL-1beta regulates a novel myeloid-derived suppressor cell subset that impairs NK cell development and function. Eur J Immunol 2010; 40:3347-57; PMID:21110318; http://dx.doi.org/10.1002/eji.201041037
- Youn JI, Nagaraj S, Collazo M, Gabrilovich DI. Subsets of myeloid-derived suppressor cells in tumor-bearing mice. J Immunol 2008; 181:5791-802; PMID:18832739; http://dx.doi.org/10.4049/jimmunol.181.8.5791
- Corzo CA, Cotter MJ, Cheng P, Cheng F, Kusmartsev S, Sotomayor E, Padhya T, McCaffrey TV, McCaffrey JC, Gabrilovich DI. Mechanism regulating reactive oxygen species in tumor-induced myeloid-derived suppressor cells. J Immunol 2009; 182:5693-701; PMID:19380816; http://dx.doi.org/10.4049/jimmunol.0900092
- Rodriguez PC, Ochoa AC. Arginine regulation by myeloid derived suppressor cells and tolerance in cancer: mechanisms and therapeutic perspectives. Immunol Rev 2008; 222:180-91; PMID:18364002; http://dx.doi.org/10.1111/j.1600-065X.2008.00608.x
- Rodriguez PC, Quiceno DG, Zabaleta J, Ortiz B, Zea AH, Piazuelo MB, Delgado A, Correa P, Brayer J, Sotomayor EM, et al. Arginase I production in the tumor microenvironment by mature myeloid cells inhibits T-cell receptor expression and antigen-specific T-cell responses. Cancer Res 2004; 64:5839-49; PMID:15313928; http://dx.doi.org/10.1158/0008-5472.CAN-04-0465
- Zea AH, Rodriguez PC, Atkins MB, Hernandez C, Signoretti S, Zabaleta J, McDermott D, Quiceno D, Youmans A, O'Neill A, et al. Arginase-producing myeloid suppressor cells in renal cell carcinoma patients: a mechanism of tumor evasion. Cancer Res 2005; 65:3044-8; PMID:15833831
- Raber PL, Thevenot P, Sierra R, Wyczechowska D, Halle D, Ramirez ME, Ochoa AC, Fletcher M, Velasco C, Wilk A, et al. Subpopulations of myeloid-derived suppressor cells impair T cell responses through independent nitric oxide-related pathways. Int J Cancer 2014; 134:2853-64; PMID:24259296; http://dx.doi.org/10.1002/ijc.28622
- Qu X, Felder MA, Perez Horta Z, Sondel PM, Rakhmilevich AL. Antitumor effects of anti-CD40/CpG immunotherapy combined with gemcitabine or 5-fluorouracil chemotherapy in the B16 melanoma model. Int Immunopharmacol 2013; 17:1141-7; PMID:24201083; http://dx.doi.org/10.1016/j.intimp.2013.10.019
- Macleod LC, Hotaling JM, Wright JL, Davenport MT, Gore JL, Harper J, White E. Risk factors for renal cell carcinoma in the VITAL study. J Urol 2013; 190:1657-61; PMID:23665301; http://dx.doi.org/10.1016/j.juro.2013.04.130
- Hjartaker A, Langseth H, Weiderpass E. Obesity and diabetes epidemics: cancer repercussions. Adv Exp Med Biol 2008; 630:72-93; PMID:18637486; http://dx.doi.org/10.1007/978-0-387-78818-0_6
- Laber DA. Risk factors, classification, and staging of renal cell cancer. Med Oncol 2006; 23:443-54; PMID:17303902; http://dx.doi.org/10.1385/MO:23:4:443
- James BR, Anderson KG, Brincks EL, Kucaba TA, Norian LA, Masopust D, Griffith TS. CpG-mediated modulation of MDSC contributes to the efficacy of Ad5-TRAIL therapy against renal cell carcinoma. Cancer Immunol Immunother 2014; 63:1213-27; PMID:25143233; http://dx.doi.org/10.1007/s00262-014-1598-8
- James BR, Tomanek-Chalkley A, Askeland EJ, Kucaba T, Griffith TS, Norian LA. Diet-induced obesity alters dendritic cell function in the presence and absence of tumor growth. J Immunol 2012; 189:1311-21; PMID:22745381; http://dx.doi.org/10.4049/jimmunol.1100587