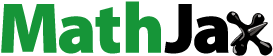
Abstract
Oral vaccination is the preferred route of immunization. However, the degradative condition of the gastrointestinal tract and the higher molecular size of peptides pose major challenges in developing an effective oral vaccination system. One of the most excellent methods used in the development of oral vaccine delivery system relies on the entrapment of the antigen in polymeric nanoparticles. In this work, trimethyl chitosan (TMC) nanoparticles were fabricated using ionic gelation teqnique by interaction hydroxypropyl methylcellulose phthalate (HPMCP), a pH-sensitive polymer, with TMC and the utility of the particles in the oral delivery of hepatitis B surface antigen (HBsAg) was evaluated employing solutions that simulated gastric and intestinal conditions. The particle size, morphology, zeta potential, loading capacity, loading efficiency, in vitro release behavior, structure, and morphology of nanoparticles were evaluated, and the activity of the loaded antigen was assessed. Size of the optimized TMC/HPMCP nanoparticles and that of the antigen-loaded nanoparticles were 85 nm and 158 nm, respectively. Optimum loading capacity (76.75%) and loading efficiency (86.29%) were achieved at 300 µg/mL concentration of the antigen. SEM images revealed a spherical shape as well as a smooth and near-homogenous surface of nanoparticles. Results of the in vitro release studies showed that formulation with HPMCP improved the acid stability of the TMC nanoparticles as well as their capability to preserve the loaded HBsAg from gastric destruction. The antigen showed good activity both before and after loading. The results suggest that TMC/HPMCP nanoparticles could be used in the oral delivery of HBsAg vaccine.
Abbreviations
TMC | = | Trimethyl chitosan |
HPMCP | = | Hydroxypropyl methylcellulose phthalate |
HBsAg | = | Hepatitis B surface antigen |
mL | = | Milliliter |
SEM | = | scanning electron microscopy |
HB | = | Hepatitis B |
PDI | = | Polydispersity index |
µg | = | Microgram |
mV | = | Millivolt |
SD | = | Standard Deviation |
n | = | number |
Introduction
Infectious disease of hepatitis B (HB) is a worldwide social health complication, particularly in developing countries where approximately 90% of the total number of HBV-infected patients reside.Citation1 Chronic HB disease induces persistent hepatitis, liver cirrhosis, and hepatic malignancy. Approximately 30% of the world's population has been infected with HBV and 350 million are persistent carriers.Citation2,3 At present, no effective therapy exists for HBV infection. Therefore, preventive vaccination continues to be the most cost-effective solution for HBV-related disease control.Citation4,5
Currently, HBV vaccination requires 3 administrations (0, 1, and 6 months) for eliciting effective responses. However, completion rate of this standard immunization protocols among adolescents is as low as 11% due to the poor compliance of the multi-dose protocol.Citation5 This problem is more severe in developing countries, which generally lack permanent clinics and healthcare professionals to effectively implement the protocol. This, in turn, exacerbates the already poor vaccination coverage in such areas.Citation4 Development of single-dose vaccine formulations could alleviate this problem by eliminating the need for follow-up visits to clinics. However, the development of hepatitis B surface antigen (HBsAg) vaccine as a single-dose form with programmed-release profiles, which can stimulate the immune system equipotential to the 3 doses of a common adjuvant (aluminum hydroxide) vaccine, is a challenging task.Citation6
Oral vaccine delivery is advised to be the most advantageous and comfortable way of vaccination, since it eliminates the local side effects created via the repetitive injection. Vaccines have inherently low absorption from the intestinal tract due to their hydrophilicity and large molecular size. Additionally, following oral administration, vaccines are extremely nonresistant to enzymatic deterioration in the stomach and intestinal environment.Citation7 Once entrapped in suitable carrier nanoparticles, antigens will be protected versus the degradative condition of the GI environment prior to their absorption in their intact form. Furthermore, using mucoadhesive polymers for the preparation of the delivery vehicle lengthens the association of the particles to the surface of mucosa while achieving the greater regional concentration of antigen in the mucus bed and minimizing dilution and destruction of antigen by the intestinal lumen contents.Citation7,8
Chitosan polymer is derived from chitin by deacetylation. It is widely investigated for applications in pharmaceutical domains. However, limited solubility has prevented its widespread use.Citation9,10 The mucoadhesive and absorption-promoting properties of chitosan have been extensively evaluated. The positive charge gained in acidic pH conditions, in which chitosan has high solubility, appears to be effective for enhanced absorption.Citation11 With due attention to very low solubility of chitosan above pH 6.5, its application is limited to absorption facilitator in the body. Therefore, chitosan derivatives with enhanced solubility at basic and neutral pH are essential.Citation12-15 It has been shown that TMC, a quaternary derivative of chitosan, enhances penetration and opens epithelial tight junctions in the environments with basic and neutral pH.Citation14
The gastric enzymes and acidic conditions pose challenges to the oral administration of vaccines. In the present study, hydroxypropyl methyl cellulose phthalate (HPMCP) was used as an enteric coating to preserve the vaccines from being destroyed by gastric acids and to prevent gastrointestinal side effects.Citation16,17 HPMCP is used in the formulation of enteric coated pharmaceutical products to protect drug ingredients from destruction by stomach acidic contents. The HPMCP free carboxyl groups have the pKa of about 5.2.Citation7,8 The charge of free carboxylic acid groups of this polymer is converted into negative at pH values of higher than 5.2 and serves as the enforcement for the electrostatic bond with TMC.Citation8
The objectives of the present work was to prepare TMC/HPMCP nanoparticles using the ionic gelation technique by cross-linking pH-sensitive HPMCP polymer with TMC and to evaluate the utility of the nanoparticles in the oral delivery of HBsAg. In order to reach optimized nanoparticles, nanoparticles with acceptable characteristics, size of the particles, morphology, zeta potential, loading capacity, and loading efficiency of the nanoparticles were analyzed and the in vitro release profile and biological activity of the released antigen were evaluated.
Results
NMR characterization of TMC and degree of quaternization
NMR spectroscopy was used for determining the degree of quaternization (percent of trimethylated amine groups) of the synthesized TMC. This polymer's 1H-NMR spectra is demonstrated in . The peak at 3.3 ppm belonged to the N(CH3)3 group and a smaller signal at 5.2 ppm owning to the N(CH3)2 group. The degree of quaternization was estimated to be 50.95 ± 9.85% using the peak appointment and intensity.
Particle size and zeta potential
In the present study, to find optimum parameters, the effect of different TMC concentrations (1, 1.5, and 2 mg/mL) on nanoparticle formation was evaluated using the Zetasizer. As shown in , 1 mg/mL of TMC and HPMCP was observed as the suitable concentration for preparing optimized nanoparticles. On the other hand, effects of HBsAg's initial concentration (100, 300, 500, 700, and 1000 µg/mL) were evaluated on the characteristics of HBsAg-loaded nanoparticles at the optimized HPMCP and TMC concentration (1 mg/mL) (). The average particle size of the optimized empty and HBsAg-loaded TMC/HPMCP nanoparticles was approximately 85 nm and 158 nm, respectively. The PDI of TMC/HPMCP nanoparticles was 0.4 and that of the HBsAg-loaded nanoparticles was 0.3, which indicated narrow and favorable particle size distribution (PDI < 0 .5). The present results demonstrated that, in optimum conditions, the zeta potentials of empty and HBsAg-loaded TMC/HPMCP nanoparticles were +30.1 ± 0.8 mV and +20 ± 0.7 mV, respectively. These results suggested that the loading of HBsAg led to a reduction in the zeta potential of the particles.
Table 1. Formulations and properties of TMC/HPMCP nanoparticles, n = 3
Table 2. Formulations and characteristics of HBsAg-loaded TMC/HPMCP nanoparticles, n = 3
Effect of HBsAg concentration on loading capacity and loading efficiency
Effect of HBsAg's initial concentration (100, 300, 500, 700, and 1000 µg/mL) on loading efficiency and capacity was analyzed in optimized conditions (1 mg/mL concentration of TMC and HPMCP) for preparing empty nanoparticles. As shown in , optimum loading capacity (76.75%) and efficiency (86.29%) were obtained at 300 µg/mL initial concentration of the antigen.
FTIR spectroscopy
The FTIR spectra of TMC, TMC/HPMCP nanoparticles, and HBsAg-loaded TMC/HPMCP nanoparticles are shown in , respectively. The FTIR spectrum of TMC showed evidence of methylation, particularly in the 1,700–1,200 cm−1 region. A new peak appeared at the high wave number, 1631cm−1, which was attributed to the quaternary ammonium salt. The strong, sharp peaks in 3450 cm−1 region and a peak at 2923 cm−1 were also observed.
Figure 3. FTIR spectrum of (A) trimethyl chitosan, (B) TMC/ HPMCP) nanoparticle and (C) TMC/ HPMCP nanoparticle containing HBs Ag (TMC 1 mg/ml, HPMCP 1 mg/ml, HBs Ag 300 µg/ml).
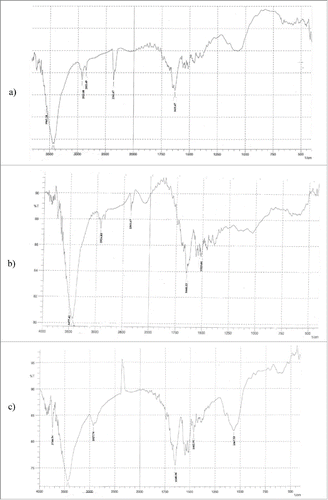
The FT-IR spectra of TMC/HPMCP nanoparticles () was unlike those of the TMC polymer (). In the spectrum of TMC/HPMCP nanoparticles, the tip of the peak at 3450 cm−1 was wider, which indicated enhanced hydrogen bonding. A new peak represented at 1735 cm−1 was attributed to C=O stretching. The FT-IR spectra were consistent with the modification of TMC film by HPMCP.
shows the FTIR spectrum of HBsAg-loaded TMC/HPMCP nanoparticles. The spectrum showed the characteristic peaks of the substituted secondary amide in the 3300–3400 cm−1 region. The peaks at 1145 cm−1 and 600 cm−1 were attributed to the C-S stretching vibrations of sulfides and disulfides.
Morphology
demonstrate representative scanning electron micrographs of empty and HBsAg-loaded TMC/HPMCP nanoparticles, respectively. These particles were spherical and had a smooth surface.
In vitro antigen release
The profiles of antigen release, under simulated intestinal (pH 6.8) and stomach (pH 2) environments, from HBsAg-loaded TMC/HPMCP nanoparticles are shown in . The amount of the antigen released from the nanoparticles after 6 h of incubation at pH 2 did not exceed 25% of the loaded protein. The particles showed good physical stability in gastric pH conditions and their size did not noticeably change. The present results showed that, under simulated intestinal conditions, 70% of the loaded antigen was released after 10 h.
Reactivity of HBsAg before and after loading
The antigen reactivity before and after loading was evaluated by Ouchterlony double immunodiffusion test (). Sample of HBsAg before entrapment was showed sharp antigen-antibody precipitin line, but HBsAg released in gastric simulated condition and PBS did not demonstrate antigen antibody reaction (). The ability of the TMC/HPMCP nanoparticles in terms of preserving the loaded HBsAg from the degradative gastric conditions is shown in . The results showed good precipitin lines and antigen-antibody reactivity for the samples before loading, antigen released in intestinal simulated condition, and antigen released in intestinal simulated condition after treatment in gastric simulated condition ().
Figure 6. Double immunodiffusion test images of antigen reactivity: (A): before loading (B), after release in simulated gastric condition (C) and PBS (P), (B): before loading (B), after release in simulated intestinal ( D), release in intestinal simulated condition after treatment in gastric simulated condition (E), [Anti-HBs Ag (A), HBs Ag (B)].
![Figure 6. Double immunodiffusion test images of antigen reactivity: (A): before loading (B), after release in simulated gastric condition (C) and PBS (P), (B): before loading (B), after release in simulated intestinal ( D), release in intestinal simulated condition after treatment in gastric simulated condition (E), [Anti-HBs Ag (A), HBs Ag (B)].](/cms/asset/5ec94eec-c1eb-4136-bf09-848120c10632/khvi_a_1053663_f0006_oc.gif)
Discussion
TMC-based nano- and micro-particles have been broadly studied for their use in mucosal vaccination.Citation8 TMC particles prepared via chemical cross-linking can be potentially toxic and the nanoparticles could also chemically modify the entrapped proteins.Citation15 Therefore, physical techniques, similar to spray drying and ionic cross-linking, are considered relatively more suitable and conservative for protein. TMC nano- and micro-particles have been fabricated by ionic cross-linking with different polyanions, such as polyglutamic acid, TPP, sodium sulfate, dextran sulfate, and anionic cyclodextrins.Citation18 The acidic environment of the stomach and enzymes pose significant challenges to the oral administration of vaccines. Therefore, in this study, HPMCP was used as the enteric coating agent for protecting HBs antigen from degradation by stomach acids.
As shown in , using the peak appointment and intensity, degree of quaternization was estimated to be be about 51%. The present results indicated that TMC was successfully synthesized and can be used for the preparation of nanoparticles.
In this work, the effect of different TMC concentrations (1, 1.5, and 2 mg/mL) on nanoparticle formation was assessed using the Zetasizer. The obtained results showed that, at constant HPMCP concentration (1 mg/mL), size of the nanoparticles increased with TMC concentration (). It appears that enhancing the TMC concentration increased the viscosity of the solution, which led to increased resistance against dispersion in the liquid phase and formation of larger particles. In the epithelial cells, smaller particles could be absorbed faster than the larger particles.
Results of the study on the influence of various initial concentrations of HBsAg on the characteristics of HBsAg-loaded nanoparticles are shown in . Accordingly, at constant HPMCP and TMC concentration (1 mg/mL), enhanced antigen concentration led to increased size and PDI of the HBsAg-entrapped nanoparticles. In this study, the optimal size of the empty nanoparticles (85 nm) and HBsAg-loaded nanoparticles (158 nm) was obtained at 1 mg/mL concentrations of both TMC and HPMCP.
The PDI of optimized TMC/HPMCP nanoparticles was 0.4 and that of the HBsAg-loaded nanoparticles was 0.3, which indicated a narrow and favorable particle size distribution (PDI < 0.5). The present results demonstrated that, in optimum conditions, the zeta potentials of empty and HBsAg-loaded TMC/HPMCP nanoparticles were +30.1 ± 0.8 mV and +20 ± 0.7 mV, respectively.
The data obtained using the Zetasizer revealed that the HBsAg-loaded TMC/HPMCP nanoparticles were larger than their empty counterparts, probably as a result of the high molecular weight and large size of the HBsAg molecules, HBsAg surface adsorption during the incubation, and negligible increase in the viscosity by HBsAg during the loading process. The zeta potential of HBsAg-loaded TMC/HPMCP nanoparticles can extremely affect their stability in suspension by electrostatic repulsion among the particles.Citation19,8 Present results demonstrated that the zeta potentials of empty and HBsAg-loaded TMC/HPMCP nanoparticles were +30.1 ± 0.8 mV and +20 ± 0.7 mV, respectively. These results suggested that the loading of HBsAg led to a reduction in the zeta potential of the particle. Because HBsAg isoelectric point is approximately 4.5, in the solutions with pH of higher than 4.5, antigen had negative charge. Therefore, encapsulation of HBsAg led to decreased zeta potential of the nanoparticles.
Effect of various HBsAg initial concentrations on loading efficiency and capacity was analyzed. The optimum loading capacity (76.75%) and efficiency (86.29%) were obtained at 300 µg/mL initial concentration of the antigen (). The high antigen loading of TMC/HPMCP nanoparticles was probably resulted from the electrostatic interactions between the antigen and TMC. Additionally, HPMCP containing chains of high molecular weight (45 kDa) could contribute to effective interactions with TMC. Such three-dimensional structures could facilitate antigen loading in nanoparticles.Citation8 The higher loading capacity is important advantage for antigen delivery systems, for the reason that by use of small quantity of antigen entrapped nanoparticle with high loading capacity great amount of antigen can be deliver to body.
The FTIR spectra of TMC was the evidence of methylation, particularly in the 1,700–1,200 cm−1 region. A new peak appeared at a high wave number, 1631cm−1, which was attributed to the quaternary ammonium salt. The strong, sharp peaks in 3450 cm−1 region were attributed to the axial stretching vibrations of O–H and N–H bonds and the peak at 2923 cm−1 was attributed to the axial stretching of C–H bonds.Citation20 The FT-IR spectrum of TMC/HPMCP nanoparticles () was different from that of the TMC matrix (). In the spectrum of TMC/HPMCP nanoparticles, the tip of the peak at 3450 cm−1 was wider, which indicated enhanced hydrogen bonding. A new peak that became apparent at 1735 cm−1 was attributed to C=O stretching. The FT-IR spectra were consistent with the modification of TMC film by HPMCP. The modification could be related to the formation of linkage among negatively charged carboxylic groups and positively charged ammonium ions.Citation21 The FTIR spectrum of HBsAg-loaded TMC/HPMCP nanoparticles () showed characteristic peaks of the substituted secondary amide in the 3300–3400 cm−1 region. The peaks at 1145 cm−1 and 600 cm−1 were attributed to C-S stretching vibrations of sulfides and disulfides. The FTIR results confirmed the encapsulation of HBsAg in nanoparticles.
The profiles of antigen release, under simulated intestinal (pH 6.8) and stomach (pH 2) environments, from HBsAg-loaded TMC/HPMCP nanoparticles () showed that the amount of the antigen released from the nanoparticles after 6 h of incubation at pH 2 did not exceed 25% of the loaded protein. The particles demonstrated good physical stability in gastric pH conditions and their size did not noticeably change. Considering HPMCP insolubility in acidic environments, physical association with insoluble HPMCP polymeric construction could probably reduce the solubility of TMC at lower pH values. Therefore, antigen release from TMC/HPMCP nanoparticles at pH 2 likely could involve the mechanism of diffusion, rather than corrosion or destruction of the polymeric matrix. Similar results have been obtained for chitosan/alginatesCitation22 and chitosan/HPMCP.Citation8 The results showed that, under simulated intestinal conditions, 70% of the loaded antigen was released after 10 h. The TMC/HPMCP nanoparticles were stable at acidic pH and rapidly dissolved in simulated intestinal environment. Such fast solubility of TMC matrix could justify the facile release of the loaded antigen at pH 6.8.
The results showed good and sharp precipitin lines and antigen-antibody reactivity for the samples before loading, antigen released in intestinal simulated condition, and antigen released in intestinal simulated condition after treatment in gastric simulated condition. Also, HBsAg released in gastric simulated condition did not show any antigen antibody reaction (). It could be suggested that TMC/HPMCP nanoparticles had protective ability for entrapped HBsAg against degradation by gastric simulated condition. These results were consistent with that reported for papain,Citation21 insulin,Citation8,23 cyclosporine A, calcitonin, and somatostatin.Citation24
In this study, a novel TMC/HPMCP nanoparticle system was successfully developed for oral administration of HBsAg. Oral administration is a favorite method of drug delivery. Advantages of oral delivery of vaccines include better management of vaccination, greater compliance, elimination of complications associated with local injection, cost-effective manufacturing, and affordability. However, the challenges faced while developing suitable oral vaccine delivery systems are significant. The present results showed that formulation with HPMCP significantly improved the stability of TMC nanoparticles in the acidic condition and their potentiality in terms of preserving the loaded antigen from gastric destruction. The present results clearly showed that pH-sensitive TMC nanoparticles are favorite delivery vehicles that could load and preserve HBsAg from degradation. Therefore, HBsAg-loaded TMC/HPMCP nanoparticles could be used as an alternative vaccine delivery and adjuvant system to the conventional parental dosage form. Further in vivo studies are underway for advancing the development of this vaccine.
Materials and Methods
Synthesis of TMC
Concisely, polymer of chitosan was dispersed in NMP. Then, sodium hydroxide solution, sodium iodide, and methyl iodide were added to the dispersion. The mixture was stirred at 60°C for 6 h. The polymer thus obtained was precipitated with acetone and dried at room temperature to yield a water-soluble white powder. The dried products were then solubilized in 20 mL of 5% (w/v) sodium chloride solution and stirred for 1 h to replace the iodide with chloride ion. Then, the polymer was precipitated, washed twice with acetone, and dried at room temperature.Citation15,16
Characterizing TMC by 1H-NMR spectroscopy
The TMC synthesized in this study was characterized by 1H-nuclear magnetic resonance (1H-NMR) spectroscopy. The NMR spectra of the solution of 5 mg of TMC in 700 μL of D2O (dissolved by heating at 80°C) were acquired. The peak corresponding to water was suppressed. The degree of quaternization (DQ) was estimated by EquationEquation (1)(1)
(1) .Citation17
(1)
(1)
where DQ (%) is the degree of quaternization expressed as percent, [(CH3)3] is the integral of the chemical shift of the trimethylamino group at 3.3 ppm, which is attributed to the 9 hydrogen atoms of the methyl groups of the trimethylamino group, and [H] is the integral of the 1H peaks between 4.7 and 5.7 ppm (reference signals), which is due to the protons attached to the carbon atom of the glucosamine unit of the glucopyranose ring.Citation17,25,26
Preparing TMC/HPMCP nanoparticles and HBsAg-loaded nanoparticles
The nanoparticles were fabricated by the ionic gelation of the positively charged TMC with the negatively charged pH-sensitive polymer HPMCP. TMC solutions of various concentrations (1, 1.5, and 2 mg/mL) were prepared. For preparing the nanoparticles, an HPMCP aqueous solution (1 mg/mL) was combined with TMC (1 mg/mL) aqueous solution slowly under constant shaking (1000 rpm, 1 h) at room temperature. Once an opalescent suspension was created, the samples were centrifuged at 15000 rpm for 30 min at 4°Ċ and the precipitate was lyophilized and weighed.Citation8 In the first step, the effects of the initial concentrations of TMC (1, 1.5, and 2 mg/ml) on the characteristics of nanoparticles were studied in terms of access to the optimum concentration of TMC for synthesising TMC/HPMCP nanoparticles.
In the second step, HBsAg-entrapped nanoparticles were fabricated by adding the HPMCP solution to the TMC solution containing different concentrations of HBsAg in optimized conditions in order to synthesize empty TMC/HPMCP. The effects of the initial concentrations of HBsAg (100, 300,500, 700, and 1000 µg/mL) on the characteristics of HBsAg-entrapped TMC/HPMCP nanoparticles were also examined. In all the steps of this work, while studying the effect of one parameter, the other parameters were kept constant.Citation27
Physicochemical properties of empty and HBsAg-loaded TMC/HPMCP nanoparticles
Structure of nanoparticles
The IR spectrum of the samples was prepared on a Fourier transform infrared spectrophotometer (FTIR- 410, Jasco Colchester, United Kingdom) with attenuated total reflection (ATR) using KBr pellets.
Particle size and zeta potential
The mean size of the particles, size distribution [polydispersity index (PDI)], and the zeta potential of the particles were determined by Zetasizer (Malvern Instruments, UK).
Morphology
The morphologies of the nanoparticles were analyzed using a scanning electron microscope (SEM) (Hitachi S- 4160, Japan) after coating with a thin layer of platinum.
HBsAg loading efficiency and loading capacity of nanoparticles
HBsAg-entrapped nanoparticles were isolated from aqueous suspension using centrifuge at 65,000g and 4°C for 30 min. The protein content (free HBsAg) supernatant was analyzed by the Bradford protein assay.Citation28,29 The HBsAg loading efficiency (LE) and loading capacity (LC) of the nanoparticles were estimated as follows:
where A is the total amount of HBsAg, B is the free amount of HBsAg, and C is the weight of nanoparticles.Citation30
In vitro antigen release
For the in vitro antigen release assessment, a known quantity of HBsAg-entrapped TMC/HPMCP nanoparticles was suspended in separate tubes containing equal volumes of 0.2 mol/L PBS (pH 7.4, simulating intestinal conditions) and HCl solution (pH 2, simulating gastric conditions). Then, the tubes were incubated at 37°C under constant shaking. One tube was removed at specific intervals (15, 30, 60, 90, 120, 240, 360, 1440, and 1800 min) and centrifuged at 65,000g and 4°C for 30 min. The amount of HBsAg released into the supernatant was then measured.Citation30
Reactivity of the HBsAg before and after loading
The antigen reactivity (before and after loading) was evaluated by the Ouchterlony double immunodiffusion test. For this purpose, after preparing agar gel plate, the reactivity of the antigen before loading and that of the antigen released into the solutions that simulated gastric, intestinal after gastric incubation stage and intestinal conditions was determined. The precipitin lines were visualized after staining with Coomassie brilliant blue solution.Citation31,32
Stability of the entrapped HBsAg in TMC/HPMCP nanoparticles against simulated gastric condition was investigated to evaluate the protective ability of the prepared nanoparticles against gastric degradation. The precipitate of the sample of in vitro release study in gastric simulated condition (after 360 min) was suspended in the solution of intestinal simulated condition. Then, the suspension was incubated at 37°C under constant shaking for 240 min, centrifuged ( 65,000g, 4°C for 30 min), and the amount of HBsAg released into the supernatant was measured. Sample of the supernatant was used for antigen reactivity experiment.
Disclosure of Potential Conflicts of Interest
No potential conflicts of interest were disclosed.
Acknowledgments
We would like to extend our thanks to Mrs Marziyeh Bayat from Masoondarou Company, Dr Mohammad Azizi and Dr Alireza Khabiri from Pasteur Institute of Iran for their kindly support through this study.
Funding
This research was financially supported by Masoondarou Biopharmaceutical Company.
References
- Franco E, Bagnato B, Marino MG, Meleleo C, Serino L, Zaratti L. Hepatitis B: Epidemiology and prevention in developing countries. World J Hepatol 2012; 4(3):74-80.
- Liaw YF, Kao JH, Piratvisuth T, Henry L, Chien RN, Liu CJ, Gane Ed, Stephen L, Lim SG, Han KH, et al. Asian-Pacific consensus statement on the management of chronic hepatitis B: a 2012 update. Hepatol Int 2012; 6(3):531-61; PMID:19669255; http://dx.doi.org/10.1007/s12072-012-9365-4.
- El-Serag HB. Epidemiology of viral hepatitis and hepatocellular carcinoma. Gastroenterology 2012; 142(6):1264-73; PMID:22537432; http://dx.doi.org/10.1053/j.gastro.2011.12.061.
- Zidan A, Scheuerlein H, Schüle S, Settmacher U, Rauchfuss F. Epidemiological pattern of hepatitis B and hepatitis C as etiological agents for hepatocellular carcinoma in iran and worldwide. Hepat Mon 2012: 12(10 HCC):e6894; PMID:23233864; http://dx.doi.org/10.5812/hepatmon.6894.
- European Association for the Study of the Liver. EASL clinical practice guidelines: management of chronic hepatitis B virus infection. J Hepatol 2012; 57(1):167-85; PMID:22436845; http://dx.doi.org/10.1016/j.jhep.2012.02.010.
- Hayden CA, Streatfield SJ, Lamphear BJ, Fake GM, Keener TK, Walker JH, Clements JD, Turner DD, Tizard IR, Howard JA, Bioencapsulation of the hepatitis B surface antigen and its use as an effective oral immunogen. Vaccine 2012; 30(19):2937-42; PMID:22406456; http://dx.doi.org/10.1016/j.vaccine.2012.02.072.
- Khafagy ES, Morishita M, Onuki Y, Takayama K. Current challenges in non-invasive insulin delivery systems: a comparative review. Adv Drug Deliv 2007; 59:1521-46; http://dx.doi.org/10.1016/j.addr.2007.08.019.
- Makhlof A, Tozuka U, Takeuchi H. Design and evaluation of novel pH-sensitive chitosan nanoparticles for oral insulin delivery. Eur J Pharm Sci 2011; 42:445-51; PMID:21182939; http://dx.doi.org/10.1016/j.ejps.2010.12.007.
- Amidi M, Mastrobattista E, Jiskoot W, Hennink WE. Chitosan-based deliver systems for protein therapeutics and antigens. Adv Drug Deliv Rev 2012; 62:59-82; PMID:19925837; http://dx.doi.org/10.1016/j.addr.2009.11.009.
- Jintapattanakit A., Junyaprasert V, Mao S, Sitterberg J, Bakowsky U, Kissel T. Peroral delivery of insulin using chitosan derivatives. A comparative study of polyelectrolyte nanocomplexes and nanoparticles. Int J Pharm 2007; 342:240-9; PMID:17597316; http://dx.doi.org/10.1016/j.ijpharm.2007.05.015.
- Mao S, Bakowsky U, Jintapattanakit A, Kissel T. Self-assembled polyelectrolyte nanocomplexes between chitosan derivatives an insulin. J Pharm Sci 2006; 95:1035-48; PMID:16565978; http://dx.doi.org/10.1002/jps.20520.
- Bayat A, Dorkoosh FA, Dehpour AR, Moezi L, Larijani B, Junginger HE, Rafiee-Tehrani M. Nanoparticles of quaternized chitosan derivatives as a carrier for colon delivery of insulin: ex vivo and in vivo studies. Int J Pharm 2008; 356:259-66; PMID:18289808; http://dx.doi.org/10.1016/j.ijpharm.2007.12.037.
- Philip AK, Philip B. Colon targeted drug delivery systems: a review on primary and novel approaches. Oman Med J 2010; 25(2):79-87; PMID:22125706; http://dx.doi.org/10.5001/omj.2010.24.
- Kokubo H, Obara S, Minemura K, Tanaka T. Development of cellulose derivatives as novel enteric coating agents soluble at pH 3.5–4.5 and higher. Chem Pharm Bull 1997; 45:1350-3; PMID:9301034; http://dx.doi.org/10.1248/cpb.45.1350.
- van der Merwe SM, Verhoef JC, Verheijden JHM, Kotze´ AF, Junginger HE. Trimethylated chitosan as polymeric absorption enhancer for improved peroral delivery of peptide drugs. Eur J Pharm Biopharm 2004; 58:225-35; PMID:15296951; http://dx.doi.org/10.1016/j.ejpb.2004.03.023.
- Thanou M, Verhoef JC, Romeijn SG, Nagelkerke JF, Merkus FWHM, Junginger HE. Effects of N-trimethyl chitosan chloride, a novel absorption enhancer, on Caco-2 intestinal epithelia and the ciliary beat frequency of chicken embryo trachea. Int J Pharm 1999; 185:73-82; PMID:10425367; http://dx.doi.org/10.1016/S0378-5173(99)00126-X.
- Snyman D, Hamman JH, Kotze´ AF, Evaluation of the mucoadhesive properties of N-trimethyl chitosan chloride. Drug Dev Ind Pharm. 2003; 29:59-67; http://dx.doi.org/10.1081/DDC-120016684.
- Amidi M, Mastrobattista E, Jiskoot W, Hennink WE, Chitosan-based delivery systems for protein therapeutics and antigens. Adv Drug Deliv Rev 2010; 62:59-82; PMID:19925837; http://dx.doi.org/10.1016/j.addr.2009.11.009.
- Mohammadpour Dounighi, N, Eskandari R, Avadi MR, Zolfagharian H, Mir Mohammad Sadeghi A, Rezayat M, Preparation and in vitro characterization of chitosan nanoparticles containing Mesobuthus eupeus scorpion venom as an antigen delivery system. J Venomous Animals Toxins Including Tropical Dis 2012; 18(1):44-52.
- Mourya VK, Nazma NI. Trimethyl chitosan and its applications in drug delivery. J Mater Sci Mater Med 2009; 20:1057-79 ; PMID:19112609.
- Sharma M, Sharma V, Panda AK, Majumdar DK, Enteric microsphere formulations of papain for oral delivery. Yakugaku Zasshi. 2011; 131(5):697-709; PMID:21532266; http://dx.doi.org/10.1248/yakushi.131.697.
- George M., Abraham T.E. Polyionic hydrocolloids for the intestinal delivery of protein drugs: alginate and chitosan – a review. J Control Release 2014 114,1-14; http://dx.doi.org/10.1016/j.jconrel.2006.04.017.
- Xiong X, Li Y, Li Z, Zhou C, Tam K, Liu Z, Xie G. Vesicles from Pluronic/poly(lactic acid) block copolymers as new carriers for oral insulin delivery, J. Control Release 2007; 120:11-7; PMID:17509718; http://dx.doi.org/10.1016/j.jconrel.2007.04.004.
- Almeida A, Souto E. Solid lipid nanoparticles as a drug delivery system for peptides and proteins. Adv Drug Deliv Rev 2007; 59:478-90; PMID:17543416; http://dx.doi.org/10.1016/j.addr.2007.04.007.
- Thanoua MM, Kotze´ A, Scharringhausena FT, Lueßenc H.L, Boerd A.Gde, Verhoefa J.C, Jungingera H.E. Effect of degree of quaternization of N-trimethyl chitosan chloride for enhanced transport of hydrophilic compounds across intestinal Caco-2 cell monolayers, J Control Release 2000; 64:15-25; PMID:10640642; http://dx.doi.org/10.1016/S0168-3659(99)00131-5.
- Sieval AB, Thanou M, Kotze´ AF, Verhoef JC, Brussee J, Junginger HE. Preparation and NMR-characterization of highly substituted N-trimethyl chitosan chloride, Carbohydr. Polym. 1998; 36:157-65.
- Mohammadpour Dounighi N, Damavandi M, Zolfagharian H, Moradi S. Preparing and characterizing chitosan nanoparticles Containing Hemiscorpius lepturus scorpion venom as an antigen delivery system, Archives of Razi Institute 2012; 67(2):145-53.
- Bradford M. A Rapid and sensitive method for the quantitation of microgram quantities of protein utilizing the principle of protein-dye binding. Anal Biochem 1976; 72:248-54; PMID:942051; http://dx.doi.org/10.1016/0003-2697(76)90527-3.
- Kruger N. The Bradford method for protein quantitation. Methods Mol Biol 1994; 32:9-15; PMID:7951753.
- Saraei F, Mohamadpour Dounighi N, Zolfagharian H, Moradi Bidhendi S, Khaki P, Inanlou F. Design and evaluate alginate nanoparticles as a protein delivery system. Archives of Razi Institute 2013; 68(2):139-46.
- Sarei F, Mohammadpour Dounighi N,Zolfagharian H, Khaki P, Moradi Bidhendi, Alginate nanoparticles as a promising adjuvant and vaccine delivery system, Indian J Pharm Sci 2013; 75(4):442-449; PMID:24302799; http://dx.doi.org/10.4103/0250-474X.119829.
- Ouchterlony O. Diffusion-in-gel methods for immunological analysis. II. Prog Allergy 1962; 6:30-154; PMID:14482809.