ABSTRACT
Animal models facilitate evaluation of vaccine efficacy at relatively low cost. This study was a comparative evaluation of the immunogenicity and protective efficacy of a new 13-valent pneumococcal conjugate vaccine (PCV13) with a control vaccine in a mouse model.
After vaccination, anti-capsular antibody levels were evaluated by pneumococcal polysaccharide (PnP) enzyme-linked immunosorbent assay (ELISA) and opsonophagocytic killing assay (OPA). Also, mice were challenged intraperitoneally with 100-fold of the 50% lethal dose of Streptococcus pneumoniae.
The anti-capsular IgG levels against serotypes 1, 4, 7F, 14, 18C, 19A, and 19F were high (quartile 2 >1,600), while those against the other serotypes were low (Q2 ≤ 800). Also, the OPA titres were similar to those determined by PnP ELISA. Comparative analysis between new PCV13 and control vaccination group in a mouse model exhibited significant differences in serological immunity of a few serotypes and the range of anti-capsular IgG in the population. Challenge of wild-type or neutropenic mice with serotypes 3, 5, 6A, 6B, and 9V showed protective immunity despite of induced relatively low levels of anti-capsular antibodies. With comparison analysis, a mouse model should be adequate for evaluating serological efficacy and difference in the population level as preclinical trial.
Introduction
Streptococcus pneumoniae is an opportunistic pathogen that colonises the upper respiratory tract asymptomatically.Citation1 It can invade the bloodstream and cause systemic diseases such as sepsis, meningitis, and invasive pneumonia.Citation2,Citation3 Mortality rates for invasive pneumococcal disease (IPD) are particularly high in high-risk populations; e.g. young children, the elderly, and immunocompromised patients. At least 1 million children <5 y of age die annually from pneumonia and IPD in developing countries.Citation4
To prevent pneumococcal disease, the pneumococcal polysaccharide vaccine (PPV23), which comprises 23 capsular serotypes and is effective in healthy adults, was first licensed in 1983.Citation5,Citation6 However, as PPV23 is poorly immunogenic in children, the elderly, and immunocompromised individuals,Citation7-9 the 7-valent pneumococcal conjugate vaccine (PCV7), which comprises the 14, 6B, 19F, 23F, 18C, 4, and 9V polysaccharide antigens conjugated to a carrier protein, was developed, and its efficacy has been demonstrated.Citation10-13 However, the incidence of IPD caused by serotypes included in the PCV7 vaccine has decreased,Citation14-16 while that of IPD due to non-PCV7 serotypes has increased.Citation17,Citation18 This has led to the development and licensing of higher-valency pneumococcal conjugate vaccines (PCV10-PCV7 plus serotypes 1, 5, and 7F; and PCV13-PCV10 plus serotypes 3, 6A, and 19A) for prevention of pneumococcal diseases.
Animal models have played an important role in vaccine development. Although determination of vaccine efficacy using animal models is subject to several limitations (strains non-pathogenic in animals, etc.), use of animal models for vaccine research facilitates their translation to clinical use.Citation19 In particular, the mouse model is more economical and convenient than other animal models and has been used in previous studies to demonstrate the immunogenicity and protective efficacy of S. pneumoniae vaccines.Citation20-22 Also, because more individuals in mouse model could be reared in limited area and involved in the experiment than other animal model, population study in a mouse model should be possible for investigating the individual difference and variation in the progress of vaccine evaluating.
In developing country, vaccine development is still needed in economical aspect, as high cost of previously licensed vaccine is a barrier to the people. The aim of this study is to assess mouse model as a preclinical platform in development of pneumococcal vaccine by comparative analysis. It was assessed whether a mouse model might be effective for evaluating the efficacy of higher-valency pneumococcal conjugate vaccines including polysaccharide antigens of S. pneumoniae strains nonpathogenic against mouse. For comparative analysis, a recently patented 13-valent pneumococcal capsular vaccine by a South Korean manufacturer (Patent No. WO 2013191459 A1) and licensed PCV13 were used as assessment materials. Moreover, we described the range of an individual difference in the serological immunogenicity of mouse population, compare with that of previously licensed PCV13 as a control. It was expected that the comparative evaluation in animal population should compensate for the drawbacks of evaluating new vaccine in a mouse model.
Results
Determination of cut-off values and immunization method
The PnP ELISA cut-off value of each serotype calculated using sera from 20 non-immunised mice was A405 = 0.142–0.204 (). For the determination of immunization method, the anti-capsular IgG level was screened in mice (n = 3 per each group) immunised s.c., i.p., and i.m. with three doses of each vaccine (new PCV13 and control vaccine). The no-diluted serum level of IgG against serotypes 5 (A405 = 0.132), 7F (A405 = 0.19), and 23F (A405 = 0.095) in mice immunised s.c with three doses was very low; indeed, those of serotypes 5 and 23F were below the cut-off. However, the level of IgG against each serotype in mice immunised i.p. and i.m. was markedly higher than that in those immunised s.c. (). Although the IgG levels almost in each serotypes did not differ significantly between i.p. immunised group and i.m immunised group, the specific IgG level of i.p. immunised group seem to be a little higher than that of i.m. immunised group (). Comparison results between new PCV13 and control vaccination group exhibited that there should not be any significant difference in the IgG level of all group. These results of small population (n = 3) lead to apply i.p. immunization method to serological evaluation study for larger population (n = 20).
Table 1. Cut-off values of pneumococal polysaccharide enzyme-linked immunosorbent assay in wild-type mice (n = 20).
Table 2. The screening results (mean of endpoint titer) of pneumococal polysaccharide enzyme-linked immunosorbent assay for the determination of immunization method.
Serological evaluation in a mouse model
In the mouse population (n = 20), the levels of anti-capsular IgG against each serotype were determined. The specific IgG endpoint titres ranged from 400–25,600 (serotype 1), 100–6,400 (serotype 3), 6,400–51,200 (serotype 4), 100–3,200 (serotype 5), 200–12,800 (serotype 6A), 200–6,400 (serotype 6B), 800–6,400 (serotype 7F), 100–12,800 (serotype 9V), 12,800–51,200 (serotype 14), 100–12,800 (serotype 18C), 400–6,400 (serotype 19A), 800–25,600 (serotype 19F), and 100–800 (serotype 23F). The quartile values (Q1, Q2, and Q3) in each vaccination groups (new PCV13 and control vaccine) are shown in . The immunogenicity of serotypes 6B, 7F, 14, 18C, and 19F differed significantly (p < 0.05) between the two groups, but that of serotypes 1, 3, 4, 5, 6A, 9V, 19A, and 23F did not (). The incidence of the endpoint titer of each serotype in the population of immunised mice (n = 20) is presented as a box plot in . In the analysis of interquartile range (IQR = Q3-Q1), the immunogenic range of serotype 3 and 6A exhibited >4-fold difference in the population (n = 20) of new PCV13 vaccination group, while serotype 1 and 19A showed .4-fold difference in control vaccination group. Other serotypes exhibited ≤ 4-fold change in the population of both immunization group. The range of the anti-capsular IgG level in the same serotype was shown differently, depending on each individual and the kind of vaccine in a mouse model.
Table 3. Quartile values of the endpoint titer of IgG against each serotype in mice (n = 20) i.p. immunised with new PCV13 and control vaccine.
Figure 1. Box plot of pneumococal polysaccharide enzyme-linked immunosorbent assay endpoint titres of each serotype in mice (n = 20) immunised with (A) new PCV13 (SK PCV13) and (B) control vaccine.
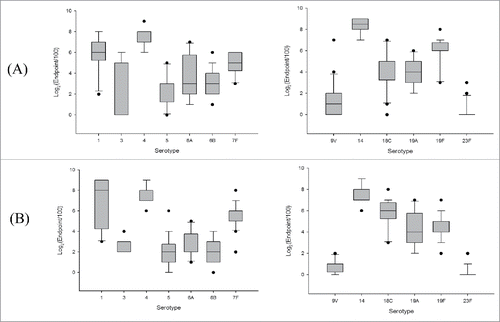
Functional antibody titres were determined by OPA. The titer was defined as the reciprocal of the serum dilution that resulted in a 50% reduction in the number of bacterial colonies, compare with the control. The OPA titres in the mice (n = 20) immunised with new PCV13 are presented as a box plot in . The titres of functional antibodies against serotypes 3, 5, 6B, 9V, and 23F were low (Q2 < 30) in both immunised populations (). However, the titres against other serotypes were higher and similar to those determined by PnP ELISA.
Challenge of animal
To assess protective immunity following immunisation with new PCV13, S. pneumoniae serotype 3 strain A66 (mouse virulent strain) was administered i.p. to mice (n = 3 per non-vaccinated group) with inoculation of 102, 103 and 105 CFU/0.1mL, respectively. All mice in each group were killed in 36 hr (). Also mice (n = 3, vaccinated group) were administered the pneumococcal strains at 105 CFU/0.1mL at 5 weeks after the final immunisation. The immunised groups showed 100% protection ().
Figure 3. Induction of protective immunity by new PCV13 (SK PCV13). S. pneumoniae serotype 3 strain A66 (mouse virulent strain) was administered i.p. to mice (n = 3 per non-vaccinated group). Lethal effect was monitored for 72 hr. In vaccinated group, mice were immunised intraperitoneally three times at 0, 2, and 4 weeks with 15.4 μg of new PCV13. At 5 weeks after the final, mice (n = 3) were challenged intraperitoneally with S. pneumoniae A66 at 105 CFU/0.1mL. Three of mice per group were used in this experiment (n = 3).
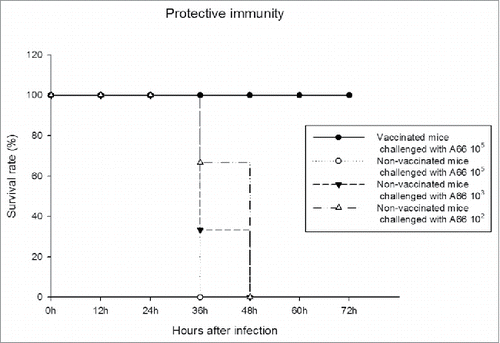
In the case of other serotypes, serotypes 1, 4, 5, and 6A showed >50% lethality in 48hr following intraperitoneal inoculation of 103- 105 CFU/0.1 mL (n = 3 of each group) (). The other serotypes showed no lethality in wild mice. So we tried to use neutropenic mouse model. Neutrophils principally participate in the defense against pneumococcoi. In neutropenic mice, neutrophils are decreased to be immunocompromised state. But other immune system such as like macrophage and complements could involve in the protection, although neutrophils is restricted. In this study, other immunsystem, especially intraperitoneal macrophage, is expected to protect, as pneumococci inoculated i.p. Also specific IgG and complements could inhibit to progress into systematic disease. In neutropenic mice, serotypes 6B, 9V, and 18C showed >50% lethality in 72hr following intraperitoneal inoculation of 106 CFU (n = 3 of each group) (). Serotypes 7F, 14, 19A, 19F, and 23F showed little (< 50%) or no lethality in wild-type and neutropenic mice (n = 3 of each group).
Table 4. Survival rate (%) of each serotype strains in wild and neutropenic mouse model (n = 3).
To assess protective immunity, mice were challenged with 100-fold the LD50 of serotypes 1, 4, 5, 6A, 6B, 9V, and 18C after three-dose vaccination. Serotypes 1, 3, 4, 5, and 6A were administered i.p. to wild mice, and the other serotypes to neutropenic mice. Wild-type and neutropenic mice immunised with new PCV13 and control vaccine showed 100% protective activity against each challenged serotypes.
Discussion
Animal model for the infectious disease has played an important role to the development of human vaccines. The evaluation of quantity and quality of immune responses in animal model could be indicators of therapeutic potential. In this study, new PCV13 vaccine was developed as condition in the manufacturing process were determined by competent level of immunogenicity and conjugation yield. Then, several critical process parameters, such as protein and polysaccharide conjugation ratio and degree of oxidation of polysaccharides, were optimized for specific serotypes. In developing the vaccine, we utilized the key principle of reductive amination process in which NaIO4 could cleave a C‒C bond (alkene) and form two aldehydes. Consequently, the amines of proteins could couple to the aldehydes to form C‒N bonds. Here, the oxidation of the polysaccharide by NaIO4 increased efficiency of the reductive amination process. Nonetheless, the cleavage of the C-C bond by NaIO4 could cause structural changes in polysaccharide epitope, influencing not all, but only a portion of polysaccharide epitope. Some changes in polysaccharides epitope might affect the immunogenicity of the polysaccharides, but our study showed that there should be not a significant impact in the immunogenicity of the polysaccharides, as the level of anti-capsular IgG was sufficiently immunogenic in the population of mouse, compare with that of control vaccine.
Prior to applying the clinical trial, the evaluation of immune response and efficacy in animal model should be efficient to translate results to the clinic. We assumed that comparative study in animal model could efficiently assess the efficacy of the newly developed vaccine, although it is difficult to directly compare with each other in the potential improvement. By comparative study, we also assessed whether a mouse model might be effective for evaluating the efficacy of higher-valency pneumococcal conjugate vaccines.
The induction of functional antibodies has been investigated in animal models by PnP ELISA and OPA.Citation23 Animal models of infection facilitate evaluation of specific capsular antibodies and their ability to prevent infection.Citation20-22,Citation24,Citation25 Animal models have been used to test pneumococcal protein vaccines; indeed, the adult mouse is a convenient model that can predict vaccine efficacy in human.Citation26 Assessment of newly developed vaccines in animal models prior to clinical testing enables evaluation of specific antibody production and protective efficacy. The mouse model facilitates vaccine population studies as a large number of animals can be used, compare with other animal models. However, measurement of the geometric mean concentrations (GMC) of anti-capsular IgG is problematic in mouse model, due to the low volume of serum that can be obtained from each immunised animal. Therefore, we adopted the reciprocal of serum dilution to determine anti-capsular IgG titres using PnP ELISA and OPA to overcome the limitation of testing immunogenicity in the mouse model.
In this study, we assessed the immunogenicity and protective efficacy of new PCV13, in comparison with those of previously licensed vaccines as a control, in a mouse model. PnP ELISA and OPA results can be used as primary endpoints for assessment of vaccine immunogenicity. Through the trial of new PCV13 to mouse population model, the range of the anti-capsular IgG level in the population was checked. The anti-capsular IgG levels against serotypes 1, 4, 7F, 14, 18C, 19A, and 19F were considerably higher (Q2 >1,600) than those of the other serotypes in both immunised groups (). However, the Q2 of specific IgG levels against the other serotypes was relatively low (Q2 ≤ 800) in both immunised groups, particularly that against serotype 23F (Q3 = 100). As the immunogenicity of serotypes 6B, 7F, 14, 18C, and 19F differed significantly (p < 0.05) (), there should be significantly different serological immunity in new PCV13 and control in a mouse model. Interquartile range (IQR = Q3-Q1) analysis showed that the immunogenic range of most serotypes exhibited ≤ 4-fold change in the population (n = 20) of both immunization group (new PCV13 and control), except of serotype 3 and 6A (new PCV13) and 1 and 19A (control) ( and ). Comparative analysis in a mouse model exhibited significant differences in serological immunity of a few serotypes and the range of anti-capsular IgG in the population of both vaccination groups. Therefore, our findings suggest that a mouse model should be adequate for evaluating serological efficacy and difference in the population level with comparison analysis.
However, there were some barriers to the results of serological evaluation in mice could be applied to the clinic. US study showed that serotype-specific IgG GMCs (μg/mL) after three-dose infant series in the clinic with current Prevnar (PCV13) ranged from 0.49 (serotype 3) to 4.74 (serotype 14).Citation27 In our data, the immunogenicity in mice of each of the 13 serotypes did not correlate completely with that of current Prevnar vaccine seen in the clinic. The immunogenicity in serotype 1, 6A, 6B, 7F, 14, and 19A exhibited >2 μg/mL of GMC in the clinic,Citation27 while serotype 1, 4, 7F, 14, 18C, and 19F exhibited >1,600 (Q2) in mice with Prevnar (PCV13). The lower value (< 1 of GMC and <400 of endpoint) was showed in serotype 3 and 9V in the clinic,Citation27 while in 9V and 23F in mice. These showed that there could be different in the immunogenicity between mice and human against the 13 serotypes, although it is difficult to compare GMC with endpoint value.
Challenge of wild-type or neutropenic mice with serotypes 3, 5, 6A, 6B, and 9V demonstrated induction of protective immunity, despite low anti-capsular antibody levels. Also, challenge of mice showed induction of protective immunity against serotypes 1, 4, 7F, and 18C, which also exhibited greater immunogenicity. However, protective immunity against all PCV13 vaccine serotypes could not be evaluated, as a model of infection with serotypes 14, 19A, 19F, and 23F was not available. S. pneumoniae strains non-pathogenic to mice have been reported, and serotype 23F was reported to be poorly immunogenic in CBA/J mice.Citation28 With challenging of less-virulent S. pneumoniae sereotype strains in a neutropenic mouse model, protection efficacy could be evaluated, but both mouse models still exhibited some limits in evaluating protection efficacy for a few avirulent serotypes strains. Animal model could not completely overcome the difference of immunoresponse and protection efficacy between human and animal. But animal model has some values for screening and evaluating immunoresponse as a translational research, prior to the clinical trial. One of the aim in this study is to assess mouse model as a preclinical platform in development of pneumococcal vaccine. Although there are some limits to assess the protection efficacy against a few serotypes, mouse model could be a good model for serological evaluation in the population level.
In summary, comparison analysis in a mouse model, including neutropenic model, should be economical and effective for developing a new vaccine and evaluating the difference width of serological immunity in the population level. However, a mouse model of infection with avirulent vaccine serotypes is required.
Materials and methods
Mice
Female 6–8-week-old BALB/c mice (Orient Bio, Sungnam, South Korea) used in this study were reared in the Department of Laboratory Animals, The Catholic University of Korea. All surgical interventions and pre- and post-surgical animal care were performed in accordance with the Laboratory Animals Welfare Act, the Guide for the Care and Use of Laboratory Animals, and the Guidelines and Policies for Rodent Survival Surgery of the Institutional Animal Care and Use Committee of the School of Medicine, The Catholic University of Korea, Seoul, South Korea (Approval No. CUMC-2013–0123–03).
Vaccines
A new PCV13 (Patent No. WO 2013191459) used in this study was provided by SK Chemical Co., Ltd. (Sungnam, South Korea) and a control PCV13 (Prevnar 13®, Wyeth Pharmaceuticals, Collegeville, PA, USA) was purchased from Wyeth Korea (Seoul, South Korea).
The technical information on the new PCV13 vaccine (Patent No. WO 2013191459) is briefly described as below.
The individual pneumococcal polysaccharides are purified through centrifugation, precipitation, filtration, and ultrafiltration/diafiltraion. Then the polysaccharides are chemically oxidized by NaIO4 to yield activated saccharides, which are directly conjugated by reductive amination to the CRM197 carrier protein to form the conjugate. In this process of reductive amination, covalent bonds between proteins and carbohydrates are formed without a formation of ligand. Periodate oxidation is used to activate polysaccharides in order to generate conjugation of saccharides to protein carriers. In periodate oxidation, sodium periodate (NaIO4) is utilized as an oxidizing agent used to yield oxidation of vicinal diols in the polysaccharides which consequently introduces reactive aldehydes. The aldehyde groups are required in conjugation with carrier proteins. In order to form a covalent bond between aldehyde and amine of proteins, sodium cyanoborohydride is especially suitable for reductive amination since the reaction rate for the reduction of iminium ions is much faster than aldehydes. The conjugation reaction is incubated under agitation using time and temperature conditions specific to each serotype. The reaction mix is diluted and purified by ultrafiltration/diafiltration processes.
Each 0.5 mL dose contains: 2.2 μg of pneumococcal purified capsular polysaccharides for serotypes 1, 3, 4, 5, 6A, 7F, 9V, 14, 18C, 19A, 19F and 23F, and 4.4 μg of pneumococcal purified capsular polysaccharides for serotype 6B.
Each serotype is individually conjugated to CRM197 carrier protein and adsorbed on aluminums phosphate.
Bacterial strains and culture
S. pneumoniae serotypes 1, 3, 4, 5, 6A, 6B, 7F, 9V, 14, 18C, 19A, 19F, and 23F were obtained from Statens Serum Institut (Copenhagen, DK) for the opsonophagocytic killing assay (OPA) and challenge of mice. S. pneumoniae strains were grown on blood agar (Hanil Komed, Co., Sungnam, South Korea) and Todd-Hewitt yeast (THY, BD, Sparks, MD, USA) agar or broth. Cultures in exponential phase were stored at –80°C in THY medium with 25% glycerol. Viability was confirmed by cell counting prior to experiments.
Immunisation, pneumococcal polysaccharide (PnP) ELISA, and OPA assay
Mice were immunised intraperitoneally (i.p. group), intramuscularly (i.m. group) or subcutaneously (s.c. group) three times at 14-day intervals with new PCV13 (15.4 or 30.8 µg total polysaccharides) or the control vaccine (Prevnar 13®). Blood samples were collected 7 d after each boost. Sera were aliquoted and stored at –70°C until required.
ELISA result was compared with the same antigen with each immunized sera (new PCV13 & Prevnar 13®). Levels of IgG against serotypes 1, 3, 4, 5, 6A, 6B, 7F, 9V, 14, 18C, 19A, 19F, and 23F were evaluated by determining endpoint titres. The endpoint titer was defined as the highest dilution above the cut-off and was calculated as the sum of the mean and two standard deviations of 20 negative mouse sera.
Serum from immunised mice was preincubated with C-polysaccharide and serotype 22F capsular polysaccharide to remove nonspecific antibodies.Citation29
PnP ELISA was performed as described previously.Citation30 Briefly, 96-well ELISA plates were coated with 10 µg/mL polysaccharide in phosphate-buffered saline (PBS) for 3 h at room temperature, followed by blocking with 1% bovine serum albumin (Sigma-Aldrich, St. Louis, MO, USA). Sera were serially diluted twofold, and the plates were incubated at 37°C for 1 h. Then, a 1:10,000 dilution of alkaline phosphatase-conjugated goat anti-mouse secondary IgG (Thermo, Rockford, IL, USA) was added, and binding was detected using p-nitrophenyl phosphate substrate (Sigma-Aldrich) in diethanolamine buffer (pH 9.8). Between each step, plates were washed with PBS-0.05% Tween 20 at least three times. Optical densities (ODs) at 405 nm were measured using a Spectramax190 microplate reader (Molecular Devices, Sunnyvale, CA, USA).
For the OPA, the murine macrophage-like cell line J774A.1 (ATCC® TIB-67™) was used,Citation31 and the assay was performed as described previously, with some modifications.Citation32 Briefly, 2 × 103 CFU/10 μL of pneumococci of each serotype were added to 40 μL of sera from immunised mice diluted with Hank's balanced salt solution (HBSS; Life Technologies Korea LLC, Seoul, South Korea). After incubation for 30 min at room temperature, 40 μL of J774A.1 at a concentration of 2.5 × 107 cells/mL, with or without 10 μL of mouse complement serum (Sigma-Aldrich) were added, and the mixture (500:1 effector-to-target cell ratio) was incubated for 1 h at 37°C with shaking. After incubation, 10 μL of the solution were plated onto THY agar in triplicate. The plates were incubated overnight at 37°C in 5% CO2, and the colonies were counted.
Challenge of mice
The 50% lethal dose (LD50) of each serotype in mice was determined by monitoring survival for 1 week following intraperitoneal administration. Establishment of sepsis was confirmed by enumerating colony-forming units (CFU) in the spleen, kidney, and lung. Mouse tissue specimens were collected, suspended in PBS, serially diluted, and then plated on THY agar. Colonies were subjected to serotype-specific PCR.Citation33 For the intraperitoneal challenge models, mice were administered the pneumococcal strains at 100-fold the LD50 at 5 weeks after the final immunisation of new PCV13, and survival was monitored for 1 week. Neutropenic mice (polymorphonuclear cell count, <100 mm3) for challenge tests of less-virulent serotypes were generated by two intraperitoneal injections of cyclophosphamide (Baxter, Halle/Westfalen, Germany) at 150 mg/kg body weight at 4 days, and 100 mg/kg at 1 day, before challenge.Citation34,Citation35
Statistical analysis
Statistical analysis was performed using the Prism software (GraphPad Software, Inc., La Jolla, CA, USA). Comparisons of PnP ELISA, OPA, and survival results were performed by unpaired t-test. A p-value <0.05 was considered to indicate statistical significance. The box-plot analysis was performed using SigmaPlot version 10.0 software (Systat Software Inc., San Jose, CA, USA).
Abbreviations
CFU | = | colony-forming units |
CRM197 | = | a genetically detoxified form of diphtheria toxin |
IPD | = | invasive pneumococcal disease |
IQR | = | interquartile range |
LD50 | = | 50% lethal dose |
OPA | = | opsonophagocytic killing assay |
PCV13 | = | 13-valent pneumococcal conjugate vaccine |
PnP ELISA | = | pneumococcal polysaccharide enzyme-linked immunosorbent assay |
Q | = | quartile |
Disclosure of potential conflicts of interest
D.G.L. received grants which were made up through his institution research program funded by SK Chemical and the government. J.H.S. and H.K. are employees of SK Chemical Co., Ltd. But none of the authors has any conflicts of interest.
Authors’ contributions
All authors have reviewed and approved the final manuscript. All authors had full access to the data. P.C. designed the study and contributed toward data analyses and interpretation in collaboration with all authors. D.G.L. and J.H.K supervised the conduct of the study and critically reviewed manuscript. J.H.S and H.K contributed forward the management of SK PCV13 vaccine in this study. The authors received no financial support or other form of compensation for the development of the manuscript.
Funding
This work was supported by The Catholic University of Korea Research and Development grant (No. 5–2013-D0061–00001) from SK Chemicals Co., Ltd. Also, it was supported in part by a grant from the Korea Health Technology R&D Project through the Korea Health Industry Development Institute (KHIDI), funded by the Ministry of Health & Welfare, Republic of Korea (No. HI14C2116).
References
- Gray BM, Dillon HC. Clinical and epidemiologic studies of pneumococcal infection in children. Pediatr Infect Dis 1986; 5:201-7; PMID:3952010; http://dx.doi.org/10.1097/00006454-198603000-00009
- Austrian R, Gold J. Pneumococcal bacteremia with specific reference to bacteremic pneumococcal pneumonia. Ann Intern Med 1964; 60:759–76; PMID:14156606; http://dx.doi.org/10.7326/0003-4819-60-5-759
- Dagan R, Englehard D, Piccard E, Israeli Pediatric Bacteremia and Meningitis Group. Epidemiology of invasive childhood pneumococcal infections in Israel. JAMA 1992; 268:3328-32; PMID:1364814; http://dx.doi.org/10.1001/jama.1992.03490230058028
- Obaro S, Adegbola R. The pneumococcus: carriage, disease and conjugate vaccines. J Med Microbiol 2002; 51:98-104; PMID:11863272; http://dx.doi.org/10.1099/0022-1317-51-2-98
- Butler JC, Breiman RF, Campbell JF, Lipman HB, Broome CV, Facklam RR. Pneumococcal polysaccharide vaccine efficacy. An evaluation of current recommendations. JAMA 1993; 270:1826-31; PMID:8411526; http://dx.doi.org/10.1001/jama.1993.03510150060030
- Shapiro ED, Berg AT, Austrian R, Schroeder D, Parcells V, Margolis A, Adair RK, Clemens JD. The protective efficacy of a polyvalent pneumococcal polysaccharide vaccine. N Engl J Med 1991; 325:1453-60; PMID:1944423; http://dx.doi.org/10.1056/NEJM199111213252101
- Douglas RM, Paton JC, Duncan SJ, Hansman DJ. Antibody response to pneumococcal vaccination in children younger than five years of age. J Infect Dis 1983; 148:131-7; PMID:6886479; http://dx.doi.org/10.1093/infdis/148.1.131
- Lee CJ, Wang TR. Pneumococcal infection and immunization in children. Crit Rev Microbiol 1994; 20:1-12; http://dx.doi.org/10.3109/10408419409113544
- Forrester HL, Jahningen DW, LaForce FM. Inefficacy of pneumococcal vaccine in a high-risk population. Am J Med 1987; 83:425-30; PMID:3661581; http://dx.doi.org/10.1016/0002-9343(87)90751-0
- Anderson EL, Kennedy DJ, Geldmacher KM, Donnelly J, Mendelman PM. Immunogenicity of a heptavalent pneumococcal conjugate vaccine in infants. J Pediatr 1996; 128:649-53; PMID:8627437; http://dx.doi.org/10.1016/S0022-3476(96)80130-2
- Black S, Shinefield H, Fireman B, Lewis E, Ray P, Hansen JR, Elvin L, Ensor KM, Hackell J, Siber G, et al. Austrian, K. Edwards, Efficacy, safety and immunogenicity of heptavalent pneumococcal conjugate vaccine in children. Northern California Kaiser Permanente Vaccine Study Center Group. Pediatr Infect Dis J 2000; 19:187-95; PMID:10749457; http://dx.doi.org/10.1097/00006454-200003000-00003
- Dagan R, Melamed R, Muallem M, Piglansky L, Greenberg D, Abramson O, Mendelman PM, Bohidar N, Yagupsky P. Reduction of nasopharyngeal carriage of pneumococci during the second year of life by a heptavalent conjugate pneumococcal vaccine. J Infect Dis 1996; 174:1271-8; PMID:8940218; http://dx.doi.org/10.1093/infdis/174.6.1271
- Dagan R, Givon-Lavi N, Zamir O, Sikuler-Cohen M, Guy L, Janco J, Yagupsky P, Fraser D. Reduction of nasopharyngeal carriage of Streptococcus pneumoniae after administration of a 9-valent pneumococcal conjugate vaccine to toddlers attending day care centers. J Infect Dis 2002; 185:927-36; PMID:11920317; http://dx.doi.org/10.1086/339525
- Black S, Shinefield H, Baxter R, Austrian R, Bracken L, Hansen J, Lewis E, Fireman B. Postlicensure surveillance for pneumococcal invasive disease after use of heptavalent pneumococcal conjugate vaccine in Northern California Kaiser Permanente. Pediatr Infect Dis J 2004; 23:485-9; PMID:15194827; http://dx.doi.org/10.1097/01.inf.0000129685.04847.94
- Whitney CG, Farley MM, Hadler J, Harrison LH, Bennett NM, Lynfield R, Reingold A, Cieslak PR, Pilishvili TD, Jackson D, et al; Active Bacterial Core Surveillance of the Emerging Infections Program Network. Decline in invasive pneumococcal disease after the introduction of protein-polysaccharide conjugate vaccine. N Engl J Med 2003; 348:1737-46; PMID:12724479; http://dx.doi.org/10.1056/NEJMoa022823
- Kaplan SL, Mason EO Jr, Wald ER, Schutze GE, Bradley JS, Tan TQ, Hoffman JA, Givner LB, Yogev R, Barson WJ. Decrease of invasive pneumococcal infections in children among 8 children's hospitals in the United States after the introduction of the 7-valent pneumococcal conjugate vaccine. Pediatrics 2004; 113:443-9; PMID:14993532; http://dx.doi.org/10.1542/peds.113.3.443
- Byington CL, Samore MH, Stoddard GJ, Barlow S, Daly J, Korgenski K, Firth S, Glover D, Jensen J, Mason EO, et al. Temporal trends of invasive disease due to Streptococcus pneumoniae among children in the intermountain west: emergence of nonvaccine serogroups. Clin Infect Dis 2005; 41:21-9; PMID:15937758; http://dx.doi.org/10.1086/430604
- Albrich WC, Baughman W, Schmotzer B, Farley MM. Changing characteristics of invasive pneumococcal disease in metropolitan Atlanta, Georgia, after introduction of a 7-valent pneumococcal conjugate vaccine. Clin Infect Dis 2007; 44:1569-76; PMID:17516400; http://dx.doi.org/10.1086/518149
- Gerdts V, Littel-van den Hurk Sv, Griebel PJ, Babiuk LA. Use of animal models in the development of human vaccines. Future Microbiol 2007; 2:667-75; PMID:18041907; http://dx.doi.org/10.2217/17460913.2.6.667
- Alonso De Velasco E, Dekker BA, Verheul AF, Feldman RG, Verhoef J, Snippe H. Anti-polysaccharide immunoglobulin isotype levels and opsonic activity of antisera: Relationships with protection against Streptococcus pneumoniae infection in mice. J Infect Dis 1995; 172:562-5; PMID:7622906; http://dx.doi.org/10.1093/infdis/172.2.562
- Musher DM, Johnson B Jr, Watson DA. Quantitative relationship between anticapsular antibody measured by enzyme linked immunosorbent assay or radioimmunoassay and protection of mice against challenge with Streptococcus pneumoniae serotype 4. Infect Immun 1990; 58:3871-6; PMID:2254015
- Saeland E, Vidarsson G, Jonsdottir I. Pneumococcal pneumonia and bacteremia model in mice for the analysis of protective antibodies. Microb Pathog 2000; 29:81-91; PMID:10906263; http://dx.doi.org/10.1006/mpat.2000.0363
- Vidarsson G, Jonsdottir I, Jonsson S, Valdimarsson H. Opsonization and antibodies to capsular and cell wall polysaccharides of Streptococcus pneumoniae. J Infect Dis 1994; 170:592-9; PMID:8077716; http://dx.doi.org/10.1093/infdis/170.3.592
- Fernsten P, Mason KW, Yu X, Tummolo D, Belanger KA, Tsao H, Zhu D, Cooper D, Hagen M, Jansen KU. 13-valent pneumococcal conjugate vaccine immune sera protects against pneumococcal serotype 1, 3, and 5 bacteremia in a neonatal rat challenge model. Hum Vaccin 2011; 7 Suppl:75-84; PMID:21266856; http://dx.doi.org/10.4161/hv.7.0.14566
- Chiavolini D, Pozzi G, Ricci S. Animal models of Streptococcus pneumoniae disease. Clin Microbiol Rev 2008; 21:666-85; PMID:18854486; http://dx.doi.org/10.1128/CMR.00012-08
- Steinhoff MC; The Pneumococcal Vaccine Animal Model Consensus Group. Animal models for protein pneumococcal vaccine evaluation: a summary. Vaccine 2007; 25:2465-70; PMID:17034908; http://dx.doi.org/10.1016/j.vaccine.2006.09.021
- Plosker GL. 13-valent pneumococcal conjugate vaccine: a review of its use in infants, children, and adolescents. Paediatr Drugs 2013; 15:403-23; PMID:24030738; http://dx.doi.org/10.1007/s40272-013-0047-z
- McCool TL, Harding CV, Greenspan NS, Schreiber JR. B- and T-cell immune responses to pneumococcal conjugate vaccines: divergence between carrier- and polysaccharide-specific immunogenicity. Infect Immun 1999; 67:4862-9; PMID:10456942
- Concepcion NF, Frasch CE. Pneumococcal type 22F absorption improves the specificity of a pneumococcal polysaccharide enzyme-linked immunosorbent assay. Clin Diagn Lab Immunol 2001; 8:266-72; PMID:11238206
- Tian H, Groner A, Boes M, Pirofski LA. Pneumococcal capsular polysaccharide vaccine-mediated protection against serotype 3 Streptococcus pneumoniae in immunodeficient mice. Infect Immun 2007; 75:1643-50; PMID:17220309; http://dx.doi.org/10.1128/IAI.01371-06
- Arulanandam BP, Lynch JM, Briles DE, Hollingshead S, Metzger DW. Intranasal vaccination with pneumococcal surface protein A and interleukin-12 augments antibody-mediated opsonization and protective immunity against Streptococcus pneumoniae infection. Infect Immun 2001; 69:6718-24; PMID:11598043; http://dx.doi.org/10.1128/IAI.69.11.6718-6724.2001
- Tian H, Weber S, Thorkildson P, Kozel TR, Pirofski LA. Efficacy of opsonic and nonopsonic serotype 3 pneumococcal capsular polysaccharide-specific monoclonal antibodies against intranasal challenge with Streptococcus pneumoniae in mice. Infect Immun 2009; 77:1502-13; PMID:19168739; http://dx.doi.org/10.1128/IAI.01075-08
- Brito DA, Ramirez M, de Lencastre H. Serotyping Streptococcus pneumoniae by multiplex PCR. J Clin Microbiol 2003; 41:2378-84; PMID:12791852; http://dx.doi.org/10.1128/JCM.41.6.2378-2384.2003
- Lee DG, Murakami Y, Andes DR, Craig WA. Inoculum effects of ceftobiprole, daptomycin, linezolid, and vancomycin with Staphylococcus aureus and Streptococcus pneumoniae at inocula of 105 and 107 CFU injected into opposite thighs of neutropenic mice. Antimicrob Agents Chemother 2013; 57:1434-41; PMID:23295932; http://dx.doi.org/10.1128/AAC.00362-12
- Shin HH, Han S, Yim DS, Lee DG, Park C, Kim SH, Kwon JC, Hong KW, Park SH, Choi SM, et al. Efficacy of vancomycin against Staphylococcus aureus according to inoculum size in a neutropenic mouse infection model. Infect Chemother 2011; 43:251-7; http://dx.doi.org/10.3947/ic.2011.43.3.251