ABSTRACT
Serotype-independent protein-based pneumococcal vaccines represent attractive alternatives to capsular polysaccharide-based vaccines. The aim of this study was to identify novel immunogenic proteins from Streptococcus pneumoniae that may be used in protein-based pneumococcal vaccine. An immunoproteomics approach and a humanized severe combined immunodeficient mouse model were used to identify S. pneumoniae proteins that are immunogenic for the human immune system. Among the several proteins identified, SP1683 was selected, recombinantly produced, and infection and colonization murine models were used to evaluate the capacity of SP1683 to elicit protective responses, in comparison to known pneumococcal immunogenic proteins (PhtD and detoxified pneumolysin, dPly). Immunisation with SP1683 elicited a weaker antibody response than immunisation with PhtD and did not provide protection in the model of invasive disease. However, similar to PhtD, it was able to significantly reduce colonization in the mouse model of nasopharyngeal carriage. Treatment with anti-IL17A and anti-IL17F antibodies abolished the protection against colonization elicited by SP1683 or PhtD + dPly, which indicated that the protection afforded in this model was Th17-dependent.
In conclusion, intranasal immunization with the pneumococcal protein SP1683 conferred IL17-dependent protection against nasopharyngeal carriage in mice, but systemic immunization did not protect against invasive disease. These results do not support the use of SP1683 as an isolated pneumococcal vaccine antigen. Nevertheless, SP1683 could be used as a first line of defence in formulations combining several proteins.
Introduction
Streptococcus pneumoniae is an encapsulated gram-positive bacterium that belongs to the commensal flora of the human upper respiratory tract. In 2008 it was estimated to have caused ∼ 0.4 million children deaths worldwide.Citation1 S. pneumoniae can cause invasive diseases such as meningitis and sepsis and respiratory infections such as pneumonia and otitis media. Especially young children, the elderly and people with an underlying disease are vulnerable for infections with S. pneumoniae. Vaccination is an effective means to control pneumococcal disease.Citation1
A 23-valent capsular polysaccharide (caps-PS)-based vaccine was made available in 1983.Citation2 The serotypes contained within this vaccine account for 90% of the serotypes causing serious pneumococcal disease in industrialized countries.Citation3 The vaccine induces serotype-specific antibodies which provide host protection by induction of opsonophagocytosis.Citation4 However, given the 90 different pneumococcal serotypes, a comprehensive caps-PS-based vaccine does not appear feasible. In addition, although caps-PS based vaccines can elicit antibody response in most healthy adults, they are ineffective in children less than two years of age and in immuno-compromised individuals.Citation5 Moreover, unconjugated caps-PS-based vaccines, due to their T-independent nature and thus the lack of T-cell help, are not able to induce B-cell immunological memory.Citation6
To overcome these problems, vaccine manufacturers have developed pneumococcal conjugate vaccines in which pneumococcal caps-PS are covalently coupled to a protein carrier in order to elicit a T cell-dependent immune response.Citation7,Citation8 Such vaccines have shown high level of effectiveness against pneumococcal diseases in children up to 5 years of age.Citation6,Citation9 Nevertheless, in some countries in which conjugated vaccines are used, an increased prevalence of serotypes that are not contained in the vaccine (replacement phenomenon) was observed.Citation10 These observations have driven the search for new approaches, such as protein-based vaccines.Citation11 Indeed, broad and serotype-independent protection can be expected if highly conserved proteins are used as vaccine components.Citation12 In addition, protein vaccines should be simpler to manufacture and, therefore, potentially cheaper than conjugate vaccines.
Although a lot of information is available on immunogenic pneumococcal proteins, only few of them have been tested in clinical trials.Citation13,Citation14 In the present study, we used an immuno-proteomics approach to identify proteins in S. pneumoniae that are immunogenic for the human immune system, hence possible pneumococcal vaccine candidates. One protein (SP1683) was selected for evaluation of its protective capacity after immunization in a colonization and an invasive disease model.
Results
Identification of immunogenic pneumococcal proteins
In a first step, we identified pneumococcal proteins that are immunogenic for the human immune system. For that, peripheral blood mononuclear cells (PBMCs) from three different adult blood donors were isolated and transferred to severe combined immunodeficient (SCID) mice. For each blood donor, four SCID/SCID mice were reconstituted with PBMCs and immunized with heat-inactivated S. pneumoniae serotype 3 (thus in total 12 mice reconstituted with PBMCs from three donors). Serum was collected two weeks after immunization.
Reactivity to caps-PS3 was confirmed by measurement of serotype-specific IgM and IgG antibodies by enzyme-linked immunosorbent assay (ELISA) (data not shown). The serum was also used in Western blotting analyses after two-dimensional separation of an extract of S. pneumoniae serotype 3. Protein spots for which there was reactivity for each of the three different blood donors were excised and identified by mass spectrometry. The most important immunogenic pneumococcal proteins that we identified are listed in and can be classified as histidine triad proteins, choline binding proteins, adhesins, proteins involved in the degradation of the extracellular matrix, transporters, stress proteins, proteins involved in various physiological processes, and hypothetical proteins.
Table 1. Identification of pneumococcal proteins that are immunogenic in humans.
Selection of an immunogenic pneumococcal protein
Out of the immunogenic pneumococcal proteins, a selection was made based on the following criteria: i) serotype-independent, ii) present on the bacterial surface, and iii) no homology with human proteins. Seven out of the 26 identified immunogenic proteins fulfilled these criteria: PsaA, ORF_0082, FBA, SP1683, SP1386, ManL, and SP0562. PsaA, ORF_0082 and FBA are adhesins helping the pneumococcus for the colonization of the upper airways. ManL, SP1386 and SP1683 are part of transporters important for the import of essential nutrients. SP0562 has been identified as a conserved hypothetical protein. PsaA has been evaluated in a clinical trialCitation15 and is part of a United States Patent Application (20090252756, Mizrachi-Nebenzahl, Yaffa, Beer Sheva, Israel). ORF_0082 has been reported not to be protective in animal study.Citation16 The polyamine transport protein PotD (SP1386) plays a role in pneumococcal pathogenesis and immunity.Citation17 Immunization with PotD (SP1386) protects CBA/N mice against systemic infection and nasopharyngeal colonization with S. pneumoniae.Citation18 The function of the hypothetical protein SP0562 is unknown. SP1683 and ManL are involved in sugar transport and have previously not been studied as vaccine candidates. We first focused on SP1683.
BLAST analysis revealed that SP1683 was conserved in a representative panel of 14 strains (serotypes 1, 2, 3, 4, 5, 6A, 6B, 10A, 11A, 14, 19A, 19F, 23F, and 35F) with more than 98% of identity. A signal peptide was predicted with a low score, but a PROSITE motif of “Prokaryotic membrane lipoprotein attachment site” was detected. Moreover, a “Bacterial extracellular solute-binding protein” domain (“SBP_bac_1”, domain found in bacterial extracellular solute-binding protein family) was identified. Taken together, these observations indicate that SP1683 protein is probably found at the bacterial cell surface. No similarity with any human protein was found.
Hence, we selected SP1683 as a potential vaccine antigen candidate for it being serotype-independent, present on the bacterial surface and showing no homology with any human protein. A recombinant version of the protein was made. The identity of the recombinant protein was confirmed by matrix-assisted laser desorption ionization-time-of-flight/time-of-flight (MALDI-TOF/TOF) mass spectrometry on two-dimensional gel electrophoresis (2D-GE) gel spots.Citation19 In addition, we could show by sodium dodecylsulphate polyacrylamide gel electrophoresis and immunoblotting that recombinant SP1683 was recognized by patients'sera and by serum from a SCID mouse transferred with human PBMCs after heat-inactivated S. pneumoniae serotype 3 vaccination. As the antibodies present in these human sera were elicited upon contact with the native SP1683, the results indicate that the recombinant SP1683 is probably immunologically similar to the native molecule.
Lethal challenge
Next, we evaluated whether immunization with SP1683 is effective to protect against invasive disease in a murine invasive pneumococcal infection model. We used polyhistidine triad protein D (PhtD) as a control protein, as this protein has already been shown to be protective in animal models.Citation20-22 Mice were immunized with adjuvanted PhtD or SP1683. On day 27, anti-PhtD and anti-SP1683 geometric mean IgG antibody concentrations were 2215 µg/mL and 122 µg/mL, respectively. After challenge with the S. pneumoniae 4/CDC strain, mouse survival was recorded over the following 10 days (). The results indicated that immunization with PhtD allowed the survival of 14/20 mice (70%), as seen after 10 days, while all animals in the control group had already died after five days. When immunized with SP1683, only 2/20 mice (10%) survived the challenge with 4/CDC strain. Challenge with 3/43 strain gave similar results (). When immunized with PhtD, the survival rate after 10 days was 75% (15/20 mice). It was 5% (1/20 mice) for both SP1683 and control groups. Mean anti-PhtD and anti-SP1683 IgG concentrations in this second challenge experiment were 1391 µg/mL and 94.5 µg/mL, respectively.
Figure 1. Mouse survival upon intranasal challenge with pneumococcal strain 4/CDC. Mice (n = 20/group) were immunized twice intramuscularly at a two-week interval with AS01 alone (control), 3 µg PhtD or SP1683 adjuvanted with AS01. Fourteen days after the second injection, mice were challenged intranasally with 5 × 106 cfu of S. pneumoniae type 4/CDC. The mortality was recorded during 10 days.
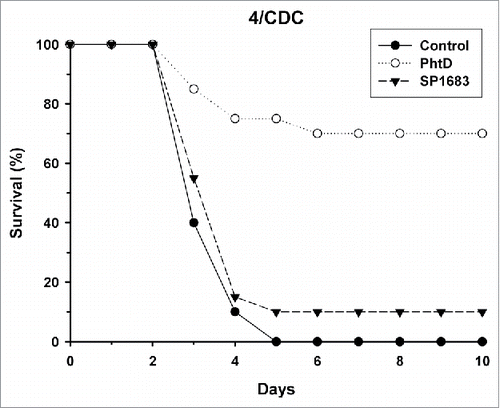
Figure 2. Mouse survival upon intranasal challenge with pneumococcal strain 3/43. Mice (n = 20/group) were immunized twice intramuscularly at a two-week interval with AS01 alone (control), 3 µg PhtD or SP1683 adjuvanted with AS01. Fourteen days after the second injection, mice were challenged intranasally with 1 × 105 cfu of S. pneumoniae type 3/43. The mortality was recorded during 10 days.
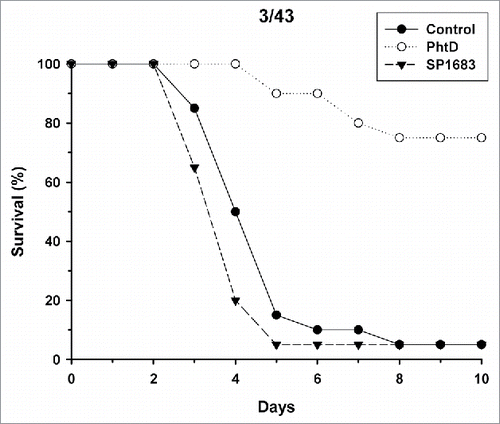
Nasopharyngeal colonisation
Next, we evaluated whether immunization with SP1683 was effective to protect against nasopharyngeal colonization. Again, PhtD was used as a control protein. Mice were immunized with PhtD or SP1683 through the intranasal route in the presence of E. coli heat-labile toxin (LT) and humoral immune responses were evaluated 13 days after the third immunization. In the PhtD group, all animals seroconverted with average anti-PhtD IgG antibody concentration being 352 [95%CI: 265-469] µg/mL. In the SP1683 group, all animals seroconverted with average anti-SP1683 IgG concentration being 59.1 [95%CI: 37-94] µg/mL. To assess the protective activity of PhtD and SP1683 immunization against nasopharyngeal carriage, mice were challenged intranasally with the 2D39 strain. As can be seen in , immunizations with PhtD (P < 0.001 against control on Day 6) and with SP1683 (P < 0.05 against control on Day 6) both protected against 2D39 nasopharyngeal colonisation, but the protection afforded by PhtD was higher than that afforded by SP1683. In another experiment, anti-PhtD and anti-SP1683 antibody concentrations were 331 [95%CI: 165-661] µg/mL and 54.7 [95%CI: 36-83] µg/mL, respectively. After challenge with 6B/CDC strain, both groups performed in a similar way (P < 0.01 against control on Day 3) ().
Figure 3. Vaccine efficacy in a S. pneumoniae nasopharyngeal colonization model. Mice were immunized intranasally with adjuvanted PhtD, SP1683 or adjuvant alone (control) before they were intranasally challenged with pneumococcal strain 2D39. Bacterial colonies in nasal washings were counted on Day 2 and Day 6 post-challenge and expressed as log10 mean cfu. Each dot represents a mouse. Dashed lines indicate the limit of detection; Black horizontal bars are means. Statistical analyses were carried out per day with ANOVA. All significant differences, compared with the control, are shown. *, P < 0.05; ***, P < 0.001; N.S., not significant.
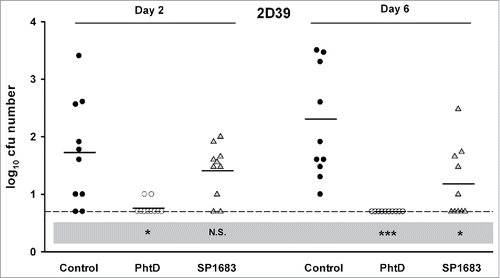
Figure 4. Vaccine efficacy in a S. pneumoniae nasopharyngeal colonization model. Mice were immunized intranasally with adjuvanted PhtD, SP1683 or adjuvant alone (control) before they were intranasally challenged with pneumococcal strain 6B/CDC. Bacterial colonies in nasal washings were counted on Day 3 post-challenge and expressed as log10 mean cfu. Each dot represents a mouse. Dashed lines indicate the limit of detection; Black horizontal bars are means. Statistical analyses were carried out with ANOVA. All significant differences, compared with the control, are shown. **, P < 0.01.
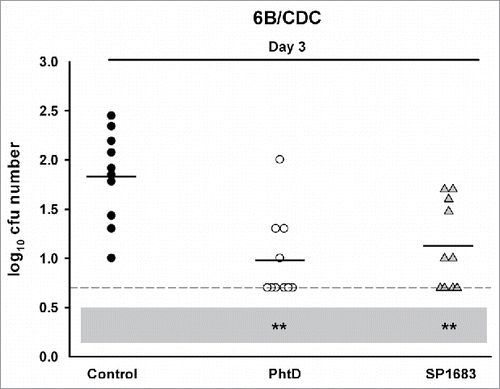
Protection from nasopharyngeal colonization by S. pneumoniae is IL17-dependent
IL17 has been implicated in adaptive immunity to colonization by S. pneumoniae.Citation23,Citation24 The role of IL17 in the protection elicited by the immunizations was analysed by treating mice previously immunized with PhtD + detoxified pneumolysin (dPly) or SP1683 with anti-IL17 antibody to inhibit the IL17 signalling pathway. Mice were immunized with PhtD/dPly or SP1683, both adjuvanted with LT, through the intranasal route. On Day 41, seroconversion was 100% in all groups. Anti-PhtD, anti-dPly and anti-SP1683 IgG concentrations were 131 [95%CI: 83-208] µg/mL, 530 [95%CI: 418-673] and 453 [95%CI: 340-604] µg/mL, respectively, for the groups that were not treated with anti-IL17 antibody, and 156 [95%CI: 97-250] µg/mL, 505 [95%CI: 396-644] and 442 [95%CI: 296-659] µg/mL, respectively, for the groups that were treated with anti-IL17 antibody. Results showed that anti-IL17 antibody treatment at the time of 2D/39 challenge was able to inhibit the immune response against the combined PhtD and dPly formulation and against SP1683, allowing colonization of the nasopharynx ().
Figure 5. Vaccine efficacy in a S. pneumoniae nasopharyngeal colonization model. Mice were immunized intranasally with adjuvanted PhtD/dPly, SP1683 or adjuvant alone (control) on Days 0, 14 and 28 before they were intranasally challenged with pneumococcal strain 2D39 on Day 42. Mice were treated or not with anti-IL17A and anti-IL17F antibodies on Days 41, 43 and 45. Bacterial colonies in nasal washings were counted on Day 2 and 4 post-challenge and expressed as log10 mean cfu. Each dot represents a mouse. Dashed lines indicate the limit of detection. Dark grey horizontal bars are means. Statistical analyses were carried out with ANOVA2. Groups were compared to each other over time. Groups SP1683 and PhtD/dPly versus control group: P < 0.001. Group PhtD/dPly versus group Phtd/dPly + anti-IL17: P < 0.001. Group SP1683 versus SP1683 + anti-IL17, P = 0.053.
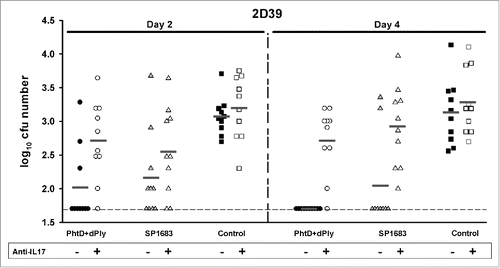
Discussion
We have identified several immunogenic pneumococcal proteins by using a humanized SCID/SCID mouse model. Many of them had previously been identified as immunogenic in humans with other methods (see ), which supports our immuno-proteomics approach. Other pneumococcal proteins identified in this study have previously been reported to be immunogenic or protective in different mouse models, or have been described to contribute to pneumococcal virulence in mice (see ). However, we have also identified new immunogenic proteins, such as SP1683, PTS system IIA component (maltose/maltodextrin, ManL), pyruvate kinase, and hypothetical proteins (SP1290 and SP0562). Selected out of the newly identified immunogenic bacterial proteins, SP1683 fulfilled the following criteria: i) potentially serotype-independent, based on the analysis of 14 strains ii) likely present on the bacterial surface, based on genetic sequence data iii) no homology with human proteins, and iv) not previously evaluated as a vaccine candidate.
SP1683 is one of the components of the ABC transporter specific for sialic acid (referred to as satABC).Citation25 SP1683 has been predicted to be the sialic acid-binding component, while the two other proteins of satABC, SP1682 and SP1681 were predicted to be permease components.Citation25 Free sialic acid is not readily available in the host upper airways but is a common terminal modification on N- and O-linked human glycoproteins that can be cleaved by the pneumococcal exoglycosidases (sialidases).Citation25 Sialic acid is an important source of carbohydrates for the bacterium so that it was shown that the import of sialic acid by satABC contributes to pneumococcal colonization.Citation25 This was further demonstrated when intranasal inoculation of mutant strains deficient in satABC led to reduced colonization compared with wild strain.Citation25 These data reinforced our choice of evaluating SP1683 as vaccine antigen.
We first evaluated the protective capacity of anti-SP1683 immune responses in mice by using both an invasive pneumococcal infection model and a colonization model. The histidine triad protein PhtDCitation20,Citation22,Citation26 was used as a positive control antigen. PhtD is a promising pneumococcal vaccine antigen candidate at the forefront of protein-based vaccine development, currently evaluated in humans in combination with dPly, another vaccine antigen candidate.Citation27,Citation28 Our data confirmed previous data showing that immunization with PhtD (i) elicits a strong antibody response, (ii) controls nasopharyngeal colonisation, and (iii) protects against lethal challenge. In contrast, systemic immunization with SP1683 elicited a weak antibody response and did not protect against lethal challenge. Absence of protection may be due to the low level of elicited antibodies or to non-functional antibodies, which was not investigated further.
In another mouse model, using the intranasal route of administration, we showed that immunization with SP1683 was able to protect against nasopharyngeal colonization, although anti-SP1683 IgG levels were again lower than those induced by immunization with PhtD. This result was important, as bacterial colonization of the nasopharynx is a necessary step before S. pneumoniae infection takes place, hence protection against colonization would help to contain pneumococcal spread and limit or impede pneumococcal disease.Citation29 In our study, some animals were still colonized by the pathogen at the latest time points investigated; indicating that a longer time may have been needed for these animals to clear the infection. Or, although all mice seroconverted after immunization, it may be that some simply are not able to clear the colonization. However, this observation does not question the validity of the experiment, as sterilizing immunity is not a goal in such type of experiments. A significant reduction in bacterial load at a given time point is sufficient to conclude about the protective effect of the injected antigen. Indeed, the clinical relevance of an incomplete protection against colonization in a mouse model is unknown, considering the differences between a challenge in mice with a defined and certainly exaggerated amount of bacterium and the occurrence of a natural pneumococcal infection in human.
In mice, there is evidence that Th17 CD4+ T cells play an essential role in the development of natural protective immunity against pneumococcal nasopharyngeal colonization.Citation30 More recently, Moffitt et al.Citation31 identified a number of Th17 antigens from killed whole cell vaccines that were protective against serotype 6B nasopharyngeal colonization as mucosal vaccines. Th17 CD4+ cell-mediated protection against nasopharyngeal colonization may occur as a consequence of IL17A-induced recruitment of monocyte/macrophage to the site of colonization and potentiation of pneumococcal killing by innate cells.Citation24,Citation32 In our model, depletion of IL17 abolished the protection against colonization that is otherwise induced by SP1683 or PhtD+dPly immunization, suggesting an important role of IL17 in protein-mediated immunity against pneumococcal nasopharyngeal colonization. Considering the importance of nasopharyngeal colonization as a precursor to non-invasive and invasive pneumococcal disease,Citation29 a Th17-mediated first line defence is considered crucial to impede or stop pneumococcal infections. Therefore, generating vaccine-induced, protective IL17A immune responses that prevent nasopharyngeal colonization is desirable (reviewed in refCitation33).
In summary, in our experimental conditions, SP1683 did not fulfil all requirements to be considered as a potential isolated pneumococcal vaccine antigen. Nevertheless, we showed that it can elicit IL17-dependent protection against nasopharyngeal colonization, in the same way as the previously described PhtD, and as such could be used as a first line of defence in combined immunizations.
Materials & methods
Transferring PBMCs to SCID mice
Peripheral blood buffy coat from healthy blood donors was obtained from the Blood Transfusion Centre of the Red Cross Leuven. Human PBMCs were prepared by density gradient centrifugation on Lymphoprep (Axis-shield Poc AS) and analyzed by flow cytometry (BD Biosciences). One day before transferring human PBMCs, the SCID/SCID mice received TMβ1 by intraperitoneal (i.p.) injection. TMβ1 is a rat monoclonal antibody recognizing the mouse IL-2 receptor beta-chain, aiming to improve the survival and functionality of the transplant.Citation34 PBMCs were dissolved in phosphate-buffered saline and injected i.p. into the SCID/SCID mice (7 × 107 cells/mouse). At the same time, the mice were immunized i.p. with 2 × 108 CFU heat-killed S. pneumoniae serotype 3. Fourteen days later, blood was drawn by heart puncture in isoflurane-anesthetized mice. Mice were euthanized by cervical dislocation after isoflurane inhalation.
2D-GE and Western blotting
A S. pneumoniae serotype 3 extract was prepared by sonication as described by Encheva et al.Citation35 Non-protein impurities from the S. pneumoniae serotype 3 extract were removed by using the 2-D clean-Up kit. Immobilized pH gradient (IPG) strips ranging from pH 4 to 7 were rehydrated overnight using 250 µg of the S. pneumoniae serotype 3 protein extract. After one dimensional isoelectric focusing on a Multiphor II Electrophoresis System according to the manufacturer's instructions, the IPG strips were equilibrated in solution I [0.05 M Tris-HCl, pH 6.8 containing 6 M urea, 35 mM SDS, 30% (v/v) glycerol and 0.25% (w/v) dithiotreitol] and solution II [0.05 M Tris-HCl, pH 6.8 containing 6 M urea, 35 mM SDS, 30% (v/v) glycerol, 0.45% (v/v) iodoacetamide and bromophenol blue] for 15 min each. Equilibrated strips were loaded on a 12.5% SDS polyacrylamide gel for separation in the second dimension, according to the manufacturer's instructions. After run, the proteins were either transferred on a polyvinylidene difluoride membrane by electroblotting using a NovaBlot apparatus or visualized by Coomassie Blue staining. Membranes were consecutively treated with 5% (w/v) bovine serum albumin for 1 h, humanized SCID mice serum (dilution 1:250) overnight, goat anti-human IgG antibodies (dilution 1:5000) for 1 h, and horseradish peroxidase-conjugated rabbit anti-goat IgG antibodies (dilution 1:5000) for 45 min. All antibodies were diluted in Tris saline buffer (TSB) containing 10 mM Tris-HCl, 150 mM NaCl and 0.1% Triton; pH 7.6. Intermittent washing steps were performed in TSB (3 × 10 min). Finally, 0.7 mM 3.3’diamino-benzidinetetrahydrochloride containing 0.1% H2O2 in TBS was added as a substrate to detect protein-antibody interactions and incubated for 5 min. Coomassie Blue-stained gels were used to excise the corresponding visualized spots. 2D-GE and Western blotting experiments were performed in triplet to confirm the identity of the excised protein spots.
Protein identification by mass spectrometry
Gel spots containing the protein of interest were washed with HPLC-grade water, dried in a SpeedVac (Savant) and digested overnight at 37°C with 10 µl of 25 ng/µl trypsin (sequence grade) in 200 mM ammonium bicarbonate. The resulting peptide mixture was cleaned up through C18 resin and analyzed by MALDI-TOF/TOF mass spectrometry in the presence of α-cyano-4-hydroxycinnamic acid in an Applied Biosystems 4800 Proteomics Analyzer. Protein identifications were executed with the MASCOT (Matrix Science) search engine using Comprehensive Microbial Resource (CMR) (http://cmr.jcvi.org) S. pneumoniae databases.
Bioinformatics
All identified pneumococcal protein vaccine candidates were looked up in the “Comprehensive Microbial Resource” (CMR) (http://cmr.jcvi.org) database. This database contains information on genes of S. pneumoniae serotype 4, R6 and G54. The “National Centre for Biotechnology Information” (NCBI) Gene databank was also consulted. The Expert Protein Analysis System (ExPASy) (http;//www.expasy.org) was used to obtain information on the protein structure. The “Protein Family Database” (Pfam) (http://pfam.sanger.ac.uk) was used to document the functional domains, the conserved binding domains, and the membrane anchoring domains.
To determine the SP1683 gene (strain ATCC BAA-334 / TIGR4) conservation across serotypes, the Basic Local Alignment Tool (BLAST) from the National Centre for Biotechnology information (NCBI) and the Universal Protein Resource Uniprot were used. Fourteen serotypes (1, 2, 3, 4, 5, 6A, 6B, 10A, 11A, 14, 19A, 19F, 23F, and 35F) were analyzed.
To predict the localization of SP1683, the SignalP 4.1 server (http://www.cbs.dtu.dk/services/SignalP/) has been used for signal peptide prediction. Moreover, a research of functional domain/motifs using the “Protein Family Database” (Pfam) (http://pfam.xfam.org/) and PROSITE (http://prosite.expasy.org/) was also performed on SP1683.
Antigens
Pneumococcal SP1683 protein was recombinantly produced as follows: ORF1683 (from TIGR4 strain) was cloned into pDest17 vector via Gateway system (Invitrogen) and transfected to Krx Escherichia coli. After induction of expression by isopropyl β-D-1-thiogalactopyranoside (1 mM), E. coli were cultured for 6 h at 37°C. The recombinant protein was harvested by centrifugation (4°C, 10 min, 8000 × g) and purified via its polyhistidine tail through a nickel-nitrilotriacetic acid purification system (Invitrogen), following the instructions of the manufacturer.
PhtD.Citation22,Citation36 was cloned from the N4 strain (serotype 4) and produced in E. coli. The protein was purified from bacterial lysate through multiple chromatography steps.
Pneumolysin was cloned from the 6B 493/73 strain, produced in E. coli and also purified from bacterial lysate through multiple chromatography steps. Further, pneumolysin was detoxified by formaldehyde treatment to obtain dPly.Citation37 Detoxification was ascertained by the absence of residual hemolytic activity in vitro, the absence of local reactogenicity after intramuscular injection in rats and the absence of in vivo toxicity after intranasal challenge in mice.
ELISA
Briefly, 96-well microtiter plates (Maxisorp, Nunc, Denmark) were coated for 2 h at 37°C with PhtD (1 µg/mL), SP1683 (1 µg/mL) or native Ply (8 µg/mL) in phosphate-buffered saline (PBS). After washing, serial two-fold dilutions of tested samples and one calibrated reference serum (in PBS-Tween-20 0.05%, PBST) were added to the wells. After incubation for 30 min at room temperature (RT) and washing, peroxidase-labelled anti-mouse IgG antibodies (Jackson 115-035-003) were added at a dilution of 1/2500 for 30 min incubation at RT. Plates were washed and then revealed by the addition of O-phenylenediamine dihydrochloride in citrate buffer 0.1 M (pH 4.5), in the presence of hydrogen peroxide. The reaction was stopped after 15 min by the addition of HCl 1 N and the optical density was read at 490 nm (620 nm for the reference filter) in a microtiter plate reader. The individual IgG concentrations (expressed as µg/ml) were calculated by the 4-parameter method using the Soft Max Pro software.
Anti-caps-PS antibodies were detected as previously described.Citation38
Lethal challenge model with type 4/CDC and 3/43 strains
MF1 female mice (4 weeks-old; n = 20/group) were immunized intramuscularly on days 0 and 14 with 2 doses of 3 µg PhtD or SP1683 formulated with AS01 (GSK, Rixensart, Belgium), which is a liposome-based Adjuvant System containing 3-O-desacyl-4’- monophosphoryl lipid A (MPL; GSK), and QS-21 (Quillaja saponaria Molina, fraction 21; licenced by GSK from Antigenics LLC, a wholly owned subsidiary of Agenus Inc, a Delaware, USA corporation). Control mice were injected with AS01 alone. Sera were taken on day 27 (13 days after last immunisation) and two serum pools/group were made to determine humoral responses. On day 28, mice were challenged intranasally with 5 × 106 cfu of S. pneumoniae type 4/CDC (DS2382-94)Citation39 or 1 × 105 cfu of S. pneumoniae type 3/43. Mortality was recorded during 10 days.
Nasopharyngeal colonization model with type 6B/CDC and 2D39 strains
Four weeks-old Balb/c mice (n = 10/group) were intranasally immunized on days 0, 14 and 28 with 2.5 µg of PhtD or SP1683 protein, adjuvanted with LT (except at the third immunization). Control animals received LT alone. Sera were collected on Day 41. The mice were challenged on day 42 with 2 × 105 cfu of the S. pneumoniae type 6B/CDC or type 2D39 strain, both heterologous for PhtD and SP1683. The bacterial load was measured in nasal washes collected 2 and 6 days post-challenge (with a total of 10 mice per group and per time-point, N = 30) for the S. pneumoniae type 2D39 strain and 3 days post-challenge (with a total of 10 mice per group, N = 30) for the type 6B/CDC. For that, the log10 weighted mean number of CFU/10 µl was determined by counting the colonies grown on Columbia agar 5% horse blood plates after plating 10 µl of 4 ten-fold serial dilutions of the washing. The arithmetic mean of the log10 weighted mean number of CFU/10 µl and the standard deviations were calculated for each group.
IL17 depletion experiment in the mouse nasopharyngeal colonization model
Four weeks-old Balb/c mice were intranasally immunized on days 0, 14 and 28 with 3 µg of PhtD/dPly (used as a positive control) or SP1683, adjuvanted with LT except at the third immunization. Control animals received LT alone. The mice were challenged on day 42 with 2 × 105 cfu of the S. pneumoniae type 2D39 strain, heterologous for PhtD, dPly and SP1683. Mice were treated or not with 100 µg anti-IL17A and 100 µg anti-IL17F monoclonal antibodies given through the intraperitoneal route on days 41, 43 and 45. The bacterial load was measured in nasal washings collected 2 and 4 days post-challenge. Anti-IL17A and anti-IL17F antibodies were obtained from the Ludwig Institute for Cancer Research, Brussels.
Disclaimer
Animal studies were carried out at GSK (Rixensart, Belgium) or at the KU Leuven in accordance with European Directive 2010/63, and the GSK Policy on the Care, Welfare and Treatment of Animals. Approval for the study was granted by the respective local ethics committees.
Disclosure of potential conflicts of interest
All authors have declared the following interests: PH, CY and FG are, or were at the time of the study, employees of the GSK group of companies. PH and FG report ownership of GSK shares and/or restricted GSK shares. PH, CY and FG are listed as inventors on patents owned by the GSK group of companies. LM, GW, XB, RD, EW and TW report no financial conflicts of interest.
Author contributions
LM, PH, FG, XB were involved in the conception and design of the study. LM, TW, PH, GW, RD, EW, CY acquired the data. LM, PH, TW, GW, TD, FG and XB analyzed and interpreted the results. All authors were involved in drafting the manuscript or revising it critically for important intellectual content. All authors had full access to the data and approved the manuscript before it was submitted by the corresponding author.
Acknowledgments
The authors thank Jacques Van Snick and Catherine Uyttenhove from the Ludwig institute for Cancer Research, Brussels for providing Anti-IL17A and anti-IL17F antibodies, Cindy Castado and Steve Labbé (GSK, Belgium) for bioinformatics analyses, Sabine Reyter, Annie Dumont, André De Groote, Mélanie De Wilde and François Galletto (GSK, Belgium) for technical support, Pascal Cadot (Xpe-Pharma & Science for GSK) for editorial assistance and Ulrike Krause (GSK, Belgium) for coordinating manuscript development.
Additional information
Funding
References
- World Health Organization. Pneumococcal vaccines WHO position paper. 2012. http://www.who.int/wer/2012/wer8714.pdf?ua = 1.
- Smart LE, Dougall AJ, Girdwood RWA. New 23-valent pneumococcal vaccine in relation to pneumococcal serotypes in systemic and non-systemic disease. J Infect. 1987;14:209–15. doi:10.1016/S0163-4453(87)93360-3.
- Advisory Committee on Immunization Practices. Prevention of pneumococcal disease: recommendations of the Advisory Committee on Immunization Practices (ACIP). MMWR Recomm Rep. 1997;46:1–24.
- van der Poll T, Opal SM. Pathogenesis, treatment, and prevention of pneumococcal pneumonia. Lancet. 2009;374:1543–56. doi:10.1016/S0140-6736(09)61114-4.
- Koskela M, Leinonen M, Häivä VM, Timonen M, Mäkelä PH. First and second dose antibody responses to pneumococcal polysaccharide vaccine in infants. Pediatr Infect Dis. 1986;5:45–50. doi:10.1097/00006454-198601000-00009.
- Bogaert D, Hermans PWM, Adrian PV, Rümke HC, de Groot R. Pneumococcal vaccines: an update on current strategies. Vaccine. 2004;22:2209–20. doi:10.1016/j.vaccine.2003.11.038.
- Black S, Shinefield H, Fireman B, Lewis E, Ray P, Hansen JR, Elvin L, Ensor KM, Hackell J, Siber G, et al. Efficacy, safety and immunogenicity of heptavalent pneumococcal conjugate vaccine in children. Northern California Kaiser Permanente Vaccine Study Center Group. Pediatr Infect Dis J. 2000;19:187–95.
- Prymula R, Peeters P, Chrobok V, Kriz P, Novakova E, Kaliskova E, Kohl I, Lommel P, Poolman J, Prieels J-P, et al. Pneumococcal capsular polysaccharides conjugated to protein D for prevention of acute otitis media caused by both Streptococcus pneumoniae and non-typable Haemophilus influenzae: a randomised double-blind efficacy study. Lancet. 2006;367:740–8. doi:10.1016/S0140-6736(06)68304-9.
- Deceuninck G, De Serres G, Boulianne N, Lefebvre B, De Wals P. Effectiveness of three pneumococcal conjugate vaccines to prevent invasive pneumococcal disease in Quebec, Canada. Vaccine. 2015;33:2684–9. doi:10.1016/j.vaccine.2015.04.005.
- Lu CY, Huang LM. Is Pneumococcal Serotype Replacement Impending? Pediatr Neonatol. 2016;57:363–4. doi:10.1016/j.pedneo.2015.08.012.
- Bittaye M, Cash P. Streptococcus pneumoniae proteomics: determinants of pathogenesis and vaccine development. Expert Rev Proteomics. 2015;12:607–21. doi:10.1586/14789450.2015.1108844.
- Barocchi MA, Censini S, Rappuoli R. Vaccines in the era of genomics: the pneumococcal challenge. Vaccine. 2007;25:2963–73. doi:10.1016/j.vaccine.2007.01.065.
- Feldman C, Anderson R. Review: Current and new generation pneumococcal vaccines. J Infect. 2014;69:309–25. doi:10.1016/j.jinf.2014.06.006.
- Pichichero ME, Khan MN, Xu Q. Next generation protein based Streptococcus pneumoniae vaccines. Hum Vaccin Immunother. 2016;12:194–205. doi:10.1080/21645515.2015.1052198.
- Rajam G, Anderton JM, Carlone GM, Sampson JS, Ades EW. Pneumococcal surface adhesin A (PsaA): a review. Crit Rev Microbiol. 2008;34:163–73. doi:10.1080/10408410802383610.
- Giefing C, Meinke AL, Hanner M, Henics T, Minh DB, Gelbmann D, Lundberg U, Senn BM, Schunn M, Habel A, et al. Discovery of a novel class of highly conserved vaccine antigens using genomic scale antigenic fingerprinting of pneumococcus with human antibodies. J Exp Med. 2008;205:117–31. doi:10.1084/jem.20071168.
- Shah P, Swiatlo E. Immunization with polyamine transport protein PotD protects mice against systemic infection with Streptococcus pneumoniae. Infect Immun. 2006;74:5888–92. doi:10.1128/IAI.00553-06.
- Shah P, Briles DE, King J, Hale Y, Swiatlo E. Mucosal immunization with polyamine transport protein D (PotD) protects mice against nasopharyngeal colonization with Streptococcus pneumoniae. Exp Biol Med (Maywood). 2009;234:403–9. doi:10.3181/0809-RM-269.
- Perkins DN, Pappin DJ, Creasy DM, Cottrell JS. Probability-based protein identification by searching sequence databases using mass spectrometry data. Electrophoresis. 1999;20:3551–67. doi:10.1002/(SICI)1522-2683(19991201)20:18%3c3551::AID-ELPS3551%3e3.0.CO;2-2.
- Adamou JE, Heinrichs JH, Erwin AL, Walsh W, Gayle T, Dormitzer M, Dagan R, Brewah YA, Barren P, Lathigra R, et al. Identification and characterization of a novel family of pneumococcal proteins that are protective against sepsis. Infect Immun. 2001;69:949–58. doi:10.1128/IAI.69.2.949-958.2001.
- Denoël P, Philipp MT, Doyle L, Martin D, Carletti G, Poolman JT. A protein-based pneumococcal vaccine protects rhesus macaques from pneumonia after experimental infection with Streptococcus pneumoniae. Vaccine. 2011;29:5495–501. doi:10.1016/j.vaccine.2011.05.051.
- Godfroid F, Hermand P, Verlant V, Denoël P, Poolman JT. Preclinical evaluation of the Pht proteins as potential cross-protective pneumococcal vaccine antigens. Infect Immun. 2011;79:238–45. doi:10.1128/IAI.00378-10.
- Moffitt KL, Gierahn TM, Lu YJ, Gouveia P, Alderson M, Flechtner JB, Higgins DE, Malley R. TH17-based vaccine design for prevention of Streptococcus pneumoniae colonization. Cell Host Microbe. 2011;9:158–65. doi:10.1016/j.chom.2011.01.007.
- Zhang Z, Clarke TB, Weiser JN. Cellular effectors mediating Th17-dependent clearance of pneumococcal colonization in mice. J Clin Invest. 2009;119:1899–909.
- Marion C, Burnaugh AM, Woodiga SA, King SJ. Sialic acid transport contributes to pneumococcal colonization. Infect Immun. 2011;79:1262–9. doi:10.1128/IAI.00832-10.
- Leroux-Roels I, Devaster JM, Leroux-Roels G, Verlant V, Henckaerts I, Moris P, Hermand P, Van Belle P, Poolman JT, Vandepapeliere P, et al. Adjuvant system AS02v enhances humoral and cellular immune responses to pneumococcal protein PhtD vaccine in healthy young and older adults: randomised, controlled trials. Vaccine. 2015;33:577–84. doi:10.1016/j.vaccine.2013.10.052.
- Leroux-Roels G, Maes C, De Boever F, Traskine M, Rüggeberg JU, Borys D. Safety, reactogenicity and immunogenicity of a novel pneumococcal protein-based vaccine in adults: a phase I/II randomized clinical study. Vaccine. 2014;32:6838–46. doi:10.1016/j.vaccine.2014.02.052.
- Prymula R, Pazdiora P, Traskine M, Rüggeberg JU, Borys D. Safety and immunogenicity of an investigational vaccine containing two common pneumococcal proteins in toddlers: a phase II randomized clinical trial. Vaccine. 2014;32:3025–34. doi:10.1016/j.vaccine.2014.03.066.
- Bogaert D, de Groot R, Hermans PWM. Streptococcus pneumoniae colonisation: the key to pneumococcal disease. Lancet Infect Dis. 2004;4:144–54. doi:10.1016/S1473-3099(04)00938-7.
- Malley R, Trzcinski K, Srivastava A, Thompson CM, Anderson PW, Lipsitch M. CD4+ T cells mediate antibody-independent acquired immunity to pneumococcal colonization. Proc Natl Acad Sci U S A. 2005;102:4848–53. doi:10.1073/pnas.0501254102.
- Moffitt KL, Malley R, Lu YJ. Identification of protective pneumococcal TH17 antigens from the soluble fraction of a killed whole cell vaccine. PLoS One. 2012;7:e43445. doi:10.1371/journal.pone.0043445.
- Lu YJ, Gross J, Bogaert D, Finn A, Bagrade L, Zhang Q, Kolls JK, Srivastava A, Lundgren A, Forte S, et al. Interleukin-17A mediates acquired immunity to pneumococcal colonization. PLoS Pathog. 2008;4:e1000159. doi:10.1371/journal.ppat.1000159.
- Khan MN, Pichichero ME. The host immune dynamics of pneumococcal colonization: implications for novel vaccine development. Hum Vaccin Immunother. 2014;10:3688–99. doi:10.4161/21645515.2014.979631.
- Tournoy KG, Depraetere S, Meuleman P, Leroux-Roels G, Pauwels RA. Murine IL-2 receptor beta chain blockade improves human leukocyte engraftment in SCID mice. Eur J Immunol. 1998;28:3221–30. doi:10.1002/(SICI)1521-4141(199810)28:10%3c3221::AID-IMMU3221%3e3.0.CO;2-S.
- Encheva V, Gharbia SE, Wait R, Begum S, Shah HN. Comparison of extraction procedures for proteome analysis of Streptococcus pneumoniae and a basic reference map. Proteomics. 2006;6:3306–17. doi:10.1002/pmic.200500744.
- Rioux S, Neyt C, Di Paolo E, Turpin L, Charland N, Labbé S, Mortier M-C, Mitchell TJ, Feron C, Martin D, et al. Transcriptional regulation, occurrence and putative role of the Pht family of Streptococcus pneumoniae. Microbiology. 2011;157:335–48. doi:10.1099/mic.0.042184-0.
- Hermand P, Vandercammen A, Mertens E, Di Paolo E, Verlant V, Denoël P, Godfroid F. Preclinical evaluation of a chemically detoxified pneumolysin as pneumococcal vaccine antigen. Hum Vaccin Immunother. 2017;13:220–8 doi:10.1080/21645515.2016.1234553.
- Moens L, Wuyts M, Meyts I, De Boeck K, Bossuyt X. Human memory B lymphocyte subsets fulfill distinct roles in the anti-polysaccharide and anti-protein immune response. J Immunol. 2008;181:5306–12. doi:10.4049/jimmunol.181.8.5306.
- Romero-Steiner S, Libutti D, Pais LB, Dykes J, Anderson P, Whitin JC, Keyserling HL, Carlone GM. Standardization of an opsonophagocytic assay for the measurement of functional antibody activity against Streptococcus pneumoniae using differentiated HL-60 cells. Clin Diagn Lab Immunol. 1997;4:415–22.
- Zhang Y, Masi AW, Barniak V, Mountzouros K, Hostetter MK, Green BA. Recombinant PhpA protein, a unique histidine motif-containing protein from Streptococcus pneumoniae, protects mice against intranasal pneumococcal challenge. Infect Immun. 2001;69:3827–36. doi:10.1128/IAI.69.6.3827-3836.2001.
- Briles DE, Hollingshead SK, Paton JC, Ades EW, Novak L, van Ginkel FW, Benjamin WH, Jr. Immunizations with pneumococcal surface protein A and pneumolysin are protective against pneumonia in a murine model of pulmonary infection with Streptococcus pneumoniae. J Infect Dis. 2003;188:339–48. doi:10.1086/376571.
- Holmlund E, Quiambao B, Ollgren J, Nohynek H, Käyhty H. Development of natural antibodies to pneumococcal surface protein A, pneumococcal surface adhesin A and pneumolysin in Filipino pregnant women and their infants in relation to pneumococcal carriage. Vaccine. 2006;24:57–65. doi:10.1016/j.vaccine.2005.07.055.
- Baril L, Briles DE, Crozier P, King J, Punar M, Hollingshead SK, McCormick JB. Characterization of antibodies to PspA and PsaA in adults over 50 years of age with invasive pneumococcal disease. Vaccine. 2004;23:789–93. doi:10.1016/j.vaccine.2004.07.033.
- McCool TL, Cate TR, Moy G, Weiser JN. The immune response to pneumococcal proteins during experimental human carriage. J Exp Med. 2002;195:359–65. doi:10.1084/jem.20011576.
- Bologa M, Kamtchoua T, Hopfer R, Sheng X, Hicks B, Bixler G, Hou V, Pehlic V, Yuan T, Gurunathan S. Safety and immunogenicity of pneumococcal protein vaccine candidates: monovalent choline-binding protein A (PcpA) vaccine and bivalent PcpA-pneumococcal histidine triad protein D vaccine. Vaccine. 2012;30:7461–8. doi:10.1016/j.vaccine.2012.10.076.
- Brooks WA, Chang LJ, Sheng X, Hopfer R. Safety and immunogenicity of a trivalent recombinant PcpA, PhtD, and PlyD1 pneumococcal protein vaccine in adults, toddlers, and infants: A phase I randomized controlled study. Vaccine. 2015;33:4610–7. doi:10.1016/j.vaccine.2015.06.078.
- Briles DE, Hollingshead SK, King J, Swift A, Braun PA, Park MK, Ferguson LM, Nahm MH, Nabors GS. Immunization of humans with recombinant pneumococcal surface protein A (rPspA) elicits antibodies that passively protect mice from fatal infection with Streptococcus pneumoniae bearing heterologous PspA. J Infect Dis. 2000;182:1694–701. doi:10.1086/317602.
- Linder A, Hollingshead S, Janulczyk R, Christensson B, Akesson P. Human antibody response towards the pneumococcal surface proteins PspA and PspC during invasive pneumococcal infection. Vaccine. 2007;25:341–5. doi:10.1016/j.vaccine.2006.07.028.
- Seo JY, Seong SY, Ahn BY, Kwon IC, Chung H, Jeong SY. Cross-protective immunity of mice induced by oral immunization with pneumococcal surface adhesin a encapsulated in microspheres. Infect Immun. 2002;70:1143–9. doi:10.1128/IAI.70.3.1143-1149.2002.
- Ling E, Feldman G, Portnoi M, Dagan R, Overweg K, Mulholland F, Chalifa-Caspi V, Wells J, Mizrachi-Nebenzahl Y. Glycolytic enzymes associated with the cell surface of Streptococcus pneumoniae are antigenic in humans and elicit protective immune responses in the mouse. Clin Exp Immunol. 2004;138:290–8. doi:10.1111/j.1365-2249.2004.02628.x.
- Laine C, Mwangi T, Thompson CM, Obiero J, Lipsitch M, Scott JAG. Age-specific immunoglobulin g (IgG) and IgA to pneumococcal protein antigens in a population in coastal kenya. Infect Immun. 2004;72:3331–5. doi:10.1128/IAI.72.6.3331-3335.2004.
- Simell B, Kilpi T, Käyhty H. Subclass distribution of natural salivary IgA antibodies against pneumococcal capsular polysaccharide of type 14 and pneumococcal surface adhesin A (PsaA) in children. Clin Exp Immunol. 2006;143:543–9. doi:10.1111/j.1365-2249.2006.03009.x.
- Scott JA, Obiero J, Hall AJ, Marsh K. Validation of immunoglobulin G enzyme-linked immunosorbent assay for antibodies to pneumococcal surface adhesin A in the diagnosis of pneumococcal pneumonia among adults in Kenya. J Infect Dis. 2002;186:220–6. doi:10.1086/341205.
- Beghetto E, Gargano N, Ricci S, Garufi G, Peppoloni S, Montagnani F, Oggioni M, Pozzi G, Felici F. Discovery of novel Streptococcus pneumoniae antigens by screening a whole-genome λ-display library. FEMS Microbiol Lett. 2006;262:14–21. doi:10.1111/j.1574-6968.2006.00360.x.
- Wizemann TM, Heinrichs JH, Adamou JE, Erwin AL, Kunsch C, Choi GH, Barash SC, Rosen CA, Masure HR, Tuomanen E, et al. Use of a whole genome approach to identify vaccine molecules affording protection against Streptococcus pneumoniae infection. Infect Immun. 2001;69:1593–8. doi:10.1128/IAI.69.3.1593-1598.2001.
- Zysk G, Bongaerts RJM, ten Thoren E, Bethe G, Hakenbeck R, Heinz HP. Detection of 23 immunogenic pneumococcal proteins using convalescent-phase serum. Infect Immun. 2000;68:3740–3. doi:10.1128/IAI.68.6.3740-3743.2000.
- Bethe G, Nau R, Wellmer A, Hakenbeck R, Reinert RR, Heinz HP, Zysk G. The cell wall-associated serine protease PrtA: a highly conserved virulence factor of Streptococcus pneumoniae. FEMS Microbiol Lett. 2001;205:99–104. doi:10.1111/j.1574-6968.2001.tb10931.x.
- Whiting GC, Evans JT, Patel S, Gillespie SH. Purification of native alpha-enolase from Streptococcus pneumoniae that binds plasminogen and is immunogenic. J Med Microbiol. 2002;51:837–43. doi:10.1099/0022-1317-51-10-837.
- Hamel J, Martin D, Brodeur BB. Heat shock response of Streptococcus pneumoniae: identification of immunoreactive stress proteins. Microb Pathog. 1997;23:11–21. doi:10.1006/mpat.1996.0124.
- Kolberg J, Hòiby EA, Aase A, Sletten K, Rodal G, Michaelsen TE, Bucher A. Streptococcus pneumoniae heat shock protein 70 does not induce human antibody responses during infection. FEMS Immunol Med Microbiol. 2000;29:289–94. doi:10.1111/j.1574-695X.2000.tb01536.x.
- Cao J, Chen D, Xu W, Chen T, Xu S, Luo J, Zhao Q, Liu B, Wang D, Zhang X, et al. Enhanced protection against pneumococcal infection elicited by immunization with the combination of PspA, PspC, and ClpP. Vaccine. 2007;25:4996–5005. doi:10.1016/j.vaccine.2007.04.069.
- Kwon HY, Ogunniyi AD, Choi MH, Pyo SN, Rhee DK, Paton JC. The ClpP protease of Streptococcus pneumoniae modulates virulence gene expression and protects against fatal pneumococcal challenge. Infect Immun. 2004;72:5646–53. doi:10.1128/IAI.72.10.5646-5653.2004.