ABSTRACT
Botulinum neurotoxins (BoNTs) are potential biological weapons because of their high toxicity and mortality. Vaccination is an effective strategy to prevent botulism. The carboxyl-terminus of the heavy chain (Hc domain) is nontoxic and sufficient to generate protective immune responses against natural BoNTs in animals. To produce a vaccine suitable for human use, a recombinant non His-tagged isoform of the Hc domain of botulinum neurotoxin serotype B (BHc) was expressed in Escherichia coli and purified by sequential chromatography. The immunogenicity of recombinant E.coli-expressed BHc and the yeast-expressed mBHc antigens was explored and compared in Balb/c mice. BHc provided comparable protective potency but elicited significantly higher antibody titer and neutralization potency against BoNT/B after twice immunization, indicating that the recombinant BHc protein expressed in E.coli have better immunogenicity than the yeast-expressed mBHc. Moreover, a frequency and dose-dependent effect was observed in mice immunized with BHc subunit vaccine and the anti-BHc ELISA antibody titers correlated well with neutralizing antibody titers and protection potency. In summary, the Alhydrogel-formulated BHc subunit vaccine afforded effective protection against BoNT/B challenge. Therefore, the non-His-tagged and homogeneous BHc expressed in E.coli represents a good potential candidate subunit vaccine for human use.
Introduction
The botulinum neurotoxins (BoNTs), which are mainly produced by the Gram-positive, anaerobic bacterium Clostridium botulinum, are the most toxic proteins.Citation1–Citation4 BoNTs can be divided into seven serotypes (BoNT/A–BoNT/G), among which serotype A, B, E, and F are associated with human botulism.Citation1,Citation5,Citation6 The BoNTs are structurally similar (150 kDa). Each BoNT consists of a heavy chain (HC, 100 kDa) and a N-terminal catalytic light chain (LC, 50 kDa) linked by a single disulfide bond.Citation1,Citation6,Citation7 The carboxyl-terminus of the heavy chain (Hc) is responsible for neuro-specific binding to receptors on the peripheral nerve cell surface, and the amino-terminus of the heavy chain (HN) mediates translocation of the LC domain across the cell membrane.Citation8–Citation10 The LC of BoNT is a zinc-dependent metalloprotease that can cleave the SNARE protein and block neuromuscular transmission, resulting in skeletal muscle paralysis, respiratory failure, and eventually death.Citation2,Citation3,Citation5,Citation11,Citation12
BoNT is a potential biological weapon because of its high toxicity and mortality. Vaccination is an effective strategy to prevent botulinum.Citation5 The carboxyl-terminus of the HC (Hc domain) is nontoxic and sufficient to generate protective immune responses against natural BoNT in animals.Citation13–Citation15 Therefore, a novel strategy for prevention of botulinum toxin poisoning based on nontoxic recombinant Hc domain of BoNT is developed.Citation2,Citation4 The recombinant Hc antigen can be synthesized in large quantities using yeast or Escherichia coli expression systems.Citation16–Citation20 For vaccine development, E. coli offers additional advantages over Pichia pastoris, including cost-effectiveness and short production circle.Citation16 Most importantly, the soluble recombinant Hc proteins of BoNTs produced by intracellular expression in E. coli are non-glycosylated and biologically similar with the native structure of BoNTs, which render them more immunogenicity.Citation16,Citation21
In this study, a prokaryotic expression vector encoding the nontoxic Hc domain of BoNT/B (BHc) was constructed and soluble recombinant BHc antigen was highly expressed in E. coli and purified by sequential chromatography. The immunogenicity and protective efficacy of recombinant BHc protein were evaluated and compared with the yeast expressed mBHc (removing its single potential glycosylation site BHcN957Q).Citation21 Our results suggest that the recombinant BHc antigen provided comparable protective potency but elicited significantly higher ELISA antibody titer and neutralization potency against BoNT/B after twice immunization. Therefore, the non-His-tagged and homogeneous BHc expressed in E.coli represents a good potential candidate subunit vaccine for human use.
Results
Purification and analysis of recombinant BHc protein expressed in E. coli
The expression of recombinant BHc in E. coli (BL21) was visualized by SDS-PAGE as a major band of 50 kDa that demonstrated a high level of expression (). Small-scale purification of non His-tagged BHc was carried out using sequential chromatography. SDS–PAGE analysis of the BHc product showed approximately 95% purity after two steps (, lane 4) and the product (50 kDa) was identified by Western blot analysis (, lane 5). Final product yields ranged from 15 to 20 mg of purified protein per liter of culture.
Figure 1. Analysis of purified recombinant BHc protein during various purification steps by SDS-PAGE and western bolt. The soluble fraction and purified BHc were subjected to 10% SDS-PAGE and Western blot analysis using hyperimmune anti-neurotoxin B serum. Lane 1, pTIG-Trx vector transformed cell lysates; Lane 2, pTIG-Trx-BHc transformed BL21 cell lysates; Lane 3, MMC column purified products; Lane 4, Source 30s column purified products; Lane 5, Western blot analysis; M, protein markers 180, 130, 95, 72(red), 55, 43, 34, 26 and 17 KDa (from top to bottom); An arrow indicates the position of the BHc.
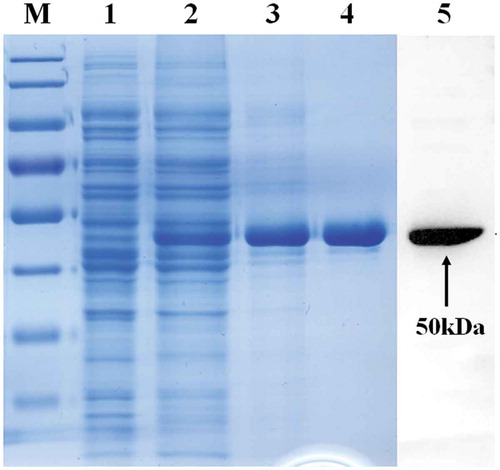
Immunogenicity of recombinant E.coli-expressed BHc and yeast-expressed mBHc antigens
In a previous study, a mBHc protein prepared in yeast was immunologically active and could confer effective protective immunity.Citation21 In this study, we evaluated and compared the immunogenicity of recombinant E.coli-expressed BHc and yeast-expressed mBHc antigens in Balb/c mice. Mice were vaccinated i.m. once or twice using two different doses of BHc, and mBHc formulated with aluminum hydroxide adjuvant. As shown in , a dose-dependent immune response to the BHc antigen was observed and anti-BHc ELISA antibody titer correlated well with protective potency against BoNT/B. Mice with single BHc vaccination had relatively low (3.08–3.11) geometric mean titers (GMT) and can be protected against 1000 LD50 of BoNT/B. After once boost immunizations, the group GMT was obviously increased (4.28–4.81) and those mice can be protected completely against 10,000 LD50 of BoNT/B.
Table 1. Survival, group antibody ELISA titers, and serum neutralization titers of mice after vaccination with BHc and mBHC antigens.
Compared with mBHc, BHc provided comparable protective potency but elicited significantly higher ELISA and neutralization antibody titer (P < 0.05) against BoNT/B after twice immunization. These results indicates that the recombinant BHc protein expressed in E.coli have better immunogenicity than the yeast-expressed mBHc.
Efficacy of BHc subunit vaccine against BoNT/B in mice
To further confirm the potency of the BHc subunit vaccine, we performed another efficacy study against BoNT/B. Mice were immunized i.m. once or twice with 0.0039, 0.0156, 0.0625, 0.25, 1 or 4 μg of recombinant BHc antigen formulated with aluminum hydroxide adjuvant and challenged with various doses of BoNT/B. A dose-dependent protective potency against BoNT/B was observed. Mice immunized with one injection of ≥ 1 μg BHc antigen or two injections of ≥ 0.0625 μg BHc antigen were completely protected against 1000 LD50 or 10,000LD50 of BoNT/B, respectively ( and ). The mouse potency bioassay was subjected to probit analysis to determine the ED50, or theoretical antigen dose that will protect 50% of the mice from lethal injection. The calculated ED50 for this potency assay was 0.244 μg with single dose or 0.013 μg with two doses.
Table 2. Survival, group antibody ELISA titers, and serum neutralization titers of mice after vaccination with BHc vaccine.
Figure 2. Survival of mice immunized with BHc vaccine. Mice alive after challenge with a dose of 1000 LD50 of BoNT/B 3 weeks after one injection or 10,000LD50 of BoNT/B 3 weeks after two injections.
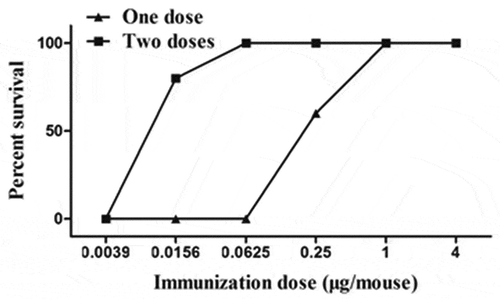
A dose-dependent anti-BHc ELISA and neutralization antibody response was also observed in mice immunized with the BHc subunit vaccine ( and ). And the anti-BHc ELISA and neutralization antibody titers correlated well with protective potency against BoNT/B. In summary, the recombinant BHc antigen formulated with aluminum hydroxide adjuvant afforded effective protection against neurotoxin challenge in Balb/c mice, indicating that this BHc protein has a functionally active conformation and can be used as a subunit candidate vaccine against BoNT/B.
Figure 3. Anti-BHc antibody titers in mice vaccinated with one or two doses of BHc formulated with aluminum hydroxide adjuvant. Mice were immunized one or two doses with 0.039 μg to 4 μg of BHc formulated with aluminum hydroxide adjuvant. Sera form individual mice from each group (n = 5) three weeks after final immunization were collected and specific antibody titer were analyzed by ELISA. Serum sample from individual mice sere assayed and the geometric mean titer (GMT) was calculated for each group.
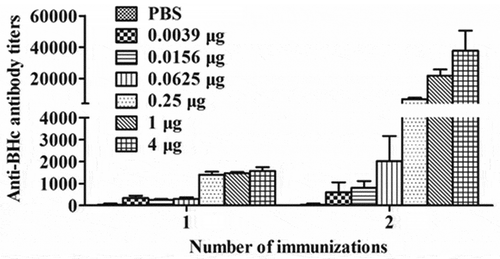
Discussion
BoNTs are the most toxic proteins, which can result in human botulism. Vaccination is an effective strategy to prevent botulism.Citation5 The Hc domain of BoNTs is nontoxic and carry most of the neutralizing epitopes, making it sufficient to generate protective immune responses against natural BoNT in animal models.Citation13–Citation15
In this study, we presented the production and evaluation of a recombinant Hc subunit vaccine as a candidate subunit vaccine for human use in the prevention of botulism serotype B. A prokaryotic expression vector encoding the nontoxic Hc domain of BoNT/B (BHc) was constructed and soluble recombinant BHc antigen was highly expressed in E. coli and purified by sequential chromatography.
The potency study indicated that the E.coli-expressed BHc have good immunogenicity and is efficacious in eliciting protective antibody against natural BoNT/B. We showed that 1μg of BHc can elicit strong immune response and protect the mice against 1000 LD50 dose of active BoNT/B after one injection and 10,000 LD50 dose after two injections, which is comparable with mBHc. However, BHc can elicit significantly higher antibody titer (P < 0.05) against BoNT/B after twice immunization compare with mBHc. Notably, recombinant glycosylated secreted wild BHc products were immunologically inert and could not confer protection against BoNT/B.Citation21 The mBHc was a non-glycosylated secreted homogeneous BHc isoform, which was successfully prepared in yeast after deleting the pro-peptide and removing its single potential glycosylation site (BHcN957Q).
To further conform the potency of the BHc subunit vaccine, a dose-dependent efficacy study was carried out in mice. The results showed that E.coli-expressed BHc can protect mice against high-dose BoNT/B challenge with low injection dosage. The immunization dosage ranged from 0.0039 μg to 4 μg by four-fold. High protective efficacy was produced in mice immunized with one injection of ≥ 1 μg BHc antigen or two injections of ≥ 0.0625 μg BHc antigen. And the group GMT correlated well with group neutralizing antibody titers and protective potency. Our results suggest that the recombinant BHc expressed in E.coli is biologically active and potent to be used as a subunit candidate vaccine against BoNT/B.
Extensive studies aimed to produce recombinant Hc of BoNTs were performed in different expression systems, among which E.coli and yeast were the systems mostly used. Previously, the recombinant BHc expressed in E.coli was insoluble in the form of inclusion bodies.Citation22–Citation24 Meanwhile, yeast expressed BHc protein was glycosylated and fail to elicit protective immunity as reported.Citation22 So we developed a non-glycosylated secreted mBHc protein in yeast that was immunologically active and can confer effective protective immunity.Citation21 Yeast expression system can promote the expression quantity and ease the purification process by secretory express. However, for vaccine development, E.coli offers additional advantages over Pichia pastoris, including cost-effectiveness and short production circle. Most importantly, the soluble recombinant Hc proteins of BoNT produced by intracellular expression in E. coli are non-glycosylated and biologically similar with the native structure of BoNTs, which render them more immunogenic.
Thus, in this study, we constructed a pTIG-Trx-BHc expression vector using the BamH I and Xho I restriction sites, and obtained fully soluble expression of recombinant BHc in E.coli.Citation25 The successfully soluble expression of BHc is largely owe to the pTIG-Trx expression vector. Because expression of the same synthetic BHc gene into soluble protein failed in other vectors in our lab and the form of inclusion bodies was biologically inactive. And the pTIG-Trx-BHc vector can express thioredoxin (Trx) together with BHc, which can help BHc protein to fold correctly and be expressed in a soluble form as previously reported.Citation26
In summary, we successfully expressed and purified a non-His-tagged homogeneous BHc in E.coli and studied its immunogenicity in mice, compared with a non-glycosylated mBHc expressed in yeast.Citation21 Our results showed that BHc was immunological active and provided comparable protective potency to mBHc, but BHc elicited higher antibody titers and neutralization potency against BoNT/B than mBHc after two immunizations. Therefore, the non-His-tagged homogeneous BHc expressed in E.coli represents a good potential candidate subunit vaccine for human use. We further need to determine the immunological and biological activity of BHc expressed in E.coli in the next study. And the efficacy of the BHc subunit vaccine in various animal models will be further characterized for preclinical studies.
Materials and methods
Constructions of expression vector
To produce recombinant non His-tagged BHc antigen in E. coli, a prokaryotic expression vector pTIG-Trx-BHc was constructed in this study. In brief, the BHc gene encoding the Hc domain of BoNT/B (BHc, amino acids 853–1291, ~ 50 kDa) was amplified by PCR from pABE293SBHc vector with primer pairs CATGG GATCC TTCAA CAAAT ACAAC TCCGA AATCT TAAAC and CATGC TCGAG GGAAC CTCAC TCGGT CCAAC CCTC.Citation27 The BHc gene was cloned into the pTIG-Trx expression vector (an optical prokaryotic expression vector maintained in our lab) using the BamH I and Xho I restriction sites,Citation25 which resulted in the recombinant plasmid pTIG-Trx-BHc confirmed by sequencing.
Expression and purification of recombinant BHc protein
The recombinant plasmid pTIG-Trx-BHc was prepared using Endofree Mega-Q kits (Qiagen, Hilden, Germany) and subsequently transformed into E. coli strain BL21 (DE3) (TRANSGEN BIOTECH, Beijing, China). The single colonies were cultured at 37°C in 2× YT (1.6 %Tryptone, 1 % Yeast extract, 0.5 % NaCl) medium containing 100 μg/ml ampicillin until the OD600 reach 0.6–1.0. Isopropyl-β-D-thiogalactopyranoside (IPTG, Promega, Madison, WI, USA) was added to the medium at a final concentration of 0.2 mM, the cultures were induced at 16°C overnight. The bacterial cells were harvested by centrifugation (8000 rpm, 20 min, 4°C), and the expression products were analyzed by sodium dodecyl sulfate-polyacrylamide gel electrophoresis (SDS-PAGE). The cells were suspended in lysis buffer (100 mM sodium phosphate, pH 6.0) and lysed by ultrasonic homogenizer. The supernatant was cleared by centrifugation and used for further purification of the BHc product.
The supernatants containing the non-His-tagged BHc product were purified using sequential cation exchange chromatography. All chromatography steps were performed on an AKTA Explorer (GE Healthcare, Piscataway, NJ, USA). Briefly, a CaptoTM MMC column (GE Healthcare, Piscataway, NJ, USA) was used for the first purification step. The column was equilibrated with buffer A (20 mM sodium phosphate, pH 6.0). Next, the column was equilibrated with 1 M NaCl in buffer A and then loaded with the supernatants. The BHc protein was eluted with 100 mM sodium phosphate (pH 7.5). A Source 30S column (GE Healthcare) was used for the second purification step. The column was equilibrated with buffer A and then loaded with previously eluted pooled solutions. The BHc protein was eluted with 200 mM NaCl in buffer A. Finally, the eluate containing BHc protein was desalted to phosphate-buffered saline (PBS, pH 7.4). The purified BHc protein was verified by 10% SDS-PAGE and Western blot using hyper-immune horse BoNT/B antiserum.
Immunization of mice and challenge with BoNT/B
Specific pathogen-free female Balb/c mice (Beijing Laboratory Animal Center, Beijing, China) at 6–8 weeks of age were randomly assigned to different treatment groups (10 mice per group). Mice were intramuscularly (i.m.) injected with 1 μg or 10 μg of recombinant BHc antigen or mBHc in 100 μl PBS (pH 7.4) containing 0.2% (w/w) alhydrogel (Aluminum hydroxide gel, Sigma–Aldrich, St. Louis, MO, USA). As a negative control, PBS instead of the antigen was formulated with the alhydrogel. The same method and amounts of BHc antigens were used for boosting immunization at 3-week intervals. To further assess the immune protective response against BoNT/B, 5 mice of each group were i.m. injected with 0.0039, 0.0156, 0.0625, 0.25, 1 or 4 μg recombinant BHc antigen one or two doses, respectively.
Three weeks after the first or second vaccination, the mice of different treatment groups were challenged with an active preparation containing 103 or 104 50% lethal doses (LD50) of BoNT/B by i.p. injection, and survival was monitored for 7 days. All animal procedures were conducted with the approval of the Beijing Institute of Biotechnology Institutional Animal Care and Use Committee and were in full compliance with the Committee’s guidelines.
Serum antibody titer measurement
To assess BHc-specific antibody responses of immunized mice, serum samples were collected at 3 weeks after each immunization and stored at −20°C before assaying. ELISA plates (Corning, NY, USA) were coated overnight at 4°C with purified recombinant BHc (0.2 μg/well). The plates were washed six times with PBS-T (PBS, 0.05% Tween-20) and blocked with PBS containing 2% albumin bovine V (BBI Life Sciences, Shanghai, China). The mouse serum samples were two-fold serially diluted in blocking buffer starting at the 1:100 dilutions, and plates were incubated with serum diluents (100 μl/well) for 1.5 h at 37°C. After washing with PBS-T six times, the total IgG titers and the individual isotype of IgG were measured using 1:2000 dilution of HRP-conjugated goat anti-mouse IgG (Jackson Immunoresearch, Lancaster, PA, USA; Santa Cruz Biotechnology, Santa Cruz, CA, USA) for 30 min at 37°C. After washing, the plates were incubated with 50 μl of a substrate solution containing 0.04 % o-phenylenediamine (OPD, w/v) and 0.02 % H2O2 (v/v) in citrate-phosphate buffer (pH5.0) for 10 min at room temperature. The reaction was stopped with 2 M H2SO4 (50 μl/well), and the plates were read with a SpectraMax 190 microplate reader (Molecular Devices, Sunnyvale, CA, USA). Antibody titers were estimated as the reciprocal of the maximum dilution where the absorbance of the serum greater than 0.3 units, after subtraction of non-specific binding detected in control serum. Serum antibody titers of individual mice was assayed and the geometric mean titer (GMT) for each group was determined.
BoNT/B neutralization assay
The neutralization potency of the sera from mice vaccinated with two doses of recombinant BHc or mBHc was measured using a BoNT/B neutralization assay as described previously.Citation28 Serially diluted anti-BoNT/B sera collected from immunized mice mixed with a standard concentration of BoNT/B (100 LD50/ml) were incubated 15 min at 37°C. Mice (18–22 g) were intraperitoneally (i.p.) injected with the mixtures in a total volume of 0.5 ml/mouse (four mice in each group). The mice were monitored for 7 days, and the survival was recorded. The neutralizing antibody titers in the sera were calculated as international units per milliliter (IU/ml) according to a WHO (World Health Organization) BoNT/B antitoxin.
Statistical analysis
Differences in the antibody immune responses were analyzed statistically by ANOVA one-way variance test or using the Student’s t-test for the analysis of differences between groups. Fisher’s exact test was used to determine statistical differences in survival between the treatment groups. For all tests only data resulting in P values < 0.05 were regarded as statistically significant. Fifty percent effective concentrations (ED50) were determined by probit analysis at the 95% confidence level with SAS (version 6.10; SAS Institute, Cary, N.C.).
Disclosure of potential conflicts of interest
The authors declare no financial or commercial conflict of interest.
Additional information
Funding
References
- Dembek ZF, Smith LA, Rusnak JM. 2007. Botulism: cause, effects, diagnosis, clinical and laboratory identification, and treatment modalities. Disaster Med Public Health Prep. 1:122–134. doi:10.1097/DMP.0b013e318158c5fd.
- Smith LA, Rusnak JM. Botulinum neurotoxin vaccines: past, present, and future. Crit Rev Immunol. 27;2007:303–318.
- Schiavo G, Matteoli M, Montecucco C. 2000. Neurotoxins affecting neuroexocytosis. Physiol Rev. 80:717–766. doi:10.1152/physrev.2000.80.2.717.
- Yu YZ, Zhang SM, Ma Y, Zhu HQ, Wang WB, Du Y, Zhou XW, Wang RL, Wang S, Yu WY, et al. 2010. Development and evaluation of candidate vaccine and antitoxin against botulinum neurotoxin serotype F. Clin Immunol. 137:271–280. doi:10.1016/j.clim.2010.07.005.
- Shukla HD, Sharma SK. 2005. Clostridium botulinum: a bug with beauty and weapon. Crit Rev Microbiol. 31:11–18. doi:10.1080/10408410590912952.
- Turton K, Chaddock JA, Acharya KR. 2002. Botulinum and tetanus neurotoxins: structure, function and therapeutic utility. Trends Biochem Sci. 27:552–558.
- Swaminathan S, Eswaramoorthy S. 2000. Structural analysis of the catalytic and binding sites of Clostridium botulinum neurotoxin B. Nat Struct Biol. 7:693–699.
- Schiavo G, Rossetto O, Santucci A, DasGupta BR, Montecucco C. 1992. Botulinum neurotoxins are zinc proteins. J Biol Chem. 267:23479–23483.
- Gu S, Jin R. 2013. Assembly and function of the botulinum neurotoxin progenitor complex. Curr Top Microbiol Immunol. 364:21–44. doi:10.1007/978-3-642-33570-9_2.
- Lee K, Gu S, Jin L, Le TTN, Cheng LW, Strotmeier J, Kruel AM, Yao G, Perry K, Rummel A, et al. 2013. Structure of a bimodular botulinum neurotoxin complex provides insights into its oral toxicity. Plos Pathogens. 9:e1003690. doi:10.1371/journal.ppat.1003690.
- Matak I, Lackovic Z. 2014. Botulinum toxin A, brain and pain. Prog Neurobiol. 119-120:39–59. doi:10.1016/j.pneurobio.2014.06.001.
- Inglesby TV, Schechter R, Inglesby TV, Henderson DA, Bartlett JG, Ascher MS, Eitzen E, Fine AD, Hauer J, Layton M, et al. 2001. Botulinum toxin as a biological weapon: medical and public health management. Jama. 285:1059–1070.
- Karalewitz AP, Barbieri JT. 2012. Vaccines against botulism. Curr Opin Microbiol. 15:317–324. doi:10.1016/j.mib.2012.05.009.
- Smith LA. Botulism and vaccines for its prevention. Vaccine. 2009;27(Suppl 4):D33–9. doi:10.1016/j.vaccine.2009.08.059.
- Atassi MZ. 2004. Basic immunological aspects of botulinum toxin therapy. Mov Disord. 19:S68–S84. doi:10.1002/mds.20020.
- Moreira GM, Cunha CE, Salvarani FM, Goncalves LA, Pires PS, Conceicao FR, Lobato FCF. 2014. Production of recombinant botulism antigens: a review of expression systems. Anaerobe. 28:130–136. doi:10.1016/j.anaerobe.2014.06.003.
- Byrne MP, Smith TJ, Montgomery VA, Smith LA. 1998. Purification, potency, and efficacy of the botulinum neurotoxin type A binding domain from Pichia pastoris as a recombinant vaccine candidate. Infect Immun. 66:4817–4822.
- Gurkan C, Ellar DJ. 2005. Recombinant production of bacterial toxins and their derivatives in the methylotrophic yeast Pichia pastoris. Microb Cell Fact. 4:1–8. doi:10.1186/1475-2859-4-1.
- Yu YZ, Li N, Wang RL, Zhu HQ, Wang S, Yu WY, Sun ZW. 2008. Evaluation of a recombinant Hc of clostridium botulinum neurotoxin serotype F as an effective subunit vaccine. Clin Vaccine Immunol Cvi. 15:1819. doi:10.1128/CVI.00239-08.
- Holley JL, Elmore M, Mauchline M, Minton N, Titball RW. 2000. Cloning, expression and evaluation of a recombinant sub-unit vaccine against clostridium botulinum type F toxin. Vaccine. 19:288–297.
- Liu B, Shi D, Chang S, Gong X, Yu Y, Sun Z, Wu J. 2015. Characterization and immunological activity of different forms of recombinant secreted Hc of botulinum neurotoxin serotype B products expressed in yeast. Sci Rep. 5:7678. doi:10.1038/srep07678.
- Byrne MP, Smith LA. 2000. Development of vaccines for prevention of botulism. Biochimie. 82:955–966.
- Gao YL, Gao S, Kang L, Nie C, Wang JL. 2010. Expression of Hc fragment from Clostridium botulinum neurotoxin serotype B in Escherichia coli and its use as a good immunogen. Hum Vaccin. 6:462–466.
- Zhou Y, Singh BR. 2004. Cloning, high-level expression, single-step purification, and binding activity of His6-tagged recombinant type B botulinum neurotoxin heavy chain transmembrane and binding domain. Protein Expr Purif. 34:8–16. doi:10.1016/j.pep.2003.10.015.
- Yu YZ, Na L, Zhu HQ, Wang RL, Yun D, Shuang W, Yu WY, Sun ZW. 2009. The recombinant Hc subunit of Clostridium botulinum neurotoxin serotype A is an effective botulism vaccine candidate. Vaccine. 27:2816–2822. doi:10.1016/j.vaccine.2009.02.091.
- Yu YZ, Gong ZW, Ma Y, Zhang SM, Zhu HQ, Wang WB, Du Y, Wang S, Yu WY, Sun ZW. 2011. Co-expression of tetanus toxin fragment C in Escherichia coli with thioredoxin and its evaluation as an effective subunit vaccine candidate. Vaccine. 29:5978–5985. doi:10.1016/j.vaccine.2011.06.039.
- Yu YZ, Shi DY, Liu S, Gong ZW, Wang S, Sun ZW. 2015. Production and evaluation of a recombinant subunit vaccine against botulinum neurotoxin serotype B using a 293E expression system. Hum Vaccin. 11:468–473. doi:10.4161/hv.29714.
- Yu YZ, Wang WB, Li N, Wang S, Yu WY, Sun ZW. 2010. Enhanced potency of individual and bivalent DNA replicon vaccines or conventional DNA vaccines by formulation with aluminum phosphate. Biologicals. 38:658–663. doi:10.1016/j.biologicals.2010.08.001.