ABSTRACT
Studies have revealed that vaccines are more often exposed to sub-zero temperatures during cold chain transportation than what was previously known. Such exposure might be detrimental to the potency of temperature-sensitive vaccines. The aim of this study was to evaluate the impact of exposure to freezing on the physicochemical properties and biological activities of recombinant hepatitis E (rHE) vaccine. Changes in rHE vaccine due to freezing temperatures were analyzed with regard to sedimentation rate, antigenicity, and antibody affinity and potency. The freezing temperature of rHE was measured, then rHE vaccine was exposed to freezing temperatures below −10°C.Significant increase of sedimentation rate was noted, according to shake test and massed precipitates. In addition, the binding affinity of rHE vaccine to six specific monoclonal antibodies was significantly reduced and the in vivo potency for eliciting a protective IgG response was also partially lost, especially for anti-HEV neutralizing antibodies. Altogether, our work indicates that exposure of rHE vaccine to a temperature below −10°C results in the loss of structural integrity and biological potency of rHE vaccine.
Introduction
Hepatitis E virus (HEV) is a non-enveloped virus with a positive-sense, single-stranded RNA genome and belongs to the genus Orthohepevirus of the Hepeviridae family.Citation1 HEV infection is the most common cause of acute hepatitis worldwide.Citation2 In developed countries, HEV infection remains a serious threat to life and productivity.Citation3 An estimated 35 million HEV infections occur annually worldwide, resulting in more than 70,000 deaths.Citation4 The average mortality rate is between 0.2 and 4%, while it can reach up to 10–25% in pregnant women who are at a higher risk of HEV infection.Citation5,Citation6 Fortunately, the recombinant antigens derived from nucleocapsid protein ORF2, named HEV 239 (Hecolin®), are immunogenic and can protect macaques and humans from HEV infection. HE vaccine, launched in China in 2012, is formulated with aluminum salts.Citation7 Hecolin® consists of an ORF2 fragment (aa 368–606) from the N-terminal region of T = 1 VLP and is adapted to be efficiently expressed in E. coli.Citation8 The vaccine is well tolerated and efficacious in the general adult population (16–65 years old).Citation9
Freezing exposure occurs across all segments of the cold chain, such as transportation and storage.Citation10,Citation11 When a vaccine is damaged by freezing, physical and immunological properties are adversely affected, and the loss of potency can never be restored.Citation12,Citation13 The World Health Organization (WHO) and manufacturer guidelines clearly state that adjuvanted vaccines, such as hepatitis B vaccine, DTP, tetanus toxoid, and Haemophilus influenzae type b, must not be exposed to freezing temperatures and should be stored at a temperature of 2–8°C.Citation14 Vaccines should be disposed when freezing occurs.
For every new vaccine, it is important to understand the impact of temperature excursions on the quality and performance. In our study, the freezing temperature of HE vaccine, and how it affected physicochemical properties and biological activities were analyzed. The results of our study provided scientific basis for ensuring the safety and effectiveness of hepatitis HE vaccine during transportation and improving the accessibility of vaccines.
Materials and methods
rHE vaccine and vaccine antigens
Hecolin® is derived from ORF2 of HEV genotype I (368–606 aa, p239). Bulk vaccine and finished product were both provided by Xiamen Innovax Biotech Co., Ltd (China), and stored at a well-controlled atmosphere of 2–8°C. Bulk vaccine is a sterile liquid containing purified recombinant capsid protein of HEV. Finished product contains 30 μg of purified protein absorbed to 0.8 mg of aluminum hydroxide and suspended in 0.5 mL of buffered saline solution.
Desorption of HE vaccine finished product
Before detection, the antigen was dissociated from aluminum adjuvant by adding desorption solution (0.1 M citrate and 0.2 M phosphate, pH 6.0),Citation15 and incubating the mix at 37°C for 4 h. After centrifugation at 12,000 rpm for 10 min, the antigen in the upper liquid phase was tested for its binding affinity to monoclonal antibodies (mAbs). The dissociated antigen is named HEV-Ag in this study.
Antibodies
Specific mAbs to p239 named 8H3, 8C11, 12F12, 8G12, and 3A11 were kindly provided by Dr. Ningshao Xia, and 4# was obtained from Dr Feng Zhang. They were prepared following immunization with p239 antigens using a standard murine mAb preparation protocol.Citation16 Among them, 8H3, 8C11, 12F12, and 8G12 recognize a conformational epitope; while 3A11 and 4# detected a linear epitope.Citation17 shows the recognized epitopes of the five mAbs.Citation15,Citation18,Citation19 These mAbs were labeled with HRP using Lightning-Link HRP conjugation kit (Novus Biologicals, USA) and used to identify the epitopes associated with HE vaccine protection.
Table 1. Comparison of the seroconversion rate of vaccine freeze exposure at −20°C and stored at 4°C. The seroconversion rate of vaccine freeze exposure at −20°C is lower than vaccine at 4°C. This result indicates that freezing and thawing caused a loss of potency for hepatitis E vaccines.
Reference material
The HEV vaccine antigen reference was prepared from bulk vaccine, kindly provided by the manufacturer, with no adjuvant and at a concentration of 60 µg/mL. The vaccine antigen reference was used in the quantitation of HEV-Ag. WHO reference reagent for quantification of HEV IgG antibody in serum of a mouse immunized by the virus (NIBSC code: 95/584) was reconstituted as directed in 0.5 mL distilled water and to a final concentration of 100 units per ml. The 8G12 antibody reference (1 mg per mL) was used to quantify HEV 8G12-like antibody in serum of mouse immunized with HE vaccine. All reference reagents were serially diluted with PBS within a certain concentration range. Antigen and antibody concentrations in tested samples were extrapolated using a linear regression-fitting algorithm from a standard curve generated by plotting reference concentration vs the OD value. After correcting for dilution factor, the concentration of the sample was determined.
Differential scanning calorimetry (DSC) assay
The freezing point of each sample was determined using a differential scanning calorimeter (Diamond DSC, PerkinElmer, Waltham, MA). Indium was used as the calibration standard. Each sample was prepared by placing a 20-μL aliquot in an aluminum calorimeter pan. The capped pan was then placed in the chamber and subjected to a thermal cycle. The thermal cycle consisted of the following: (1) heat to 50°C at 10°C/min; (2) hold at 50°C for 1 min; (3) cool to −40°C at 10°C/min; (4) heat to 50°C at 10°C/min; (5) hold for 1 min at 50°C; and (6) cool to −40°C at 10°C/min. The melting/freezing point was determined during the heating phase from −40°C to 50°C. Data were analyzed by soft Origin.
Freezing treatment of HE vaccine
For determining the freezing temperature, vaccines were exposed to temperatures ranging from 0 to −20°C in 1°C increments without agitation in the environmental chamber (LH1.5, Associated Environmental Systems, Ayer, MA, USA). The temperature was confirmed by placing a calibrated thermometer inside. Visual inspection determined freezing of the samples. Specifically, every 30 min after each incubation the vial was gently tilted to observe the flow of the content. To evaluate the freezing stability, samples were placed in an environmental chamber at the target temperature. After 24 h of storage, they were transferred to a 2–8°C cold storage until tested.
Sedimentation rate
Shake tests for the detection of freezing damage were performed as described.Citation20 Briefly, vials were frozen until the contents were completely solid, then let it thaw at 2–8°C. On the contrary, a vial from the same batch was stored in 2–8°C. Both vials were vigorously shaken for 10–15 s and placed on a flat surface side-by-side for continuous observation.
Continuous 30 s measurements of absorbance at A280 and A600 were used as indicators of protein and particles content in the upper liquid. Sediment yield was calculated as follows: sedimentation rate = (A280 of untreated sample – A280 of treated sample)/A280 of non-frozen sample.
Phase contrast microscopy analysis on vaccine morphology
The appearance of 10 μL of non-frozen and frozen-damaged vaccine samples was investigated with phase contrast microscopy after vigorously shaking each sample.
Quantitation of total protein on frozen vaccine
In order to determine if the freezing process impacted the desorption of antigens, total protein dissociated from aluminum adjuvant was quantified by bicinchoninic acid disodium (BCA) assay kit (Thermo Scientific™ 23227, USA). The principle of the BCA reaction is based on reduction of the cupric (Cu2+) ion to cuprous (Cu1+) ion by protein.Citation21
Quantitation of HEV-Ag on frozen vaccine
In this system, the microplate was coated with 8C11. When the HEV-Ag and HRP labeled 8H3 were successively added, the enzyme catalyzed a color reaction after adding the substrate inducing. Within a certain range, the intensity of color reaction was directly proportional with the concentration of antigen. Briefly, the antigen of HE vaccine samples and the HEV-Ag reference reagent were serially diluted with PBS and incubated with mouse monoclonal anti-ORF2 antibody 8C11 immobilized on a 96 well microplate. The antigen of HE vaccine was also detected with HRP-8H3. HEV-Ag concentration was calculated by multiplying the dilution factor with the concentration obtained from the standard curve.
Binding affinity of mAbs to antigen on frozen vaccine
To test the binding affinity of mAbs to antigen on frozen vaccines, a Two-Antibody Sandwich Microplate Enzyme Immunoassay was performed. HEV-Ags before and after freezing were plated on a 96 well microplate pre-coated with different HRP labeled mAbs, such as, 8H3, 8C11, 8G12, 12F12, 3A11, and 4#Citation22 The difference of A450 value reflected the freezing effect on HEV vaccine.
In vivo potency assay
The mouse potency test was performed using female BALB/c mice with an intraperitoneal injection of a single HEV vaccine dose. The vaccine was diluted by a factor of four to 1.6, 0.4, 0.1, and 0.025 μg/mL with the vaccine dilution solution. Mice were injected with vaccine diluent solution corresponded to the control group (a total of 5 groups with 10 mice per group). Serum was collected 4 weeks after immunization. Indirect ELISA was used to quantitate antibodies against HEV 239 protein present in the samples. Specifically, microplate was coated with HEV 239 protein and incubated with the mouse serum. Then the HRP-labeled secondary antibody and the enzyme reagent were added, which resulted in a colorimetric reaction. Within a certain range, intensity of the generated color was directly proportional with the concentration of HE antibody, thus concentration of the antibody was determined by extrapolating the results from a calibration curve. The seroconversion (with >2.1-fold raise in OD value) percentage of mice at each dose was calculated. The 50% seroconversion (ED50) results were analyzed based on the dose-response curve using the Reed-Muench model and expressed in units of micrograms of antigen per mouse.Citation23
Quantification of anti-HEV IgG
Anti-HEV IgG levels in serum were quantified with commercial HEV IgG detection kit produced by Wantai Co., Ltd (China) and using an HRP-labeled goat anti-mouse antibody. Anti-HEV IgG concentration in serum samples was calculated by multiplying the concentration obtained from the standard curve with the dilution factor.
Quantitation of 8G12 competitive antibody
Presence of 8G12 antibody was predominant in vaccine-induced anti-HEV antibody, and it had a protective and neutralizing capacity, which could significantly block virus infection in host cells.Citation18 In addition, the 8G12-like antibody presented a similar dynamic pattern as anti-HEV antibody during “prime-boost” vaccination, and the proportion of 8G12-like antibodies in the total of anti-HEV antibodies increased with boosting vaccination. The 8G12-like antibody concentration in serum was determined by competitive ELISA and calculated by multiplying the dilution factor with the concentration obtained from the standard curve.Citation24
Statistical analysis
All statistical analyses were performed with Graphpad Prism software packages. Normally distributed data were analyzed for differences among experimental groups by using one-way ANOVA. Comparisons resulting in p-values ≤0.05 were considered statistically significant.
Results
Freezing temperature of HE vaccine
Freezing point is a variable measurement due to the effects of supercooling but might be closer to what is encountered in the field. The melting point determines the temperature at which a vaccine is susceptible to freezing.Citation25 The freezing point (the onset melting temperature) of HE vaccine is approximately −1.9°C, as determined by the DSC assay (supp.1). Then, vaccine was exposed to temperatures ranging from 0 to −20°C in 1°C increments without agitation in the environmental chamber. Visual inspection revealed the presence of liquid flow from 0 to −9°C, but a completed frozen liquid at −10°C or lower temperatures. At −10°C, the vaccine required 1 h to partially freeze and 2 h to completely freeze.
The sedimentation rate and shake tests
Shake test was recommended for detecting freezing damage to vaccines by WHO. Vaccines were exposed to −10 or −20°C for 24 h and observed at room temperature with non-frozen sample (stored at 2–8°C) for cooperation. Vaccines were vigorously shaken for 15 s and then left at room temperature. The settlement and obvious stratification were observed in frozen and damaged vaccines at −10°C or −20°C in 3 min (). Similar observation was obtained in samples receiving 1, 2 or 3 cycles of −20°C exposure. On the contrary, vaccine incubated at 2–8°C corresponded to a stable emulsion of adjuvanted HEV239 dispersed throughout an aqueous phase.
Figure 1. Freeze damage identification of HE vaccine indicated by shake test and A280 andA600. (A) The sedimentation and stratification were observed in freezing treated vaccine,while untreated vaccine was still in the form of a stable emulsion dispersed throughout an aqueous phase suspension. (B and C) Constant absorbance values were shown both in A280 and A600 for untreated vaccine. A280 for treated vaccine decreased from 1.100 to 0.366 in 10 min. A600 reflected the size and density of particles. Due to the particle aggregation, the initial A600 of untreated vaccine was 0.500, which is higher than 0.350 in untreated vaccine. And then the particles settled rapidly within 3 min and A600 value decreased to 0.100 at 10 min. Both absorbance values and shake tests could discriminate freezing exposure on rHE vaccine.
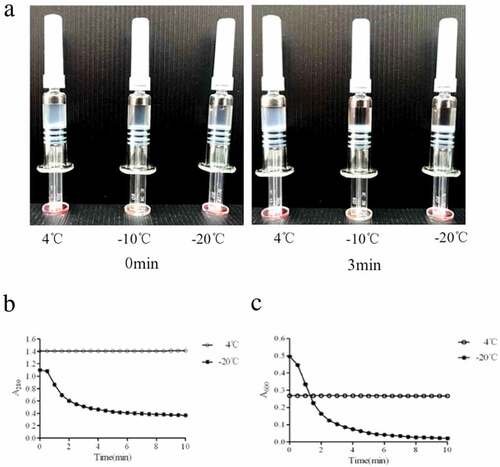
Quantitation of the sedimentation rate analysis
For the quantitative sedimentation rate test, absorbance of vaccine supernatant at A280 and A600 was measured with an ultraviolet spectrophotometer (SpectraMaxM2, Molecular Devices). The A280 value reflected protein concentration of vaccine, and A600 reflected the size and density of particles. A280 and A600 values of treated vaccine decreased from 1.100 to 0.366 and from 0.500 to 0.021, respectively, in 10 min ( and ). However, absorbance values of untreated vaccine remained constant during observation. Thus, both absorbance values and shake tests characterized freezing exposure of HE vaccine.
Phase contrast microscopy of frozen vaccine
Phase contrast microscopy is a common method of identifying freezing damage in vaccines.Citation26 The results showed that frozen vaccine samples contained large conglomerates including massed precipitates with amorphous, crystalline, solid, and needlelike structures, whereas vaccines maintained within 2–8°C showed a fine-grain structure under phase contrast microscopy ().
The antigen activity assay on frozen vaccine
The antigen activity was evaluated using ELISA. The antigen activity of frozen vaccines was significantly reduced after exposure to freezing temperatures of −10 or −20°C (p < 0.05) compared with that of vaccines maintained within 4°C (). Due to the repeated freezing and thawing, the antigen activity was significantly reduced (p < 0.01) as the freezing times increased (). No significant difference in protein content was observed (and ). All the results demonstrated that the antigen structure might have been impacted by freezing-thaw process. Therefore, subsequently, we analyzed neutralization capacity of vaccine toward mAb by ELISA.
Figure 3. Comparison of dissolved antigen activity of vaccine before and after freeze exposure. (A) The antigen activity significantly decline in freezing damaged vaccine (p < 0.05), (B) Repeated freezing-thawing cycle significantly reduced the concentration of vaccine antigen(p < 0.01). (C and D) Consistent content of total protein were detected by BCA before and after freezing treatment. These results demonstrated the activity of antigen was decreased after freeze exposure. Error bars the standard deviation of three independent replicates.
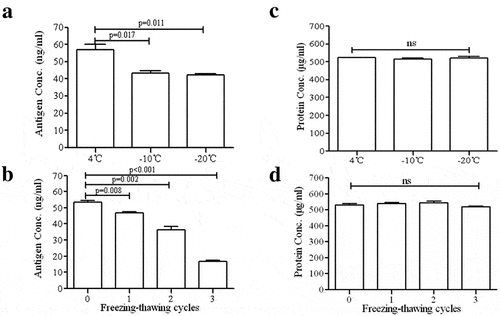
Binding affinity of antigen to frozen vaccine
The binding activity of specific mAbs revealed different epitopes of the antigen. Six mAbs, which recognized different epitopes, were used to evaluate the immune reactivity of HEV239 in vaccine samples stored at 4°C or treated at −10 and −20°C for 24 h. The sandwich ELISA assessed the HEV239 antigenicity. shows the binding profile of goat-anti-HEV239 polyclone antibody as capture Ab and each of six mAbs as detection Ab in ELISA. The relative antigenicity was calculated by normalizing the OD value of freezing treated samples to untreated samples. Antigenicity to each mAb significantly decreased from 0.44 to 0.85 (p < 0.001) (). This result demonstrated that incubation of the samples at freezing temperatures damaged the epitope of antigen. We suspected that the freezing damage was caused by aluminum adjuvant present in HEV vaccine. The antigenicity gradually reduced as the number of freeze-thaw cycles increased (p < 0.001) (). These results suggested that aluminum adjuvant in HEV vaccine may be responsible for the epitope damage on the HEV-Ag.
Figure 4. The epitope characteristics of the antigen reflected by 6 mAb. (A) The relative antigenicity to 6 mAb reduced significant in freezing treated samples (p < 0.001). (B) The antigenicity reduced gradually as the number of freeze-thaw cycles increases (p < 0.05). Error bars the standard deviation of three independent replicates.
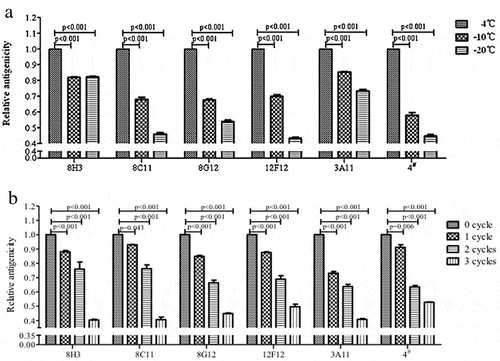
Freezing caused potency loss of HE vaccines
Potency is an important immunogenicity indicator of vaccines. In this study, we analyzed potency of the HEV vaccine by subcutaneously injecting 1.0 mL of different vaccine dilutions or the diluent into BALB/c mice of the corresponding group. After 28 days, all mice were euthanized, and sera were collected. Specific antibodies in each individual serum were assayed using indirect ELISA, and the ED50 was calculated. The seroconversion rate is shown in . Compared with non-frozen sample, the seroconversion rate of mouse immunized with vaccine incubated at −20°C decreased with the dose. ED50 of vaccine treated at −20°C (0.21 μg/mL) was higher than ED50 of sample stored 4°C (0.04 μg/mL). Thus, the results indicated that freezing and thawing caused potency loss of HE vaccines.
Table 2. The seroconversion rate of treated vaccine and untreated vaccine.
Total IgG and 8G12-like antibodies serum of mouse immunized with frozen vaccine
A 4-fold increase in total IgG was a key indicator for an effective HE vaccine in a clinical trial and the epitope of 8G12 was identified as a key epitope involved in HEV239Citation19 Studies reported that 8G12 antigen could block most binding of HEV vaccine-induced neutralizing antibodies to vaccine antigen, and thus was used as a convenient indicator for HEV vaccine potency.Citation24 Total concentration of IgG and 8G12-like antibodies in the serum were detected by using indirect and competitive ELISA, respectively.Citation25,Citation27 As shown in , total IgG of frozen vaccine was 3.6-fold lower than that of non-frozen vaccine (mice were immunized with 1.6 μg/ml HE vaccine). Presence of 8G12-like antibody was undetected in the serum of mouse immunized with frozen vaccine, while the mean value of serum 8G12 antibody in non-frozen vaccine mice was 42.4 ng/mL ().
Figure 5. Quantification oftotal IgG and 8G12-like antibodies in mice serum vaccinated by 1.6 μg freezing treated or untreated vaccine. (A) Total IgG in serum vaccinated by treated vaccine decreased 3.6-fold than that in serum vaccinated by untreated vaccine (p = 0.005) (B) Specific neutralizing antibody 8G12-like antibody couldn’t be detected in frozen vaccine immunity serum, but the mean level of 8G12-like antibody in control group was 42.45 ng/ml.
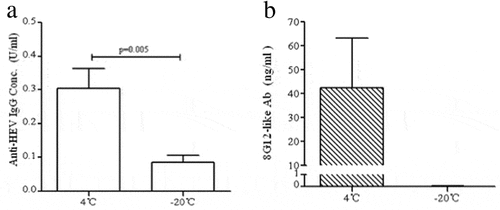
Discussion
HE is caused by HEV infection. HEV infection remains a serious threat to life and productivity in the developing world. In developed countries, an increasing number of autochthonous HE cases have been reported, suggesting that this threat is not limited to developing regions.Citation28 Although many experimental HEV vaccines have been evaluated in virus challenge studies in non-human primates, until mid-2017 only one vaccine was licensed for human use worldwide. This vaccine was licensed in China in December 2012 for use in persons aged 16 years and older. It is based on a 239-amino acid recombinant HEV peptide, corresponding to amino acids 368–606 in ORF2, which encodes the capsid protein of genotype 1 HEV.Citation8,Citation9,Citation29,Citation30 Hecolin® is a vaccine with high efficacy against HE disease in individuals involved in animal husbandry, students, members of the armed forces, food-handlers, and women of childbearing age, especially for endemic regions such as India, Mexico, and Uganda.Citation31
Vaccines must be kept within narrow temperature ranges from the point of manufacture to injection.Citation32 When exposed to temperatures outside of this narrow range, the loss of potency may accelerate a negative impact on physical and immunological properties of the vaccine.Citation33 Use of vaccine that were exposed to freezing temperatures could result in compromised immunogenicity in recipients and increased local reactions such as sterile abscesses.Citation34
As stipulated by the manufacturer, rHE vaccine should be stored at 2–8°C, protected from direct sunlight, for not more than 36 months as the vaccine shelf life, and under appropriate storage conditions. Analysis of bulk lots as well as the final products showed that the vaccine is stable when stored at 30–37°C for 2 months.Citation35 Exposure at lower or higher temperatures has not been investigated.
In this study, we were unable to freeze the HE vaccine at −9°C even with 144 h of exposure. The freezing point of HE vaccine used in this study is approximately −1.9°C, which is lower than that of the water (0°C), and higher than that of HB vaccines (−2.8°C) reported by Dexiang Chen;Citation36 this is partly due to the presence of excipients in the formulation. Furthermore, the phenomenon of super-cooling was present in HE vaccine, but it disappeared when the vaccine was shaken at low temperatures (−10°C), or the vaccine was frozen after 1 h. Scanning electron microscopy and X-ray analyses have been used to evaluate structural damage in adsorbed vaccines.Citation37 In our study, the pattern of frozen vaccine was investigated using phase contrast microscopy; the structure of aluminum-adsorbed HEV239 was destroyed by freezing and showed aggregated particles. Shake test was performed to determine whether adsorbed vaccines were affected due to freezing by observing the sedimentation rates in control and test vials.Citation24 In our study, significant increase in sedimentation rate was observed in the shake and OD280 tests. Then, the results obtained from sedimentation rate experiments of vaccines exposed for 24 h at −5, −8, −9, −10, and −20°C were compared with those of the shake test. There was no obvious precipitate in vaccine exposed at −5, −8, or −9°C (supp.3), which demonstrated that HE vaccine was not damaged by freezing temperature higher than −9°C. Previous reports demonstrated that immunogenicity was reduced after exposure to freezing temperaturesCitation38–Citation40 and then, the effect of freezing on the HEV239 epitope and the vaccine immunogenicity were studied.
Antigenic activity and binding affinity to specific monoclonal antibodies were used as indicators of the freezing effect on the HEV239 epitope. The HEV239 activity was measured with a sandwich ELISA using 8H3 as capture Ab and HRP-8C11 as detection Ab. The decreased antigenicity of frozen samples indicated that epitope matching with the 8H3 or 8C11 mAbs were damaged during freezing. This work also assessed the characteristics of key antigen epitopes after recovery from the freezing temperatures of the adjuvanted vaccine by using a panel of murine monoclonal antibodies. Using a multiple epitope approach for analysis of recombinant vaccine antigens leads to a more comprehensive evaluation of the epitope integrity.Citation41 Among the mAbs used for detecting the HEV antigen, 8C11 and 8G12 were two protective and neutralizing antibodies. MAb 8C11 recognized conformational epitopes that were composed of three discontinuous peptide segments in a dimeric form of the truncated HEV capsid protein. On the other hand, the mAb 8G12 recognized both genotype 1 and 4 through the epitopes in the dimerization region, and had protective and neutralizing capacities, which could significantly block viral infection of host cells. Structure-based mutagenesis and cell-model assays with HEV239 virus-like particles identified several conserved residues (Glu549, Lys554, and Gly591) that were essential for 8G12 neutralization.Citation19 Therefore, the mAbs 8C11 and 8G12 could be used in different ways as useful molecular probes for antigen quality assessment, such as in vitro relative potency determinations, as in the case of human papillomavirus.Citation42 Binding activity to the neutralizing and protective mAbs, such as 8C11 and 8G12, could be used as a surrogate marker for vaccine efficacy.Citation19 The binding activity was significantly reduced, as freezing temperatures damaged the HEV239 epitope formed during vaccine production. Moreover, the decrease in linear epitope activity may be due to a change in spatial structure that caused the linear epitope-binding site to be masked.
The ability to induce production of protective antibodies is critically important for structural and functional analyses of vaccines. Among these, the potency assay is the most important for vaccine characterization and lot release testing. The study indicated that the freezing process reduced the HE vaccine potency. Quantification of specific induced-antibodies by vaccine administration revealed that total antibody concentration significantly decreased, and the 8G12-like antibody was undetected. As previously described, 8G12-like antibody was a surrogate marker of vaccine efficacy in the generation of protective and neutralizing antibodies. Therefore, decrease of 8G12-like antibody in serum implied the damage of vaccine structure and a decline of vaccine immunogenicity.
In addition, aluminum adjuvants form insoluble particles that can aggregate and be phagocytosed by macrophages, which was been shown to stimulate IL-1β production.Citation43,Citation44 To investigate the safety of frozen HE vaccine, we collected the splenocytes from vaccinated mice. Inflammatory factors IL-1β, IL-6, and TNF-α were detected using ELISA. According to our results, aluminum adjuvant in HE vaccine may be responsible for the change of the conformation of the HE vaccine after repeated freezing and thawing. Aluminum adjuvant induces inflammation at the injection site, and endogenous products released from damaged cells (damage or danger associated signals), and stimulate damage-associated molecular pattern molecules, activating inflammasomes. As pro-inflammatory cytokines, IL-1β, IL-6 and TNF-α are related to neuropathic pain.Citation45 We further analyzed their expression in splenocytes from mice immunized with frozen and thawed HE vaccines. Even though no significant difference was observed when cultures were stimulated with HE vaccine bulk, the levels of IL-1β and IL-6 produced in the splenocytes from frozen vaccine immunized mice were higher than those in the control group (supp.4). The result demonstrated that freezing and thawing not only had an impact on potency of HE vaccine, but also may influence the inflammatory reaction, which might enhance pain.
World Health Organization (WHO) guidelines and manufacturer product inserts recommend that all vaccines, except oral polio vaccine, should be kept at 2–8°C during national distribution.Citation46 However, a poorly functioning cold chain may deviate from this target range and expose vaccines to freezing temperaturesCitation47 Freezing of aluminum-adjuvant-containing vaccines may occur accidentally during storage and shipmentCitation48 Improperly adjusted refrigeration equipment, poor compliance with cold chain procedures, inadequate monitoring, and poor understanding of the dangers of freezing could result in the inactivation of the vaccineCitation49 Sensitivity of HE vaccine to freezing may be due to the presence of aluminum-salt adjuvant.Citation50–Citation52 Therefore, it is important to explore the freezing temperature and the impact of freezing on the physical properties and immunogenicity of HE vaccine.
In our study, the decrement of rHE potency after freezing indicated that the main epitopes affected by freezing corresponded to those analyzed in previous studies. However, the limitation of our study is obvious: the experimental data are insufficient to further prove the effect of physical property change to the application of rHE. However, levels of inflammatory factors induced by administration of frozen vaccines, such as IL-1β, increased, which indicated that frozen vaccines may increase the risk of adverse reactions.
To summarize, all the obtained results demonstrated that HE vaccine was temperature sensitive. Damage of recombinant vaccines containing aluminum salt adjuvant due to exposure to freezing temperatures represents a real risk to an effective immunization. Therefore, the cold chain system should be strictly controlled, or the development of temperature-stable vaccine formulations should take place.
Disclosure of potential conflicts of interest
No potential conflicts of interest were disclosed.
Supplemental Material
Download Zip (276.7 KB)Supplemental Material
Supplemental data for this article can be accessed on the publisher's website.
Additional information
Funding
References
- Tam AW, Smith MM, Guerra ME, Huang CC, Bradley DW, Fry KE, Reyes GR. Hepatitis E virus (HEV): molecular cloning and sequencing of the full-length viral genome. Virology. 1991;185:120–31. doi:10.1016/0042-6822(91)90760-9.
- Riveiro-Barciela M, Rodríguez-Frías F, Buti M. Hepatitis E virus: new faces of an old infection. Ann Hepatol. 2013;12(6):861. doi:10.1016/S1665-2681(19)31290-6.
- Krain LJ, Nelson KE, Labrique AB. Host immune status and response to hepatitis E virus infection. Clin Microbiol Rev. 2014;27:139–65.
- Aggarwal R. The global prevalence of hepatitis E virus infection and susceptibility: a systematic review. Geneva (Switzerland): World Health Organization; 2010.
- Khuroo MS, Teli MR, Skidmore S, Sofi MA, Khuroo MI. Incidence and severity of viral hepatitis in pregnancy. Am J Med. 1981;70:252–55. doi:10.1016/0002-9343(81)90758-0.
- Kumar A, Beniwal M, Kar P, Sharma JB, Murthy NS. Hepatitis E in pregnancy. Int J Gynaecol Obstet. 2004;85:240–44. doi:10.1016/j.ijgo.2003.11.018.
- Proffitt A. First HEV vaccine approved. Nat Biotechnol. 2012;30(4):300. doi:10.1038/nbt0412-300a.
- Li SW, Zhang J, Li YM, Ou SH, Huang GY, He ZQ, Ge SX, Xian YL, Pang SQ, Ng MH. A bacterially expressed particulate hepatitis e vaccine: antigenicity, immunogenicity and protectivity on primates. Vaccine. 2005;23(22):2893–901. doi:10.1016/j.vaccine.2004.11.064.
- Zhu FC, Zhang J, Zhang XF, Zhou C, Wang ZZ, Huang SJ, Wang H, Yang CL, Jiang HM, Cai JP. Efficacy and safety of a recombinant hepatitis E vaccine in healthy adults: a large-scale, randomised, double-blind placebo-controlled, phase 3 trial. Lancet. 2010;376(9744):895–902. doi:10.1016/S0140-6736(10)61030-6.
- Das MK, Arora NK, Mathew T, Vyas B, Sindhu M, Yadav A. Temperature integrity and exposure to freezing temperature during vaccine transfer under the universal immunization program in Three States of India. Indian J Publ. Health. 2019;63(2):139–42. doi:10.4103/ijph.IJPH_123_18.
- Lloyd J, Lydon P, Ouhichi R, Zaffran M. Reducing the loss of vaccines from accidental freezing in the cold chain: the experience of continuous temperature monitoring in tunisia. Vaccine. 2015;33(7):902–07. doi:10.1016/j.vaccine.2014.10.080.
- World Health Organization (WHO). The effects of freezing on the appearance, potency, and toxicity of adsorbed and unadsorbed DTP vaccines. Wkly Epidemiol Rec. 1980;55:385–92.
- Dietz V, Ga1azka A, van Loon F, Cochi S. Factors affecting the immunogenicity and potency of tetanus toxoid: implications for the elimination of neonatal and non-neonatal tetanus as public health problems. Bull World Health Organ. 1997;75:81–93.
- Galazka A, Milstien J, Zaffran M. Thermostability of vaccines. Geneva (Switzerland): World Health Organization; 1998. [WHO document WHO/GPV/98.07].
- Zhan Y, Li M, Yang F, Li Y, Zheng Z, Zhang X, Lin Q, Wang Y, Li S, Xia N. Comparable quality attributes of hepatitis e vaccine antigen with and without adjuvant adsorption-dissolution treatment. Hum Vaccin Immunother. 2015;11(5):1129–39. doi:10.1080/21645515.2015.1009343.
- Zhang J, Gu Y, Ge SX, Li SW, He ZQ, Huang GY, Zhuang H, Ng MH, Xia NS. Analysis of hepatitis e virus neutralization sites using monoclonal antibodies directed against a virus capsid protein. Vaccine. 2005;23(22):0–2892. doi:10.1016/j.vaccine.2004.11.065.
- Zhang F, Li X, Li Z, Harrison TJ, Chong H, Qiao S, Huang W, Zhang H, Zhuang H, Wang Y. Detection of HEV antigen as a novel marker for the diagnosis of hepatitis E. J Med Virol. 2010;78(11):1441–48. doi:10.1002/jmv.20717.
- Gu Y, Tang X, Zhang X, Song C, Sivaraman J. Structural basis for the neutralization of hepatitis E virus by a cross-genotype antibody. Cell Res. 2015;25(5):604–20. doi:10.1038/cr.2015.34.
- Zhao M, Li XJ, Tang ZM, Yang F, Wang SL, Cai W, Zhang K, Xia NS, Zheng ZZ. A comprehensive study of neutralizing antigenic sites on the hepatitis E virus (HEV) capsid by constructing, clustering, and characterizing a tool box. J Biol Chem. 2015;290(32):19910–22. doi:10.1074/jbc.M115.649764.
- Milstien JB, Galazka AM, Kartoglu Ü, Zaffran M. Temperature sensitivity of vaccines. Geneva (Switzerland): World Health Organization; 2006. https://apps.who.int/iris/handle/10665/69387.
- European Pharmacopoeia Edition 9.0,2.5.33, Total protein.
- Zhao C, Geng Y, Harrison TJ, Huang W, Song A, Wang Y. Evaluation of an antigen-capture EIA for the diagnosis of hepatitis e virus infection. J Viral Hepat. 2015;22(11):957–63. doi:10.1111/jvh.12397.
- Reed LJ. A simple method of estimating fifty percent endpoints. Am J Hyg. 1938;27(3). doi:10.1093/oxfordjournals.aje.a118408.
- Wu X, Chen P, Lin H, Su Y, Hao X, Cao F, Li L, Zhu F, Liang Z. Dynamics of 8G12 competitive antibody in “prime-boost” vaccination of hepatitis e vaccine. Hum Vaccin Immunother. 2017;13(6):1. doi:10.1080/21645515.2017.1291105.
- White JA, Estrada M, Weldon WC, Chumakov K, Kouiavskaia D, Fournier-Caruana J, Stevens E, Gary HE Jr, Maes EF, Oberste MS. Assessing the potency and immunogenicity of inactivated poliovirus vaccine after exposure to freezing temperatures. Biologicals. 2018;53:30–38. doi:10.1016/j.biologicals.2018.03.002.
- Kartoglu U, Ozguler NK, Wolfson LJ, Kurza˛tkowski W. Validation of the shake test for detecting freeze damage to adsorbed vaccines. Bull World Health Organ. 2010;88:624–31. doi:10.2471/BLT.08.056879.
- Bendall R, Ellis V, Ijaz S, Ali R, Dalton H. A comparison of two commercially available anti-HEV igg kits and a re-evaluation of anti-HEV igg seroprevalence data in developed countries. J Med Virol. 2010;82(5):799–805. doi:10.1002/jmv.v82:5.
- Li SW, Zhao Q, Wu T, Chen S, Zhang J, Xia NS. The development of a recombinant hepatitis e vaccine HEV 239. Hum Vaccin Immunother. 2015;11(4):908–14. doi:10.1080/21645515.2015.1008870.
- Zhang J, Liu CB, Li RC, Li YM, Zheng YJ, Li YP, Luo D, Pan BB, Nong Y, Ge SX. Randomized-controlled phase ii clinical trial of a bacterially expressed recombinant hepatitis E vaccine. Vaccine. 2009;27(12):1869–74. doi:10.1016/j.vaccine.2008.12.061.
- Zhang J, Zhang XF, Huang SJ, Wu T, Hu YM, Wang ZZ, Wang H, Jiang HM, Wang YJ, Yan Q. Long-term efficacy of a hepatitis e vaccine. N Engl J Med. 2015;372(15):914–22. doi:10.1056/NEJMoa1406011.
- WHO. Hepatitis e vaccine: who position paper, May 2015–recommendations. Vaccine. 2015;34:3.
- Yakum MN, Ateudjieu J, Pélagie FR, Walter EA, Watcho P. Factors associated with the exposure of vaccines to adverse temperature conditions: the case of north west region, Cameroon. BMC Res Notes. 2015. doi:10.1186/s13104-015-1257-y.
- Organization, WH, Fund, UNC. How to monitor temperatures in the vaccine supply chain. In: International joint conference on artificial intelligence. San Francisco (CA): Morgan Kau-fmann Pubishers Inc.; 2015. p. 948–50.
- Davaalkham D, Ojima T, Wiersma S, Lkhagvasuren T, Nymadawa P, Uehara R, Watanabe M, Oki I, Nakamura Y. Evidence based public health policy and practice: administration of hepatitis b vaccine in winter as a significant predictor of the poor effectiveness of vaccination in rural Mongolia: evidence from a nationwide survey. J Epidemiol Community Health. 2007;61(7):578–84. doi:10.1136/jech.2006.051375.
- Zhang X, Wei M, Sun G, Wang X, Li M, Lin Z, Li Z, Li Y, Fang M, Zhang J. Real-time stability of a hepatitis E vaccine (Hecolin ®) demonstrated with potency assays and multifaceted physicochemical methods. Vaccine. 2016;34(48):5871–77. doi:10.1016/j.vaccine.2016.10.045.
- Chen D, Tyagi A, Carpenter J, Perkins S, Sylvester D, Guy M, Kristensen DD, Braun LJ. Characterization of the freeze sensitivity of a hepatitis b vaccine. Hum Vaccin. 2009;5(1):26–32. doi:10.4161/hv.5.1.6494.
- Kurzątkowski W, Kartoğlu Ü, Staniszewska M, Górska P, Krause A, Wysocki MJ. Structural damages in adsorbed vaccines affected by freezing. Biologicals. 2013;41(2):71–76. doi:10.1016/j.biologicals.2011.10.011.
- Ho MM, Mawas F, Bolgiano B, Lemercinier X, Crane DT, Huskisson R, Corbel MJ. Physico-chemical and immunological examination of the thermal stability of tetanus toxoid conjugate vaccines. Vaccine. 2002;20(29):3509–22.
- Kanra G, Viviani S, Yurdakök K, Ozmert E, Anemona A, Yalçin S, Demiralp O, Bilgili N, Kara A, Cengiz AB. Effect of aluminum adjuvants on safety and immunogenicity of Haemophilus influenzae type b-CRM197 conjugate vaccine. Pediatr Int. 2010;45(3):314–18. doi:10.1046/j.1442-200X.2003.01706.x.
- Dimayuga R, Scheifele D, Bell A. Effects of freezing on DPT and DPT-IPV vaccines, adsorbed. Can Commun Dis Rep. 1995;21:101–03.
- Sitrin RD, Zhao Q, Potter CS, Carragher B, Washabaugh MW. Recombinant virus-like particle protein vaccines. In: Nunnally B, Turula V, Sitrin R, editors. Vaccine analysis: strategies, principles, and control. Berlin, Heidelberg: Springer; 2015. p. 81–112. https://doi.org/10.1007/978-3-662-45024-6_3.
- Shank-Retzlaff M, Wang F, Morley T, Anderson C, Hamm M, Brown M, Rowland K, Pancari G, Zorman J, Lowe R. Correlation between mouse potency and in vitro relative potency for human papillomavirus type 16 virus-like particles and gardasil vaccine samples. Hum Vaccin. 2005;1(5):191–97. doi:10.4161/hv.1.5.2126.
- Rimaniol AC, Gras G, Verdier F, Capel F, Grigoriev VB, Porcheray F, Sauzeat E, Fournier JG, Clayette P, Siegrist CA. Aluminum hydroxide adjuvant induces macrophage differentiation towards a specialized antigen-presenting cell type. Vaccine. 2004;22(23):3127–35. doi:10.1016/j.vaccine.2004.01.061.
- Li H, Nookala S, Re F. Aluminum hydroxide adjuvants activate caspase-1 and induce IL-1beta and IL-18 release. J Immunol. 2007;178(8):5271. doi:10.4049/jimmunol.178.8.5271.
- Floris I, Appel K, Rose T, Lejeune B. LARTH®, a micro-immunotherapy medicine, exerts anti-inflammatory effects in vitro and reduces TNF-α and IL-1β secretion. J Inflam Res. 2018;11:397–460. doi:10.2147/JIR.S174326.
- Matthias DM, Robertson J, Garrison MM, Newland S, Nelson C. Freezing temperatures in the vaccine cold chain: a systematic literature review. Vaccine. 2007;25(20):0–3986. doi:10.1016/j.vaccine.2007.02.052.
- Braun LJ, Tyagi A, Perkins S, Carpenter J, Sylvester D, Guy M, Kristensen D, Chen D. Development of a freeze-stable formulation for vaccines containing aluminum salt adjuvants. Vaccine. 2009;27(1):72–79. doi:10.1016/j.vaccine.2008.10.027.
- Solanki VA, Jain NK, Roy I. Stabilization of tetanus toxoid formulation containing aluminium hydroxide adjuvant against agitation. Int J Pharm. 2012;423(2):297–302. doi:10.1016/j.ijpharm.2011.11.039.
- Fortpied J, Wauters F, Rochart C, Hermand P, Hoet B, Moniotte N, Vojtek I. Stability of an aluminum salt-adjuvanted protein d-conjugated pneumococcal vaccine after exposure to subzero temperatures. Hum Vaccin Immunother. 2018;14(5):1243–50. doi:10.1080/21645515.2017.1421878.
- Boros CA, Hanlon M, Gold MS, Roberton DM. Storage at −3 °C for 24 h alters the immunogenicity of pertussis vaccines. Vaccine. 2001;19(25–26):3537–42. doi:10.1016/S0264-410X(01)00063-9.
- Bolgiano B, Mawas F, Yost SE, Crane DT, Lemercinier X, Corbel MJ. Effect of physico-chemical modification on the immunogenicity of Haemophilus influenzae type b oligosaccharide–CRM (197) conjugate vaccines. Vaccine. 2001;19(23–24):3189–200. doi:10.1016/S0264-410X(01)00024-X.
- Diminsky D, Moav N, Gorecki M, Barenholz Y. Physical, chemical and immunological stability of CHO-derived hepatitis B surface antigen (HBsAg) particles. Vaccine. 1999;18(1–2):3–17. 27. doi:10.1016/S0264-410X(99)00149-8.