ABSTRACT
The class II membrane fusion induced by flavivirus E proteins is a unique pH-dependent membrane fusion process differently from the class I or III membrane fusion by other enveloped virus proteins. The fusion peptide on the DII of the flavivirus E proteins can insert into the cell membrane as a cell entry process besides the receptor bindings. A traditional assay using C6/36 mosquito cells infected by dengue viruses has been previously reported but did not provide efficient quantitation to measure the virus-triggered membrane fusion. Here we reported the development of a quantitative cell fusion assay for four serotypes of dengue viruses and the recently emerged Zika viruses. We used a pCI-neo vector encoding the prME genes of dengue and Zika viruses and investigated the cell fusion in transfected 293, Vero and CHO cells. Donor cells were co-transfection of the prME genes of dengue and Zika prME gene and T7 RNA polymerase to react with the indicator cells transfected with the luciferase gene under the control of the T7 promoter. Quantification of the virus-induced cell fusion was determined by the luciferase expression levels under a switch of pH from 7.4 to 5.4 in the co-cultured donor and indicator cells. The quantitative luciferase-based assay was applied to measure the anti-fusion activity by two monoclonal antibodies mAb 4G2 and mAb DB42 against dengue virus infections. This assay could quality as a quantitative bioassay for testing the potency of anti-fusion monoclonal antibodies.
KEYWORDS:
Introduction
Dengue viruses (DENVs) and Zika viruses (ZIKVs) are small enveloped positive-strand RNA viruses belonging to the Flavivirus genus of the Flaviviridae family.Citation1 The RNA genome of flaviviruses encodes three structural genes (core C, membrane precursor prM, and envelope E) and seven non-structural genes (NS1, NS2A, NS2B, NS3, NS4A, NS4B, and NS5), with two untranslated regions (UTR) genes flanking the 5′ and 3′ ends.Citation1 The E protein has a “herringbone” structure consisting of 180 subunits of a head-to-tail dimer (90 prefusion dimers). Each monomer is composed of three distinct domains: a central beta-barrel domain I (DI), an extended finger-like dimerization domain II (DII), and an immunoglobulin-like domain III (DIII).Citation2,Citation3 The E protein dimer is the major viral protein involved in receptor binding and membrane fusion for cell entry.Citation2,Citation3 The membrane fusion is mediated by the DII fusion loop (FL) from the pre-fusion E dimer to the post-fusion E trimer through a pH-dependent conformational change, resulting in inserting FL peptides to the endosomal membranes.Citation2,Citation3
Flavivirus E protein-mediated membrane fusion belongs to the class II fusion that is different from the class I fusion triggered by the influenza virus hemagglutinin (HA) protein, or the class III membrane fusion triggered by the vesicular stomatitis virus G protein.Citation4 The FL peptides of class II fusion proteins are located at an internal single loop formed by antiparallel β-strand and stabilized by disulfide bonds.Citation5 The FL peptides of most class I proteins with the N-terminal end by proteolytic processing, or from the FL peptides of class III proteins with internal bipartite loops.Citation5 The class II fusion biochemical process is thus structurally different from the class I and III fusion processes.Citation5 The FL sequences for all flaviviruses mostly consist of 16 conserved amino acids of DRGWGNXCGXFGKGXX (with X representing variable amino acids), mostly hydrophobic amino acids with a high glycine content and a salt bridge with K110 residue.Citation3,Citation6 Several small-molecule inhibitors screened from chemical libraries have been reported to prevent the membrane fusion required for DENV, ZIKV, or other flavivirus infections.Citation7 Citation8 Citation9 Citation10 Citation11 Citation13 Citation14 Citation15–Citation16 Since the membrane fusion is required for DENV infection, several anti-fusion human monoclonal antibodies (mAbs) derived from patients with secondary infections but not primary infections were reported to present high-avidity and potent neutralization.Citation17,Citation18 These high-avidity and potent neutralizing anti-fusion mAbs would be expected to be protective in vivo.
Determination of the viral envelope protein(s)-mediated membrane fusion can be accessed using the cell–cell fusion assay with (a) a donor cell expressing viral fusion proteins (eg. HTLV-I env or herpes virus gD, gB, gH/gL) and the T7 RNA polymerase, and (b) an indicator cell that can express the reporter gene under the control of the T7 promoter.Citation19,Citation20 The subsequent cell–cell fusion between the donor cells and the indicator cells in the co-cultured system can result in the reporter gene activation for quantitative detection.Citation19,Citation20 Such a reporter cell–cell fusion assay has been established to measure the envelope-mediated fusion of the hepatitis C virus (also a flavivirus) E1E2.Citation21,Citation22 In this study, we established a luciferase-based cell–cell fusion assay to quantitatively measure DENV and ZIKV membrane fusion in vitro. This assay could quality as a quantitative bioassay for the potency of anti-fusion monoclonal antibodies.
Materials and methods
Cell–cell fusion of C6/36 cells infected with DENV and ZIKV
C6/36 mosquito cells were obtained from the Bioresource Collection and Research Center (BCRC) and grown in Leibovitz-15 medium (L-15, Invitrogen) containing 10% fetal bovine serum (FBS), 0.3% tryptose phosphate broth (TPB), 1% non-essential amino acid (NEAA), 25 mM HEPES buffer, and 100 U/ml penicillin/streptomycin (P/S) at 28°C. Monolayer C6/36 cells in 6-well plates were infected with DENV1 (Hawaii strain), DENV2 (NGC strain), DENV3 (H87 strain), DENV-4 (infectious clone strain 814669), and ZIKV (PRVABC59 strain) at an MOI of 0.2. After 7 d post-infection (dpi), the medium was replaced by growth medium with 10 mM MES at pH 5.4, 5.8, or 6.2 or growth medium with 10 mM HEPES at pH 6.6, 7.0, or 7.4 for 2 h at 40°C. Cells were washed with PBS three times and stained with Liu’s stain. Fusion was photographed by the IX70 microscope (Olympus).
Construction of prME expression plasmids
The cDNAs of DENV1, DENV2, DENV3, DENV4, or ZIKV containing the prME gene fragment was used as the source of prME. The full-length prME gene and the 3ʹ 60-nucleotide region of the C gene of each virus were inserted between the EcoRI and KpnI sites of the pCI-neo vector (Promega). The expression of prME was under the control of the cytomegalovirus (CMV) promoter. For the pCI-D2prME-VSVG, pCI-D2prME-CD4 pCI-ZprMEVSVG, or pCI-ZprMECD4 vector, the transmembrane and cytoplasmic domain (TM) of the DENV2 or ZIKV E gene were replaced with the TM gene of the VSV G or the CD4 receptor respectively by side-direct mutagenesis.
Cell–cell fusion assay with a T7 pol luciferase reporter gene
As the donor cells, 293A cells were seeded in 96-well plates at a density of 2 × 10Citation4 cells/well at 37°C overnight and then transfected with 0.2 µg of expression plasmids pCI-prME together with 0.1 µg of T7 RNA polymerase-expressing plasmid pCAGT7pol. As effector cells, 293A, CHO-K1, Vero, or BHK-21 cells were seeded in 6-well plates at a density of 106 cells/well at 37°C overnight and then co-transfected with 4 µg of reporter plasmid pT7EMCVLuc and 0.4 µg of control plasmid pRL-TK (Promega). The PT7EMCVLuc plasmid contains a firefly luciferase gene under the control of the T7 promoter.Citation23 The pRL-TK plasmid has a Renilla luciferase gene with the regulation of thymidine kinase (TK) promoter as an internal control.Citation23 After 72 h post-transfection, indicator cells were detached by 0.02% EDTA in PBS and added to the effector cells. After 5 h of incubation at 37°C, the co-cultured cells were incubated with fusion medium (PBS with 10 mM MES at pH 5.4, 5.8, or 6.2 or PBS with 10 mM HEPES at pH 6.6, 7.0, or 7.4) for 5 min at 37°C and then incubated with growth medium for 7 h. For the anti-fusion activity assay, anti-fusion mAbs were treated with the co-cultured cells for 1 h at 37°C before being incubated in fusion medium. Firefly and Renilla luciferase activities were measured by a dual-luciferase reporter assay system (Promega) according to the manufacturer’s instructions. Relative luminometer units (RLUs) were measured with a VICTOR3 Multi-labeled Microplate Reader (PerkinElmer). The cell fusion activity was determined by firefly luciferase activity and normalized to the activity of Renilla luciferase.
Statistical analysis
Statistical analyses were performed using GraphPad Prism (GraphPad Software, Inc.). The statistical significance of differences between groups was assessed using one-way ANOVAs. Differences with a p value of less than 0.05 (*) were considered statistically significant. All experiments were repeated at least twice.
Results and discussion
C6/36 mosquito cells infected with DENV and ZIKV for cell–cell fusion activity
To demonstrate the pH-dependent cell–cell membrane fusion triggered by DENV and ZIKV infection, C6/36 mosquito cells were grown in the medium at pH 5.4, 5.8, 6.2, 6.6, 7.0, or 7.4, and infected with DENV or ZIKV at an MOI = 0.2. The results indicated that mosquito C6/36 cells infected with DENV1, DENV2, DENV3, and DENV4 triggered cell–cell fusion at the pH range from 5.4 to 6.6. The infected cells at 7 dpi were stained with Liu’s stain and showed the formation of syncytia triggered by membrane-membrane fusion as shown in . Similarly, mosquito C6/36 cells infected with ZIKV were also found to induce the membrane fusion-induced syncytia formation at the pH range from 5.8 to 6.6 in the infected cells (). Therefore, the pH-dependent cell–cell fusion was demonstrated in C6/36 mosquito cells infected with DENV and ZIKV.
A quantitative luciferase-based cell–cell fusion assay for DENV2
To develop a quantitative cell–cell fusion assay for DENV or ZIKV infection, we first constructed a pCI-neo vector encoding the prME genes of DENV2 NGC strain (pCI-DENV2prME) and a T7 RNA polymerase-expressing plasmid pCAGT7pol for co-transfection to the donor cells (). We also constructed a pT7EMCVluc plasmid encoding the firefly luciferase gene under the transcriptional control of a T7 promoter, and a pRL-TK plasmid encoding the Renilla luciferase gene under the TK promoter for co-transfection to the indicator cells (). The transfected donor and indicator cells were co-cultured to trigger the cell–cell fusion as detected by firefly luciferase expression (). The Renilla luciferase activity was measured in the co-cultured cells as the internal negative control (). The donor 293A cells were co-transfected with pCI-DENV2prME and pCAGT7pol plasmids. The indicator 293A cells were co-transfected with pT7EMCVLuc and pRL-TK plasmids. The results showed that the firefly luciferase expression triggered by DENV2prME cell–cell fusion was at the highest levels around 180–200 RLU at pH5.4 and pH5.8 (). In contrast, the internal control for the Renilla luciferase activity remained approximately the same levels under all pH conditions tested (). Our results indicated that the luciferase-based cell–cell fusion activity triggered by the DENV2 E protein was demonstrated to be feasible, but the luciferase expression levels were significantly lower compared to those reported for HCV E1E2 and Influenza virus HA proteins.Citation24,Citation25 One possibility is that DENV prME may be expressed poorly on the cell surface. DENV contains an endoplasmic reticulum (ER) retention signal on the TM domain of the E protein that can cause prME proteins to remain mainly in the ER.Citation26,Citation27
Figure 2. Schema of luciferase-based cell–cell fusion assay.
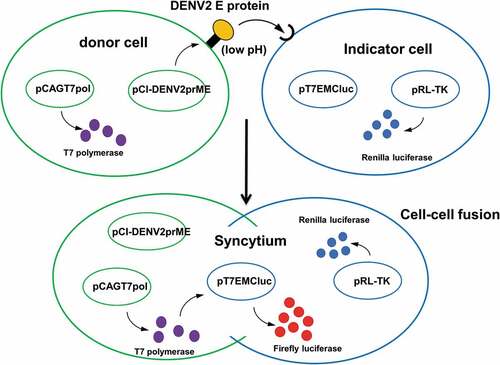
Figure 3. pH dependence of cell–cell fusion mediated by D2prME.
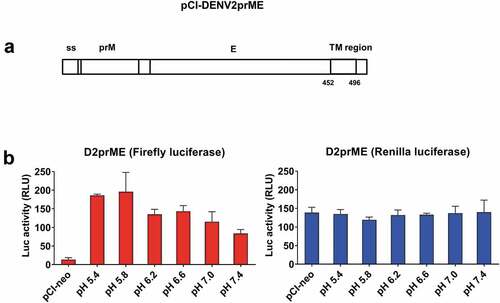
Replacement of the transmembrane and cytoplasmic domain with the VSV G and CD4 genes for vector construction and the use of different indicator cell types
It was reported that the membrane targeting signals may present as an ER retention signalCitation26,Citation27 and affect the cell–cell membrane fusion as reported for the retrovirus-pseudotyped DENVs.Citation28 The pCI-DENV2prME vector was further constructed where the transmembrane and cytoplasmic domain (TM) of the DENV2 E gene were replaced with the TM gene of the VSV G (D2prME-VSVG) or the CD4 receptor (D2prME-CD4), respectively (). The results showed that the replacement with the VSV G TM gene for the D2prME-VSVG vector resulted in a similar pattern for pH-dependent firefly luciferase expression, the highest levels at pH5.4 and pH5.8 around 120–140 RLU which were 30-40% lower than the use of pCI-DENV2prME construct (). The internal control for the Renilla luciferase expression remained the same levels for detection (). However, the replacement with the CD4 TM gene for the D2prME-CD4 vector did not trigger cell–cell membrane fusion under all pH conditions for firefly luciferase expression as well as the internal control for consistent Renilla luciferase expression (). The replacement of the transmembrane and cytoplasmic domain of DENV prME with the VSV G and CD4 genes did not increase fusion activity. Since DENV also contains an ER retention signal at the stem region of the E protein as reported,Citation26,Citation27 we may need to replace both the stem and TM domain on the E protein to enhance the fusion activity in this cell-based reporter assay.
Figure 4. Fusion activity of pCI-D2prME-VSVG and pCI-D2prME-CD4 and the use of different indicator cell types.
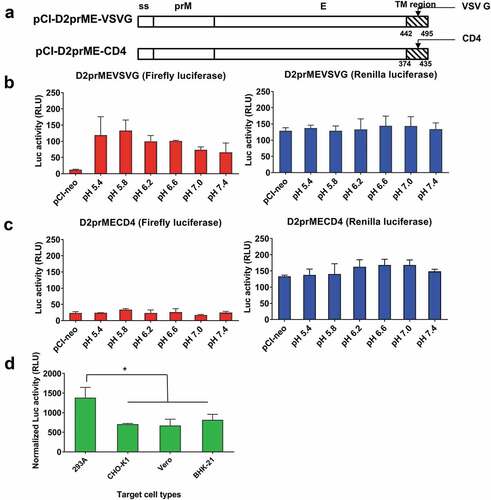
We also examined the use of other indicator cells, including CHO-K1, Vero, and BHK-21 cells, as compared to 293A cells, transfected by the-DENV2prME vector for the expression of firefly and Renilla luciferase activity (as the normalized RLU activity). The 293A indicator cells were co-cultured with the donor cell lines of 293A, CHO-K1, Vero, or BHK-21 cells at pH-5.8 and determine the expression of normalized luciferase activity triggered by cell–cell fusion. Among these four indicator cells, CHO-K1, Vero, and BHK-21 cells had relatively lower fusion activity compared to the use of 293A cells ().
A quantitative luciferase-based cell–cell fusion assay for DENV1, DENV3, and DENV4 serotypes
We further constructed the pCI-neo vector encoding the prME genes of the other three DENV serotypes (pCI-DENV1prME, pCI-DENV3prME, pCI-DENV4prME) and co-transfected with pCAGT7pol into 293A donor cells. After co-cultures with the 293A indicator cells transfected with pT7EMCVluc and pRL-TK at pH 5.8, the expression levels of firefly luciferase were measured as shown in . All of these three serotype vectors were able to induce the expression of luciferase activity triggered by the viral E protein-mediated cell–cell fusion in 293A cells (). We found no significant differences in the firefly luciferase expression levels among DENV1, DENV3, and DENV 4 ().
A quantitative luciferase-based cell–cell fusion assay for ZIKV
To evaluate whether the luciferase-based cell–cell fusion could be mediated by ZIKV prME, the 293A indicator cells were co-transfected with the pCI-ZprME and pCAGT7pol plasmids and co-cultured with 293A donor cells to investigate the cell–cell fusion between the donor and indicator cells at pH 5.4, 5.8, 6.2, 6.6, 7.0, or 7.4. Results indicated that no pH-dependent cell–cell membrane fusion activity was observed under the pH conditions tested (). We further replaced the TM domain of the ZIKV E gene with the VSV G (pCI-ZprMEVSVG) or the CD4 (pCI-ZprMECD4). The results again indicated that no pH-dependent cell–cell membrane fusion activity was detected for these constructs (). The luciferase-based cell–cell fusion assay failed in detecting the cell–cell membrane fusion triggered by ZIKV E proteins. In contrast, the cell–cell fusion activity was shown in the mosquito C6/36 cells infected with ZIKV (). It is likely that the inherent E sequence for ZIKV in the donor cells may hinder the cell–cell membrane fusion triggered by low pH conditions. According to the high-resolution ZIKV E protein structure determined by cryo-EM, the ZIKV E is more thermally stable than DENV E and has a glycan-protruding loop with an insertion of five residues in DI that is adjacent to the fusion loop.Citation29,Citation30 The DII of ZIKV E protein, also unlike the DII of DENV E, dips slightly closer to the viral membrane.Citation29 All of these differences may account for the failure to establish the luciferase-based cell–cell fusion assay for ZIKV infection.
Application for anti-fusion monoclonal antibodies against DENV2
We used two anti-DENV fusion mAbs 4G2 and DB42, to demonstrate the application of the quantitative luciferase-based cell assay for in vitro inhibition studies. The mAb 4G2 was obtained from the culture supernatant of ATCC HB-112 hybridoma cells and purified by recombinant Protein G Agarose (Invitrogen). The transfected donor and indicator cells were co-cultured and incubated with 1 mg/ml of mAb 4G2 or mAb DB42, as compared to the PBS control. Results indicated that the expression levels of firefly luciferase in co-cultured 293A cells were significantly reduced by mAb 4G2 and mAb DB42, but not the PBS-treated control group (). Although the differences in inhibitory activity between mAb 4G2 and mAb DB42 compared to the PBS control are significant, we did not observe the inhibition activity using at lower mAb concentrations and DENV antisera (data not shown). This quantitative luciferase-based fusion bioassay still needs further improvement to amply the signals to detect anti-fusion antibodies against DENV infection. An improvement over this anti-fusion assay has been reported by measuring the fusion of DENV and ZIKV virions with trypsin-loaded liposomes using the digestion of the viral core proteins.Citation12–Citation16 The particle-liposome assay may be applicable to measure anti-fusion activity by mAbs and DENV antisera.
Conclusion
We developed a luciferase-based cell–cell fusion assay to quantify the potency of anti-fusion activity with a simple, rapid, and quantitative T7 luciferase reporter gene to substitute C6/36 cell infection fusion assay. All four serotypes of DENV were found to trigger fusion activity using the luciferase-based cell–cell fusion assay. The limitations of this bioassay include (1) low levels for pH-dependent firefly luciferase expression by four-serotype DENV E proteins, (2) no pH-dependent firefly luciferase expression by ZIKV E protein, and (3) no detectable levels of anti-fusion antibodies in DENV antisera. However, this luciferase-based assay could quality as a quantitative bioassay for testing the potency of anti-fusion monoclonal antibodies.
Disclosure of potential conflicts of interest
No potential conflicts of interest were disclosed.
Acknowledgments
We thank Yoshihara Matsuura of Osaka University for pCAGT7 and pT7EMCluc vector plasmids and Han-Chun Wu of Academia Sinica for mAb DB42.
Additional information
Funding
References
- Lindenbach BD, Rice CM. Molecular biology of flaviviruses. Adv Virus Res. 2003;59:23–61.
- Modis Y, Ogata S, Clements D, Harrison SC. Structure of the dengue virus envelope protein after membrane fusion. Nature. 2004;427(6972):313–19. doi:10.1038/nature02165.
- Rey FA, Heinz FX, Mandl C, Kunz C, Harrison SC. The envelope glycoprotein from tick-borne encephalitis virus at 2 A resolution. Nature. 1995;375(6529):291–98. doi:10.1038/375291a0.
- Harrison SC. Viral membrane fusion. Nat Struct Mol Biol. 2008 Jul;15(7):690–98. doi:10.1038/nsmb.1456.
- White JM, Delos SE, Brecher M, Schornberg K. Structures and mechanisms of viral membrane fusion proteins: multiple variations on a common theme. Crit Rev Biochem Mol Biol. 2008;43:189–219. doi:10.1080/10409230802058320.
- Seligman SJ. Constancy and diversity in the flavivirus fusion peptide. Virol J. 2008;5:27. doi:10.1186/1743-422X-5-27.
- Zhou Z, Khaliq M, Suk JE, Patkar C, Li L, Kuhn RJ, Post CB. Antiviral compounds discovered by virtual screening of small-molecule libraries against dengue virus E protein. ACS Chem Biol. 2008;3:765–75. doi:10.1021/cb800176t.
- Kampmann T, Yennamalli R, Campbell P, Stoermer MJ, Fairlie DP, Kobe B, Young PR. In silico screening of small molecule libraries using the dengue virus envelope E protein has identified compounds with antiviral activity against multiple flaviviruses. Antiviral Res. 2009;84:234–41. doi:10.1016/j.antiviral.2009.09.007.
- Poh MK, Yip A, Zhang S, Priestle JP, Ma NL, Smit JM, Wilschut J, PY S, MR W, Schul W. A small molecule fusion inhibitor of dengue virus. Antiviral Res. 2009;84:260–66.
- Schmidt AG, Yang PL, Harrison SC. Peptide inhibitors of dengue-virus entry target a late-stage fusion intermediate. PLoS Pathog. 2010;6:e1000851. doi:10.1371/journal.ppat.1000851.
- Schmidt AG, Lee K, Yang PL, Harrison SC. Small-molecule inhibitors of dengue-virus entry. PLoS Pathog. 2012;8:e1002627. doi:10.1371/journal.ppat.1002627.
- De Wispelaere M, Lian W, Potisopon S, Li PC, Jang J, Ficarro SB, Clark MJ, Zhu X, Kaplan JB, Pitts JD, et al. Inhibition of flaviviruses by targeting a conserved pocket on the viral envelope protein. Cell Chem Biol. 2018;25(8):1006–1016.e8. doi:10.1016/j.chembiol.2018.05.011.
- Chao LH, Jang J, Johnson A, Nguyen A, Gray NS, Yang PL, Harrison SC. How small-molecule inhibitors of dengue-virus infection interfere with viral membrane fusion. Elife. 2018;7:ii:e36461. doi:10.7554/eLife.36461.
- Lian W, Jang J, Potisopon S, Li PC, Rahmeh A, Wang J, Kwiatkowski NP, Gray NS, Yang PL. Discovery of immunologically inspired small molecules that target the viral envelope protein. ACS Infect Dis. 2018;4(9):1395–406. doi:10.1021/acsinfecdis.8b00127.
- Li PC, Jang J, Hsia CY, Groomes PV, Lian W, de Wispelaere M, Pitts JD, Wang J, Kwiatkowski N, Gray NS, et al. Small molecules targeting the flavivirus E protein with broad-spectrum activity and antiviral efficacy in Vivo. ACS Infect Dis. 2019;5(3):460–72. doi:10.1021/acsinfecdis.8b00322.
- Pitts J, Hsia CY, Lian W, Wang J, Pfeil MP, Kwiatkowski N, Li Z, Jang J, Gray NS, Yang PL. Identification of small molecule inhibitors targeting the Zika virus envelope protein. Antiviral Res. 2019;164:147–53. doi:10.1016/j.antiviral.2019.02.008.
- Tsai WY, Chen HL, Tsai JJ, Dejnirattisai W, Jumnainsong A, Mongkolsapaya J, Screaton G, Crowe JE Jr, Wang WK. Potent neutralizing human monoclonal antibodies preferentially target mature dengue virus particles: implication for novel strategy for dengue vaccine. J Virol. 2018;92(23):e00556e18. doi:10.1128/JVI.00556-18.
- Tsai WY, Lai CY, Wu YC, Lin HE, Edwards C, Jumnainsong A, Kliks S, Halstead S, Mongkolsapaya J, Screaton GR, et al. High-avidity and potently neutralizing cross-reactive human monoclonal antibodies derived from secondary dengue virus infection. J Virol. 2013;87(23):12562–75. doi:10.1128/JVI.00871-13.
- Okuma K, Nakamura M, Nakano S, Niho Y, Matsuura Y. Host range of human T-cell leukemia virus type I analyzed by a cell fusion-dependent reporter gene activation assay. Virology. 1999;254(2):235–44. doi:10.1006/viro.1998.9530.
- Saw WT, Matsuda Z, Eisenberg RJ, Cohen GH, Atanasiu D. Using a split luciferase assay (SLA) to measure the kinetics of cell-cell fusion mediated by herpes simplex virus glycoproteins. Methods. 2015;90:68–75. doi:10.1016/j.ymeth.2015.05.021.
- Kobayashi M, Bennett MC, Bercot T, Singh IR. Functional analysis of hepatitis C virus envelope proteins, using a cell-cell fusion assay. J Virol. 2006;80(4):1817–25. doi:10.1128/JVI.80.4.1817-1825.2006.
- Denolly S, Cosset FL, Freitas N. Membrane fusion assays for studying entry hepatitis C virus into cells. Methods Mol Biol. 2019;1911:219–34.
- Aoki Y, Aizaki H, Shimoike T, Tani H, Ishii K, Saito I, Matsuura Y, Miyamura T. A human liver cell line exhibits efficient translation of HCV RNAs produced by a recombinant adenovirus expressing T7 RNA polymerase. Virology. 1998;250(1):140–50. doi:10.1006/viro.1998.9361.
- Su Y, Yang H, Zhang B, Qi X, Tien P. A dual reporter gene based system to quantitate the cell fusion of avian influenza virus H5N1. Biotechnol Lett. 2008;30(1):73–79. doi:10.1007/s10529-007-9521-4.
- Takikawa S, Ishii K, Aizaki H, Suzuki T, Asakura H, Matsuura Y, Miyamura T. Cell fusion activity of hepatitis C virus envelope proteins. J Virol. 2000;74(11):5066–74. doi:10.1128/JVI.74.11.5066-5074.2000.
- SC H, IJ L, CC K, GJ C, Wang WK. A strong endoplasmic reticulum retention signal in the stem-anchor region of envelope glycoprotein of dengue virus type 2 affects the production of virus-like particles. Virology. 2008;374(2):338–50. doi:10.1016/j.virol.2007.12.041.
- Purdy DE, Chang GJ. Secretion of noninfectious dengue virus-like particles and identification of amino acids in the stem region involved in intracellular retention of envelope protein. Virology. 2005;333(2):239–50. doi:10.1016/j.virol.2004.12.036.
- HP H, SC H, CC K, Wang WK. Characterization of retrovirus-based reporter viruses pseudotyped with the precursor membrane and envelope glycoproteins of four serotypes of dengue viruses. Virology. 2007;368(2):376–87. doi:10.1016/j.virol.2007.06.026.
- Kostyuchenko VA, Lim EX, Zhang S, Fibriansah G, Ng TS, Ooi JS, Shi J, Lok SM. Structure of the thermally stable Zika virus. Nature. 2016;533(7603):425–28. doi:10.1038/nature17994.
- Sirohi D, Chen Z, Sun L, Klose T, Pierson TC, Rossmann MG, Kuhn RJ. The 3.8 A resolution cryo-EM structure of Zika virus. Science. 2016;352(6284):467–70. doi:10.1126/science.aaf5316.