ABSTRACT
The novel coronavirus SARS-CoV-2 emerged in China in 2019 and quickly spread globally, causing a pandemic. There is an urgent need to develop vaccines against the virus, and both convalescent plasma and immune globulin are currently in clinical trials for treatment of patients with COVID-19. It is unclear whether antibodies induced by SARS-CoV-2 have neutralizing capacity and whether they can protect from future infection. Seasonal human coronaviruses (HCoV) have been circulating for decades. It is currently unknown whether antibodies against seasonal HCoV may cross-neutralize SARS-CoV-2. Data from neonates suggest that trans-placental antibodies against HCoV may have neutralizing capacity. Here we briefly review the epidemiologic observations on HCoV and discuss the potential implications for neutralizing and cross-neutralizing antibodies against SARS-CoV-2.
KEYWORDS:
As states across America prepare to reopen, one of the critical questions in the management of COVID-19 is the individual susceptibility to infection. SARS-CoV-2 is a novel coronavirus that emerged in China in December 2019 and has since created a public health crisis (COVID-19) globally. While symptoms of SARS-CoV-2 infection may be milder than those seen in previous pandemics caused by other coronaviruses, including Middle East Respiratory Syndrome (MERS) and Severe Acute Respiratory Syndrome (SARS-CoV), worldwide, SARS-CoV-2 has killed more people than MERS and SARS-CoV combined. This is thought to be due to the efficient person-to-person transmission of SARS-CoV-2 and lack of population-level immunity.Citation1 SARS-CoV-2 infection appears to induce an antibody response in the patient; however, it is not clear whether these antibodies prevent re-infection.
Based on their serological relationships, human coronaviruses (HCoV) fall into two groups ().Citation2 Serological cross-reactivity has been reported between viruses in the same group.Citation3 HCoV-229E (group 1) and HCoV-OC43 (group 2) were identified in 1962 and 1967, respectively.Citation4,Citation5 Although HCoV-NL63 (group 1) was first discovered in 2004, serology data from pregnant women and their infants suggest that it was circulating before 1999.Citation6,Citation7 SARS-CoV (group 2) emerged in 2002, HCoV-HKU1 (group 2) was identified in 2004, and in 2012, MERS-CoV (group 2) was identified.Citation8–10 SARS-CoV-2 (group 2) emerged in late 2019 and, as of May 2020, is still resulting in high death rates globally.Citation11
Table 1. Group 1 and Group 2 Human coronaviruses
Although SARS and MERS caused short-lived pandemics, seasonal human coronaviruses (OC43, 229E, NL63, HKU1) have been circulating in the community for decades;Citation12 these viruses can cause upper and lower respiratory tract infections, and are considered to be the second-most frequent cause of the common cold.Citation13 Similar to SARS-CoV-2, seasonal HCoVs are enveloped, positive-sense RNA viruses with four distinct structural proteins: spike (S), membrane (M), envelope (E), and nucleocapsid (N).Citation12 Antibodies directed against S protein are proposed to have neutralizing capacity.Citation14 Although there is a striking homology between S protein of SARS-CoV and SARS-CoV-2, it is divergent from seasonal HCoV S protein.Citation15
Data on HCoV-induced antibody response and neutralizing capacity come from studies of natural infection and human challenge studies.Citation14 Infants less than 1 year old consistently account for the smallest proportion of cases due to seasonal coronaviruses ().Citation16–20 The rate of infection peaks among children ages 1–5 years, followed by those between 6 and 17 years of age ().Citation16–20 The low rate of HCoV infection in infants less than 1 year of age was attributed to the placental transfer of maternal antibodies and their neutralizing capacity.Citation3 In a longitudinal serological survey study of 25 newborns, Dikjman et al.Citation3 showed that all infants had antibodies directed to the N protein of seasonal HCoVs (OC43, 229E, NL63, HKU1) at birth, but the antibody levels decreased to low detectable levels within a few months. In a separate experiment with a larger cohort of children, the investigators confirmed that the majority (64%) of infants younger than 6 months of age had antibodies against both NL63 and 229E, which decreased over time.Citation7 Approximately 22% of those who were 12 months old were seropositive.Citation7 The seropositive rate increased with time, and among children 3.5 to 5 years of age, the seropositivity rate rose to 65% for HCoV-229E and 75% for HCoV-NL63. The investigators concluded that maternal antibodies protect infants from seasonal HCoV and most children become susceptible to infection between 18 and 42 months of age.Citation7 The observations from SARS-CoV-2 exposed infants are similar; transmission of SARS-CoV-2 to a newborn appears to be very rare when the mother is acutely infected.Citation21 In infants, in addition to the differences in angiotensin converting enzyme 2 (ACE2) receptor expression, trans-placental antibodies may provide protection against SARS-CoV-2.
Table 2. Mean Age of Pediatric Coronavirus Infections
Data from the 2003 SARS-CoV pandemic also suggest a potential role for neutralizing antibodies in the current SARS-CoV-2 outbreak. In 2003, convalescent plasma treatment led to a shorter hospital stay and lower mortality among those with SARS-CoV.Citation22 Recently, in China, treatment with convalescent plasma with high titers of receptor binding domain specific IgG and IgM was reported to improve the clinical outcomes in patients with SARS-CoV-2 infection.Citation23 Convalescent plasma has recently been shown to be effective for the treatment of COVID-19 in clinical trials, and intravenous immune globulin is currently being tested for the treatment of pediatric patients with Multi-System Inflammatory Syndrome (MIS-C) related to SARS-CoV-2.Citation24,Citation25
Cross-neutralizing antibodies directed to N protein have been recognized among seasonal HCoV.Citation3 In a sequence of seroconversion study in children, prior infection with OC43 (group 2) was observed to be protective from infection with HKU1 (group 2). However, the opposite was not true; those previously infected with HKU1 were not protected against infection with OC43.Citation3 Longitudinal observational studies show that the incidence of different HCoVs oscillates from year to year, especially for OC43 and HKU1 (group 2), which make approximately half of all HCoV infections every year ().Citation20,Citation26 If one of the group 2 viruses dominates 1 year, the other group 2 virus appears to dominate the following year ().Citation26 In addition, if it was a particularly bad season with group 2 viruses (such as 2012–13 and 2014–15), the following season, group 2 viruses did not appear to be as frequent ().Citation26 A similar pattern was observed by Talbot et al.Citation27 who reported the incidence of human coronaviruses (OC43, NL63 and 229E) in children over a 20 year period and by Killerby et al.Citation20 who examined The National Respiratory and Enteric Virus Surveillance System (NREVSS) data on laboratory detected HCoV in the United States during 2014–2017. These data suggest that recent exposure and immune response to one group 2 HCoV may influence the susceptibility to infection with another group 2 virus. SARS-CoV and SARS-CoV-2 are group 2, lineage B coronaviruses.Citation28 Antibodies induced by infection with seasonal group 2, lineage A HCoV may provide cross-protection to infection with SARS-CoV and SARS-CoV-2 and lead to milder or asymptomatic disease.Citation29 So far, there are limited data on the circulating seasonal HCoV during fall/winter 2019–2020. Recently Nowak et al.Citation30 suggested that NL63 (n = 23) and HKU1 (n = 13) were the main group 1 and group 2 viruses circulating in the New York area between March 16 – April 20, 2020.
Figure 1. Incidence of 4 Seasonal Coronaviruses (OC43, 229E, NL63, HKU1) at the University of Michigan Health Care System between 2010–2018
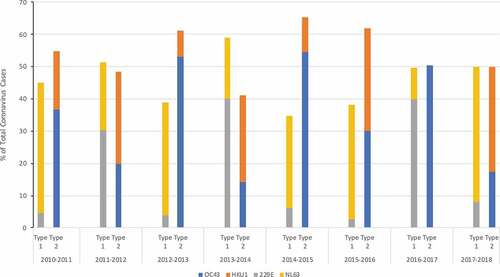
SARS-CoV-2 and SARS-CoV share 74.5% genome identity, and a SARS-CoV serology assay may give false-positive results in 85% of SARS-CoV-2 patients within 10 days of onset of illness.Citation31 In in vitro experiments, serum from a patient who recovered from SARS moderately inhibited the transduction by SARS-CoV-2 pseudovirion.Citation32 Pinto et al.Citation33 recently identified a monoclonal antibody from memory B cells of an individual who was infected with SARS-CoV in 2003; the antibody potently neutralized SARS-CoV-2 and SARS-CoV pseudoviruses as well as SARS-CoV-2 in vitro.Citation33 Sera from patients who recovered from SARS-CoV was proposed for the treatment of patients with SARS-CoV-2 infection.Citation34
Recent data from de Assis, et al.Citation35 showed that sera from patients with SARS-CoV-2 infection (n = 7) contained IgG and IgA antibodies reacting with S protein of OC43 (group 2) and 229E (group 1). There appears to be some homology between N protein of SARS-CoV and seasonal HCoV.Citation36 Zhao et al.Citation37 showed that sera collected from two individuals 1 year prior to the SARS pandemic contained N protein specific antibodies that cross-reacted with SARS-CoV. Serum samples obtained from patients who recently recovered from SARS-CoV had antibodies that cross-reacted in vitro with nucleocapsid proteins of HCoV-OC43 and 229E.Citation38 It is important to further understand the neutralizing effect of antibodies directed against viral proteins other than S-protein on SARS-CoV-2 pathogenesis.
As vaccines and immunotherapeutics are developed against SARS-CoV-2, epidemiologic data are needed on the temporal pattern of SARS-CoV-2 and its relationship with seasonal HCoV.Citation14 The annual incidence of combined group 1 and combined group 2 HCoV disease appears to be stable and distributed equally over time, but the predominant group 2 virus causing disease, OC43 versus HKU1, appears to alternate from year to year ().Citation26 Though SARS-CoV-2 is another group 2 coronavirus, it belongs to a different lineage as OC43 and HKU1 () and is not known whether the immune response to circulating seasonal HCoV will have any influence on SARS-CoV-2 emergence and severity in the upcoming year. Data on seasonal HCoVs that circulated during the fall/winter of 2019–2020 will be very useful, and may be generated by using frozen patient samples stored in influenza registries.
The Center for Disease Control (CDC) has begun to track and report weekly SARS-CoV-2 activity in COVIDView.Citation39 Going forward, epidemiologic data on SARS-CoV-2 may be examined within the context of data on seasonal HCoV and other respiratory tract viruses, since they frequently co-infect patients.Citation20 Gorse et al.Citation40 demonstrated that in a small cohort of older adults (n = 15; 60 yr and older) with respiratory tract infections, acute infection with HCoV induced neutralizing antibodies in 46% of the individuals. The elderly in nursing homes may be isolated and less exposed to seasonal HCoV, and they may have lower level of antibodies at the beginning of a season. As we better understand the role of neutralizing and cross-neutralizing antibodies, in the future, sensitive and specific quantitative seasonal HCoV serology assays may be incorporated into patient care to help determine an individual’s risk for severe SARS-CoV-2 infection.
In addition, there is an urgent need to collect longitudinal seasonal HCoV and SARS-CoV-2 serology data on all pregnant women and their infants to understand the role of antibodies to prevent neonatal infections. In pregnant women who are infected with SARS-CoV-2, it is also necessary to understand the timing and efficiency of transplacental antibody transfer, the factors that impair the transfer, as well as the half-life of the maternal antibody in the neonate.Citation41
These data collected will be useful to develop safe and effective immunotherapies and vaccines against SARS-CoV-2.
Disclosure of potential conflicts of interest
The authors report no conflicts of interest.
Additional information
Funding
References
- Kwok KO, Lai F, Wei WI, Wong SYS, Tang JWT. Herd immunity – estimating the level required to halt the COVID-19 epidemics in affected countries. J Infect. 2020 Mar 21 [accessed 2020 May 10]; 2. doi:10.1016/j.jinf.2020.03.027.
- Woo PCY, Wang M, Lau SKP, Xu H, Poon RWS, Guo R, Wong BHL, Gao K, Tsoi H-W, Huang Y, et al. Comparative analysis of twelve genomes of three Novel Group 2c and Group 2d Coronaviruses reveals unique group and subgroup features. J Virol. 2007;81:1574–85. doi:10.1128/JVI.02182-06.
- Dijkman R, Jebbink MF, Gaunt E, Rossen JWA, Templeton KE, Kuijpers TW, van der Hoek L. The dominance of human coronavirus OC43 and NL63 infections in infants. J Clin Virol. 2012;53:135–39. doi:10.1016/j.jcv.2011.11.011.
- Hamre D, Procknow JJ. A new virus isolated from the human respiratory tract. Proc Soc Exp Biol Med. 1966;121:190–93. doi:10.3181/00379727-121-30734.
- McIntosh K, Dees JH, Becker WB, Kapikian AZ, Chanock RM. Recovery in tracheal organ cultures of novel viruses from patients with respiratory disease. Proc Natl Acad Sci U S A. 1967;57:933–40. doi:10.1073/pnas.57.4.933.
- van der Hoek L, Pyrc K, Jebbink MF, Vermeulen-Oost W, Berkhout RJM, Wolthers KC, Wertheim-van Dillen PME, Kaandorp J, Spaargaren J, Berkhout B. Identification of a new human coronavirus. Nat Med. 2004;10:368–73. doi:10.1038/nm1024.
- Dijkman R, Jebbink MF, Idrissi NBE, Pyrc K, Muller MA, Kuijpers TW, Zaaijer HL, van der Hoek L. Human Coronavirus NL63 and 229E seroconversion in children. J Clin Microbiol. 2008;46:2368–73. doi:10.1128/JCM.00533-08.
- Drosten C, Günther S, Preiser W, van der Werf S, Brodt H-R, Becker S, Rabenau H, Panning M, Kolesnikova L, Fouchier RAM, et al. Identification of a Novel Coronavirus in patients with severe acute respiratory syndrome. N Engl J Med. 2003;348:1967–76. doi:10.1056/NEJMoa030747.
- Woo PCY, Lau SKP, Chu C-M, Chan K-H, Tsoi H-W, Huang Y, Wong BHL, Poon RWS, Cai JJ, Luk W-K, et al. Characterization and complete genome sequence of a Novel Coronavirus, Coronavirus HKU1, from patients with Pneumonia. J Virol. 2005;79:884–95. doi:10.1128/JVI.79.2.884-895.2005.
- Fehr AR, Channapannavar R, Perlman S. Middle East respiratory syndrome (MERS): emergence of a pathogenic human Coronavirus. Annu Rev Med. 2017;68:387–99. doi:10.1146/annurev-med-051215-031152.
- Singhal TA. Review of Coronavirus Disease-2019 (COVID-19). Indian J Pediatr. 2020;87:281–86. doi:10.1007/s12098-020-03263-6.
- Lim YX, Ng YL, Tam JP, Liu DX. Human Coronaviruses: a review of virus–host interactions. Diseases. 2016:4. doi:10.3390/diseases4030026.
- Boncristiani HF, Criado MF, Arruda E. Respiratory Viruses. Encycl Microbiol. 2009 Feb 17 [accessed 2020 May 21]; 18. doi:10.1016/B978-012373944-5.00314-X.
- Huang AT, Garcia-Carreras B, Hitchings MDT, Yang B, Katzelnick LC, Rattigan SM, Borgert BA, Moreno CA, Solomon BD, Rodriguez-Barraquer I, et al. A systematic review of antibody mediated immunity to coronaviruses: antibody kinetics, correlates of protection, and association of antibody responses with severity of disease. medRxiv. 2020 Apr 17 [accessed 2020 May 18]; 47. doi:10.1101/2020.04.14.20065771.
- Li F. Evidence for a common evolutionary origin of coronavirus spike protein receptor-binding subunits. J Virol. 2012;86:2856–58. doi:10.1128/JVI.06882-11.
- Varghese L, Zachariah P, Vargas C, LaRussa P, Demmer RT, Furuya YE, Whittier S, Reed C, Stockwell MS, Saiman L. Epidemiology and clinical features of human coronaviruses in the pediatric population. J Pediat Inf Dis Soc. 2018;7:151–58. doi:10.1093/jpids/pix027.
- Stockman LJ, Massoudi MS, Helfand R, Erdman D, Siwek AM, Andersosn LJ, Parashar UD. Severe acute respiratory syndrome in children. Pediatr Infect Dis J. 2007;26:68–74. doi:10.1097/01.inf.0000247136.28950.41.
- Thabet F, Chehab M, Bafaqih H, AlMohaimeed S. Middle East respiratory syndrome coronavirus in children. Saudi Med J. 2015;36:484–86. doi:10.15537/smj.2015.4.10243.
- Lu X, Zhang L, Du H, Zhang J, Li YY, Qu J, Zhang W, Wang Y, Bao S, Li Y, et al. SARS-CoV-2 infection in children. N Engl J Med. 2020;382:1663–65. doi:10.1056/NEJMc2005073.
- Killerby ME, Biggs HM, Haynes A, Dahl RM, Mustaquim D, Gerber SI, Watson JT. Human coronavirus circulation in the United States 2014–2017. J Clin Virol. 2018;101:52–56. doi:10.1016/j.jcv.2018.01.019.
- Schwartz DA. An analysis of 38 pregnant women with COVID-19, their newborn infants, and maternal-fetal transmission of SARS-CoV-2: maternal coronavirus infections and pregnancy outcomes. Arch Pathol Lab Med. 2020 March 17 [accessed 2020 May 14]; 25. doi:10.5858/arpa.2020-0901-SA.
- Mair-Jenkins J, Saavedra-Campos M, Baillie JK, Cleary P, Khaw F-M, Lim WS, Makki S, Rooney KD, Nguyen-Van-Tam JS, Beck CR. The effectiveness of convalescent plasma and hyperimmune immunoglobulin for the treatment of severe acute respiratory infections of viral etiology: a systematic review and exploratory meta-analysis. J Infect Dis. 2015;211:80–90. doi:10.1093/infdis/jiu396.
- Shen C, Wang Z, Zhao F, Yang Y, Li J, Yuan J, Wang F, Li D, Yang M, Xing L, et al. Treatment of 5 Critically Ill Patients With COVID-19 With Convalescent Plasma. JAMA. 2020;323:1582–89. doi:10.1001/jama.2020.4783.
- Liu STH, Lin H-M, Baine I, Wajnberg A, Gumprecht JP, Rahman F, Rodriguez D, Tandon P, Bassily-Marcus A, Bander J, et al. Convalescent plasma treatment of severe COVID-19: A matched control study. medRxiv. 2020 May 22 [accessed 2020 May 23]; 22. doi:10.1101/2020.05.20.20102236.
- Bonam SR, Kaveri SV, Sakuntabhai A, Gilardin L, Bayry J. Adjunct Immunotherapies for the Management of Severely Ill COVID-19 Patients. Cell Rep. 2020:1. doi:10.1016/j.xcrm.2020.100016.
- Monto AS, DeJonge PM, Callear AP, Bazzi LA, Capriola SB, Malosh RE, Martin ET, Petrie JG. Coronavirus occurrence and transmission over 8 years in the HIVE cohort of households in Michigan. J Infect Dis. 2020 Apr 4 [accessed 2020 Apr 30]; 8. doi:10.1093/infdis/jiaa161.
- Talbot HK, Shepherd BE, Crowe JE, Griffin MR, Edwards KM, Podsiad AB, Tollefson SJ, Wright PF, Williams JV. The pediatric burden of human Coronaviruses evaluated for twenty years. Pediatr Infect Dis J. 2009;28:682–87. doi:10.1097/INF.0b013e31819d0d27.
- Paules CI, Marston HD, Fauci AS. Coronavirus infections—more than just the common cold. JAMA. 2020;323:707–08. doi:10.1001/jama.2020.0757.
- Meyer B, Drosten C, Müller MA. Serological assays for emerging coronaviruses: challenges and pitfalls. Virus Res. 2014;194:175–83. doi:10.1016/j.virusres.2014.03.018.
- Nowak MD, Sordillo EM, Gitman MR, Mondolfi AEP. Co-infection in SARS-CoV-2 infected patients: where Are Influenza Virus and Rhinovirus/Enterovirus? J Med Virol. 2020 Apr 30 [accessed 2020 May 24]; 7. doi:10.1002/jmv.25953.
- Wan WY, Lim SH, Seng EH. Cross-reaction of sera from COVID-19 patients with SARS-CoV assays. medRxiv. 2020 Mar 23 [accessed 2020 May 12]; 12. doi:10.1101/2020.03.17.20034454.
- Ou X, Liu Y, Lei X, Li P, Mi D, Ren L, Guo L, Guo R, Chen T, Hu J, et al. Characterization of spike glycoprotein of SARS-CoV-2 on virus entry and its immune cross-reactivity with SARS-CoV. Nat Commun. 2020;11:1620. doi:10.1038/s41467-020-15562-9.
- Pinto D, Park Y-J, Beltramello M, Walls AC, Tortorici MA, Bianchi S, Jaconi S, Culap K, Zatta F, De Marco A, et al. Cross-neutralization of SARS-CoV-2 by a human monoclonal SARS-CoV antibody. Nature. 2020 May 18;[accessed 2020 May 20]. 27. doi:10.1038/s41586-020-2349-y.
- Tamburello A, Marando M. Immunoglobulins or convalescent plasma to tackle COVID-19: buying time to save lives – current situation and perspectives. Swiss Med Wkly. 2020;150:1718. doi:10.4414/smw.2020.20264.
- de Assis RR, Jain A, Nakajima R, Jasinskas A, Felgner J, Obiero JM, Adenaiye O, Tai S, Hong F, Norris P, et al. Analysis of SARS-CoV-2 Antibodies in COVID-19 convalescent blood using a Coronavirus antigen microarray. medRxiv. 2020 Apr 17 [accessed 2020 May 14]; 21. doi:10.1101/2020.04.15.043364.
- Rota PA, Oberste MS, Monroe SS, Nix WA, Campagnoli R, Icenogle JP, Peñaranda S, Bankamp B, Maher K, Chen M-H, et al. Characterization of a novel coronavirus associated with severe acute respiratory syndrome. Science. 2003;300:1394–99. doi:10.1126/science.1085952.
- Zhao J, Wang W, Wang W, Zhao Z, Zhang Y, Lv P, Ren F, Gao X-M. Comparison of immunoglobulin G responses to the Spike and Nucleocapsid Proteins of Severe Acute Respiratory Syndrome (SARS) Coronavirus in patients with SARS. Clin Vaccine Immunol. 2007;14:839–46. doi:10.1128/CVI.00432-06.
- Che X-Y, Qiu L-W, Liao Z-Y, Wang Y-D, Wen K, Pan Y-X, Hao W, Mei Y-B, Cheng VCC, Yuen K-Y. Antigenic cross-reactivity between severe acute respiratory syndrome-associated coronavirus and human coronaviruses 229E and OC43. J Infect Dis. 2005;19:2033–37. doi:10.1086/430355.
- Centers for Disease Control and Prevention. COVIDView. Washington (DC); 2020 May 19 [accessed 2020 May 21]. https://www.cdc.gov/coronavirus/2019-ncov/covid-data/covidview/index.html
- Gorse GJ, Donovan MM, Patel GB. Antibodies to coronaviruses are higher in older compared with younger adults and binding antibodies are more sensitive than neutralizing antibodies in identifying coronavirus-associated illnesses. J Med Virol. 2020;92:512–17. doi:10.1002/jmv.25715.
- Fouda GG, Martinez DR, Swamy GK, Permar SR. The impact of IgG transplacental transfer on early life immunity. ImmunoHorizons. 2018;2:14–25. doi:10.4049/immunohorizons.1700057.