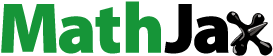
ABSTRACT
This first-in-human study (NCT03032588), conducted in Belgium, evaluated a new inactivated poliovirus vaccines (IPV) candidate based on Sabin poliovirus strains grown on the high-yield PER.C6® cell line. Healthy adults (N = 32) were randomized (1:1) to receive a single dose of PER.C6-based Sabin-IPV (sIPV, 15:35:112.5 DU/dose) or conventional Salk-IPV (cIPV, 40:8:32 DU/dose). Reactogenicity was assessed up to 7 days after vaccination, immunogenicity 28 days after vaccination, and safety up to 6 months after vaccination.
Solicited adverse events (AEs) were mild to moderate, no changes of concern in vital signs or safety laboratory values were observed, and no severe AEs (SAEs) or vaccine-related unsolicited AEs were reported after vaccination. A trend to more frequent solicited AEs after sIPV than after cIPV administration was observed. Most participants had preexisting neutralizing antibodies against poliovirus types (titer ≥8), which were strongly boosted by sIPV. Post-vaccination geometric mean titers were high (≥12,000) and similar across the two vaccination groups. Only participants with very high preexisting antibody levels did not show a vaccine-induced response, defined in seropositive participants as a 4-fold titer increase. The 10 initially seronegative (titer <8) participants (n = 5 in each study group) seroconverted and all participants had seroprotective antibody levels post-vaccination. The antibodies elicited by sIPV neutralized both Sabin and Salk poliovirus strains.
In conclusion, the PER.C6®-based sIPV was well tolerated and highly immunogenic in adults with preexisting antibodies to poliovirus.
Introduction
Broad use of inactivated polio vaccines (IPV) and oral polio vaccines (OPV), from the mid-1950s, first in industrialized countries, then in worldwide polio immunization programs, rapidly decreased the global incidence of poliomyelitis.Citation1 In 2017, 30 years after the World Health Organization’s (WHO) resolution to eradicate polio,Citation2 endemic wild poliovirus was reduced to only three countries and the number of yearly reported cases of poliomyelitis had fallen from 350,000 to 20.Citation3,Citation4 In 2018, 33 confirmed cases have been reported in two countries, and 165 in 2019.Citation5
Trivalent live-attenuated OPV was the cornerstone of that success, particularly through its ability to induce herd immunity. Yet, as the end of wild poliovirus transmission seems near, the routine use of OPV will also have to come to an end. The same trait that made OPV an efficient protection tool when the disease was highly prevalent, its live replicating nature, carries inherent risks. Indeed, OPV related vaccine associated paralytic poliomyelitis, that is linked to the reversion to virulence of the attenuated strains in OPV and the potential for circulation of vaccine derived poliovirus are antagonistic to the final stages of poliovirus eradication.Citation6 Consequently, in 2008, the World Health Assembly endorsed the cessation of OPV routine vaccination after polio eradication.Citation7 Following last detection in 1999, the eradication of wild poliovirus type 2 was certified in 2015 and the type 2 component was withdrawn from all OPV immunization programs in April 2016.Citation8,Citation9 As a result, at least one dose of trivalent IPV is now advocated to ensure immunity against type 2.Citation10,Citation11 Ultimately, once polio is eradicated, the aim is to transition to two or three doses of IPV.
As progress is being made toward polio eradication, attention has turned to the requirements of a polio-free world and available options to reach adequate IPV supply.Citation12,Citation13 This is currently constrained and based on traditional Vero-based manufacturing. In addition, as the incidence of poliomyelitis falls, vaccine production facilities themselves become focal points for a potential poliovirus transmission risk. Consequently, use of poliovirus strains with lower biosafety risk has been called for, along with the search for affordability solutions.Citation7 Initiatives have focused on SabinCitation14 or genetically modifiedCitation15 poliovirus strains to decrease biosafety risks during production. In parallel, increasing capacity has been targeted through the use of fractional intradermalCitation16 or adjuvantedCitation17 IPV doses. To date several Sabin-IPV (sIPV) have been assessed within clinical trials in adultsCitation18,Citation19 and infants,Citation20–25 and some are licensed in JapanCitation26 and China.Citation23
The IPV evaluated here is based on the safer-to-manufacture Sabin poliovirus strains and the PER.C6® platform. This platform supports a high productivity of polioviruses,Citation27–29 which showed immunogenicity in preclinical models.Citation29 We describe here the first clinical assessment of the PER.C6® sIPV in adult volunteers.
Methods
Design and objectives
This Phase 1, randomized, controlled, double-blind study was conducted at a single study center in Belgium (Center for Vaccinology, Ghent University Hospital) between October 23 2017 and May 18 2018 (Clinicaltrials.gov identifier: NCT03032588). The clinical procedures were approved by the Ethical Committee of the study center and the study was carried out according to good clinical practice principles, in accordance with the declaration of Helsinki.Citation30,Citation31 All participants provided written informed consent before undergoing the screening procedures.
The primary objective of the study was to assess the safety and tolerability of PER.C6®-based sIPV in terms of solicited local and systemic adverse events (AEs) in the 7 days after vaccination, unsolicited events in the 28 days after vaccination, and serious AEs (SAEs) in the 6 months following vaccination. Information on safety laboratory parameters was also collected at screening, immediately before vaccination, and 7 days after vaccination.
The immunogenicity of the vaccine was assessed as a secondary objective by measuring neutralizing antibody titers against the three poliovirus types, immediately before and 28 days after vaccination.
Study participants
The study was designed to vaccinate 32 healthy adults aged between 18 and 45 years. Participants were randomized 1:1 to receive a single dose of the PER.C6®-based sIPV or the conventional cIPV (Salk-IPV, Sanofi Pasteur’s Imovax™).
Before receiving study vaccine, participants were screened for eligibility based on physical examination, medical history, vital signs, and clinical laboratory tests. The main exclusion criteria from study participation were: polio vaccination in the past 6 months; allergy; immunological deficiency, immune-suppressive treatment, or autoimmune disease; any other vaccination received within the 30-day period before vaccination, or planned vaccination up to 4 weeks post-vaccination; acute disease and/or fever at the time of vaccination; investigational drug received within 3 months or an experimental vaccine received within 6 months before administration of study vaccine; participation in another investigational study during the course of this study; pregnancy or breastfeeding. The effect of this vaccine on a fetus as well as the effect on sperm is unknown. From the day of first vaccination until 3 months after vaccination, male participants had to use a condom when engaging in sexual intercourse and women of childbearing potential had to agree to practice a highly effective method of contraception.
Vaccination
The PER.C6®-based sIPV candidate was produced by Janssen with a target antigen content of 15 D-antigen units (DU) for poliovirus type 1, 35 DU for type 2, and 112.5 DU for type 3.
The conventional cIPV contained 40 DU of poliovirus type 1 (Mahoney), 8 DU of type 2 (MEF-1), and 32 DU of type 3 (Saukett).
The vaccine was administered into the deltoid muscle. Study vaccines were prepared and administered by designated unblinded study personnel who were not involved in the post-vaccination assessment of participants.
Safety analyses
Safety laboratory assessments were performed on blood samples collected at screening, before administration of the vaccine, and 7 days thereafter for the detection of emerging laboratory abnormalities. Laboratory abnormalities were determined according to the FDA/CBER toxicity grading tablesCitation32 and the normal ranges of the testing laboratory.
Participants were observed for 30 minutes after vaccination at the study site. Vital signs were measured immediately before and 30 minutes after vaccination. Participants received diary cards for daily recording of their body temperature and occurrences of solicited local and systemic AEs in the 7 days after vaccination. Information on other (unsolicited) AEs and on concomitant medication related to AEs/SAEs was collected at every study visit and additional contact until Week 4 after vaccination. Information on potential SAEs was collected until 6 months after vaccination.
Electronic data capture for the safety analyses was performed using Medidata Rave®.
Immunogenicity analyses
A blood sample was collected immediately before and 28 days after vaccination for measuring neutralizing antibody titers against the wild-type Salk poliovirus strains (type 1 [Mahoney], type 2 [MEF-1], and type 3 [Saukett]), as well as the Sabin poliovirus strains (types 1, 2, and 3) in accordance with the WHO recommendations for immunogenicity assessment of IPV.Citation33 The assays were performed at Centers for Disease Control and Prevention in the United States. Polio neutralizing antibodies were determined by 2-fold serial dilution of serum, which was added to HEp-2(C) cells for 5 days, together with Salk Polio (Mahoney, MEF-1, or Saukett) or Sabin (type 1, 2, or 3) virus at 100 CCID50/25 l, at 35°C. Cells were stained by crystal violet (0.05%) and titer was determined by the mean of the triplicate, based on the number of wells positive for neutralization.Citation34 The assay range was extended to 18.5 log2 to allow the measurement of unusually high titers induced by boosting pre-immune adults.
Statistical analyses
The primary population for the safety and immunogenicity analyses consisted, respectively, of all vaccinated participants with safety data available and of all vaccinated participants with post-vaccination immunogenicity data available.
Statistical analyses for phase 1 reporting were performed using SAS® version 9.4 (SAS Institute Inc., Cary, NC, USA).
Regarding demographic characteristics, mean and standard deviation were provided for age, height, weight, and body mass index.
Baseline for safety laboratory tests and vital signs was defined as the last evaluation before vaccination. Safety data were analyzed descriptively. The verbatim terms used to identify unsolicited AEs were coded using the Medical Dictionary for Regulatory Activities (MedDRA version 21). All solicited and unsolicited AEs with onset within 7 or 28 days, respectively, after vaccination, including abnormalities in safety laboratory values and vital signs in the 7 days after vaccination, were included in the analysis. For each AE, the percentage of participants who experienced at least one occurrence of the event was calculated per vaccine group.
The proportion of participants with antibody titers against poliovirus ≥8, seroprotective threshold in the Salk neutralizing assay, and geometric mean titers (GMT) with 95% confidence intervals (Cis) were calculated per vaccination group for each poliovirus type in the two assays. Means with 95% CI of the log2 transformed values were back-transformed to estimate GMT with 95% CI. As per WHO’s definition,Citation33 seroconversion was defined for initially seronegative (titer <8) individuals as a post-vaccination antibody titer ≥8 and for individuals who were initially seropositive (titer ≥8) as a ≥ 4-fold increase in antibody titer, testifying of a vaccine-induced immune response. Reverse cumulative distribution curves of antibody titers were plotted per vaccine group for each poliovirus type.
Results
A total of 32 participants were vaccinated and included in the analysis of safety and immunogenicity data. The disposition of the study participants is presented in .
Demographics
Demographic characteristics were similar across the two groups (). More women than men were enrolled in each study group.
Table 1. Demographic characteristics of vaccinated participants (mean ± SD)
Polio vaccination of children before the age of 18 months was made mandatory in Belgium in 1967. The complete polio vaccination coverage rate in Belgium in 2016 was 93%; therefore, it is assumed that a similar proportion of enrolled participants had received primary vaccination against poliovirus during childhood.Citation35 Previous polio vaccination history was not recorded at study entry, but information was sought at the end of the trial from four participants with high pre-vaccination titers who had not shown a 4-fold increase in titer: two had received an IPV booster as travel vaccines 4 and 5 years before the trial respectively, the other two participants had no vaccination records nor memory of receiving a polio vaccine booster in preceding years.
Safety results
Most study participants – 15 (93.8%) in the sIPV group and 10 (62.5%) in the cIPV group – experienced AEs at the injection site: these were almost exclusively occurrences of pain/tenderness (). A single occurrence of swelling/induration was reported in the sIPV group; erythema was not reported. Local solicited AEs were all graded 1 (mild) in intensity, except one occurrence of pain/tenderness in the cIPV group, which was of Grade 2 intensity (moderate). Most participants in the two groups reported systemic AEs: 13 (81.3%) in the sIPV group and nine (56.3%) in the cIPV group. The most frequent event was fatigue, described by nine (56.3%) participants in the sIPV group and seven participants (43.8%) in the cIPV group, followed by myalgia, reported in eight (50%) of the sIPV participants and four (25%) of the cIPV recipients. Systemic AEs judged as caused by vaccination were reported with a frequency of 62.5% in the sIPV group and 50% in the cIPV group. Most solicited systemic AEs were mild in intensity. There was a single occurrence of Grade 2 for each of the solicited systemic AEs in the sIPV group. Grade 2 fever (38.5–38.9°C) in one participant was present on the day of vaccination only; the event lasted 1 day, was resolved by Day 2 and was considered related to vaccination. No Grade 3 events were reported during the study. The median time to onset of solicited AEs was similar in the two groups: 1 day for local AEs and 2 days for most systemic events. The median duration was 1 day for all solicited AEs in the two groups.
Figure 2. Local and systemic solicited adverse events reported during the 7 days after vaccination. cIPV = conventional Salk-IPV; sIPV = Sabin-IPV
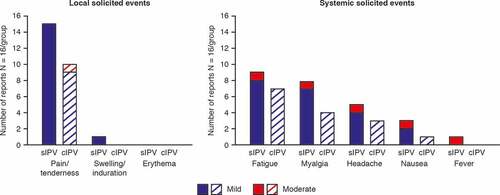
Other (unsolicited) AEs were reported by five study participants (31.3%) in the sIPV group and 10 participants (62.5%) in the cIPV group. One occurrence of hemorrhage at the injection site (in the cIPV group) was caused by the vaccination procedure. Unsolicited AEs were mainly represented by respiratory tract infections and headache (11 out of 15 occurrences), and none was judged as causally related to the administration of the study vaccines.
One safety laboratory abnormality emerging after vaccination was detected in each group: a marginal increase in aspartate aminotransferase concentration of approximately 1.2-fold compared to the upper limit of normal, which met the definition of a Grade 1 increase (defined as a 1.1–2.5-fold increase).
No SAEs were reported during the 6-month post-vaccination period, and overall the vaccine was well tolerated, showing an acceptable safety profile within this population.
Immunogenicity results
Similar immunogenicity results were obtained using the Salk and Sabin neutralization assays for the primary analysis (). From here on, immunogenicity data are provided from the Salk neutralization assay. Most participants (12–16 from a total of 16) had seroprotective titers (≥8) of neutralizing antibodies against at least one poliovirus type before vaccination. sIPV vaccination induced a strong increase in polio neutralizing antibody titers as measured 28 days later (, ). Overall, the magnitude of the increase in titers was similar with each vaccine, as illustrated by reverse cumulative distribution curves (). Due to the limited group size, no formal statistical comparison of titers was performed. All participants mounted titer increases of at least 4-fold, with the exception of two in the sIPV group and four in the cIPV group. After vaccination, all initially seronegative participants (for polio types 1, 2, and 3: n = 4, n = 2, and n = 4, respectively in the sIPV group; n = 4, n = 1, and n = 4, respectively in the cIPV group) had mounted antibody titers ≥8 which defines seroprotection as measured in the Salk assay. Overall, the sIPV candidate was immunogenic toward the three types of Salk and Sabin poliovirus strains.
Table 2. Poliovirus neutralizing antibody seroprotection rates and seroconversion rates for wild-type and Sabin poliovirus strains before and 28-days post-vaccination
Figure 3. Geometric mean titers (with 95% CI) of poliovirus neutralizing antibodies before and after vaccination. Neutralizing titers to Salk (A) or Sabin (B) virus type 1, 2 and 3 are plotted for each group (n = 16 per group). Blue bars show the baseline titers of the subjects before vaccination, yellow bars the titers 28 days after vaccination. The lower limit of detection of the assay is 2Citation2,Citation7 (6.49). Seroprotection in the Salk assay is a titer of 8 for each type 1, 2 and 3. Seroprotection titers for Sabin (type 1, 2 and 3) are not defined. cIPV = conventional Salk-IPV; CI = confidence interval; sIPV = Sabin-IPV
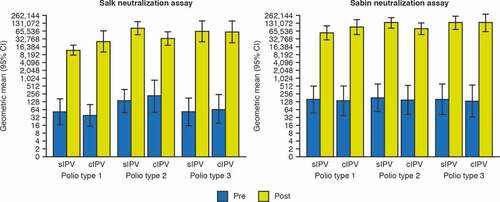
Figure 4. Reverse cumulative distribution curves of poliovirus neutralizing antibody titers against Salk strains before and 28 days after vaccination. Dotted line = before vaccination; Solid line = 28 days after vaccination. The vertical line at 2.7 shows the lower limit of quantitation of the assay. Red line = subjects who received sIPV; Blue line = subjects who received cIPV. cIPV = conventional Salk-IPV; sIPV = Sabin-IPV
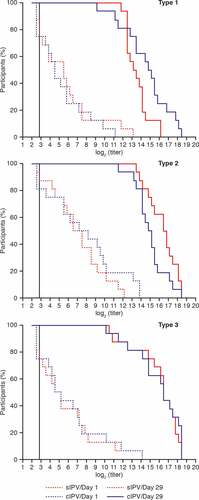
Discussion
The first demonstration of the feasibility for the use of Sabin PV strains for IPV was obtained in 1988 in a clinical study in adults, where the vaccine boosted preexisting neutralizing antibody levels and induced seroprotection in seronegative individuals.Citation36 The current first-in-human study assessed the safety and immunogenicity of a new Sabin-based IPV for which the polio virus strains were grown on the high yield PER.C6® cell line. This vaccine candidate supports the aim of lower risk manufacturing while the use of the PER.C6® cell line platform has the potential for the production of a sIPV with lower cost-of-goods than conventional production platforms.
The primary objective of our study was to assess the safety of a high dose of the sIPV candidate in healthy adult volunteers. The vaccine appeared safe and was well tolerated overall. No changes of concern in laboratory safety parameters or vital signs were noted. The local and systemic AEs classically associated with vaccination were reported as mild or moderate, and the only noteworthy observation was their higher frequency in the sIPV group, which may be explained by the high vaccine dose (15:35:112.5 DU). Of note, an imbalance in the other direction (i.e., more frequently reported in the cIPV group), was observed for unsolicited symptoms, which were not judged as caused by vaccination. This highlights the possible chance occurrence of imbalances with such small sample sizes. Further assessment of this sIPV candidate in pediatric studies will include formulations with lower total antigenic content than the dose used in this Phase 1 study.
The reactogenicity and safety data of this study showed similar trends to previous reports obtained with a sIPV assessed in adults in PolandCitation18 and Cuba,Citation19 although administered at other dosages. As in the current study, AEs were generally mild and moderate in intensity; the main local AE was injection-site pain. Although it is currently not possible to compare antigenic contents of different sIPVs, the establishment of the first WHO international standard is underway and will make this feasible in the future.Citation37
The robust boosting of poliovirus neutralizing antibody levels observed in this study was also reported for adults immunized in infancy and childhood in Poland and Cuba.Citation18,Citation19 Although a direct comparison was not possible, due to the different antigen contents of these vaccines, the post-vaccination GMTs in the adult study in Poland were very similar to those from our study in Belgium.Citation18 In the latter, the response to poliovirus type 2 was strong, confirming previous findings that the lower immunogenicity of Sabin type 2 strains in rodent models is not observed in humans.Citation19 The antibodies elicited by sIPV neutralized both the Salk and the Sabin poliovirus strains. Recent data have indicated that sIPV are able to elicit a broad neutralizing humoral immunity against a diversity of wild and vaccine-derived poliovirus,Citation38 confirming sIPV as a valuable tool in the prevention of poliomyelitis.
Our study illustrates the limitations inherent to Phase 1 trials and the small sample size does not permit the precise determination of the frequency of AEs.
Both the sIPV and cIPV were highly immunogenic in this adult population (likely vaccinated against polio during childhood) in which approximately 75% of individuals had preexisting immunity against poliovirus at baseline. After vaccination, all participants had poliovirus neutralizing antibody titers above seroprotective levels, including those participants with undetectable preexisting immunity. The acceptable safety and immunogenicity profile justifies further evaluation of this new sIPV in the pediatric population. A Phase 2 study in infants is currently ongoing.
Polio eradication efforts will require more IPV to accompany the withdrawal of type 2 OPV. In addition, once OPV is discontinued entirely post-eradication, the global public health system will need significantly more IPV at an affordable price than is currently available to support IPV-only immunization programs. sIPV manufactured on the highly productive PER.C6® cell line has the potential to be a safe, effective, and affordable alternative IPV for the sustained protection of global populations against polio.
Disclosure of potential conflicts of interest
G.S. H.S. and M.S. are full-time employees of the study sponsor. C.C., R.d.R., and H.V.Z. were full-time employees of the sponsor at the time of the trial. J.-M. J. is an independent consultant under contract with the study sponsor. I.L.-R and G.L-R. have received grants from Janssen Vaccines to finance the conduct of this study in their institution. H.S. holds shares in Johnson & Johnson. C.C. holds shares in Johnson & Johnson and GlaxoSmithKline.
Acknowledgments
The authors would like to thank the following: all volunteers who participated in this trial; the investigators and clinical team at CEVAC, in particular sub-investigators Annelies Aerssens and Cathy Maes; Anniek Desimpel, study nurse and project manager; the CDC for performing the assays; and Janssen study personnel.
Additional information
Funding
References
- Ward NA. Poliomyelitis: a review. Trop Doct. 1983;13:21–28. doi:10.1177/004947558301300108.
- WHA 41st World Health Assembly. Global eradication of poliomyelitis by 2000. Geneva World Health Organization [resolution WHA41.28]. 1988 [accessed 2020 Mar 16]. http://polioeradication.org/wp-content/uploads/2016/07/19880513_resolution-2.pdf.
- Khan F, Datta SD, Quddus A, Vertefeuille JF, Burns CC, Jorba J, Wassilak SGF. Progress toward polio eradication — worldwide, January 2016–March 2018. MMWR Morb Mortal Wkly Rep. 2018;67(18):524–28. doi:10.15585/mmwr.mm6718a4.
- Zaffran M, McGovern M, Hossaini R, Martin R, Wenger J. The polio endgame: securing a world free of all polioviruses. Lancet. 2018;391(10115):11–13. doi:10.1016/S0140-6736(17)32442-X.
- World Health organization. Global polio eradication initiative. Polio this week. 2020 [accessed 2020 Mar 16]. http://polioeradication.org/polio-today/polio-now/this-week/.
- Okayasu H, Sutter RW, Jafari HS, Takane M, Aylward RB. Affordable inactivated poliovirus vaccine: strategies and progress. J Infect Dis. 2014;210(Suppl 1):S459–464. doi:10.1093/infdis/jiu128.
- World Health Assembly. Poliomyelitis: mechanism for management of potential risks to eradication. Geneva: World Health Organization [resolution WHA61.1]. 2008 [accessed 2020 Mar 16]. http://polioeradication.org/wp-content/uploads/2016/07/WHA61_Resolution_English.pdf.
- Hampton LM, Farrell M, Ramirez-Gonzalez A, Menning L, Shendale S, Lewis I, Rubin J, Garon J, Harris J, Hyde T, et al. Cessation of trivalent oral poliovirus vaccine and introduction of inactivated poliovirus vaccine — worldwide, 2016. MMWR Morb Mortal Wkly Rep. 2016;65(35):934–38. doi:10.15585/mmwr.mm6535a3.
- Global Polio Eradication Initiative. Polio eradication and endgame strategic plan 2013–2018. 2013 [accessed 2020 Mar 16]. http://polioeradication.org/wp-content/uploads/2016/07/PEESP_EN_A4.pdf.
- World Health Organization. Meeting of the strategic advisory group of experts on immunization, November 2012 – conclusions and recommendations. Polio eradication. Weekly Epidemiological Rec 2013;88:5–7.
- World Health Organization. Polio vaccines: WHO position paper - March 2016. Weekly Epidemiological Rec 2016;12:145–68.
- Global Polio Eradication Initiative. Polio post-certification strategy: a risk mitigation strategy for a polio-free world. Geneva: World Health Organization. License: CC BY-NC-SA 3.0 IGO. 2018 [accessed 2020 Mar 16]. http://polioeradication.org/wp-content/uploads/2018/04/polio-post-certification-strategy-20180424-2.pdf.
- World Health Organization. Meeting of the strategic advisory group of experts on immunization, April 2017 – conclusions and recommendations. Polio Eradication. Weekly Epidemiological Rec 2017;92:308–10.
- Okayasu H, Sein C, Hamidi A, Bakker WAM, Sutter RW. Development of inactivated poliovirus vaccine from Sabin strains: a progress report. Biologicals. 2016;44(6):581–87. doi:10.1016/j.biologicals.2016.08.005.
- Knowlson S, Burlison J, Giles E, Fox H, Macadam AJ, Minor PD. New strains intended for the production of inactivated polio vaccine at low-containment after eradication. PLoS Pathog. 2015;11(12):e1005316. doi:10.1371/journal.ppat.1005316.
- Okayasu H, Sein C, Chang Blanc D, Gonzalez AR, Zehrung D, Jarrahian C, Macklin G, Sutter RW. Intradermal administration of fractional doses of inactivated poliovirus vaccine: a dose-sparing option for polio immunization. J Infect Dis. 2017;216(Suppl 1):S161–167. doi:10.1093/infdis/jix038.
- Rivera L, Pedersen RS, Peña L, Olsen KJ, Andreasen LV, Kromann I, Nielsen PI, Sørensen C, Dietrich J, Bandyopadhyay AS, et al. Immunogenicity and safety of three aluminium hydroxide adjuvanted vaccines with reduced doses of inactivated polio vaccine (IPV-Al) compared with standard IPV in young infants in the Dominican Republic: a phase 2, non-inferiority, observer-blinded, randomised, and controlled dose investigation trial. Lancet Infect Dis. 2017;17(7):745–53. doi:10.1016/S1473-3099(17)30177-9.
- Verdijk P, Rots NY, MGCT VO, Oberste MS, Boog CJ, Okayasu H, Sutter RW, Bakker WAM. Safety and immunogenicity of inactivated poliovirus vaccine based on Sabin strains with and without aluminum hydroxide: a phase I trial in healthy adults. Vaccine. 2013;31(47):5531–36. doi:10.1016/j.vaccine.2013.09.021.
- Resik S, Tejeda A, Fonseca M, Alemañi N, Diaza M, Martinez Y, Garcia G, Okayasu H, Burton A, Bakker WAM, et al. Reactogenicity and immunogenicity of inactivated poliovirus vaccine produced from Sabin strains: a phase I trial in healthy adults in Cuba. Vaccine. 2014;32(42):5399–404. doi:10.1016/j.vaccine.2014.07.109.
- Liao G, Li R, Li C, Sun M, Li Y, Chu J, Jiang S, Li Q. Safety and immunogenicity of inactivated poliovirus vaccine made from Sabin strains: a phase II, randomized, positive-controlled trial. J Infect Dis. 2012;205(2):237–43. doi:10.1093/infdis/jir723.
- Okada K, Miyazaki C, Kino Y, Ozaki T, Hirose M, Ueda K. Phase II and III clinical studies of diphtheria-tetanus-acellular pertussis vaccine containing inactivated polio vaccine derived from Sabin strains (DTaP-sIPV). J Infect Dis. 2013;208(2):275–83. doi:10.1093/infdis/jit155.
- Verdijk P, Rots NY, van Oijen MGCT, Weldon WC, Oberste MS, Okayasu H, Sutter RW, Bakker WAM. Safety and immunogenicity of a primary series of Sabin-IPV with or without aluminium hydroxide in infants. Vaccine. 2014;32(39):4938–44. doi:10.1016/j.vaccine.2014.07.029.
- Liao G, Li R, Li C, Sun M, Jiang S, Li Y, Mo Z, Xia J, Xie Z, Che Y, et al. Phase 3 trial of a Sabin strain–based inactivated poliovirus vaccine. J Infect Dis. 2016;214(11):1728–34. doi:10.1093/infdis/jiw433.
- Nakano T, Sumino S, Takanami Y, Mitsuya N, Nakatome K. A phase 2 study of a combined diphtheria-tetanus-acellular pertussis vaccine with a Sabin-derived inactivated poliovirus vaccine in children. Hum Vaccin Immunother. 2018;14(12):2940–49. doi:10.1080/21645515.2018.1504538.
- Chu K, Ying Z, Wang L, Hu Y, Xia J, Chen L, Wang J, Li C, Zhang Q, Gao Q, et al. Safety and immunogenicity of inactivated poliovirus vaccine made from Sabin strains: a phase II, randomized, dose-finding trial. Vaccine. 2018;36(45):6782–89. doi:10.1016/j.vaccine.2018.09.023.
- Shimizu H. Development and introduction of inactivated poliovirus vaccines derived from Sabin strains in Japan. Vaccine. 2016;34(16):1975–85. doi:10.1016/j.vaccine.2014.11.015.
- Sanders BP, Edo-Matas D, Custers JHHV, Koldijk MH, Klaren V, Turk M, Luitjens A, Bakker WAM, Uytdehaag F, Goudsmit J, et al. PER.C6(®) cells as a serum-free suspension cell platform for the production of high titer poliovirus: a potential low cost of goods option for world supply of inactivated poliovirus vaccine. Vaccine. 2013;31(5):850–56. doi:10.1016/j.vaccine.2012.10.070.
- Sanders BP, de Los Rios Oakes I, van Hoek V, Liu Y, Marissen W, Minor PD, Wimmer E, Schuitemaker H, Custers JHHV, Macadam A, et al. Production of high titer attenuated poliovirus strains on the serum-free PER.C6(®) cell culture platform for the generation of safe and affordable next generation IPV. Vaccine. 2015;33(48):6611–16. doi:10.1016/j.vaccine.2015.10.091.
- Bockstal V, Tiemessen MM, Achterberg R, Van Wordragen C, Knaapen AM, Serroyen J, Marissen WE, Schuitemaker H, Zahn R. An inactivated poliovirus vaccine using Sabin strains produced on the serum-free PER.C6® cell culture platform is immunogenic and safe in a non-human primate model. Vaccine. 2018;36(46):6979–87. doi:10.1016/j.vaccine.2018.09.068.
- World Medical Association. World medical association declaration of Helsinki: ethical principles for medical research involving human subjects. JAMA. 2013 Accessed on 16 Jul 2020);310(20):2191–94. doi:10.1001/jama.2013.281053.
- EMA. ICH good clinical practice guidelines. 2016 [accessed 2020 Jul 16]. https://www.ema.europa.eu/en/documents/scientific-guideline/ich-e-6-r2-guideline-good-clinical-practice-step-5_en.pdf.
- Food and Drug Administration. Center for biologics evaluation and research. guidance for industry. Toxicity grading scale for healthy adult and adolescent volunteers enrolled in preventive vaccine clinical trials. 2007 Sept.
- World Health Organization. Expert committee on biological standardization (ECBS), sixty-fifth report. Recommendations to assure the quality, safety and efficacy of poliomyelitis vaccines (inactivated). WHO Technical Report Series No. 993, Annex 3, 2015.
- Weldon WC, Oberste MS, Pallansch MA. Standardized methods for detection of poliovirus antibodies. Methods Mol Biol. 2016;1387:145–76. doi:10.1007/978-1-4939-3292-4_8.
- KCE Report 313. Performance of the Belgian health system – report 2019. [accessed 2020 Jul 16] https://www.healthybelgium.be/metadata/hspa/p1_p2_p3.pdf.
- Murph JR, Grose C, McAndrew P, Mickiewicz C, Mento S, Cano F, Radick L, Ritchey M, Stout MG. Sabin inactivated trivalent poliovirus vaccine: first clinical trial and seroimmunity survey. Pediatric Infect Dis J. 1988;7(11):760–65. doi:10.1097/00006454-198811000-00003.
- World Health Organization. Expert committee on biological standardization Geneva, 29 October to 2 November 2018. Report on the WHO collaborative study to establish the 1st International Standard for Sabin inactivated polio vaccine (sIPV). 2018 [accessed 2020 Mar 16]. http://www.who.int/biologicals/BS.2018.2338_sIPV_IS_Collaborative_Study_Report_version.pdf.
- Sun M, Li C, Xu W, Liao G, Li R, Zhou J, Li Y, Cai W, Yan D, Che Y, et al. Immune serum from Sabin inactivated poliovirus vaccine immunization neutralizes multiple individual wild and vaccine-derived polioviruses. Clin Infect Dis. 2017;64(10):1317–25. doi:10.1093/cid/cix110.