ABSTRACT
Adjuvants are central to the efficacy of subunit vaccines. Although several new adjuvants have been approved in human vaccines over the last decade, the panel of adjuvants in licensed human vaccines remains small. There is still a need for novel adjuvants that can be safely used in humans, easy to source and to formulate with a wide range of antigens and would be broadly applicable to a wide range of vaccines. In this article, using the Respiratory Syncytial Virus (RSV) nanoparticulate prefusion F model antigen developed by Sanofi, we demonstrate in the macaque model that the polyacrylate (PAA)-based adjuvant SPA09 is well tolerated and increases vaccine antigen-specific humoral immunity (sustained neutralizing antibodies, memory B cells and mucosal immunity) and elicits strong TH1-type responses (based on IFNγ and IL-2 ELISpots) in a dose-dependent manner. These data warrant further development of the SPA09 adjuvant for evaluation in clinical trials.
Introduction
New precision vaccine approaches based on specific protein or peptide antigens have gained interest over the last decades due to advantages in terms of safety, purity, quality control, and batch-to-batch consistency but they often require adjuvants to induce robust immune responses.Citation1,Citation2 There are not many adjuvants available which have been approved for human use and these comprise aluminum salts, oil-in-water emulsions, a CpG oligonucleotide (ISS1018) and GSK’s adjuvant systems AS04 and AS01.Citation3,Citation4 Moreover, most of these adjuvants require specific manufacturing or formulation skills for production. Hence, there remains a need for novel adjuvants that could be safely used in humans, would be easy to source and to formulate with various antigens and would be broadly applicable to a wide range of vaccines.
Polyelectrolytes are widely known for their immunostimulatory properties.Citation5–7 We recently introduced a novel polyelectrolyte adjuvant, termed SPA09, based on high molecular weight linear polyacrylate (PAA) readily available and easy to formulate with vaccine antigens.Citation8
In previous studies in mice, SPA09 was found to work as a strong TH1-type adjuvant when tested with various types of antigens including the recombinant glycoprotein CMV-gB and a Staphylococcus aureus polysaccharide conjugate (patent WO2017218819A1). It was found to be more potent than MF59 (squalene oil-in-water emulsion – Novartis) when tested with CMV-gB. SPA09 was also capable of activating human antigen-presenting cells in vitro when tested via the innate immune module of the human MIMIC® system (patent WO2017218819A1).Citation8
Although mouse models are useful to the evaluation of adjuvant effect and mechanism, they are not fully predictive of innate and adaptive immunity after vaccination in humans. Non-human primates (NHPs) are considered to be more translatable models of human vaccine responses due to the high degree of similarity in the distribution and function of immune cell subsets. There is also similarity in the distribution and expression of pathogen recognition receptors (PRRs such as toll-like receptors; TLR) in addition to modeling doses and injection sites more precisely than mouse models do.Citation9
In the study reported herein, we evaluated the immunogenicity of SPA09 at two different doses of PAA (500 µg and 2000 µg) mixed with the model antigen RSV Pre-F-NP in cynomolgus macaques (Macaca fascicularis). Pre-F-NP is a recombinant protein antigen particle produced in CHO cells and composed of the RSV-F glycoprotein blocked in the prefusion (pre-F) conformation and fused to a self-assembling Helicobacter pylori ferritin moiety.Citation10 In this study, SPA09 was compared to the squalene-based oil-in-water emulsion adjuvant, AF03,Citation11 and a non-adjuvanted group was used as control. No specific adverse effects related to the vaccines were reported based on local and systemic observations. We found that SPA09 increased humoral and cellular immune responses in a dose-dependent manner. Compared to AF03, the 2000 µg dosage of SPA09 elicited similar humoral responses but significantly higher cellular responses (IFNγ/IL-2 ELISpots).
This study confirmed the ability of SPA09 to promote strong antibody and T cell responses in a clinically relevant animal model and warrants further evaluation of this novel adjuvant in human clinical trials.
Materials and methods
Animals and ethics statement
Cynomolgus macaques (Macaca fascicularis – Noveprim) were housed at Cynbiose, SA (Marcy l’Etoile – France). This study was reviewed by the Animal Welfare Body of Cynbiose and the Ethics Committee of VetAgro-Sup (1 avenue Bourgelat, 69280 Marcy l’Étoile, France) and approved under number 1633-V3 (MESR number: 2016071517212815). All experiments were conducted in accordance with the European Directive 2010/63/UE as published in the French Official Journal of February 7th, 2013.
Antigen, adjuvants and immunization
Sixteen macaques (24 to 30 months old) were randomized into four immunization groups of four animals each. Fifty micrograms of adjuvanted or non-adjuvanted Pre-F-NP were administered intramuscularly (500 µL) into the deltoid muscle at day 0 and day 28. Two adjuvants were tested (): SPA09 at doses of 500 µg and 2000 µg of PAA and a squalene-based oil-in-water emulsion AF03 (2.5% squalene).Citation11
Table 1. Adjuvant groups
Antigen and adjuvant stock solutions were sterilized by sterile filtration on a 0.22 µm pore membrane and characterized for low endotoxin content (<5 IU/mL) by using Endosafe® cartridges and an Endosafe®-PTSTM spectrophotometer (Charles River Laboratories International, Inc., Wilmington, MA).
Adjuvanted formulations were prepared by mixing extemporaneously, under a laminar flow hood, one volume of a two-fold concentrated adjuvant solution with one volume of a two-fold concentrated antigen solution as to yield experimental vaccines containing 100 µg/mL of Pre-F-NP and 2 or 4 mg/mL of SPA09, or 2.5% squalene for AF03 (benchmark). Before immunization, pH was measured using a Mettler Toledo pH meter and osmolality was measured using a Fiske® Model 210 Micro-Osmometer Fiske Associates apparatus.
Macaque samples
Sera were collected at baseline and at different time points over 6 months post-dose 1 (D0 to D164) to assess humoral responses, including RSV-A2 neutralizing antibodies by a plaque reduction neutralizing test (PRNT60) and F-specific IgG by ELISA.
Peripheral blood mononuclear cells (PBMCs) were collected at baseline, D7, D35, D119, and D161 to assess cellular-mediated immunity and memory B cell responses.
Bronchoalveolar lavages (BAL) were performed on anesthetized animals and trachea was infused with a solution of 5 mL/kg isotonic sodium chloride NaCl 0.9%. BAL fluids were collected in 15 mL-tubes and were placed at +4°C. Tubes were vortexed in order to release antibodies and then centrifuged at 400 x g for 7 min at +4°C to pellet cells and mucus. Supernatants were aliquoted and protease inhibitor (Halt™ Protease Inhibitor Cocktail Thermo Fischer ref 78438 – initial concentration: 100X) was added in order to get 1X final concentration. Supernatants were stored at −80°C.
Clinical monitoring
Immediate reactions were assessed within 30 minutes and potential local reactions at the injection site were observed over the 7 days following each immunization. The scoring of the irritation was evaluated by the method of Draize (0: no evidence of irritation; 1: minimal erythema, barely perceptible; 2: definite erythema, readily visible, minimal edema, or minimal papular response; 3: erythema and papules and 4: definite edema). The macaques were monitored every day and symptoms such as decreased food intake, restricted mobility, polypnea, local and systemic reactions were recorded throughout the study. Body weight and body temperature (using transponder chips) were recorded at baseline and at regular intervals throughout the study.
For hematology, blood samples were transported to Charles River (Les Oncins 69210 Saint Germain-Nuelles- France) at room temperature (RT) within 3 hours (± 30 min). For blood biochemistry, blood samplings were first centrifugated at 1800 x g for 10 min at +4°C. Then, centrifugated tubes were prepared for the transport to Charles River at +4°C within 3 hours (± 30 min) after blood sampling.
Systemic RSV F-specific IgG ELISAs
RSV-F specific IgG were tested by enzyme-linked immunosorbent assay (ELISA). Briefly, microtiter plates (Greiner Bio-one, cat.655061) were coated with 1 µg/mL of F protein (SinoBiologicals, cat. 11049-V08B) in bicarbonate buffer (Sigma, cat. C3041). Plates were incubated overnight at +4°C and then blocked with PBS-Tween 0.05%-milk 5% for 1 h.
Sera were twofold serially diluted in PBS-Tween 0.05%-milk 5% in the coated plate. After 1.5-h incubation at 37°C, plates were washed (PBS-Tween 0.05%) and incubated for 1.5 hours at 37°C with a goat anti-monkey IgG-HRP diluted at 1:10 000 (Biorad, cat. AAI42P). After washing, plates were incubated with 3,3,5,5-tetramethylbenzidine (TMB) substrate (Tebu-bio, cat. TMB100-1000) for 30 minutes in the dark at RT. Colorimetric reaction was stopped with 1 N HCl (VWR Prolabo, cat 30024290). Optical densities (OD) were measured at 450–650 nm on a Versamax plate reader (Molecular Devices). RSV-F–specific IgG titers were quantified through an internal human RSV+ serum reference. The titer of this reference was previously calculated as the reciprocal dilution to obtain an OD of 1.
D25- and palivizumab-competitive assays
D25 and palivizumab competition were evaluated by enzyme-linked immunosorbent assay (ELISA). The prefusion DS-Cav1 recombinant protein (Thermo Scientific, 1 µg/mL) was coated on microtiter plates (Greiner Bio-one) overnight at 4°C and then blocked with PBS-0.05% Tween – 5% nonfat dry milk for 1 h at 37°C. Sera were serially diluted twofold (initial dilution 1:5) and incubated for 1.5 hours at 37°C. After PBS-0.05% Tween washes, competitive biotinylated D25 or palivizumab (Synagis) monoclonal antibody were added and incubated 30 minutes at 37°C. Plates were washed and an anti-biotin HRP conjugate (Invitrogen, cat. PA 1–30595) was added 1.5 hours at 37°C. Final washes were performed, then plates were developed for 30 min using TMB (Tebu-bio, cat. TMB100-1000) and stopped with 1 N HCl (VWR Prolabo, cat. 30024290). Optical densities (OD) were read at 450 nm–650 nm. After blank subtraction, the percentage of antibody binding inhibition was calculated as follows (1-(immune serum OD/baseline serum OD))*100. The immune serum dilution needed to achieve 50% of inhibition was obtained by a 4-parameter logistic regression.
Neutralizing antibody titer analysis
RSV-A2 60% plaque reduction titers (PRNT60) were determined. Briefly, serum samples were heat inactivated, initially diluted 1:10, and fourfold serially diluted in virus growth medium (VGM) containing DMEM (Gibco, cat.31980–022), 1% Penicillin – Streptomycin (PS) (Gibco, cat. 15140–122), and 2% of Fetal Calf Serum (FCS – Hyclone, cat. SH30084-04). RSV-A2 was diluted to 1200 plaque-forming units (PFU/mL) in VGM with or without 10% guinea pig complement (Cedarlane, cat. CL5000) and added in a 1:1 ratio to diluted serum. After incubation at 37°C for 1.5 hours, 24-well plates containing confluent Vero cells were inoculated with this mixture and incubated for 1.5 hours at 37°C. Then, a methylcellulose overlay was added (0.75% methylcellulose (SIGMA, cat. 64632) in MEM (Gibco, Cat. 21430–020), 2% PS, 8 mM L-Glutamine (Gibco, cat.25030–032) and 0.2% NaHCO3 (SIGMA, cat. S8761)) and plates were incubated 5 days at 37°C, 5% CO2. Then, the overlay was removed, and the monolayers were fixed with ice-cold absolute methanol, washed with water, blocked with PBS-milk 5%, and stained with anti-RSV antibody conjugated to horse radish peroxidase (Abcam, cat. 20686). After 3 hours, the plates were washed with water and developed with TrueBlue HRP substrate (SeraCare, Cat.5510–0030). Stained plaques were counted using a dissecting microscope and neutralizing antibody titers were determined at the 60% reduction endpoint.
Avidity assay
Avidity assay directed against F protein was performed by ELISA. Microtiter plates (Greiner Bio-one, cat. 655061) were coated with 1 µg/mL of RSV F protein (SinoBiologicals, cat. 11049-V08B) in bicarbonate buffer (Sigma, cat. C3041). Plates were incubated overnight at 4°C and then blocked with PBS-Tween 0.05%-milk 5% for 1 h.
Serum were serially diluted in blocking buffer and dispensed over three dilutions to frame an OD of 1 (based on F-specific IgG ELISA results) in eight replicates (one for each urea concentration). After 1.5 hour incubation at 37°C, plates were washed with PBS-Tween 0.05% and urea treatment (Sigma, cat. 51456) was performed with different concentrations of urea (0, 2, 3, 4, 5, 6, 7 and 8 M; 100 µL/well) for 10 min at RT and the reaction was stopped by washing the plates. Then, plates were incubated for 1.5 hours at 37°C with a goat anti-monkey IgG-HRP diluted 1:10,000 (Biorad, cat. AAI42P). Plates were washed and incubated with TMB substrate (Tebu-bio, cat. TMB100-1000) for 30 min in the dark at RT. Colorimetric reaction was stopped with 1 N HCl (VWR Prolabo, cat 30024290). OD were measured at 450–650 nm on a Versamax plate reader (Molecular Devices), the percentage of antibody binding was determined for each urea concentration as follows: OD[No Urea]/OD[Urea]*100 and AUC (Area under curves) was determined on Graph Pad Prism.
Mucosal RSV F-specific IgG and IgA
RSV-F-specific IgA and IgG responses were assessed performed by ELISA on BAL samples. RSV-F was coated (SinoBiologicals, cat11049-V08B, 1 µg/mL) overnight on Maxisorb 96-well plates (Nunc, cat. 439454) at 4°C. The protein excess was eliminated and the plates were blocked with PBS-0.05% Tween – 5% nonfat dry milk for 1 h at 37°C. For IgG detection, BAL samples were initially diluted 1:2, and twofold serially diluted in blocking buffer and incubated for 1.5 hours at 37°C in the 96-well plates. For IgA detection, BAL were diluted 1:2 and incubated for 1.5 hours at 37°C. After PBS-0.05% Tween washes, specific IgG were directly detected after incubation with a goat anti-monkey γ-chain specific IgG HRP (Acris, cat. R1344) for 1.5 hours at 37°C. In parallel, specific IgA were detected after incubation with a goat anti-human/NHP IgA biotin (Mabtech, cat. 3830–4) for 1.5 hours at 37°C. After washes, streptavidin-HRP (Mabtech, cat. 3310–9) was employed (1 h at RT) for finalizing IgA detection. Plates were developed using TMB (Tebu-bio, cat. TMB100-1000) and stopped with 1 N HCl (VWR Prolabo, cat. 30024290). OD were read at 450 nm – 650 nm. RSV-F–specific IgG titers were quantified through an internal human RSV+ serum reference. The titer of this reference was previously calculated as the reciprocal dilution to obtain OD of 1. RSV-F-specific IgA were quantified as an OD value after subtraction of blank OD values and multiplying by the dilution factor. Samples with titers bellow the limit of quantification (LOQ = 0.4) were automatically given a value of 0.2 (half the LOQ).
F-specific IgG/IgA Memory B-cell ELISpots
F-specific B cell memory responses were evaluated after 5 days of PBMC polyclonal stimulation (R848 + IL-2) to allow quiescent memory B cells to differentiate into antibody-secreting cells (ASC). ASC frequency was then measured by the Human IgG/IgA FluoroSpot kit from Mabtech (cat. FS-05R24G-10) adapted to measure specific responses to the F antigen (SinoBio cat. 11049-V08B). Briefly, PBMCs were cultured in complete RPMI (Gibco, cat. 31870–025) containing 10% FCS, 200 mM L-Glutamine, 100 U/mL penicillin and 10 μg/mL streptomycin supplemented with R848 (1 μg/mL) and recombinant human IL-2 (10 ng/mL). After 5 days of culture, the cells were recovered, washed, and used in the FluoroSpot assay as described below.
MultiscreenTM 96-well IPFL plates (Millipore, cat. S5EJ104I07) were pre-wetted with 35% ethanol for 1 min, washed with sterile water, then with sterile PBS and coated overnight at 4°C with either the F antigen (SinoBio, cat. 11049-V08B) or a mix of capture mAbs (anti-human IgG mAb MT91/145 and anti-human IgA mAb MT57) to detect total IgG and IgA secreting cells. Plates were washed with PBS and blocked with complete medium for 1 h at 37°C. After washing with complete medium, PBMCs were counted, plated (F-specific wells: 4x105, 2x105 and 1x105 cells/well; total IgG/IgA wells: between 2,500 and 20,000 cells/well) and incubated for 5 hours at 37°C. Following washes in PBS containing 0.05% Tween20 and washes in PBS, the plates were incubated for 2 hours at 37°C with anti-human IgG-550 (MT78/145) or anti-human IgA-490 detection mAbs. After washing with PBS, fluorescent spots were enumerated with a spot reader equipped with filters for Cy3 and FITC fluorescence (Microvision). Percentages of F-specific IgG memory B cells were calculated over total IgG secreting cells.
RSV F-specific IFNγ/IL-2 FluoroSpots
FluoroSpot assay was performed using monkey IFNγ/IL-2 FluoroSpot kit from Mabtech (cat. FS-2122-10) to detect and enumerate cells secreting one or both cytokines. Briefly, MultiscreenTM 96-well IPFL plates (Millipore, cat. S5EJ104I07) were first pre-wetted with ethanol 35% for 1 minute at RT, washed with sterile PBS and coated overnight at 4°C with 100 µL of a mix of anti-monkey IFNγ and anti-IL-2 purified clones MT126L and MT2A91 (15 µg/mL), respectively. Wells were washed with sterile PBS, followed by a saturation step with complete RPMI (Gibco, Cat. 31870–025) containing 10% fetal calf serum, 200 mM L-Glutamine, 100 U/mL penicillin, and 10 μg/mL streptomycin for 2 hours at 37°C. After elimination of complete medium, 200,000 PBMCs were then added to each well with anti-human CD28 mAb (Mabtech, cat.3608-1-50) at 0.1 µg/mL as co-stimulator factor to circumvent IL-2 capture effects and incubated for 24 hours with 2 µg/mL of RSV F protein (Sino Biological, cat.11049100 _V08B), a positive control (anti-human CD3, Mabtech, cat.3605-1-250) or RPMI medium as unstimulated control. After washes in PBS 0.25% BSA, 100 µL of a mix of FITC-conjugated anti-IFNγ (7-B6-1-FS clone) and biotinylated anti-IL-2 (MT8G10 clone) were added for 2 hours at RT in the dark. After washes in PBS 0.25% BSA, 100 µL of a the mix of FITC/anti-FITC-490 antibody and SA-550 streptavidin was added to each well and incubated at RT for 1 h in the dark. Plates were further washed with PBS 0.25% BSA. Finally, the back of the plates was removed, and undersides of wells quickly rinsed with water. Plates were air-dried and stored in the dark until reading. Each spot corresponding to a single or double producer cell of IFNγ and/or IL-2 was enumerated with a fluorescent plate reader equipped with a filter for Cy3 and FITC fluorescence (Microvision). Spots obtained in the unstimulated control (RPMI medium alone) were subtracted from to the F-specific spots for each sample.
Responder thresholds were determined for each cytokine by calculating the average number of spots at baseline + 2 standard deviation. IFNγ responder threshold/106 PBMCs: 8 spots; IL-2 responder threshold: 11 spots; IFNγ/IL-2 double-positive responder threshold: 8 spots. Data bellow the responder threshold were automatically assigned a value of 5.
Statistical analysis
All parametric analyses were performed on SAS v9.4®, non-parametric analysis on EverStat6®. All the readouts were Log transformed.
The model’s residuals were studied to test the model’s validity (normality, extreme individuals). In case of heterogeneity in the results, it was taken into account in the model. A margin of error of 5% was used for effects of the factors.
For ELISA RSV F–specific binding antibodies titers and F-specific IgG titers in bronchoalveolar lavages (BALs), a 2-way Analysis Of Variance (ANOVA) with groups (adjuvanted and unadjuvanted) and time as fixed factors was performed. The repeated option was used to consider the pairing of the data across time. Depending on the data, the intra monkey variance covariance matrix was modeled using a compound symmetry (CS) or AR (1) structure. For comparisons to the unadjuvanted groups, Dunnett adjustment was performed. For comparison adjuvanted groups, Tukey adjustment was performed.
For PRNT, an Analysis of CoVariance (ANCOVA) with groups as category factor and time as continuous factor was performed. Time effect was analyzed with repeated measurements on monkeys. Concerning the modeling of the time, a quadratic model was used. The intra monkey variance covariance matrix was modeled using a CS structure.
For D25 – and palivizumab-competition assays, for memory B cell and T-cell responses, due to the non-normality of the data, non-parametric tests were performed to compare groups (Wilcoxon).
Correlation analyses were done between RSV F–specific binding antibodies (ELISA) and RSV-specific neutralizing antibodies (PRNT60) and between F-specific IgG titers in BALs and in sera. The RSV-A2 neutralizing and F-specific IgG titers in BALs were modeled using F-specific IgG titers and the time effect. The association between these two read-outs was carried out using analysis of covariance (ANCOVA) taking into account that observations by animal were paired along the time. To model the intra-animal variance/covariance matrix a CS matrix was used. The Pearson coefficient of correlation (r) was calculated from RSV-A2 neutralizing titers predicted by the above model adjusted by the time effect and F-specific IgG titers.
For correlation between RSV-A2 neutralizing titers and D25-competitive assay and between RSV-A2 neutralizing titers and palivizumab-competitive assay, Spearman coefficient of correlation (r) were calculated.
Results
SPA09 is well tolerated in Pre-F-NP-vaccinated macaques
pH and osmolality of the different formulations were found between 6–8 and 200–800 mOsm/kg respectively, indicating that formulations were compatible with intramuscular (IM) injections ().
Four groups of four cynomolgus macaques were immunized IM with Pre-F-NP adjuvanted with either SPA09(500 µg), SPA09(2000 µg), AF03 or without adjuvant on day 0 and on day 28 (). No unexpected deaths occurred during the study. However, one animal in group B (SPA09(2000 µg) – ) was euthanized on day 101 as it achieved humane endpoints. Histopathological analyses at autopsy revealed the presence of a parasite, Balantidum ssp., in large amount in the intestine that could be responsible for the animal’s health degradation.
Figure 1. Schematic representation of the study schedule. Four groups of four cynomolgus macaques were immunized intramuscularly with Pre-F-NP adjuvanted with either SPA09(500 µg), SPA09(2000 µg), AF03 or without adjuvant on day 0 (D0) and on day 28 (D28). Cellular and humoral immune responses were assessed at different time points over 6 months
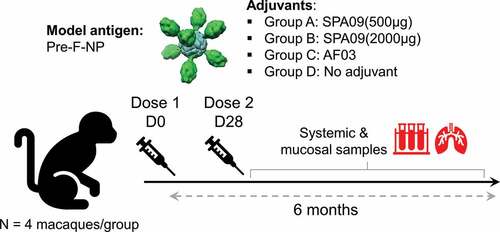
Vaccination site observations were performed for seven consecutive days post-prime and post-boost, respectively. The visual Draize score remained low: a score of 1 was reached, i.e. minimal erythema after the second injection, barely perceptible in one animal of group A: SPA09(500 µg). No adverse local reaction was observed after the two vaccinations. Random bleeding was sometimes observed just after administration with the occurrence of scab which resolved within the next days.
Body temperature was monitored weekly during the in vivo experimental phase and every day for 7 days post-immunizations. Individual body temperature monitoring is presented per group in . No adverse variations in body temperature were observed during the study period. From day 71 to 90 post-immunization, the animal that became sick in group B (SPA09(2000 µg)) presented a progressive decrease of body temperature correlated with a loss of body weight and health status deterioration described before. Other observed fluctuations post-immunizations were in the normal range in monkeys of this species and age.
Figure 2. Body temperature evolution. Body temperature was recorded using transponder chips at baseline and at regular intervals throughout the study. Individual monkey data are shown for each group (n = 4/group). Arrows = immunizations; dotted lines = body temperature normal range in cynomolgus macaques
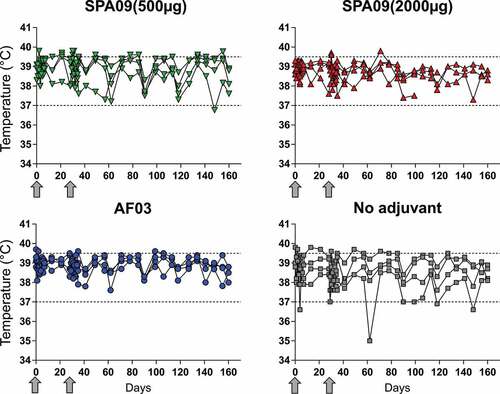
Blood samples for biochemistry were taken on day 0, 2, 28, and 30 post-immunization. The evolution of CRP, globulin, albumin, and total bilirubin per group is depicted in Supplementary Fig. 1.
Elevation of CRP above normal range (0–2.82 mg/L) was observed at day 2 post dose 1 in two animals of the AF03 (squalene emulsion) group but not in other groups. On day 28 (before dose 2), elevation of CRP above normal range was observed for one animal in the non-adjuvanted group, in two animals in the SPA09(2000 µg) and AF03 groups and in three animals of the SPA09(500 µg) group which came back to baseline on day 30 (2 days post-dose 2). However, some animals were above normal range pre-vaccination, so those observations may not be treatment-related. Other blood biochemistry parameters were in normal range.
No marked variations of red blood cell count and hemoglobin were observed during the study. Mild neutrophilic leukocytosis was observed for two animals from group SPA09(2000 µg) on day 2 and for three animals on day 30, while neutrophil count was within normal range on baseline samples. Mild neutrophilic leukocytosis was sporadically observed, regardless of groups. No significant variation of monocyte and eosinophil count was observed during the study.
SPA09 increases systemic humoral responses in a dose-dependent manner
Only adjuvanted formulations elicited both RSV F-specific binding antibodies (ELISA) and RSV-specific neutralizing antibodies, as quantified by 60% plaque reduction neutralization test (PRNT60) ( and B and Supplementary Fig. 3).
Figure 3. Humoral immune responses to the Pre-F-NP vaccines. (A) RSV F-specific IgG ELISA titers, (B) RSV-A2 neutralizing titers (PRNT60) without and with complement following intramuscular vaccination of four cynomolgus macaques without adjuvant or with: SPA09(500 µg), SPA09(2000 µg) or AF03 on day 0 and day 28 (**P-value < 0.01; ***P-value < 0.001; NS = Not Significant). (C) Correlation of PRNT60 and F-specific IgG ELISA titers. Correlation was observed at all time points, in all vaccine groups. P-value for Pearson rank-correlation test. Line = linear regression; dotted lines = 95% confidence intervals. (D) Antibody avidity. The avidity of serum F-specific IgG was assessed at day 28, day 49, day 91 and day 161 post-immunizations using urea as the chaotropic agent. The avidity area under curve (AUC) was calculated. GMT = Geometric Mean Titers; SD = Geometric Standard Deviation; dotted lines = limit of quantification; arrows = vaccination on day 0 and day 28
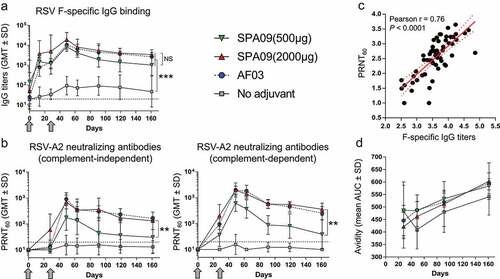
F-specific IgG titers were significantly higher in the adjuvanted groups than in the nonadjuvanted group (~100-fold) at all tested time points post-immunization (P-value <0.01) and peaked at day 49 (3 weeks post-dose 2). SPA09(2000 µg) tended to be more efficient than SPA09(500 µg) at inducing F-specific antibodies; however, this trend did not reach statistical significance ().
RSV-A2 virus neutralizing antibodies (complement-dependent and complement-independent) were only induced by adjuvanted formulations (P-value <0.05) (). SPA09(2000 µg) induced significantly higher (~10-fold) neutralizing titers than SPA09(500 µg) (P-value <0.05). No significant differences were shown between SPA09(2000 µg) and AF03. In those two groups, the PRNT60 titers significantly increased after dose 2 (P-value <0.001) peaked on day 49 (geometric mean titers: ~800 without complement and ~2000 with complement) and stabilized at day 91 (geometric mean titers: ~400 without complement and ~600 with complement).
Responders were defined as animals with a ≥ threefold rise over the assay limit of detection (LOD) of 20 (i.e. post-dose titers ≥60). Applying this criterion, in presence of complement, the SPA09(2000 µg) and AF03 groups reached a 100% seroconversion rate by day 28 (). In contrast, the SPA09(500 µg) group showed only 50% responders on day 28. In the absence of complement, only groups corresponding to SPA09(2000 µg) and AF03 reached a 100% seroconversion rate at day 49, when SPA09(500 µg) had 3 out of 4 responders. At the end of the study (day 161 = 5 months post-dose 2), SPA09(2000 µg) still had 100% responders, while the rate of responders in the SPA09(500 µg) group was only 25% (1 out of 4 macaques).
Table 2. RSV-A2-specific functional antibody responders among Pre-F-NP vaccinated NHPs. Responder animals were defined as animals with a ≥ threefold rise over the assay limit of detection (LOD) of 20 (post-dose titers ≥60). N = 4 animals/groups
Binding antibody titers correlated with neutralizing antibody titers in the vaccinated animals for all vaccine groups and at all time-points (Pearson r = 0.76; P-value <0.0001; ).
Antibody avidity is an important measurement of the strength with which an antibody can interact with its cognate antigen. Thus, we wanted to test whether the observed differences in the magnitudes of the systemic antibody responses that were dependent on the vaccine adjuvant would also be reflected in antibody avidity. Overall, the antibody avidity increased over time in all groups, with only a trend for higher avidity in the adjuvanted groups compared to the non-adjuvanted group; however, this trend was not statistically significant (P-value >0.05 – ).
SPA09(2000 µg) elicits antibodies to the highly neutralizing epitope site Ø of the RSV pre-F
There are five major neutralizing sites (Ø, II, III, IV, and V) on the surface of the RSV pre-F glycoprotein, and the most neutralization sensitive is at the apex (site Ø) ().Citation12 Site Ø is only present on prefusion F and is recognized by the D25 monoclonal antibody.Citation13 Site II is neutralization-sensitive and targeted by the palivizumab (SYNAGIS) licensed to prevent RSV infection in infants.Citation14
Figure 4. Epitope specificity of antibody responses. (A) Localization of site Ø and site II on prefusion F. Serum IgG antibodies were tested by competitive ELISA at baseline, day 49 and day 161 for specificity to (B) site Ø and (C) site II epitopes on Pre-F. Shown are individual values, with bars representing median ± 95% confidence interval in each of the vaccine groups. *P-value < 0.05. NS = not significant. Correlation between PRNT60 and D25-competitive assay (D) and palivizumab-competitive assay (E)
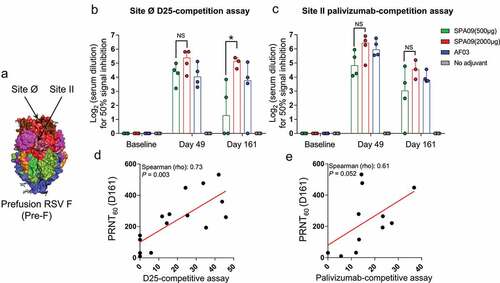
Using D25- and palivizumab-competitive ELISAs, we demonstrated that all adjuvanted formulations elicited a pre-F-specific response in macaques. Moreover, SPA09(2000 µg) induced significantly higher site Ø-specific antibodies than SPA09(500 µg) on day 161 (). No significant differences were observed between SPA09(2000 µg) and AF03. For all adjuvanted groups, no significant differences were observed for site II-specific antibodies (). D25- and palivizumab-competitive ELISA correlated with neutralizing titers ( and E).
Transudation of F-specific IgGs from blood to the airways
It has been shown that preexisting RSV-specific nasal antibodies (IgG and IgA) correlate with protection against RSV infection in human.Citation15–17 Mucosal F-specific IgG were detected in bronchoalveolar lavages of immunized macaques (). SPA09(2000 µg) induced significantly higher mucosal IgG titers than the SPA09(500 µg) formulation (P-value <0.01) and equivalent titers compared to AF03. Those IgG responses did correlate with serum IgG titers (r = 0.81) suggesting a possible transudation of systemic IgG to mucosal surfaces of the airways (). In contrast, no F-specific IgA were detected in those samples (Supplementary Fig. 4).
Figure 5. F-specific IgG titers in bronchoalveolar lavages (BALs). IgG ELISA were performed on BAL samples at baseline and several timepoints following intramuscular vaccination of four cynomolgus macaques without adjuvant or with: SPA09(500 µg), SPA09(2000 µg) or AF03 on day 0 and day 28 (**P-value < 0.01). Individual monkey data are shown for each group (n = 4/group). Dotted lines = limit of quantification; arrows = vaccination on day 0 and day 28
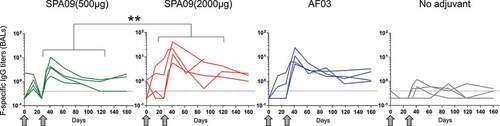
SPA09 induces specific memory B cells and T-cell immunity in a dose-dependent manner
The success of humoral memory depends on at least two layers of defense: preexisting protective antibodies secreted by long-lived plasma cells as a first line of defense (constitutive humoral memory) and pathogen-experienced memory B cells as a second line of defense that can be rapidly reactivated to produce antibodies (reactive humoral memory).Citation18,Citation19 Having assessed circulating antibody responses, we then assessed circulating memory B cells. F-specific memory B cells were quantified by ELISpot assay in peripheral blood mononuclear cells (PBMCs) collected from the immunized macaques on day 119 and day 161 after the first injection to assess the vaccine-induced long-term immune responses. F-specific IgG memory B cells were significantly detected at day 161 in SPA09(2000 µg) and AF03 groups, at similar levels (geometric means ranging from: 0.009 to 0.02% of total IgG-secreting cells) but not in SPA09(500 µg) and the non-adjuvanted groups ().
Figure 7. F-specific IgG memory B-cell ELISpot responses in PBMCs from immunized macaques. F-specific memory B cell ELISpot results at baseline, day 119 and day 161 following intramuscular vaccination of cynomolgus macaques with Pre-F-NP adjuvanted or not on day 0 and day 28. Bars = Geometric mean; dotted line = responder threshold; **P-value < 0.01
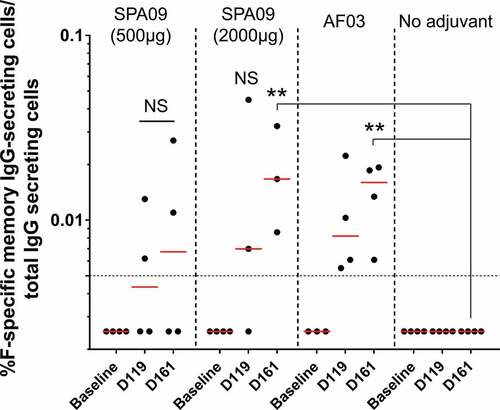
CD4+ T cells exert essential helper functions and are critical for B-cell activation and differentiation.Citation20 Interferon (IFN)-γ and interleukin (IL)-2 were selected to analyze TH1 immune responses. SPA09(2000 µg) vaccinated monkeys developed strong cellular immune responses to F with 100% macaques above the responder threshold at day 7, as shown by IFNγ and IL-2 ELISpot assays one week after the first and second injection (). SPA09(2000 µg) induced significantly higher IFNγ and IL-2 responses than SPA09(500 µg), AF03 and the non-adjuvanted groups (P-value <0.01). On day 35, IFNγ spot-forming cells (SFC) ranged from 300 to 1000 per million PBMCs () and IL-2 SFCs ranged from 400 to 1500 per million PBMCs (). IFNγ/IL-2 double-positive cells were also measured between 150 and 500 SFC per million PBMCs ().
Figure 8. Characterization of cellular immune responses in macaques following vaccination. F-specific T-cell ELISpot responses at day 7 (7 days post-dose 1) and day 35 (7 days post-dose 2) in PBMCs from immunized macaques. (A) IFNγ ELISpot results. (B) IL-2 ELISpot results. (C) IFNγ/IL-2 double positive. Bars = Geometric mean; dotted line = responder threshold; ** P-value<0.01
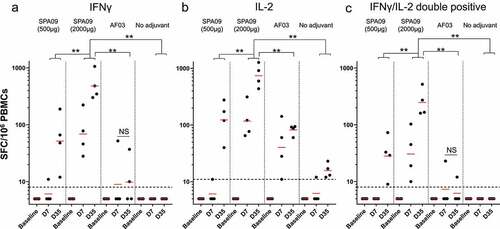
Discussion
Technologies such as “reverse vaccinology”Citation21 immunoproteomicsCitation22,Citation23 and other omics technologiesCitation24 offer rapid access to protein vaccines with antigen precision and good safety profiles. However, such antigens are often poorly immunogenic and need to be combined with adjuvants to improve their immuno-protective properties against the target disease. To enlarge the panel of adjuvants that could be suitable for human use, we recently introduced a novel PAA adjuvant called SPA09 based on the long-standing history of Carbomer (or Carbopol) in the field of veterinary vaccine adjuvants. While carbomer adjuvants are typically based on very high molecular weight randomly crosslinked PAAs (>106 Da), SPA09 is made of straight PAAs with a molecular weight in the range of 350–650 kDa. SPA09 has the advantage over Carbomers of being better defined (no random crosslinking), less viscous and easier to sterilize by filtration.
We have previously reported that SPA09 was a potent adjuvant in mice, able to induce high antibody titers and TH1-type cellular-mediated immunity even in the absence of interaction with the vaccine antigen.Citation8 In this regard, SPA09 may differ from other polyanionic polymer adjuvants, such as the biodegradable poly[di(carboxylatophenoxy)phosphazene] (PCPP), which has been described as forming water soluble, self-assembled protein-polymer complexes upon mixing with vaccine antigens.Citation25,Citation26 Polyphosphazenes were studied in different human and veterinary vaccine programs where they generally promoted robust adjuvant effects with a balanced TH1/TH2 profile.Citation7 One such study using PCPP for the adjuvantation of a soluble form of the postfusion RSV-F glycoprotein in mice indicated that PCPP could spontaneously self-assemble with the vaccine antigen, and enhance both TH1 immune responses and protection from RSV challenge, in comparison to a standard aluminum adjuvant.Citation27 Another study using PCPP for the intranasal delivery into mice of a formalin-inactivated bovine RSV (FI-bRSV) vaccine combined with immunostimulatory CpG oligodeoxynucleotides indicated that the FI-bRSV/CpG/PCPP co-formulation could induce virus neutralizing antibody responses in the serum, IFN-γ responses in the spleen and mucosal IgA responses in the lung, as well as protection from bRSV challenge.Citation28, Citation29, Citation30
These studies highlight the potential of such polyanionic adjuvants, which are also advantageous in terms of readiness of supply, easiness of formulation by simple mixing processes and quality control by using nuclear magnetic resonance (NMR) and high-performance size exclusion chromatography (HPSEC) techniques.Citation8
The aim of the present study was to confirm the adjuvant effect of SPA09 in a higher animal species closest to human, i.e. NHP. Confirming the adjuvant effect of SPA09 in macaques was deemed important before considering clinical application and investigating its mechanism of action. Another aim of the present study was to assess the optimal dosage of SPA09. Previous studies in mice, horses and dogs suggested that SPA09 would be at least as active as the high molecular weight Carbopol 974P used in marketed equine vaccines at a dose of 4000 µg (e.g. ProteqFlu-Te®).Citation31 A dose of 2000 µg was then selected as a reasonable high dose for humans after this dose was found to be safe and active following three consecutive IM injections in the rabbit, the usual species for vaccine/adjuvant safety evaluation.Citation32
In the present immunogenicity study in macaques, no safety signal was observed in any of the vaccine groups, based on clinical observations, blood biochemistry and complete blood counts. Mild neutrophilic leukocytosis was sporadically observed, regardless of groups. As this modification was observed even in baseline samples and/or was a sporadic modification, it may correspond to a “stress leukogram”, caused by stress experienced by animals during blood sampling and/or administration. However, one animal that received RSV Pre-F-NP + SPA09(2000 µg) had to be euthanized as it achieved humane endpoints. An autopsy was performed, and histopathological analyses revealed the presence of a parasite, Balantidum ssp., in large amount in the intestine that could be responsible for the animal’s health degradation.
Adjuvanted Pre-F-NP induced functional neutralizing antibodies against the homologous RSV-A2 strain, with SPA09(2000 µg) being significantly more potent than SPA09(500 µg), and as effective as AF03 (2.5% squalene emulsion). Serum neutralizing antibody responses remained detectable up to at least 5 months post-dose 2, demonstrating response longevity (referred to as constitutive humoral memory).Citation19 Those circulating protective antibodies secreted by long-lived plasma cells (mainly located in the bone marrow) function as a first line of defense against infection if their levels in blood or at the site of infection are sufficiently high.Citation18,Citation33 Neutralizing titers obtained in our study were in the same range as than those observed in a study in Rhesus macaques two weeks post-immunization with the prototype RSV prefusion F antigen DS-Cav1 mixed with 500 μg of poly I:C adjuvant,Citation13 and higher than those observed in cynomolgus macaques immunized with an RSV soluble fusion protein (RSV sF, a postfusion form) adjuvanted with glucopyranosyl lipid A (GLA) in an oil-in-water emulsion (stable emulsion [SE]).Citation34 Although titers could not be strictly compared as the assays were not strictly identical.
Moreover, SPA09(2000 µg) induced F-specific IgG-secreting memory B cells in blood (reactive humoral memory)Citation19 at similar levels as AF03, while SPA09(500 µg) did not elicit significant levels of memory B cells. These pathogen-experienced memory B cells induced by vaccination do not constitutively secrete antibodies, but might have a critical role to reactivate the production of neutralizing antibodies upon exposure to the pathogen if circulating neutralizing antibodies are not high enough to confer protection.Citation18,Citation19
Interestingly, mucosal F-specific IgGs were detected in bronchoalveolar lavages of immunized macaques. Those IgG responses did correlate with serum IgG titers suggesting that SPA09 induced sufficiently high titers in sera to allow transudation to mucosal surfaces of the airways. Of note, we visually checked that there was not blood contamination of the BALs, however, we cannot completely exclude this possibility. In contrast, no F-specific IgA were detected. Those observations are not surprising as the immunization route was IM, generally described as a weak inducer of mucosal immunity.Citation35
SPA09 was found to be more effective than AF03 at increasing the IL-2 and IFNγ secreting cell frequencies in PBMCs collected 7 days after each immunization, confirming its TH1-oriented profile. Those F-specific IFNγ responses were in the same range as those observed in a study using RSV sF + GLA-SE in cynomolgus macaques.Citation34 Of note, the 2000-µg dose of SPA09 was found to be more potent than the 500-µg dose at increasing IL-2 and IFNγ responses and both serum and local (BAL) antibody responses, confirming a dose-effect relationship.
In this study, we were able to confirm the ability of SPA09 to promote a strong and sustained functional humoral and T cell-mediated immune responses in the macaque model. Thus, SPA09 appears as a promising adjuvant candidate for inclusion in vaccines against infectious diseases for which humoral and/or IFNγ-mediated cellular responses are associated with protection.Citation33 Such vaccines would include vaccines against Tuberculosis,Citation36 pathogens with latent disease phase such as ZosterCitation37 and vaccines against pathogens with complex life cycles such as Malaria.Citation38
Regarding the mechanism of action, our previous studies using SPA09 with the CMV-gB antigen suggested that SPA09 could exert adjuvant activity without apparent interaction with the vaccine antigen.Citation8 This feature remains to be assessed for the Pre-F-NP and other antigens. Indeed, the polyelectrolyte adjuvants are generally described as dual-functionality adjuvants integrating immunostimulatory and delivery modalities, the latter depending strongly on the ability of the antigen to interact with the adjuvant.Citation7 Since it was shown that antigen-polymer association could play an important role in promoting or increasing the adjuvant effects of polyanionic polymers including polyacrylates,Citation39 studies are underway to fine-characterize and try enhancing the interactions between vaccine antigens and SPA09.
Although the mechanistic studies are not complete yet, early results obtained in vitro and in mice confirmed the ability of SPA09 to strongly stimulate the innate immune system in a TLR-independent manner. Transcriptomic and proteomic analyses evidenced the upregulation of genes encoding cytokines and chemokines and their receptors, genes encoding pathogen-recognition receptors and genes associated with the MHC-I, the inflammasome and type-I interferon pathways in the muscle and draining lymph nodes (dLNs) within hours following IM injection of SPA09. Immunohistochemistry and FACS analyses confirmed that SPA09 promoted an influx of antigen-presenting cells in the injected muscle and in the dLNs and supported the formation of germinal centers in the dLNs of immunized mice. Experiments comparing the adjuvant effect of SPA09 in wild type and a series of knockout (KO) mice revealed that the TH1 adjuvant activity of SPA09 was essentially dependent on the MyD88 and type I interferon pathway and to a lesser degree on the mitochondrial antiviral-signaling protein (MAVS) pathway. These mechanistic studies are currently under completion and will be published in detail in a follow up publication.
With respect to the development of an adjuvanted recombinant protein RSV vaccine, although high virus neutralizing titers and TH1-biased immune responses as such induced by the SPA09-adjuvanted Pre-F-NP candidate are generally associated with protection in preclinical challenge models,Citation12 it remains to be seen if this translates into humans. Early clinical trials will also be key to confirm the good safety profile observed so far with SPA09 in the present NHP study and other preclinical studies.
Conclusion
In conclusion, SPA09 was found to be a potent adjuvant in several preclinical species including mice, dogs, pigs, and horses (WO2017218819A1). The present NHP study can be considered as an ultimate preclinical step confirming the adjuvant effect of SPA09 in a human-relevant animal model and supporting its progression into clinical evaluation. Studies are in progress in our group to confirm the adjuvant effect of SPA09 in humans and to elucidate the mechanism of this adjuvant effect.
Author contributions
VP, FB, and JH conceived and designed the study. HB, FG, and SM executed the experiments. All authors analyzed, interpreted, discussed the data, and wrote the paper and participated in manuscript revisions.
Disclosure of potential conflicts of interest
The authors were all employees of Sanofi Pasteur at the time of this study and hold company stocks.
Supplemental Material
Download Zip (4.2 MB)Acknowledgments
We thank Marie Garinot, Sandrine Cigarini, Laurent Revet for providing and characterizing the SPA09 and AF03 adjuvants and formulations. We thank Sylviane Gautheron, Julie Piolat and Joseline Ruiz for her contribution to the statistical analysis of the results. We also thank Bachra Rokbi, Fred Vogel (Sanofi Pasteur) and Anirban Sanyal (Sanofi) for critical review of the manuscript and for editorial assistance and manuscript coordination.
Supplementary material
Supplemental data for this article can be accessed on the publisher’s website.
Additional information
Funding
References
- Reed SG, Orr MT, Fox CB. Key roles of adjuvants in modern vaccines. Nat Med. 2013;19:1597–608. doi:10.1038/nm.3409.
- Nanishi E, Dowling DJ, Levy O. Toward precision adjuvants: optimizing science and safety. Curr Opin Pediatr. 2020;32:125–38. doi:10.1097/MOP.0000000000000868.
- Bonam SR, Partidos CD, Halmuthur SKM, Muller S. An overview of novel adjuvants designed for improving vaccine efficacy. Trends Pharmacol Sci. 2017;38:771–93. doi:10.1016/j.tips.2017.06.002.
- Knudsen NP, Olsen A, Buonsanti C, et al. Different human vaccine adjuvants promote distinct antigen-independent immunological signatures tailored to different pathogens. Sci Rep. 2016;6:19570. doi:10.1038/srep19570.
- Regelson W. The biologic activity of polyanions: past history and new perspectives. J Polym Sci. 1979;66:483–538.
- MI M. Polyelectrolytes in immunology: fundamentals and perspectives. Turk J Chem. 1996;20:126–38.
- Powell BS, Andrianov AK, Fusco PC. Polyionic vaccine adjuvants: another look at aluminum salts and polyelectrolytes. Clin Exp Vaccine Res. 2015;4:23–45. doi:10.7774/cevr.2015.4.1.23.
- Garinot M, Piras-Douce F, Probeck P, Chambon V, Varghese K, Liu Y, Luna E, Drake D, Haensler J. A potent novel vaccine adjuvant based on straight polyacrylate. Int J Pharm. 2020;2:100054.
- Thompson EA, Lore K. Non-human primates as a model for understanding the mechanism of action of toll-like receptor-based vaccine adjuvants. Curr Opin Immunol. 2017;47:1–7. doi:10.1016/j.coi.2017.06.006.
- Swanson KA, Rainho-Tomko JN, Williams ZP, et al. A respiratory syncytial virus (RSV) F protein nanoparticle vaccine focuses antibody responses to a conserved neutralization domain. Sci Immunol. 2020;5. doi:10.1126/sciimmunol.aba6466
- Klucker MF, Dalencon F, Probeck P, Haensler J. AF03, an alternative squalene emulsion-based vaccine adjuvant prepared by a phase inversion temperature method. J Pharm Sci. 2012;101:4490–500. doi:10.1002/jps.23311.
- Graham BS. Immunological goals for respiratory syncytial virus vaccine development. Curr Opin Immunol. 2019;59:57–64. doi:10.1016/j.coi.2019.03.005.
- McLellan JS, Chen M, Joyce MG, et al. Structure-based design of a fusion glycoprotein vaccine for respiratory syncytial virus. Science. 2013;342:592–98. doi:10.1126/science.1243283.
- Geskey JM, Thomas NJ, Brummel GL. Palivizumab: a review of its use in the protection of high risk infants against respiratory syncytial virus (RSV). Biologics. 2007;1:33–43.
- Habibi MS, Jozwik A, Makris S, Dunning J, Paras A, DeVincenzo JP, de Haan CA, Wrammert J, Openshaw PJ, Chiu C. Impaired antibody-mediated protection and defective IgA B-cell memory in experimental infection of adults with respiratory syncytial virus. Am J Respir Crit Care Med. 2015;191:1040–49. doi:10.1164/rccm.201412-2256OC.
- Vissers M, Ahout IM, de Jonge MI, Ferwerda G. Mucosal IgG levels correlate better with respiratory syncytial virus load and inflammation than plasma IgG levels. Clin Vaccine Immunol. 2015;23:243–45. doi:10.1128/CVI.00590-15.
- Walsh EE, Falsey AR. Humoral and mucosal immunity in protection from natural respiratory syncytial virus infection in adults. J Infect Dis. 2004;190:373–78. doi:10.1086/421524.
- Akkaya M, Kwak K, Pierce SK. B cell memory: building two walls of protection against pathogens. Nat Rev Immunol. 2020;20:229–38. doi:10.1038/s41577-019-0244-2.
- Kurosaki T, Kometani K, Ise W. Memory B cells. Nat Rev Immunol. 2015;15:149–59. doi:10.1038/nri3802.
- Plotkin SA, Orenstein WA, Offit PA, Edwards KM. Plotkin’s Vaccines. Elsevier; 2018. https://www.elsevier.com/books/T/A/9780323357616
- Sette A, Rappuoli R. Reverse vaccinology: developing vaccines in the era of genomics. Immunity. 2010;33:530–41. doi:10.1016/j.immuni.2010.09.017.
- Altindis E, Tefon BE, Yildirim V, Ozcengiz E, Becher D, Hecker M, Özcengiz G. Immunoproteomic analysis of Bordetella pertussis and identification of new immunogenic proteins. Vaccine. 2009;27:542–48. doi:10.1016/j.vaccine.2008.11.020.
- Dennehy R, McClean S. Immunoproteomics: the key to discovery of new vaccine antigens against bacterial respiratory infections. Curr Protein Pept Sci. 2012;13:807–15. doi:10.2174/138920312804871184.
- Holtfreter S, Kolata J, Stentzel S, Bauerfeind S, Schmidt F, Sundaramoorthy N, Bröker BM. Omics approaches for the study of adaptive immunity to staphylococcus aureus and the selection of vaccine candidates. Proteomes. 2016;4. doi:10.3390/proteomes4010011
- Payne LG, Andrianov AK. Protein release from polyphosphazene matrices. Adv Drug Deliv Rev. 1998;31:185–96. doi:10.1016/S0169-409X(97)00122-1.
- Payne LG, Jenkins SA, Andrianov A, Roberts BE. Water-soluble phosphazene polymers for parenteral and mucosal vaccine delivery. Pharm Biotechnol. 1995;6:473–93.
- Cayatte C, Marin A, Rajani GM, Schneider-Ohrum K, Snell Bennett A, Marshall JD, Andrianov AK. PCPP-adjuvanted respiratory syncytial virus (RSV) sF subunit vaccine: self-assembled supramolecular complexes enable enhanced immunogenicity and protection. Mol Pharm. 2017;14:2285–93. doi:10.1021/acs.molpharmaceut.7b00118.
- Mapletoft JW, Oumouna M, Kovacs-Nolan J, Latimer L, Mutwiri G, Babiuk LA. Intranasal immunization of mice with a formalin-inactivated bovine respiratory syncytial virus vaccine co-formulated with CpG oligodeoxynucleotides and polyphosphazenes results in enhanced protection. J Gen Virol. 2008;89:250–60. doi:10.1099/vir.0.83300-0.
- Andrianov AK, Marin A, Chen J. Synthesis, properties, and biological activity of poly[di(sodium carboxylatoethylphenoxy)phosphazene]. Biomacromolecules. 2006;7:394–99. doi:10.1021/bm050790a.
- Dilai M, Piro M, El Harrak M, Fougerolle S, Dehhaoui M, Dikrallah A, Legrand L, Paillot R, Fassi Fihri O. Impact of mixed equine influenza vaccination on correlate of protection in horses. Vaccines (Basel). 2018;6,71. doi:10.3390/vaccines6040071
- Baldrick P. Dose site reactions and related findings after vaccine administration in safety studies. J Appl Toxicol. 2016;36:980–90. doi:10.1002/jat.3314.
- Plotkin SA. Correlates of protection induced by vaccination. Clin Vaccine Immunol. 2010;17:1055–65. doi:10.1128/CVI.00131-10.
- Patton K, Aslam S, Shambaugh C, Lin R, Heeke D, Frantz C, Zuo F, Esser MT, Paliard X, Lambert SL. Enhanced immunogenicity of a respiratory syncytial virus (RSV) F subunit vaccine formulated with the adjuvant GLA-SE in cynomolgus macaques. Vaccine. 2015;33:4472–78. doi:10.1016/j.vaccine.2015.07.025.
- Pavot V, Rochereau N, Genin C, Verrier B, Paul S. New insights in mucosal vaccine development. Vaccine. 2012;30:142–54. doi:10.1016/j.vaccine.2011.11.003.
- Ellner JJ, Hirsch CS, Whalen CC. Correlates of protective immunity to Mycobacterium tuberculosis in humans. Clin Infect Dis. 2000;30(Suppl 3):S279–82. doi:10.1086/313874.
- Cunningham AL, Levin MJ. Herpes zoster vaccines. J Infect Dis. 2018;218:S127–S33. doi:10.1093/infdis/jiy382.
- Ferreira MU, da Silva Nunes M, Wunderlich G. Antigenic diversity and immune evasion by malaria parasites. Clin Diagn Lab Immunol. 2004;11:987–95. doi:10.1128/CDLI.11.6.987-995.2004.
- Mustafaev MI, Yucel F, Ozturk S, Cirakoglu B, Bermek E. Cu(2+)-mediated complex formation between polyacrylic acid (PAA) and bovine serum albumin. J Immunol Methods. 1996;197:31–37. doi:10.1016/0022-1759(96)00107-X.
- Andrianov AK, Marin A, Fuerst TR. Molecular-level interactions of polyphosphazene immunoadjuvants and their potential role in antigen presentation and cell stimulation. Biomacromolecules. 2016;17:3732–42. doi:10.1021/acs.biomac.6b01251.